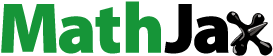
ABSTRACT
The application of HVAC in the present age has affected our lives in much far-reaching range with an extensive variety of applications. HVAC is slowly becoming the pillar of our lifestyle and performs a crucial role in future sustainable growth. Vapour compression refrigeration system is predominantly used in an HVAC sector, which make use of the high-grade energy and causes depletion of ozone layer and global warming due to environmental unfriendly refrigerant. In this regard, ASHRAE has made available a list of low GWP refrigerant for various applications. This paper reviews thermodynamic, flammability properties of these low GWP refrigerants. The compatibility of the low GWP refrigerant with construction material and lubricant is presented.
1. Introduction
The overall market for Heating, Ventilation and Air Conditioning (HVAC) systems is estimated to reach US$241.8 billion by 2025 driven by the ever-present importance of temperature control in homes and production environments (Grand view Research Citation2021). Given that HVACs are important and essential with universal applications in virtually all buildings and factories, their growing carbon footprint is a major area of concern. There is no better system that would replace vapour compression refrigeration system (Atilla Gencer Devecioglu and Oruc Citation2015). The refrigerants are used in HVAC systems to trap and transfer heat from indoor to outdoor. Most of these refrigerants are volatile in nature and also emit greenhouse gases, which are blameable for depletion of earth ozone layer (Sivasakthivel and Siva Kumar Reddy Citation2011). Most of the refrigerants that are currently in use possess high GWP. The currently used refrigerant has 100-year global warming potential of R134a is 1430, R410 is 2088, R22 is1810, R11 is 4000, and hence these refrigerants will be phase out or phase down. Increase in concern about atmosphere urges to use refrigerants which have low GWP and ODP.
It is a high time to replace the refrigerant which has high GWP with suitable alternate refrigerant. The selection of refrigerant based on this single criteria is not acceptable today (Bhatkar, Kriplani, and Awari Citation2013). New candidate refrigerant should possess low GWP, low ODP, should be safe, sustainable, readily available, economically viable and high efficiency (Longo Citation2008; Bobbo et al. Citation2018). Nearly set of 18,400 small molecules were tested by applying screening ratio imposed based on low GWP, less flammable and less toxic. The cut off value 320–420 K is set that is the temperature in general refrigeration and air conditioning systems are designed to work, GWP less than 1000 were set as cut off value. 138 fluids displayed these two conditions. 62 fluids exhibited a GWP value less than 200 (McLinden et al. Citation2017). The evaluation of a good refrigerant should consider several aspects which include thermodynamic, thermo physical properties, environmental issues such as Ozone Depleting Potential (ODP) and Global Warming Potential (GWP), safety issues such as toxicity and flammability, chemical characteristics such as stability and compatibility with the system materials, availability and cost along with energy efficiency. (Longo, Righetti, and Zilio Citation2019).
A new generation of refrigerants were introduced because of scientific findings, regulatory requirement, and market pressure to reduce GHG emission along with low ODP. The new refrigerant should provide high efficiency, along with low GWP, and short atmospheric life time else changes made to reduce low GWP may fail with high GHG emission rather than to reduce net GHG emission (Calm Citation2008). Newly investigated halogenated olefins and those with fluorinated propene isomers have emerged as new refrigerants. Many of these new low-GWP alternatives are classified as A2L refrigerants (mildly flammable). This requires designing system with minimum charge, effective method to detect the leakage, etc. It is possible to design the system considering safety measure with efficiencies equal to or even higher than existing system.
2. Transition of low GWP refrigerant for HVAC
Many refrigerants are used since the advent of vapour compression refrigeration system. With the advancement of refrigeration technology, new substances are subsequently introduced. In the initial stages, Ether was used as a refrigerant. Ammonia, carbon dioxide, water, hydrocarbon, sulphur dioxide, methyl chloride and air were used till 1925. In 1930, American Chemical Society Thomas Midley invented Freon, which gave a solution for long-term search for nontoxic, non-flammable and odourless refrigerant. Thus began an era of chlorofluorocarbons (CFC). It took 50 years to understand its threat to the ozone layer. In 1987 Montreal Protocol, an international agreement was made to phase out CFC. Impact of fluorinated refrigerant on climate change caused a shift to development of new refrigerants in agreement with change in policies. lists specific agreements and their impact on refrigerants.
