Abstract
The evolution of articulated sclerites via soft membranes, termed arthrodization, is arguably one of the most critical innovations in animals. Defining the megaphylum Arthropoda, the arthrodization of appendages, or arthropodization, likely predated that of the body, the combination of both being diagnostic of true arthropods (Euarthropoda) – all of these innovations occurring during the Cambrian explosion. Here, thanks to dozens of exceptionally preserved fossils from the Cambrian Wuliuan Stage Burgess Shale (Tulip Beds locality on Mount Stephen, British Columbia, Canada), we show that a distinct but comparable system of imbricated sclerotic elements evolved in the paraphyletic sister group of arthropods, the lobopodians. Entothyreos synnaustrus gen. et sp. nov. has characteristic body plan features of the Collinsovermidae (order Luolishaniida), including anterior limbs for suspension-feeding and stout anchoring posterior limbs. Uniquely, however, E. synnaustrus also displays segmental sclerotic sheets along the trunk, covered in a thin layer of integument, as well as overlapping sclerotized annuli on posterior-most limbs. While the latter elements likely served a protective function, the dorsolateral trunk sheets, which also carry spines, may have facilitated body erection and suspension-feeding. Other luolishaniids possess separate ring-like structures connecting the base of metameric spines which are covered by the apical layer of the lobopodian integument. E. synnaustrus and related taxa illustrate, therefore, an arguably parallel evolution of arthropod-like morphoanatomical features early during the rise of panarthropods. This finding broadens our perspectives on the uniqueness of major synapomorphies and the importance of including canalization in macroevolutionary narratives.
http://zoobank.org/urn:lsid:zoobank.org.pub:D4A01784-E587-481A-AB04-A812B4AAE422
Introduction
Arthrodization refers to the articulation of cuticular sclerites via flexible membranes (called arthrodial membranes), a property that is pivotal to the colossal evolutionary and ecological success of arthropods since the early Cambrian (Aria, Citation2022; Boxshall, Citation2004; Budd, Citation2001; Giribet & Edgecombe, Citation2017). Advances in palaeontology have revealed that the arthrodization of limbs (called arthropodization), specifically that of the frontal-most appendages, evolved separately from the arthrodization of the rest of the body, involving dorsal (tergites), lateral (pleurae) and ventral (sternites) chitinous sclerites. Full ring-like units of the arthropod appendages – the podomeres – are structured differently from body segments (Boxshall, Citation2004), as emphasized by their developmental (Wolff & Scholtz, Citation2008) and neuromuscular (Wolf & Harzsch Citation2002) characteristics, further justifying this distinction. Nevertheless, the external manifestation of such structures, as sclerites joined by arthrodial membranes, is the same, and therefore arthrodization can also reasonably be used in a general sense to designate the same articulating morphological feature.
Arthropoda has traditionally been defined by the presence of arthrodization (Bergström et al., Citation2008), and recent findings regarding the relationships of early pan- and euarthropods support such a nomenclatural decision (Aria, Citation2019). Accordingly, Arthropoda would be defined by the appearance of the first arthrodized appendages in the disparate radiodontans, which otherwise have segmented but unarthrodized bodies (e.g. Moysiuk & Caron, Citation2019). The acquisition of body arthrodization in the closely related megacheirans (Aria et al., Citation2020; Zeng et al., Citation2020) would then define the ‘true’ arthropods, Euarthropoda (Aria, Citation2019).
However, it remains unclear whether these conditions emerged suddenly, as it currently appears, or whether there existed intermediary steps. In recent phylogenies, unsclerotized segments are evolutionarily replaced by jointed sclerites, and lobopodous, annulated limbs are replaced by articulating podomeres (Aria & Caron, Citation2015; Aria et al., Citation2020; Edgecombe, Citation2020; Zeng et al., Citation2020). Sclerotic elements are present in the paraphyletic group giving rise to Arthropoda, the lobopodians, which, in Cambrian ecosystems, were relatively rare but diversified relatives of onychophorans and tardigrades (Caron & Aria, Citation2017; Smith & Ortega-Hernández, Citation2014). However, the homology of head and body sclerites between ‘armoured’ lobopodians and arthropods, specifically radiodontans, has been difficult to ascertain, because intermediary taxa, notably xenusiids, lack sclerites (Caron & Aria, Citation2020a, Citationb). It had been proposed that Xenusion had dorsal sclerites (Dzik & Krumbiegel, Citation1989), but the thick serial lobes thus interpreted are now well documented to be digestive glands in sister taxa (Vannier et al., Citation2014). Certain lobopodians had developed hypertrophied and sclerotized annuli resembling podomeres (Liu et al., Citation2011), but those were arguably not arthrodized (Aria & Caron, Citation2015; Ma et al., Citation2013) and of a different origin than bona fide sclerites in other taxa. The significance of lobopodian sclerites in the context of panarthropod evolution and the genetic-developmental origin of arthrodization therefore remain open questions.
We present here exceptionally preserved fossil evidence of a previously undescribed collinsovermid from the Tulip Beds locality of the Burgess Shale, Cambrian Series 3, Wuliuan stage. Sclerotic elements along both the body and limbs of this new species, comparable to arthrodized elements, provides a new impetus to discuss the origin of arthrodization and its evolutionary significance.
Material and methods
Fossil material
The Tulip Beds (S7 locality in Fletcher & Collins, Citation2003) was discovered in 1983 by the Royal Ontario Museum, with the first specimens of this new lobopodian collected in 1989. The bulk of the material comes from subsequent field collections in 1996, 2000, 2008 and 2016, with a total of 51 specimens of this new species recovered to date. The Tulip Beds site has so far yielded about 10,000 collected specimens representing a rich array of soft-bodied organisms, including its namesake species, the ‘tulip’ animal, Siphusauctum gregarium (O’Brien & Caron, Citation2012). The Tulip Beds belongs to the base of the Campsite Cliff Shale Member, one of the oldest members of the ‘thick’ Burgess Shale Formation (Fletcher & Collins, Citation1998). The depositional setting of the Tulip Beds suggests a more distal palaeoenvironment from the edge of the Cathedral Escarpment compared to the nearby Walcott Quarry, but otherwise shares similar taphonomic pathways with fossils being buried by rapid mud-flow deposits (O’Brien et al., Citation2014). The community composition of the Tulip Beds is dominated by sessile organisms, in particular suspension feeders (O’Brien & Caron, Citation2015). As in other Burgess-Shale type localities, lobopodians represent a small fraction of the palaeocommunity overall, in both diversity and abundance, but the new species (Undet Lobopodian TB-A, fig. 9 in O’Brien & Caron, Citation2015) is the most common.
Observations, imagery and drawings
Part and counterpart of each specimen were observed when present. Some specimens were prepared using a micro-engraving tool equipped with a tungsten bit to remove sediment coating small areas of the body, like spines and claws. A Leica M205C stereomicroscope was used for observation of the specimens and a Canon EOS 5DSR SLR camera was used for photography under different lighting conditions, including cross-polarized lighting and specimens immersed in water. Superposed images of part and counterpart of the same specimens were obtained using the tool ‘Apply Image’ in Adobe Photoshop 2022. For scanning electron imagery, one specimen (ROMIP 53239) was examined with a scanning electron microscope (FEI Quanta 200 FEG) at the University of Windsor Great Lakes Institute for Environmental Research, Canada. Elemental mapping was performed with energy dispersive spectroscopy (EDS) using an EDAX Octane Plus Silicon Drift X-Ray detector using a 12 kV beam accelerating voltage under 70 Pa chamber pressure (low vacuum). Technical and life reconstruction drawings were based on observations of all preserved specimens, while some interpretative line drawings of individual specimens were produced for clarity.
Phylogenetic analyses
Tree searches were based on a dataset of 48 taxa and 81 discrete characters, modified from Caron and Aria (Citation2017, Citation2020a) and complemented based on Kihm et al. (Citation2023). All characters were unordered and unweighted, with Priapulida used as outgroup. Bayesian phylogenetic analyses followed an Mkv + Γ model (Lewis, Citation2001) with four runs each with four chains for 1,000,000 generations and burn-in at 20%, and were performed using MrBayes (Ronquist et al., Citation2012) v.3.2.6 x64. No backbone or time constraints were used. Convergence was assessed using MrBayes’ diagnostic tools, namely an estimated sample size ≫ 200, and a potential scale reduction factor = 1.00. Character list, matrix, run file and full Bayesian consensus phylogram are available in Supplemental material and online at https://github.com/cedricaria/lobphyl_pub/releases/tag/v1.0.0.
Preservation
Fossils are originally preserved as carbonaceous films replicated by aluminosilicates ( and Supplemental Fig. S1), as is typical of Burgess-Shale type fossils from deposits with a diagenetic history influenced by metamorphism (Gaines et al., Citation2008). The posterior, anchoring tagma is consistently and discretely more poorly preserved than the anterior region of the animal, or even entirely missing (, , , ), except in the two best specimens (, ). This taphonomic pattern cannot be explained by differences in body composition, since the posterior part of the body is as sclerotized as the front, or even more so. A possible line of reasoning is that most specimens are carcasses of moulting individuals, with the old, more rigid anterior part of the body being shed while the softer-skinned animal is present posteriorly. Although this hypothesis might be consistent with the scattering of well-preserved posterior spines in these specimens, it does not readily explain the complete absence of rear end, spines and claws included. Another explanation could come from the lifestyle itself of the animal: being erect during suspension-feeding, and strongly anchored to a substrate, the shear kinetic force of transportation caused by the event of entombment (likely, a mudflow) would rip apart the anterior and posterior parts of the body. Comparable signs of tearing can be observed in other sessile taxa from the Burgess Shale, such as hemichordate tubes (Nanglu et al., Citation2016). In our description, we identify possible ‘cone-in-cone’ patterns in claws (): the presence of only one clear imbricated claw inside another may represent a pre-moulting morphology; however, the fact that this type of sclerotic pattern is only known among lobopodians in BST panarthropods (Smith & Ortega-Hernández, Citation2014) suggests it is more likely evidence of a cone-in-cone growth pattern.
