An error occurred in Philosophical Magazine, Volume 92, Issue 11, 11 April 2012, pp. 1349–1368, in the article ‘Mobile effect of hydrogen on intergranular decohesion of iron: first-principles calculations’, by M. Yamaguchi (MY), et al.
For the calculation of a reduction in the cohesive energy (2γint) at a bcc FeΣ3(111) symmetrical tilt grain boundary (GB) by mobile and immobile hydrogen segregation on the GB and its fracture surfaces (FS's), the internal segregation energy terms () have been only considered in Equation (Equation12) in the MY paper, as a first approximation,
This approach was found to be incorrect. Instead, 2γint should be calculated as a free energy difference before and after segregation on GB/FS () that are represented by the internal segregation energy and the temperature-dependent configurational entropy of hydrogen. Therefore, its correct formula can be re-written by
According to Equation (Equationc5), the value of 2γ int for slow and fast fracture decreases monotonically and parallel with increasing log10 C H as shown in (corrected) and (corrected). The onset of slow decohesion occurs above C H = 10−13 atomic fraction (300 K). In a realistic range of C H around 10−5–10−8 atomic fraction in iron and ferritic steels, the re-calculated 2γ int at 300 K decreases about 30–40% at most under the condition of constant chemical potential ( (corrected)). Although the re-calculated 2γ int is not largely reduced for slow fracture especially in a low region of C H, the combined effects of mobile and immobile hydrogen still bring about more prominent GB decohesion of iron, compared with a sole effect of immobile hydrogen. Finally, it should be pointed out that the segregation behavior of hydrogen, influencing the diffusion time, is not changed by the modified analysis of 2γ int, as illustrated in (corrected).
Figure 8 (corrected). Comparison of reduction in the cohesive energy (2γint) of the bcc Fe Σ3(111) GB caused by only immobile effect of hydrogen under the condition of constant composition (fast fracture) and combined effects of mobile and immobile hydrogen under the condition of constant chemical potential (slow fracture) with increasing bulk hydrogen content (C H) at 300 K (a) and 170 K (b).
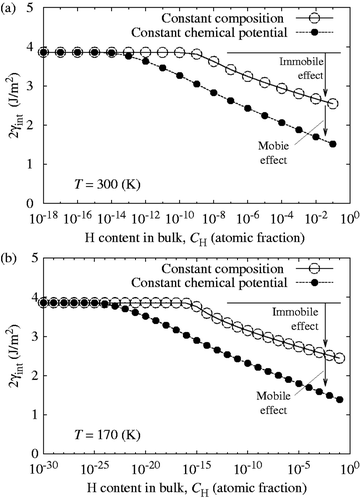