Table 1. International agreements made year-wise (EDGE Guidance Document for Citation2017).
The Montreal Protocol (EDGE Guidance Document for Citation2017) is one of the most successful environment protection agreement made in recent years. It sets the mandatory timeline for the phase out of ozone depleting substances. This timetable is reviewed regularly and accelerates the date for phase out of refrigerants following advanced technology and the understanding of science. Montreal protocol imposed a restriction on production, usage, and business of CFCs to safeguard the ozone layer. As a result, CFCs which has long atmospheric life has discharged to atmosphere during service/replace causing an alarming increase in concentration of CFC. HFCs are introduced in place of by Montreal Protocols (Ciconkov Citation2018). Further, the study implied a restriction on the usage of HCFC which has a low ODP but high GWP. The European Union (EU) introduced ‘regulation on the usage of certain fluorinated greenhouse gases’ (F-Gas regulation) in 2014. Global framework has been set by the Paris agreement which limits the global warming to well below 2°C and continued efforts to reduce it to 1.5°C. The KIGALI agreement adds to the Montreal Protocol to set the time for phase-down of the production and consumption of HFCs.
2.1. CFC
CFC are chlorofluoro carbons (example R11, R12) are phased out by January 1996 under Montreal protocol as they possess high ODP. ODP has major impact on ozone depletion and global warming as these contains chlorine and fluorine. They have long atmospheric life too.
2.2. HCFC
Hydrochlorofluoro carbons (R22, R123) are ozone depleting substances (ODS) but their contribution is less as they possess low atmospheric life. Their dates (by 2020) for phase out have been scheduled under the Montreal protocol.
2.3. HFC
Hydrofluorocarbons (R-134a, R-245fa, R-125, R-32) are non-ODS, but they do possess high GWPs as they have fluorine content. Currently these refrigerants are targeted for global phase down under the Montreal Protocol by 2030.
2.4. FIC
Fluoroiodocarbons belong to family, which has iodine fluorine, and carbon such as perfluoropropyl iodide and trifluoromethyl iodide. FICs are new refrigerants are blends of fluoroiodocarbons () with hydrocarbons, ethers, HFGs, perfluorocarbons, and/or alkyl chlorides (Nimitz and Lankford Citation2021). These possess zero ODP, negligible GWP and hence short atmospheric life. Their measurements showed better energy efficiency and capacity when compared to CFC 12. These refrigerants can be used as blends.
2.5. HFO
Hydrofluorooelifins (R1234yf, R1234ze) belongs to the family of HFCs. These are derived from unsaturated hydrocarbons molecules. The HFO molecules are designed to facilitate degradation in atmosphere in days rather than years (Majurin et al. Citation2015). HFOs are comparatively stable, and they possess lower atmospheric life and hence less GWP. The efficiency of the HFO systems tends to reduce as the GWP reduces. Adopting HFO refrigerant requires trade-off between GWP and system efficiency (Goetzler et al. Citation2014). The HFO refrigerants appeared as a solution for high GWP refrigerants. The consequences of using HFO are discussed in Section 4.3.
2.6. Natural refrigerants
These are chemicals which are known as refrigerant from nineteenth century. Ammonia (R717), CO2 (R744) and hydrocarbons such as isobutane (R600a) and propane (R290) are the most used refrigerants. The natural refrigerants are seeing an extreme rate of adoption by supermarkets, and food retail chains in the North American and European regions. However, ammonia has a toxicity issue. The CO2 has a challenge of high pressure for operation, requires high engineering standards. But the same can be overruled by adopting a secondary circuit system in refrigeration (Fleet et al. Citation2017). Natural refrigerants do not have fluorine or chlorine, hence will not produce later strong acid, which can run to untimely failure of compressor.
Natural refrigerants fall under a category of A2L and there is concern about safety but other factors such as GWP, ODP, Breakdown by-products, availability, and option in high ambient have no issues. Natural refrigerants seem to be sustainable refrigerant for sustainable environment.