Institutional abbreviations
KUNMIP, University of Kansas, Biodiversity Institute, Division of Invertebrate Paleontology, Lawrence, USA; ROMIP, Royal Ontario Museum Invertebrate Palaeontology, Toronto, Canada; YKLP, Key Laboratory for Palaeobiology, Yunnan University, China.
Systematic palaeontology
Superphylum Panarthropoda Nielsen, Citation1995
Order Luolishaniida Hou & Bergström, Citation1995
Family Collinsovermidae Caron & Aria, Citation2020a, Citationb
Genus Entothyreos gen. nov
Type species
Entothyreos synnaustrus sp. nov.
Derivation of name
From the Ancient Greek entos, ‘inside’, and thyreos, a type of large shield.
Diagnosis
Collinsovermid lobopodian with elongate body, bearing a distinct head, 11 lobopod pairs and corresponding spine sets, plus a posterior-most lobopod pair and corresponding reduced somite. Differentiated head somite bearing anteriorly a pair of flattened, feather-like projections and posteriorly a single pair of short and stout spines. Basal portions of anterior six lobopods covered in a dense array of small spines. Each post-cephalic metameric spine set composed of four spines: two lateral and two longer and stouter dorsal. Within each somite, dorsolateral spines connected to a large, subrectangular, sclerotic sheet, covered in a thin layer of relatively flexible cuticle (more prone to deformations and folding), extending laterally on each side from the point of lobopod insertion and occupying the entire dorsolateral area of the somite. Stout single claws of posterior five lobopods produced at ventral mid-length into a recurved element, forming a non-articulated pincer-shaped claw. Distal margins of annuli convex and adorned with shorter and longer spines; minute spines present on the remainder of the margin. Posterior first four limb pairs (7–10) with strong sclerotized annulations, the distal-most two annuli being twice as long as wide. Annuli of posterior-most lobopods developed into imbricated sclerites.
Entothyreos synnaustrus sp. nov
–, Supplemental Fig. S1
Figure 1. Entothyreos synnaustrus gen. et sp. nov., taphonomy. A, Paratype ROMIP 53233. Full specimen (posterior region missing). Spine arrangement quasi in place but underlying plates not visible. B, Paratype ROMIP 53241. Possible moult with spine arrangement little disturbed, missing endocuticular plates. C, Paratype ROMIP 53244. Weathered specimen preserving annulated posterior lobopods (arrow). D–H, Paratype ROMIP 53239. D, Full specimen (composite image of the part and counterpart, specimen immersed in water). Insets as indicated. E, Close-up of a pair of anterior lobopods. Inset is F. F, Close-up of proximal-most portion of lobopods with dense array of setae or small spines. G, EDS imaging of carbon (red) showing minute elements along spines otherwise rich in aluminium (light blue). H, BSE imaging of anterior spines showing minute external ornamentation. Abbreviations: al, anterior lobopod; -l, left; pc, paired claw; pl, posterior lobopod; -r, right. Scale bars: A, B, 10 mm; C, D, 5 mm; E, H 2 mm; F, G, 1 mm.
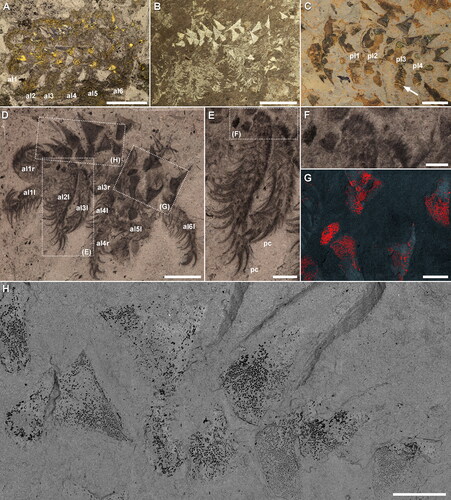
Figure 2. Entothyreos synnaustrus gen. et sp. nov., range of preservation types, ROMIP 59505. A, Full slab, with Entothyreos paratype specimens numbered 1–9. Insets as indicated. B, ROMIP 59505.2, anterior section with front limbs. C, ROMIP 59505.3. dissociated anterior section. D, ROMIP 59505.5, dissociated spines and dorsolateral sheets. E, ROMIP 59505.6, anterior section F, ROMIP 59505.7, isolated spine and claw. G, H, ROMIP 59505.8, isolated spines and claws. G, Full specimen. H, Close-up of claws of posterior lobopod. I, ROMIP 59505.9. fragment of posterior section showing dissociated dorsolateral sclerotic trunk sheets and spines. Abbreviations: al, anterior lobopod; ds, dorsal sclerotic sheets; pl, posterior lobopod. Scale bars: A, 50 mm; B, D, E, G, I, 5 mm; C, F, 2.5 mm; H, 1 mm.
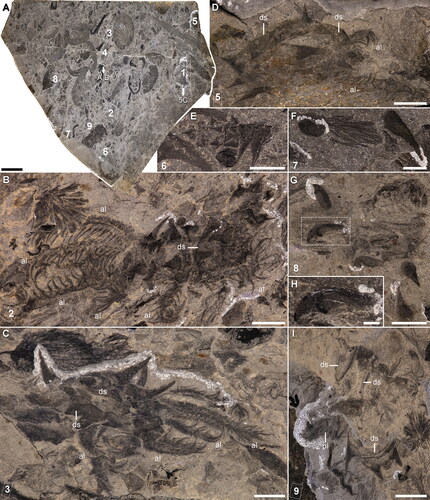
Figure 3. Entothyreos synnaustrus gen. et sp. nov., morphoanatomy. Holotype ROMIP 53234. A, Full specimen preserving body outline and head, showing lobopods of the 11th somite (arrow), and margins of sclerotic trunk sheets (arrowheads). Insets as indicated. B, Close-up of anterior-most area. C, Close-up of head, showing antennal organs and pair of head spines. D, Close-up of twisted left posterior lobopod of somite 10 showing smaller and longer spines (arrows) on the annuli margins (arrowheads). E, Close-up of posterior-most lobopods. F, Interpretative drawing of E, numbers correspond to sclerotic annuli. One of the proximal sclerites (sa) of the left lobopod is displaced, showing marginal spines. Abbreviations: ao, antennal organ; cn, somite n; h, head; hs, head spine; -l, left; -m, median; pc, paired claw; pl, posterior lobopod; -r, right; sn, spine n; sc, single claw; si, spinule. Scale bars: A, 5 mm; E, 2.5 mm; B, D, 2 mm; C, 1 mm.
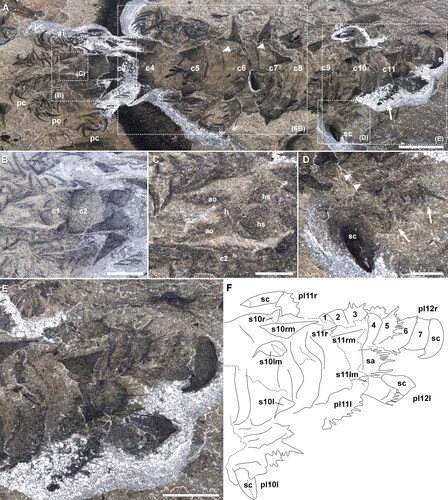
Figure 4. Entothyreos synnaustrus gen. et sp. nov., morphoanatomy. Paratype ROMIP 64650. A, B, Full specimen, partially disarticulated, exposing sclerotic trunk sheets and spines. Insets as indicated. A, Part. B, Composite images of the part and counterpart. Asterisks point to empty intercalary space between sclerotic trunk sheets. C. Close-up of middle body section, showing the posterior margin of sclerotic trunk sheets (arrow), the basal margin of spine (bold arrow), and the demarcation line between left and right halves of sclerotic trunk sheets (arrowhead). D, Close-up of non-terminal posterior lobopod, showing enlarged annuli (arrowheads). Inset is E. E, Close-up of margin of annuli of posterior lobopod, showing smaller and longer spines on the annuli margins (arrows) and annuli margins (arrowheads). F, Close-up of posterior-most lobopod. Numbers correspond to sclerotic annuli. Inset is G. G, Close-up of single claw in posterior-most lobopod, showing additional claw outgrowth (arrowhead). Abbreviations: al, anterior lobopod; ds, dorsal sclerotic sheets; h, head; ie, intercalary element; -l, left; pl, posterior lobopod; r, right. Scale bars: A, B, C, 5 mm; D, F, 2.5 mm; G, 1 mm; E, 0.5 mm.
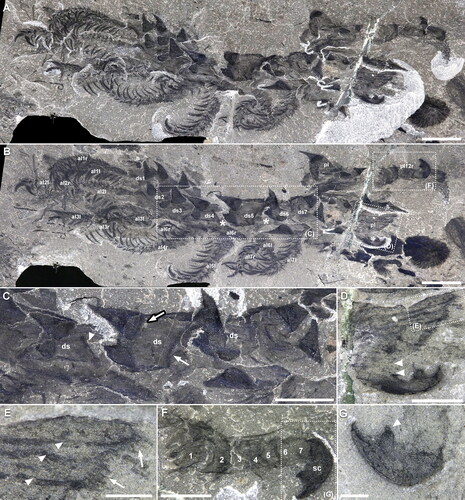
Derivation of name
From the Ancient Greek synnaustros, ‘convergent’.
Type specimens
Holotype: ROMIP 53234. Paratypes: ROMIP 53233, 53239, 53244, 59505.1-9 (9 specimens on a single slab), 64650, 66325. Fourty additional specimens (See Supplemental Table of specimens).
Occurrence
Middle Cambrian, Wuliuan Stage, Campsite Cliff Shale Member, ‘Thick’ Stephen (Burgess Shale) Formation, Burgess Shale, Tulip Beds, Mount Stephen, Yoho National Park, British Columbia, Canada.
Diagnosis
As for genus.
Description
Collinsovermid lobopodian with elongate body (c. 5 cm), 12 somites divided into two tagmata, bearing a total of 11 lobopod pairs (six + five) and corresponding stout to slenderer spine sets for pairs 1 to 10 (, , , , ). Anterior-most, presence of a distinct head somite bearing posteriorly a single pair of short and stout spines, and anteriorly a pair of short projections with possibly sensory function (). Body thickens posteriad along anterior tagma to reach maximal diameter at the level of the first lobopod pair of the posterior tagma (somite 8 – limb pair 7). Body then tapers to reduced terminal somite bearing last lobopod pair (, ).
Each post-cephalic and pre-terminal somite bearing a set of differentiated sclerotic elements composed of four stout, conical, transversally aligned spines (, , , ), a sub-apical cuticular sheets and intercalary elements. Lateral spines slightly shorter than median pair; dorsolateral spines all increasing in size to post-cephalic somite five, the longest spine being slightly smaller (c. 4/5th) than body width, then gradually decreasing in size posteriad (–, Supplemental Fig. S1); anterior-most cephalic and posterior-most lateral smallest, c. 1/10th of body diameter. Base of spines circular (, , ), with diameter from c. half (smallest spines) to slightly more than 1/3rd of spine length (largest spines). Ventral margin of spines slightly curved. Spine surface ornamented by hundreds of minute (ca. 25–75 μm across) carbonaceous rhomboidal elements (). Spines embedded within the anterior half of rigid trapezoidal sheets extending over the entire length of the somite anteroposteriorly and laterally to the extent of lateral spine insertions (, , , , ). Sclerotic sheets covered by thin layer of flexible integument, which stops just above the base of the spines (, ). Seemingly associated with the posterior portion of these sub-apical cuticular sheets are darker intercalary elements likely involved in articulation (, ). In some disarticulated material, sheets preserve in association with spines, showing connectivity of tissues (, , , ). In other specimens, the presence of spines in rough anatomical position but with little or no evidence of sheets suggests these sheets were probably thinner and perhaps decayed more quickly (, ).
Lobopods differentiated into two discrete sets: an anterior set of seven long and slender lobopod pairs, and a posterior set made of four stouter, conical, annulated limb pairs (–, Supplemental Fig. S1). Lobopod interspace increasing from somite 2 to 7, then decreasing to posterior-most somite. Long and slender lobopods subequal in size, covered on their dorsal side with a multitude of very short spines, and bearing ventrally two rows of very long (up to more than 3 times lobopod diameter), curved, strongly sclerotized, chevron-shaped, claw-like spines, spaced by c. 1/3 of lobopod diameter (, , , , , , Supplemental Fig. S1A, B); termination bearing a pair of curved claws similar to the chevron-shaped spines but stouter and showing possible evidence of cone-in-cone structures (); base covered in a denser array of small spines or setae ().
Figure 5. Entothyreos synnaustrus gen. et sp. nov., morphoanatomy. A–E, Paratype ROMIP 66325. A, Part (image flipped vertically). Specimen associated with Naraoia compacta. Insets as indicated. B, Close-up of distal paired claws, showing possible cone-in-cone growth pattern (arrowheads). C, Counterpart. Anterior portion of specimen. Inset is E. D, Close-up of Naraoia compacta showing partially overlapping anterior and posterior shields (arrowhead); note similarity with overlap of sclerotic trunk sheets and intercalary elements in Entothyreos (E). E, Close-up of contact area between dorsolateral sclerotic sheets and intercalary areas (arrowheads point to posterior margins of sclerotic sheets). Scale bars: A, 10 mm; C, D, 5 mm; E, 1 mm; B, 0.5 mm.
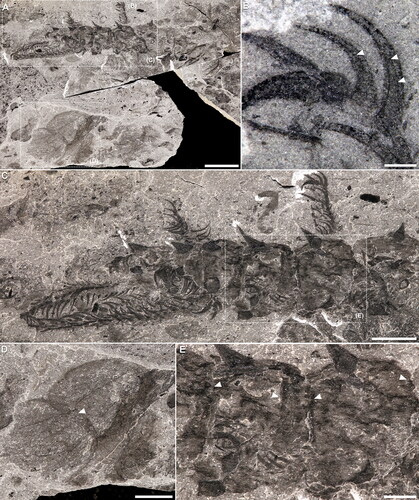
Several specimens, including the holotype, preserve six pairs of anterior elongate lobopods (, , Supplemental Fig. S1A, B). Among Collinsovermidae, this regionalization is consistent with the anatomy of at least Collinsium (Yang et al., Citation2015a) and Collinsovermis (Caron & Aria, Citation2020a, Citationb). The holotype also displays a total of 10 post-cephalic spine-bearing somites. In turn, paratype ROMIP 53244 () preserves four posterior annulated lobopods on one side of the animal. This evidence altogether supports our diagnosis and reconstructions (, ). However, none of these specimens provide a full succession of limbs including both last anterior and first pair of posterior, conical lobopods.
Figure 6. Entothyreos synnaustrus gen. et sp. nov., line tracings. A, Paratype ROMIP 64650, full specimen, positive (see ). B, Holotype ROMIP 53234, central trunk section, positive (see ). C, Paratype ROMIP 66325, central trunk section, negative (see ). Colours: dark yellow, surface cuticular layer; light orange, sclerotic sheet; purple, intercalary element. Line legend as indicated in A.
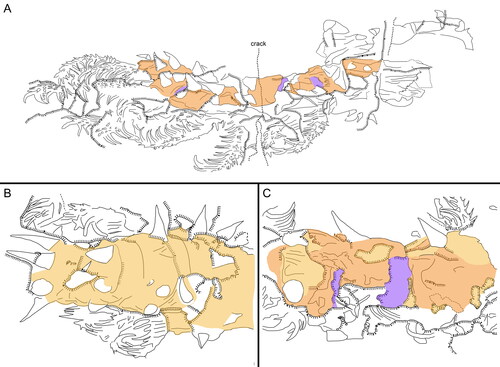
First four pairs of posterior lobopods about three times as long as wide, composed of annuli with greater degrees of sclerotization compared to close relatives and lobopodian average, ending in single, very large, stout claws (, ); tapering conically with typical annulation shape pattern up to distal-most two annuli, which are about twice as long; annuli with dorsal margins adorned with succession of smaller and shorter spines (, ), remainder of margin with minute spines. Posterior-most lobopod pair with annuli differentiated into seven imbricated sub-rectangular to quadrate sclerites in lateral view but with generously convex distal margins adorned with succession of smaller and shorter spines; these lobopods directly attached to body termination without posterior trunk extension (, ).
Remarks
Our interpretation of paratype ROMIP 64650 indicates the possible presence of a seventh lobopod pair (), which appears inconsistent with other specimens. In the absence of additional evidence allowing to resolve this issue, we keep here to a conservative interpretation but note that Entothyreos – or at least some of its individuals – may bear a seventh pair of elongate suspension-feeding lobopods in lieu of anchoring lobopods.
The fossil material is composed of two morphotypes differentiated mainly by spine length. The diagnosis and description are based on the largely more abundant first morphotype, with tubular body and longest dorsolateral spines subequal to body width. The other morphotype, of comparable overall size, and represented by only four specimens (Supplemental Table of specimens), sports a stouter and more compact body and more elongate dorsolateral spines (). The absence of notable body length difference and the fact that both morphotypes are present on the same surface (ROMIP 53235) strongly suggests that these morphological differences represent sexual dimorphism, as opposed to a different species.
In reference to the body regionalization of E. synnaustrus and other luolishaniids, we use the terms ‘tagma’ and ‘tagmata.’ However, it should be noted that euarthropod tagmatization is defined by regionalized sets of segments underlined by different Hox gene expressions (Hughes & Kaufman, Citation2002). Whether homologous pathways and patterns were involved in the regionalization of luolishaniid bodies cannot be ascertained at present (especially given known differences in expression patterns of tardigrades and onychophorans; Smith et al., Citation2016), and therefore ‘tagma’ should be understood as ‘pseudo-tagma,’ as we used previously (Caron & Aria, Citation2017, Citation2020a, Citationb).