2.6.1. Hydrocarbons
These are natural refrigerants. Hydrocarbons have exceptional thermodynamic properties; hence can be used as refrigerants. Alkane, alcohols, ketones and ethers belong to these categories. Most of the time alkanes are preferred as a refrigerant and these hydrocarbons are flammable (Siddegowda et al. Citation2019). Isobutane, propane and R-441A have been used in small domestic and commercial refrigeration appliances. Being highly flammable, hydrocarbon requires good miscibility with most lubricants at all operating conditions. Pure hydrocarbons (ethane, propane, isobutane) are stable with water (Ikem et al. Citation2018). The positive evaporator pressure of the evaporator ensures no entry of moist air into the system.
Hydrocarbons are said to be combustible under the following conditions. Hydrogen needs to be released to atmosphere, and it should mix with proper proportion of air and exposure to ignition source having surface temperature more than 440°C else source with energy greater than 2.5 × 104 kJ must be present. However, this can be minimised by reducing the refrigerant charge, installing appropriate ventilation and eliminating the source of ignition associated with the system (Bolaji and Huan Citation2013).
2.6.2. Ammonia (R717)
Ammonia is a natural refrigerant. Ammonia is toxic but an environmentally safe fluid. It is gaining revived attention as it has many favourable thermodynamic and thermophysical properties which are essential for refrigeration. It can be effectively used for both vapour absorption and vapour compression refrigeration system. It is used as refrigerant since nineteenth century. Its outstanding working efficiency spans over a wide operating conditions from −40°C freezers to +90°C heat pumps, longevity of installations (typically >20 years) is a major factor in its success. Ammonia based compression system is limited for industrial surrounding where adequate knowledge and facilities are available for handling refrigerant leak. Learning from the various benefits there was a proposal to extend the usage of ammonia plant in areas occupied by common public are limited to avoid accidents and panic aroused in case of leakage. However, a refrigeration installation or a heat pump with more than 10 kg of refrigerant ammonia must meet the PGS 13 directive.
2.6.3. R744 (CO2)
Carbon dioxide is a natural refrigerant. Since early years of refrigeration, carbon dioxide is used as refrigerant. It has very high operating pressure. It is used in combination with ammonia refrigeration to decrease the amount of ammonia in the system. Condensation of CO2 does not take place at constant temperature but at temperature range (temperature glide). Transcritical temperature of CO2 is 31°C. It serves as a best refrigerant when heating and cooling are desired together. Low critical point of CO2 makes the thermodynamic cycle to operate in the single-phase region. Its use in tropical country like India, Mexico is limited because of high condensing pressure.
2.6.4. Water (R718)
Water can also be used as a refrigerant. Water will not damage the environment, it is nontoxic, non-flammable, and it is easily available. Water can be used effectively at high temperature > 100°C. At 100°C, pressure in other refrigerants becomes too high. But it has disadvantage of low density in the gaseous state. This requires large compressor capacity.
3. Basis of selection of a suitable/good refrigerant
The thermodynamic and thermophysical properties of refrigerant (thermal conductivity, vapour pressure, viscosity, density), characteristics of refrigeration cycle at different combinations of suction and condensation temperature (theoretical heating and cooling capacities, influence of superheating/sub cooling on COP, exit temperature of hot gas from the compressor), practical information from new refrigerant (material stability and compatibility, leakage tendency, practical energy performance based on measurements), safety aspects flammability, toxicity; Environmental performance (impact on local and global environment during different lifetime stages); cost aspects. The properties of the refrigerant themselves depend on the operating temperature and pressure of the refrigerant. No ideal refrigerant will meet all the requirements. Trade-off is required in selecting a suitable refrigerant for application.
3.1. Thermodynamic properties
The Refrigerant thermodynamic properties influence the efficiency of thermodynamic cycles. Thermodynamic properties are interconnected, and these are mainly depending on normal boiling point, critical temperature, molecular weight and structure of the molecule. Thus choice is based on system optimisation performance characters of the compressor and refrigeration machine. Normal boiling temperature directly related to operating pressure. Critical temperature of the refrigerant is another most desirable property of the refrigerant (McLinden et al. Citation2017; Hamza and Khan Citation2020). It is evident that refrigerant which has high critical temperature yields higher COP but possess low vapour pressure which in turn reduces volumetric capacity. This involves trade-off between high COP and high volumetric efficiency.