Phylogenetic results
The consensus tree topology of our phylogenetic analysis is similar to previous results obtained with this dataset (Caron & Aria, Citation2017, Citation2020a, Citationb) (, Supplemental material, https://github.com/cedricaria/lobphyl_pub/releases/tag/v1.0.0). Many posterior probabilities remain low owing to our coding strategy, which minimizes homology assumptions and the coding of states non-observable in fossils, compared to concurrent datasets (Caron & Aria, Citation2017). Tactopoda is favoured here over Onychophora + Arthropoda, but without compelling branch support. Luolishaniids and hallucigeniids represent the onset of the panarthropod radiation, here with Onychodictyon taxa as intermediate between basal luolishaniids and derived hallucigeniids. Within Luolishaniidae, Entothyreos synnaustrus gen. et sp. nov. is resolved as a sister taxon to the Emu Bay Shale Collins’ monster, while the other Collinsovermidae form a separate clade. By contrast with a previous phylogenetic analysis, Luolishania is here grouped with the ‘spine-less’ luolishaniids, a scenario which reinforces the derived nature of the loss of sclerites in these taxa and the loss of posterior limbs in Facivermis. The presence of posterior limbs with paired claws, the uniformization of lobopod shape and length towards short and conical (onychophoran type) and the ventralization of the mouth opening define the lobopodian grade leading to the successive appearance of tardigrades, onychophorans and arthropods (, Supplemental Fig. S3).
While our work was being reviewed, Kihm et al. (Citation2023) provided a long-awaited integration of tardigrade morpho-anatomical phylogenetics with fossil evidence. The claim was notably made that luolishaniids could constitute sister taxa to tardigrades. Considering widespread homoplasies and relative poor node support across the lobopodian tree (Caron & Aria, Citation2017; Ma et al., Citation2009a; Moysiuk & Caron, Citation2022), a few synapomorphies suffice to form a consistent clade, as the results of Kihm and colleagues show (Kihm et al., Citation2023), and so these diagnostic features must be scrutinized. Although the latter study brought several interesting elements to the discussion, we contest that a number of the proposed key character states linking tardigrades and luolishanids can be ascertained with the current evidence:
Some luolishaniids bear sensory projections with relatively wide bases (Caron & Aria, Citation2020a, Citationb; Yang et al., Citation2015b; this study). Among extinct lobopodians, similar structures are found notably in Antennacanthopodia and Onychodictyon ferox – it is therefore present outside of Luolishanida. Cirri A of tardigrades do not only have structural differences (e.g., they are thinner and have differentiated insertion points), they also count among a variety of identically structured cirri along the tardigrade body in many species (as illustrated by Kihm et al., Citation2023), which lobopodians lack. It is also uncertain whether the topological placement is the same, since the mid-head of tardigrades is morphologically differentiated, when there is no mid-head boundary per se among lobopodians. We therefore code uncertainty in tardigrades. Sensory structures were coded as present in Facivermis by Kihm and colleagues without definitive evidence.
The proboscis of Ovatiovermis is not visibly adorned and is equally comparable to the soft tissue of the evaginated introvert of priapulids (Caron & Aria, Citation2017).
Kihm et al. (Citation2023) accept that the subtle rods described along the head of Kerygmachela are apodemes, but then call them rostral spines and homologize them with the tardigrade stylet. We consider that the evidence is insufficient at this time to justify effectively coding homology.
In Kihm et al. (Citation2023), elements associated with the lobopod terminal spines in Onychodictyon ferox are homologized with the basal extensions of the typical heterotardigrade claws. However, the illustrated cases show that in O. ferox the orientation of this element relative to the main claw is variable, and in two cases opposite to the tardigrade condition (pointing dorsolaterally instead of ventrolaterally). We deduce that this element might have been partly movable and functionally convergent with the anchoring claw extension described on the posterior claws of Entothyreos. Although the number of individual claw elements in Aysheaia was likely higher than in tardigrades (Whittington, Citation1978), these elements are curved and aligned at their base as they are in most tardigrades, homology that we maintain alongside a ‘claw number’ character.
Neomorphic character 30 in Kihm et al. (Citation2023) has considerable impact on the monophyly of luolishanids and tardigrades since it is associated with nine dependent transformational characters. This character and its dependences deal with the presence of two sets of differentiated lobopodous appendages along the body, although only the posterior-most pair in tardigrades shows accounted differentiations. Dependencies are then used to code for anterior limb shape, number, number of claws, etc. in luolishanids and tardigrades separately from other lobopodian taxa. We posit that this creates an undesirable bias where, for instance, number of claws or limb adornments should be coded in a character that also includes other lobopodians. The anchoring posterior tagma of luolishanids is arguably more differentiated and should instead be the focus of separate coding. In addition, other lobopodians display differentiations associated with the posterior-most lobopod pair, if only by the forward orientation of the claws, and the tardigrade condition should first be considered in this light (as is done redundantly in character 119 of Kihm et al., Citation2023) rather than forcefully homologized with the morpho-functional appendage subdivision of luolishanids.
Kihm and colleagues dismissed the evidence in their coding for the symplesiomorphic condition of pharyngeal armatures in panarthropods, but we disagree. The authors state in their supplementary information files (Character 70, p. 14) that microspines are absent “in the other cockroach relatives” (presumably other crown-group arthropods?), but this is incorrect, since Elzinga (Citation1998) reported microspines being present in the pharynges of chelicerates (including pycnogonids), chilopods and insects, and otherwise absent in crustaceans, diplopods and onychophorans. In Kihm et al. (Citation2023), dependent character 71 does not provide any ingroup resolution, while character 72 is only coding for the autapomorphy of Hallucigenia sparsa. We synthesized instead the information into a new ‘pharyngeal armature type’ character.
Our own result following careful assessment and integration of data from Kihm et al. (Citation2023) maintains a basal placement of luolishaniids and offers even stronger support for the resolution of Aysheaia as the closest tardigrade sister taxon based on these apomorphies: Sensorial structures surrounding the mouth: present (c. 31:1); Limb tip, type: set of four or more slender claws with strongly-curved tips (c. 70:1); Lobopodous trunk posterior termination type: vestigial (‘truncated’) (c. 73:0) (local apomorphy); Number of limb pairs affected by the reduction of posterior interspace: two (penultimate and last) (c. 76:0). Stabilization of Aysheaia as the tardigrade sister taxon strongly suggests general onychophoran-like morphological traits (e.g. annulated bodies and lobopods, frontal sensory organs, dermal papillae, cylindrical bodies with numerous limb pairs) are part of the non-luolishaniid/hallucigeniid panarthropod ground pattern, regardless of the placement of extant onychophorans themselves.
Discussion
Identity of sclerotic elements
Entothyreos synnaustrus gen. et sp. nov. displays general and diagnostic characteristics of collinsovermids (, ). A number of 12 somites is intermediate between Ovatiovermis (Caron & Aria, Citation2017) and the collinsovermid from the Emu Bay Shale (García-Bellido et al., Citation2013) and other luolishaniids, and it autapomorphically bears trunk sets of four dorsal spines, increasing morphological diversity in this group, which is otherwise characterized by trunk sets of 3, 5 or considerably more (in Acinocricus (Caron & Aria, Citation2020a, Citationb)). The animal also atypically sports a pair of spines on its head somite, which appears to also be a feature of Luolishania (Ma et al. Citation2009b). More importantly, however, E. synnaustrus is characterized by two exceptional features: wide dorsal sheets to which the spines are connected, and series of imbricated sclerites on posterior lobopods.
Figure 7. Entothyreos synnaustrus gen. et sp. nov., range of preservation types, ROMIP 59505. A, Paratype ROMIP 59505.4. Full specimen, with overlap of body somites preserved in a similar way as articulated podomeres of radiodontan appendages on the same bedding plane. B, Close-up of specimen (posterior region missing) showing well-developed dorsolateral sclerotic sheets (composite image of the part and counterpart, specimen immersed in water). Asterisks point to soft integument covering the base of dorsolateral spines. C, Paratype ROMIP 59505.1 showing segmental sclerotic sheets and intercalary elements (arrows). Abbreviations: al, anterior lobopod; ds, dorsal sclerotic sheets. Scale bars: A, 10 mm; B, C, 5 mm.
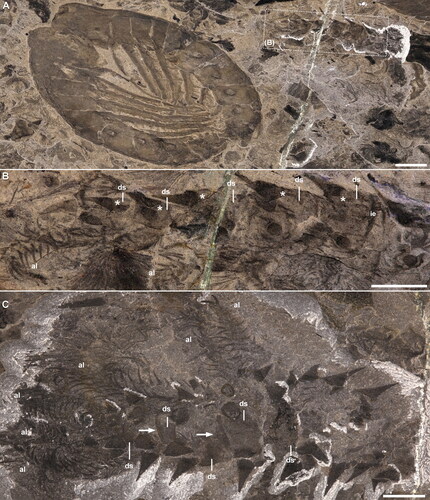
Figure 8. Dominant taphonomic gradient of the trunk in Entothyreos synnaustrus gen. et sp. nov. A, Fully preserved with body outline including flexible upper layer of the integument, covering sclerotic sheets and their intercalary elements as well as the base of the spines. Lateral constriction may represent an initial degree of decay following loss of muscle tissue. B, Flexible upper layer of the integument decayed away or absent from moult carcass. C, Flexible upper layer of the integument and intercalary elements decayed away or absent from moult carcass. D, Spines only, conserving original disposition.

The dorsolateral sclerotic sheets are preserved separately from the main body mass, with distinct margins, in some disarticulated specimens (, ) – those are either moults, decayed specimens, or a combination of both, owing to the disarticulation and lack of body outline (see also ). In the holotype, which preserves the body outline and associated soft tissue in full in dorsoventral aspect, these sheets only appear via the impression of their anteroposterior boundaries (, ). The soft, flexible cuticular surface covering the whole body and the base of the spines, as well as the underlying sclerotic sheets, can also be seen in specimens preserved in latero-oblique aspect (, , ). In those cases, however, the body outline is harder to disambiguate from the sheets because the latter lie close to the dorsal surface of the animal. This indicates that these sheets, like the base of the spines, were covered by an additional, soft cuticular layer more typically encountered across lobopodians as the surface integument.
Figure 9. Entothyreos synnaustrus gen. et sp. nov., technical drawings. A, lateral view; B, dorsal view. All drawings by Danielle Dufault © ROM. Abbreviations: ao, antennal organ; cs, spinose cover of lobopod; ds, dorso-lateral sheets; ie, intercalary element; h, head; hs, head spine; -l, left; ln, lobopod n; -m, median; pc, paired claw; -r, right; sn, spine n; sa, sclerotized annulus; sc, single claw; si, spinule.
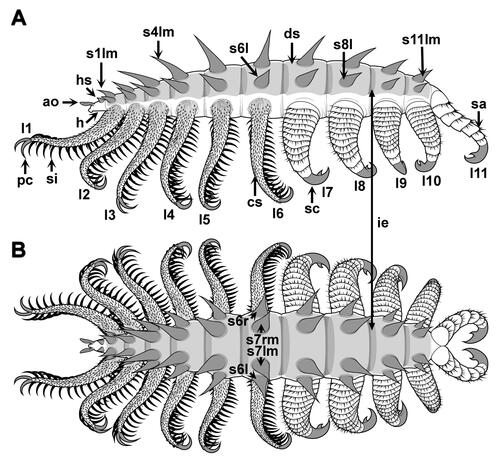
This interpretation is consistent with the rest of the fossil evidence accumulated thus far. Extensive but thin sclerotic rings of cuticle between spines characterize Acinocricus (), but the presence of somewhat more extensive sclerotic sheets associated with the dorsal spines is particularly similar in Luolishania and Collinsium (Caron & Aria, Citation2020a, Citationb; Ma et al., Citation2009b; Yang et al., Citation2015a) (). In these two taxa, it is also clear that these sheets are covered by a thin, flexible cuticular layer, either because of their typical preservation as ‘internal organs,’ in the form of fainter, variably occurring patches always within the body’s boundaries (Ma et al., Citation2009b; Yang et al., Citation2015a) (), or because of the direct observation of the base of the spines lying beneath the integument (Yang et al., Citation2015a, fig. S5) (). Documented evidence for Luolishania and Collinsium displays a range of aspects, from the inter-spine connective ring being covered at various degrees, which corroborates our observations in Entothyreos. The presence of cuticular patches in-between spines born by the ring in Acinocricus (Caron & Aria, Citation2020a, Citationb, fig. 6) confirms that the ring itself was also covered by layer of integument. Taphonomic gradients as well as visible separation between the margin of the spine base and the surrounding plate also show that spines and sheets are related but dissociable elements (, , , , ).
Figure 10. Entothyreos synnaustrus gen. et sp. nov., life reconstruction. Drawing by Danielle Dufault © ROM.
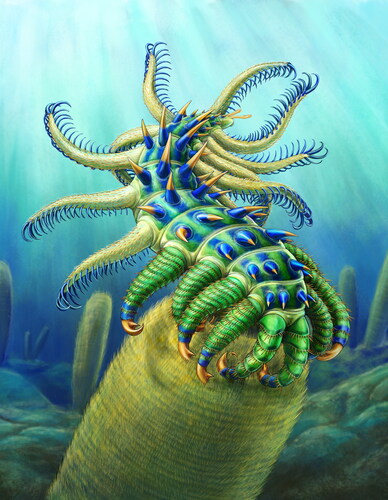
By contrast, the elongate and overlapping structures on the posterior-most lobopods are clearly covering the surface of the appendages. Their distinct, imbricated margins suggest these elements are sclerotic, and different from thick annuli (). However, the podomere-like sclerites must logically derive from differentiated annuli (see below), and therefore a certain structural continuity is expected between these features. We call them here ‘sclerotic annuli’.
Morpho-anatomical implications
Our observations suggest that the dorsal sclerotic sheets in E. synnaustrus and other luolishaniids are broad extensions of tissue around the base of the spines intercalated under another cuticular layer representing the surface of the animal. The panarthropod cuticle, secreted by the epidermis, is typically composed of two morphofunctionally distinct layers: the thicker procuticle, made up of crystalline laminae of chitin microfibrils and responsible for the shape and strength of the cuticle; and the superficially microsculpted but largely amorphous epicuticle, composed of a variety of proteins and lipids underlying the surface envelope – which is fundamental in its protection against dehydration and soaking (Hadley, Citation1986; Moussian, Citation2013; Robson, Citation1964). The procuticle has traditionally been divided into a thicker, more flexible endocuticle, and a more rigid exocuticle.
The latter distinction has its importance in the physical micrometric sub-division of the cuticle, since the epicuticle is too thin to be likely identifiable in fossils.
With respect to the structure of the panarthropod cuticle (Moussian, Citation2013; Robson, Citation1964), the sclerotic sheets of Entothyreos therefore seem to be endocuticular in nature, although it cannot be determined whether they were constituted of the entire endocuticle or only part of it. In addition, Entothyreos provides evidence of intercalary elements associated with the posterior portion of endocuticular sclerites, but seemingly distinct from both sheets and spines, which we construe were involved in the articulation of the endodermal sclerites. A precise reconstruction of these intercalary elements, which lie underneath the endodermal sclerites, is difficult at present.
Spine insertion in hallucigeniids may shed light on the potential developmental origin of the sclerotic sheets of Entothyreos. In Hallucigenia, the base of the spines is often embedded in thick knobs, especially in larger specimens (Smith & Caron, Citation2015) (). Given their size, these knobs likely encompass the entire thickness of the integument and perhaps subdermal tissue. This implies that the spine is produced directly by highly modified epidermal cells, and that the surrounding cuticle layer likely corresponds to both procuticle and epicuticle, as would be expected in an early panarthropod (Robson, Citation1964). Extreme modification of epidermal cells at the origin of integumental specializations, such as pharyngeal teeth, has been reported in priapulids (Shapeero, Citation1962). This suggests that, like pharyngeal teeth, which are retained throughout Panarthropoda (Caron & Aria, Citation2017), lobopodian spines and possibly other types of sclerites are connected directly to and produced by highly differentiated cells of the epidermis, rather than being mainly exocuticular structures fed by the epidermis.
The interpretations above raise questions relative to cuticle morphogenesis and moulting, because an onychophoran-like stacked growth pattern of sclerites (Smith & Ortega-Hernández, Citation2014) has been shown to be likely plesiomorphic in Panarthropoda (Caron & Aria, Citation2017, Citation2020a), notably characterizing claws and dorsal spines in armoured lobopodians including E. synnaustrus (). Partial association of the spines and endocuticular sclerites could imply that the spines were moulted separately, while endocuticular sclerites would have been grown and shed along with the remainder of the cuticle. Large assemblages of isolated hallucigeniid and Microdictyon spines (Caron et al., Citation2013) could further point to separate moulting events for these structures. However, these assemblages cannot be differentiated from mass mortality events out of which only the hardest structures would remain (Haug et al., Citation2013). In addition, asynchronous moulting in extant panarthropods is known only as an apomorphy of isopods (viz., biphasic moulting; George, Citation1972), and, despite constituting a plausible explanations for specific Cambrian moult assemblages (Haug et al., Citation2013), it appears unlikely to be an ancestral panarthropod trait. Thus, spines and associated plates are considered for now to belong to the same moulting cycle.
The evolutionary acquisition of the endocuticular sclerites is also in question. The limited lateral extensions of the sclerotic rings in Acinocricus would suggest that endocuticular sclerites indeed grew as chitinization of connective tissue around the spine base, as in Collinsium and Luolishania (), although these taxa are not retrieved as basal within Collinsovermidae (). Because of their subapical cuticular location, endocuticular sclerites are distinct from euarthropod tergites, but it is also possible that endocuticular sclerites of luolishaniids and tergites share a genetic basis. Like an external sclerite, endocuticular sclerites must have been permeable to pore canals and dermal gland ducts in order to allow for the transport of lipids as well as proteins, such as chitinases for ecdysis or transglutaminases for repair (Moussian, Citation2013) ().
Figure 11. Evolutionary implications. A, Simplified consensus phylogram from a Bayesian analysis of lobopodian relationships and evolution of body cuticular organization. Stars mark lineages with cases of parallel evolution of segmental plates or sclerotization of body/limb units; purple stars correspond to non-articulating elements; bold magenta stars correspond to elements presenting overlap and/or articulation mechanisms. Greek letters refer to lobopod types in B. Both luolishaniids and hallucigeniids are considered suspension feeders, but with weaker overall morphoanatomical specialization in the latter (red to yellow branches); condition uncertain in Onychodictyon. Bold branches are extant. Boxed diagrams illustrate integumental structure in key groups: contrary to armoured hallucigeniids, armoured luolishaniids possess more or less strong and extensive sclerites around and between ‘cone-in-cone’ spines (sp), the endocuticular sclerite (ES), lying underneath the exocuticle (EX) and perhaps the upper part of the endocuticle (EN). The whole cuticle is produced and fed by the epidermis (ED), which is composed of specialized housekeeping cells for large cuticular structures like spines, and provides macromolecules and notably lipids to the cuticular envelop through pore canals (pc); heterotardigrades have their ‘exocuticle’ (or inner epicuticle, IE) forming fibrillous pillars and a more flexible ‘endocuticle’ (intra- and procuticle, I + P); in euarthropods, the entire cuticle is more sclerotized and constituted of chitinous laminae throughout, although the more rigid exocuticle commonly also rests atop a more flexible endocuticle. Hallucigenia sparsa illustration by Danielle Dufault © ROM, Kerygmachela kierkegaardi by © Rebecca Gelernter (nearbirdstudios.com), used with permission. B, Disparity and hypothetical origins of walking limb morphotypes across panarthropods. The onychophoran lobopod (α) serves here as general morphological reference for the plesiomorphic limb condition (regardless of the distinction between elongate and shorter, conical forms). The annulus (orange) is its external constitutive unit. In Entothyreos (β, γ), posterior limbs have more strongly sclerotized annuli with some degree of differentiation (β), while the annuli of posterior-most lobopods are all longer and overlapping (γ). Arguably, the latter represents a greater degree of transformation of the former. In Diania (δ), annuli also form stronger units which also bear spines. In tardigrades (ε), there are no visible external divisions of the limbs, and thus the ancestral annulus is considered assimilated within the uniform outer cuticle. In arthropods (ζ), we construe that the annulus unit may evolutionarily correspond to a podomere, despite differences in internal structure (such as muscle attachments). The question mark denotes that the origin of the endopod and the arthropod limb as a whole remains debated, and that lobopod flaps may represent morphological intermediaries.