Latent heat of vaporisation and specific heat depend on structure and molecular weight. Trouton’s rule demonstrates that the lower molecular weight refrigerant exhibits high latent heat of vaporisation. Refrigerant molecular structure is associated with the specific heat. Lower specific heat results in higher degree of superheat. Since liquid and vapour super heat are interlinked, it usually results in higher flash gas losses.
3.2. Thermophysical properties
The efficiency of the heat exchanger (condenser and evaporator) in the refrigerating machine is influenced by thermophysical properties. Thermophysical properties include density, viscosity, kinematic viscosity, thermal conductivity, surface tension and other properties. The thermophysical properties, flammability and toxicity are related to the structure of the molecules for halogenated hydrocarbon (Palm Citation2011). They offer hydraulic resistance during flow of liquid and gaseous refrigerant and responsible for intensity of heat and mass transfer in the machine. These properties are also accountable for irreversible losses which affect overall energy efficiency of refrigeration devices and their design characteristics. Liquid viscosity is another transport characteristic of refrigerant, lower viscosity indicates less friction and the system utilises less energy (Atilla Gencer Devecioglu and Oruc Citation2015).
A normal boiling point shows the useful temperature levels as it is directly related to the operating pressures. The refrigerant which has high critical point will have low vapour pressure, smaller compressor superheat and smaller flash gas losses and yields higher COP. Volumetric efficiency will be low because of low volumetric pressure (Venkatarathnam and Srinivasa Murthy Citation2012). The lower specific heat capacity and high adiabatic index of a refrigerant give rise to higher compressor exhaust temperature which results in premature compressor failure (He et al. Citation2014). It is very much essential to ensure that refrigerant does not contain any traces of water, condensable gas and other impurities, as these impurities contained in the refrigerant affect the thermodynamic properties. Specifically, at low pressure the presence of impurities leads to increase the temperature and boiling pressure. State standards impose limits of moisture and other impurities in the refrigerants (Ikem et al. Citation2018).
3.3. Chemical and physiochemical properties
An important chemical property of the refrigerant is the interaction with the lubricating oil and structural material of the system. Chemical stability of the refrigerant is characterised by flammability, explosiveness and decomposition temperature. The decomposition temperature of the refrigerants used in VCR system should be much higher than the temperatures at which the thermodynamic cycles are carried out (Ikem et al. Citation2018). Refrigerant after decomposition gives hydrogen chloride and fluoride along with traces of phosgene. The number of fluorine content in the refrigerant decides the initial temperature at which the decomposition starts and it increases with increase in fluorine content and further depends on the material in contact. The rate of decomposition is higher in the presence of nickel and alloy steels and decreases in the presence of carbon steels (Ikem et al. Citation2018). The presence of lubricating oil will also reduce the thermal stability of the refrigerant. Mineral oils have more deterioration effect than synthetic oils (Ding Citation2007). Selecting the lubricant for refrigeration is limited by solubility in the lubricant (Canter Citation2009). Compressor reliability and efficiency is influenced by miscibility of the lubricant and the refrigerator liquid phase (Canter Citation2009).
3.4. Environmental and physiological properties of refrigerant
There are multiple number of agents in the atmosphere that would contribute to the greenhouse effect and depletion of ozone layer. Refrigerants are the major source for the destruction of the ozone layer (Sivasakthivel and Siva Kumar Reddy Citation2011). It is very much clear from previous studies that refrigerants are harmful to the environment only when they are released to the atmosphere (Pham and Harvey Citation2010; Sivasakthivel and Siva Kumar Reddy Citation2011). It is reported that a mobile air conditioner and large supermarket refrigeration systems will leak up to 30% annually ().
Table 2. Typical lifetimes, charge sizes, and leakage rates for HVAC&R equipment (Goetzler et al. Citation2014).