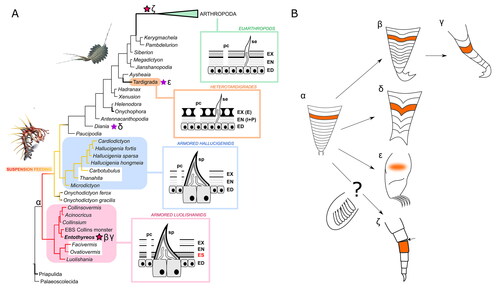
Figure 12. Cuticular taphonomy and relation to spines in luolishaniids. A–C, Acinocricus stichus. A, B, KUMIP 204353, close-up of middle body section dry (A) and wet (B). Upper softer cuticle is darker than sediment and annulated. Sclerotic ring and spines overlaid in cyan in A. C, KUMIP 153095. Array of spines joined together by sclerotic endocuticular ring. D, E, Luolishania longicruris, middle body section with sclerotic spine rings. Images courtesy of Xiaoya Ma. D, YKLP 11279. E, YKLP 11278. F–H, Collinsium ciliosum, sclerotic spine rings. Upper softer cuticle has a grey-blue colour. Images courtesy of Xiguang Zhang. F, YKLP 12131. G, YKLP 12135. H, YKLP 12137. I, J, Hallucigenia sparsa ROMIP 57776. I, Full specimen. Inset is J. J, Close-up of spine insertion, showing embedding in upper cuticle. Arrows point to soft upper cuticular remains; arrowheads point to parts of the endocuticular sclerotic tissue. Scale bars: A, B F–H, I, 5 mm; C, 10 mm; D, E, J, 1 mm.
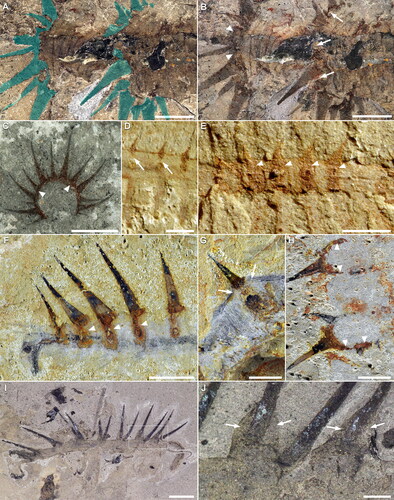
The only known comparable case of segmental or serial plates among extant panarthropods is found in heterotardigrades. Echiniscoidea and Arthrotardigrada possess dorsal and sometimes ventral sclerites resembling tergites and sternites, but lack any specific mechanism of articulation, and are not typically overlapping (Czerneková & Vinopal, Citation2021). The number and anatomical distribution of ventral plates greatly differ among armoured tardigrades (Fontoura et al., Citation2017), and can be segmental, intersegmental or a combination of both (Kaczmarek et al., Citation2012). In heterotardigrades, these plates derive from the fusion of pillars sometimes associated with the ‘endocuticle’ (Gąsiorek & Vončina, Citation2019) but corresponding in fact with the upper layer of the cuticle, likely equivalent to the arthropod exocuticle (Dewel et al., Citation1993), also called ‘inner epicuticle’ (Czerneková & Vinopal, Citation2021). The unique pillar system and its apical cuticular location suggests it represents yet another strategy of segmental plate formation ().
Morpho-functional interpretations
The reason behind the subapical cuticular location of sclerites in Enthoryreos is difficult to determine, but we consider this condition more likely the result of an evolutionary trade-off, tied to the origin of spines themselves and the presence of strengthening connective tissue in other luolishaniids, which have been a prerequisite to stability of large and numerous spines. Adaptively, they could be also seen as a form of endoskeleton. Considering that luolishaniids were erect suspension-feeders in water (Caron & Aria, Citation2017, Citation2020a), the development of these plates was possibly selected, not directly for protection, but to increase body rigidity. ‘Unlinked’ sub-dermal spines in hallucigeniids might simply result from developmental continuity with luolishaniid ancestors, but embedding these spines in integument and therefore creating a robust point of attachment also could have been essential to allow for extremely large size compared to the body, particularly in H. sparsa (Smith & Caron, Citation2015).
The imbricated sclerites on the posterior, anchoring lobopods are, by contrast, clearly external and better suited for protection: compared to the spinule-bearing frontal limbs, posterior limbs in other luolishaniids were indeed more vulnerable. By contrast to arthropod podomeres, these sclerites extensively overlap, akin to the plates of a knight’s armour, and seem to cover only the dorsal side of the appendage.
Evolutionary implications
Until now, Diania cactiformis represented a singular and notorious case of greater limb sclerotization among lobopodians (Liu et al., Citation2011), although arguably not forming podomeres as in arthropods (Ma et al., Citation2013). In spite of separate phylogenetic positions, the spinose and strongly sclerotized ‘segments’ of Diania’s lobopods are likely also derived from annuli, as seems to be the case in Entothyreos, although they do not overlap. Entothyreos would therefore shed light on Diania’s morphology by showing that the controversial (Legg et al., Citation2011; Ma et al., Citation2009b; Mounce & Wills, Citation2011) ‘arthropod-like’ aspect of body and limbs in this taxon is in fact part of a broader plasticity of cuticular structure among lobopodians.
In arthropods, podomeres are distinct for instance from articulating annuli owing to their individual muscle attachments (Boxshall, Citation2004). It is also unclear whether the arthropod limb arose from the modification of an annulated, walking lobopod, or from a dinocaridid flap (Aria, Citation2022; Budd & Daley, Citation2012; Lerosey‐Aubril & Ortega‐Hernández, Citation2022; Van Roy et al., Citation2015). Posterior-most lobopods in Entothyreos show, however, that an evolution of unarticulated annuli into articulated podomere-like sclerites is possible, and we therefore consider here the possibility of a deep historical structural correspondence between annuli and podomeres (; Jockusch, Citation2017).
E. synnaustrus therefore combines two distinct traits convergent with forms of articulation typified by euarthropods: a set of articulated dorsal-lateral sheets, shared with other collinsovermid representatives, and overlapping sclerites covering limbs. As another feature strongly expressed among collinsovermids, E. synnaustrus also shares with euarthropods a form of regionalization of body somites affecting limb morphology along the anteroposterior axis – which in the latter is called tagmatization, based by definition on the organization of arthrodized segments. Altogether, in light of our phylogenetic results () and of other examples of articulation or segmental sclerotization strategies, this constitutes a particularly advanced case of independent acquisition of phenotypic innovations, likely triggered by similar selective pressures in emerging Cambrian marine ecosystems, as observed in other lobopodian taxa (Howard et al., Citation2020) and other groups.
Because the sclerotization strategies discussed here co-occurred in separate but relatively closely related taxa, it is legitimate to ask whether they could share a common underlying factor, and, correlatively, whether arthrodization has a deeper intimate origin as a phenotypic product. Independent acquisitions of phenotypes in closely related taxa is called parallelism, which, as a sub-type of convergence, can be defined based on the close similarity of underlying genetic pathways (Stayton, Citation2015; but see Arendt & Reznick, Citation2008)). Sclerotization itself in all cases is likely following a similar process of covalent protein linkage with phenolic substances (Moussian, Citation2013); however, the other structural differences discussed here between lineages that evolved segmental plates among panarthropods question the extent to which this case of parallelism involved the same sets of genes. Perhaps it is more accurate to hypothesize the recruitment of partial genetic regulatory networks (GRNs). The co-option of similar GRNs in different lineages has been proposed to reconcile molecular and morphological data on the evolution of segmentation (Chipman, Citation2010). Recent studies have otherwise found that patterns of disparity during the Cambrian explosion could be explained by a concomitant build-up of GRNs (Aria, Citation2020; Deline et al., Citation2018), implying poorly canalized phenotypic pathways and therefore phenotypic output (Peterson et al., Citation2009). We hypothesize here that genes fundamental to articulation strategies were present early in Panarthropoda, but were integrated in different ways by different, emergent GRNs during the early evolution of the phylum, which led to a series of structurally distinct cases of plate formation and imbrication.
Conclusions
E. synnaustrus puts into perspective the uniqueness of ‘arthropod arthro(po)dization’ and its role in the tremendous success of this group. It reinforces in particular the importance of considering canalization, taken in the sense of a process buffering phenotypic expression (Peterson et al., Citation2009), in a macroevolutionary context, by suggesting that arthrodization is but one possible product of an emergent genetic-developmental pathway within panarthropods. In the course of their evolution, canalization might have allowed for genetic regulatory networks to co-opt ‘switched off’ phenotypic expression into new pathways (Artur & Kajala, Citation2021). Hypothetically, this could have turned arthrodization into an exaptation, providing arthropods with a rigidifying yet functional morphology, comparable to certain lobopodians, and which would develop as the basis of their tremendous morpho-functional diversity. In other words, arthrodization as defined by arthropods might be regarded less as a ‘key innovation’ but more as the by-product of a complex morpho-functional genome in the making.
Associate editor: Gregory Edgecombe
Supplemental Material
Download Zip (6.8 MB)Acknowledgments
Burgess Shale fossil material was collected by the Royal Ontario Museum under several Parks Canada Research and Collection permits from 1989 to 2016. We are grateful to Danielle Dufault (ROM) for the illustrations and to Sharon Lackie (Windsor) for providing Environmental Scanning Electron Microscopy images of specimens. We also thank two anonymous reviewers and Associate Editor Gregory Edgecombe for their insightful and constructive comments. This research was supported by a NSERC Discovery Grant (#341944 to J-B.C.). J-B.C. acknowledges the Dorothy Strelsin Foundation (ROM) for the purchase of a Leica M205C stereomicroscope which was used in this research and the French Embassy in Ottawa, Canada for a travel grant to CA. This is Royal Ontario Museum Burgess Shale project number 93.
Disclosure statement
No potential conflict of interest was reported by the authors.
Supplemental material
Supplemental material for this article can be accessed here: http://dx.doi.org/10.1080/14772019.2024.2356090.
Additional information
Funding
References
- Arendt, J., & Reznick, D. (2008). Convergence and parallelism reconsidered: what have we learned about the genetics of adaptation? Trends in Ecology & Evolution, 23, 26–32. https://doi.org/10.1016/j.tree.2007.09.011
- Aria, C. (2019). Reviewing the bases for a nomenclatural uniformization of the highest taxonomic levels in arthropods. Geological Magazine, 156, 1463–1468. https://doi.org/10.1017/S0016756819000475
- Aria, C. (2020). Macroevolutionary patterns of body plan canalization in euarthropods. Paleobiology, 46, 569–593. https://doi.org/10.1017/pab.2020.36
- Aria, C. (2022). The origin and early evolution of arthropods. Biological Reviews, 97, 1786–1809. https://doi.org/10.1111/brv.12864
- Aria, C., & Caron, J.-B. (2015). Cephalic and limb anatomy of a new isoxyid from the Burgess Shale and the role of ‘stem bivalved arthropods’ in the disparity of the frontalmost appendage. PLoS ONE, 10, e0124979. https://doi.org/10.1371/journal.pone.0124979
- Aria, C., Zhao, F., Zeng, H., Guo, J., & Zhu, M. (2020). Fossils from South China redefine the ancestral euarthropod body plan. BMC Evolutionary Biology, 20, 4. https://doi.org/10.1186/s12862-019-1560-7
- Artur, M. A. S., & Kajala, K. (2021). Convergent evolution of gene regulatory networks underlying plant adaptations to dry environments. Plant, Cell & Environment, 44, 3211–3222. https://doi.org/10.1111/pce.14143
- Bergström, J., Hou, X., Zhang, X., & Clausen, S. (2008). A new view of the Cambrian arthropod Fuxianhuia. GFF, 130, 189–201. https://doi.org/10.1080/11035890809452772
- Boxshall, G. A. (2004). The evolution of arthropod limbs. Biological Reviews, 79, 253–300. https://doi.org/10.1017/s1464793103006274
- Budd, G. E. (2001). Why are arthropods segmented? Evolution and Development, 3, 332–342. https://doi.org/10.1046/j.1525-142X.2001.01041.x
- Budd, G. E., & Daley, A. C. (2012). The lobes and lobopods of Opabinia regalis from the middle Cambrian Burgess Shale. Lethaia, 45, 83–95. https://doi.org/10.1111/j.1502-3931.2011.00264.x
- Caron, J.-B., & Aria, C. (2017). Cambrian suspension-feeding lobopodians and the early radiation of panarthropods. BMC Evolutionary Biology, 17, 29. https://doi.org/10.1186/s12862-016-0858-y
- Caron, J., & Aria, C. (2020a). The Collins’ monster, a spinous suspension‐feeding lobopodian from the Cambrian Burgess Shale of British Columbia. Palaeontology, 63, 979–994. https://doi.org/10.1111/pala.12499
- Caron, J.-B., & Aria, C. (2020b). Corrigendum: The Collins’ monster, a spinous suspension-feeding lobopodian from the Cambrian Burgess Shale of British Columbia. Palaeontology, 63, 995–996.
- Caron, J. B., Smith, M., & Harvey, T. H. P. (2013). Beyond the Burgess Shale: Cambrian microfossils track the rise and fall of hallucigeniid lobopodians. Proceedings of the Royal Society B: Biological Sciences, 280, 20131613.
- Chipman, A. D. (2010). Parallel evolution of segmentation by co-option of ancestral gene regulatory networks. BioEssays, 32, 60–70. https://doi.org/10.1002/bies.200900130
- Czerneková, M., & Vinopal, S. (2021). The tardigrade cuticle. Limnological Review, 21, 127–146. https://doi.org/10.2478/limre-2021-0012
- Deline, B., Greenwood, J. M., Clark, J. W., Puttick, M. N., Peterson, K. J., & Donoghue, P. C. J. (2018). Evolution of metazoan morphological disparity. Proceedings of the National Academy of Sciences, 115, E8909–E8918. https://doi.org/10.1073/pnas.1810575115
- Dewel, R. A., Nelson, D. R., & Dewel, W. C. (1993). Tardigrada. In: F. W. Harrison & E. M. Rice (Eds.), Microscopic anatomy of invertebrates: Vol. 12. Onychophora, chilopoda and lesser protostomata (pp. 143–183). Wiley-Liss.
- Dzik, J., & Krumbiegel, G. (1989). The oldest ‘onychophoran’ Xenusion: a link connecting phyla? Lethaia, 22, 169–181. https://doi.org/10.1111/j.1502-3931.1989.tb01679.x
- Edgecombe, G. D. (2020). Arthropod origins: Integrating paleontological and molecular evidence. Annual Review of Ecology, Evolution, and Systematics, 51, 1–25. https://doi.org/10.1146/annurev-ecolsys-011720-124437
- Elzinga, R. J. (1998). Microspines in the alimentary canal of arthropoda, onychophora, annelida. International Journal of Insect Morphology and Embryology, 27, 341–349. https://doi.org/10.1016/S0020-7322(98)00027-0
- Fletcher, T. P., & Collins, D. (1998). The Middle Cambrian Burgess Shale and its relationship to the Stephen Formation in the southern Canadian Rocky Mountains. Canadian Journal of Earth Sciences, 35, 413–436.
- Fletcher, T. P., & Collins, D. (2003). The Burgess Shale and associated Cambrian formations west of the Fossil Gully Fault Zone on Mount Stephen, British Columbia. Canadian Journal of Earth Sciences, 40, 1823–1838. https://doi.org/10.1139/e03-057
- Fontoura, P., Bartels, P. J., Jørgensen, A., Kristensen, R. M., & Hansen, J. G. (2017). A dichotomous key to the genera of the marine heterotardigrades (Tardigrada). Zootaxa, 4294. https://doi.org/10.11646/zootaxa.4294.1.1
- Gaines, R. R., Briggs, D. E. G., & Zhao, Y. L. (2008). Cambrian Burgess Shale-type deposits share a common mode of fossilization. Geology, 36, 755–758. https://doi.org/10.1130/G24961A.1
- García-Bellido, D. C., Edgecombe, G. D., Paterson, J. R., & Ma, X. (2013). A ‘Collins’ monster’-type lobopodian from the Emu Bay Shale Konservat-Lagerstatte (Cambrian), South Australia. Alcheringa, 37, 474–478. https://doi.org/10.1080/03115518.2013.792456
- Gąsiorek, P., & Vončina, K. (2019). New Echiniscidae (Heterotardigrada) from Amber Mountain (Northern Madagascar). Evolutionary Systematics, 3, 29–39. https://doi.org/10.3897/evolsyst.3.33580
- George, R. Y. (1972). Biphasic moulting in isopod Crustacea and the finding of an unusual mode of moulting in the antarctic genus Glyptonotus. Journal of Natural History, 6, 651–656. https://doi.org/10.1080/00222937200770591
- Giribet, G., & Edgecombe, G. D. (2017). Current understanding of Ecdysozoa and its internal phylogenetic relationships. Integrative and Comparative Biology, 57, 455–466. https://doi.org/10.1093/icb/icx072
- Hadley, N., F. (1986). The arthropod cuticle. Scientific American, 255, 104–113. https://doi.org/10.1038/scientificamerican0786-104
- Haug, J., Caron, J. B., & Haug, C. (2013). Demecology in the Cambrian – synchronized molting in arthropods from the Burgess Shale. BMC Biology, 11, 64. https://doi.org/10.1186/1741-7007-11-64
- Hou, X., & Bergström, J. (1995). Cambrian lobopodians—ancestors of extant onychophorans? Zoological Journal of the Linnean Society, 114, 3–19.