GWP, TEWI and LCCP are most widely used environmental metrics in refrigerant selection.
3.4.1. Global warming potential (GWP)
GWP is an index, which compares the emission of a greenhouse gas in relation to the impact from the emission of similar amount of CO2. A time horizon of 100 years is mostly adopted. The lesser the GWP, lesser its contribution to global warming ().
Table 3. Classification of 100 year GWP levels (UNEP Citation2019).
3.4.2. TEWI
All energy consuming device will indirectly affect the environment. This is originated from CO2 emission from the energy production device. TEWI accounts for the global warming impact of both direct and indirect emissions and calculated as sum of both. TEWI is an environmental metric which is simpler to use compared to LCCP and more accurate than GWP and very helpful in the selection of an environmental friendly refrigerant (Wypior Citation2014). To avoid ‘unintended consequences’ TEWI should be considered as a comparison metric. A HFO refrigerant with 150 GWP is less efficient than a R32 of GWP 675(Pham and Harvey Citation2010)
where GWP, global warming potential of refrigerant; L, annual leakage rate in the system (3% of refrigerant charge annually); N, life of the system, (15 years), n, system running time, years (based on weather data, 4910 h), Ea, annual energy consumption, (modelled for each refrigerant), β, carbon dioxide emission factor kg CO2-equivalent emissions per kWh (165gCO2/kWh).
3.4.3. Life-cycle climate performance (LCCP)
Analysis is a helpful method to quantify among all Global House Gas (GHG) emissions that occur due to the heat pump based heating system operation through their entire lifetime (Makhnatch Citation2014). It is an effective method as it accounts both direct and indirect emissions during entire lifetime.
where GWP, global warming potential of refrigerant; L, annual leakage rate in the system, kg; n, life of the system, years; Ea, annual energy consumption, kW h y−1; β, carbon dioxide emission factor kg CO2-equivalent emissions per kWh; I, other indirect emissions kg CO2-equivalent; D, other direct emissions kg CO2-equivalent.
The carbon dioxide emissions from a refrigeration system can be divided into two broad categories: direct emission and indirect emission (Beshr et al. Citation2014). The environmental effect of the refrigerant production, transportation and the end of life will be considered as a direct emission. The energy needed for production, transportation and operation of the system are treated as indirect emission. The total emission from the HVAC and commercial refrigeration system can be better evaluated using LCCP tool. It accounts both direct and indirect emissions of the equipment from ‘cradle to grave’.
Charge loss from the equipment will lead to performance deterioration and energy related emissions. This may be treated as a third component, which may exceed the refrigerant related effect in equipment using low GWP refrigerants. This underscores the importance of minimising refrigerant loss from leakage and service even with the usage low GWP refrigerants. Proper equipment installation and maintenance thus needed.
HVAC system can be generally grouped in to residential and commercial refrigeration system. The residential HVAC are important as they are predominant in the market and their energy consumption is high. Thus they account for indirect emission. Whereas commercial refrigeration system holds large refrigerant charge and high rate of leakage and account for direct emission. Several LCCP studies reveals CO2 emission due to electrical energy is major contributing factor for HVAC system lifetime emission (Zhang and Muehlbauer Citation2012; Troch et al. Citation2016). Thus conclusion can be taken that for residential HVAC sector, selecting an energy efficient system will reduce the global warming while for the commercial refrigeration system, moving towards low GWP refrigerant and minimising the leakage rate will contribute more in reducing global warming.
3.4.4. Toxicity and flammability
Flammability and toxicity are important considerations in selecting refrigerant. These properties define refrigerant classifications and practical limits for system for different access categories. Ease of ignition and the pressure rise for ignition in confined spaces determines flammability. Classification of refrigerant is based on Lower Flammability Limit (LFL), Heat of Combustion (HoC) and Burning Velocity. The refrigerant toxicity classification has 400 ppm occupational exposure limit as the divide between higher and lower toxicity refrigerants. presents the flammability and toxicity classification.