- Howard, R. J., Hou, X., Edgecombe, G. D., Salge, T., Shi, X., & Ma, X. (2020). A tube-dwelling early Cambrian lobopodian. Current Biology, 30, 1529–1536.e2. https://doi.org/10.1016/j.cub.2020.01.075
- Hughes, C. L., & Kaufman, T. C. (2002). Hox genes and the evolution of the arthropod body plan. Evolution & Development, 4, 459–499. https://doi.org/10.1046/j.1525-142x.2002.02034.x
- Jockusch, E. L. (2017). Developmental and evolutionary perspectives on the origin and diversification of arthropod appendages. Integrative and Comparative Biology, 57, 533–545. https://doi.org/10.1093/icb/icx063
- Kaczmarek, Ł., Zawierucha, K., Smykla, J., & Michalczyk, Ł. (2012). Tardigrada of the Revdalen (Spitsbergen) with the descriptions of two new species: Bryodelphax parvuspolaris (Heterotardigrada) and Isohypsibius coulsoni (Eutardigrada). Polar Biology, 35, 1013–1026. https://doi.org/10.1007/s00300-011-1149-0
- Kihm, J.-H., Smith, F. W., Kim, S., Rho, H. S., Zhang, X., Liu, J., & Park, T.-Y. S. (2023). Cambrian lobopodians shed light on the origin of the tardigrade body plan. Proceedings of the National Academy of Sciences, 120, e2211251120. https://doi.org/10.1073/pnas.2211251120
- Legg, D. A., Ma, X., Wolfe, J. M., Ortega-Hernández, J., Edgecombe, G. D., & Sutton, M. D. (2011). Lobopodian phylogeny reanalysed. Nature, 476, E1–E1. https://doi.org/10.1038/nature10267
- Lerosey‐Aubril, R., & Ortega‐Hernández, J. (2022). A new lobopodian from the middle Cambrian of Utah: did swimming body flaps convergently evolve in stem‐group arthropods? Papers in Palaeontology, 8, e1450. https://doi.org/10.1002/spp2.1450
- Lewis, P. O. (2001). A likelihood approach to estimating phylogeny from discrete morphological character data. Systematic Biology, 50, 913–925. https://doi.org/10.1080/106351501753462876
- Liu, J., Steiner, M., Dunlop, J. A., Keupp, H., Shu, D., Ou, Q., Han, J., Zhang, Z., & Zhang, X. (2011). An armoured Cambrian lobopodian from China with arthropod-like appendages. Nature, 470, 526–530. https://doi.org/10.1038/nature09704
- Ma, X., Edgecombe, G. D., Legg, D. A., & Hou, X. (2013). The morphology and phylogenetic position of the Cambrian lobopodian Diania cactiformis. Journal of Systematic Palaeontology, 12, 445–457. https://doi.org/10.1080/14772019.2013.770418
- Ma, X., Hou, X., & Bergström, J. (2009a). Morphology of Luolishania longicruris (Lower Cambrian, Chengjiang Lagerstätte, SW China) and the phylogenetic relationships within lobopodians. Arthropod Structure & Development, 38, 271–291. https://doi.org/10.1016/j.asd.2009.03.001
- Ma, X., Hou, X., & Bergström, J. (2009b). Morphology of Luolishania longicruris (Lower Cambrian, Chengjiang Lagerstätte, SW China) and the phylogenetic relationships within lobopodians. Arthropod Structure & Development, 38, 271–291. https://doi.org/10.1016/j.asd.2009.03.001
- Mounce, R. C. P., & Wills, M. A. (2011). Phylogenetic position of Diania challenged. Nature, 476, E1–E1. https://doi.org/10.1038/nature10266
- Moussian, B. (2013). The arthropod cuticle. In A. Minelli, G. Boxshall, & G. Fusco (Eds.), Arthropod Biology and Evolution (pp. 171–196). Springer. https://doi.org/10.1007/978-3-662-45798-6_8
- Moysiuk, J., & Caron, J.-B. (2019). A new hurdiid radiodont from the Burgess Shale evinces the exploitation of Cambrian infaunal food sources. Proceedings of the Royal Society B: Biological Sciences, 286, 20191079. https://doi.org/10.1098/rspb.2019.1079
- Moysiuk, J., & Caron, J.-B. (2022). A three-eyed radiodont with fossilized neuroanatomy informs the origin of the arthropod head and segmentation. Current Biology, 32, 3302–3316.e2. https://doi.org/10.1016/j.cub.2022.06.027
- Nanglu, K., Caron, J.-B., Conway Morris, S., & Cameron, C. B. (2016). Cambrian suspension-feeding tubicolous hemichordates. BMC Biology, 14, 56. https://doi.org/10.1186/s12915-016-0271-4
- Nielsen, C. (1995). Animal evolution interrelationships of the living phyla (p. 467). Oxford University Press.
- O’Brien, L. J., & Caron, J. B. (2012). A new stalked filter-feeder from the Middle Cambrian Burgess Shale, British Columbia, Canada. PLOS ONE, 7, e29233. https://doi.org/10.1371/journal.pone.0029233
- O’Brien, L. J., & Caron, J.-B. (2015). Paleocommunity analysis of the Burgess Shale Tulip Beds, Mount Stephen, British Columbia: Comparison with the Walcott Quarry and implications for community variation in the Burgess Shale. Paleobiology, 42, 27–53. https://doi.org/10.1017/pab.2015.17
- O’Brien, L. J., Caron, J.-B., & Gaines, R. R. (2014). Taphonomy and depositional setting of the Burgess Shale Tulip Beds, Mount Stephen, British Columbia. PALAIOS, 29, 309–324. https://doi.org/10.2110/palo.2013.095
- Peterson, K. J., Dietrich, M. R., & McPeek, M. A. (2009). MicroRNAs and metazoan macroevolution: insights into canalization, complexity, and the Cambrian explosion. BioEssays, 31, 736–747. https://doi.org/10.1002/bies.200900033
- Robson, E. A. (1964). The cuticle of Peripatopsis moseleyi. Quarterly Journal of Microscopical Science, S3-105, 281–299. https://doi.org/10.1242/jcs.s3-105.71.281
- Ronquist, F., Teslenko, M., van der Mark, P., Ayres, D. L., Darling, A., Hohna, S., Larget, B., Liu, L., Suchard, M. A., & Huelsenbeck, J. P. (2012). MrBayes 3.2: Efficient Bayesian phylogenetic inference and model choice across a large model space. Systematic Biology, 61, 539–542. https://doi.org/10.1093/sysbio/sys029
- Shapeero, W. L. (1962). The epidermis and cuticle of Priapulus caudatus Lamarck. Transactions of the American Microscopical Society, 81, 352. https://doi.org/10.2307/3223786
- Smith, F. W., Boothby, T. C., Giovannini, I., Rebecchi, L., Jockusch, E. L., & Goldstein, B. (2016). The compact body plan of tardigrades evolved by the loss of a large body region. Current Biology, 26, 224–229. https://doi.org/10.1016/j.cub.2015.11.059
- Smith, M. R., & Caron, J.-B. (2015). Hallucigenia’s head and the pharyngeal armature of early ecdysozoans. Nature, 523, 75–78. https://doi.org/10.1038/nature14573
- Smith, M. R., & Ortega-Hernández, J. (2014). Hallucigenia’s onychophoran-like claws and the case for Tactopoda. Nature, 514, 363–366. https://doi.org/10.1038/nature13576
- Stayton, C. T. (2015). What does convergent evolution mean? The interpretation of convergence and its implications in the search for limits to evolution. Interface Focus, 5. https://doi.org/10.1098/rsfs.2015.0039
- Vannier, J., Liu, J., Lerosey-Aubril, R., Vinther, J., & Daley, A. C. (2014). Sophisticated digestive systems in early arthropods. Nature Communications, 5, 3641–3641. https://doi.org/10.1038/ncomms4641
- Van Roy, P., Daley, A. C., & Briggs, D. E. G. (2015). Anomalocaridid trunk limb homology revealed by a giant filter-feeder with paired flaps. Nature, 522, 77–80. https://doi.org/10.1038/nature14256
- Whittington, H. B. (1978). The lobopod animal Aysheaia pedunculata Walcott, Middle Cambrian, Burgess Shale, British Columbia. Philosophical Transactions of the Royal Society of London B, 284, 165–197.
- Wolf, H., & Harzsch, S. (2002). Evolution of the arthropod neuromuscular system. 1. Arrangement of muscles and innervation in the walking legs of a scorpion: Vaejovis spinigerus (Wood, 1863) Vaejovidae, Scorpiones, Arachnida. Arthropod Structure & Development, 31, 185–202. https://doi.org/10.1016/S1467-8039(02)00043-9
- Wolff, C., & Scholtz, G. (2008). The clonal composition of biramous and uniramous arthropod limbs. Proceedings of the Royal Society B-Biological Sciences, 275, 1023–1028.
- Yang, J., Ortega-Hernandez, J., Gerber, S., Butterfield, N. J., Hou, J., Lan, T., & Zhang, X. (2015a). A superarmored lobopodian from the Cambrian of China and early disparity in the evolution of Onychophora. Proceedings of the National Academy of Sciences of the United States of America, 112, 8678–8683. https://doi.org/10.1073/pnas.1505596112
- Yang, J., Ortega-Hernandez, J., Gerber, S., Butterfield, N. J., Hou, J., Lan, T., & Zhang, X. (2015b). A superarmored lobopodian from the Cambrian of China and early disparity in the evolution of Onychophora. Proceedings of the National Academy of Sciences of the United States of America, 112, 8678–8683. https://doi.org/10.1073/pnas.1505596112
- Zeng, H., Zhao, F., Niu, K., Zhu, M., & Huang, D. (2020). An early Cambrian euarthropod with radiodont-like raptorial appendages. Nature, 588, 101–105. https://doi.org/10.1038/s41586-020-2883-7