4. Alternate refrigerants
To preserve the environment two things need to be done one is to minimise green gas emission and another is to ban the use of coolant pollutant i.e. high GWP refrigerants. Hence CFC, HFC, HCFC were to be replaced by low GWP refrigerants. Primary aim is to use single component refrigerants and of mixtures with significantly reduced GWP, similar thermodynamic, thermophysical properties are to be same as HFC/CFC/HCFC refrigerants. There is a possibility to enhance the thermodynamic properties of the refrigerant by adding nanoparticles. NAno refrigerants are further discussed in section 4.4. To assess the suitability of new refrigerants for use with current HVAC&R system, one needs to check the compatibility of new refrigerant with structural material, lubricants, and for retrofit options.
ASHRAE has made available the list of alternate low GWP refrigerants. lists some of the options available for various RAC sectors referred by ASHRAE. To present the evolution and trends, application approach is the best method (Devin Citation2015). Some of the considered include domestic refrigeration, commercial refrigeration (storage, food industry, other industrial applications), land refrigerated transport, marine refrigerated transport, unitary air conditioning and heat pumps, water chillers and mobile air conditioning (Bhatkar, Kriplani, and Awari Citation2013).
Table 4. Low GWP refrigerants for various RAC sectors referred by ASHRAE (ISHRAE Citation2015).
From the list, it is clear that there are limited options for single component low GWP refrigerant which can be used in small air conditioning, heating and refrigeration, which has a similar volumetric capacity of R404A/R410A (Domanski et al. Citation2017; McLinden et al. Citation2017). Direct alternatives for these refrigerants need to be constructed. A mixture of HFO, HC, CO2 are options available.
But most of these refrigerants belongs to A2L (Mildly flammable) and A3 (Flammable) (). Apart from this HFO belongs to HFC family and have at least one double bond between the carbon atoms. Natural refrigerants are flammable (). The selection of refrigerant should be made judiciously for each application knowing safety and environmental risk, which should include development of leak free and high-efficiency equipment and clear and improved refrigerant handling practices during commissioning of equipment, servicing, and decommissioning.
Table 5. Properties of ASHRAE referred low GWP refrigerants.
4.1. Blends and mixture
Blends or mixtures offer the advantage of tailoring the composition to suit various temperature requirements. Refrigerant blends offer a compromise between flammability and GWP (McLinden et al. Citation2017). Refrigerant blends increase the flexibility to choose trade-off between COP, volumetric efficiency, GWP and flammability (Domanski et al. Citation2017; McLinden et al. Citation2017). Refrigerant blends are used for wide applications. These are used either to replace the existing refrigerant as a drop in or to obtain desired properties such as low ODP, low GWP, bubble point temperature, oil solubility, flammability, etc. These blends have been carefully formulated and mathematically modelled to change their characteristics to match certain properties of the refrigerants they are replacing and to provide maximum system efficiency and performance. The main aim of developing new blends and mixture is to reduce ODP and to keep low or no flammability. The mixture used in refrigeration system can be divided into four categories based on glide, namely azeotropes, near-azeotrope, zoetrope and very wide boiling zeotropes. These groups are proposed based on their normal boiling point for azeotropic mixtures and bubble point value in normal condition for zeotropic mixtures.
4.1.1. Zeotropes
A zeotropic blend is also a mixture of two or more components. The components of this refrigerant have different boiling points. These components will evaporate and condense at different temperatures. An example of a zeotropic blend is the 400 series refrigerants. Hydrocarbon blends are zeotropic blends, which have better potential for energy efficiency and greater potential for capacity potential (Mohanraj, Jayaraj, and Muraleedharan Citation2009a).
4.1.2. Near-azeotrope
Near-azeotrope mixture that shows small temperature variation during phase change and a small difference in composition in liquid and vapour phase at equilibrium. An example for near azeotropes is the addition of small amount of propane to R502 to increase its solubility in lubricating oil. The refrigerants such as R407c and R410a fall under this group. Despite extensive research done for 20 years, R407 is the only successful refrigerant, whose glide is greater than 2 K (R407 has glide of 5–7 K). This sets an example for future research and use of refrigerant mixture.
4.1.3. Azeotrope
An azeotropic refrigerant is a mixture of two or more components that boil at the same temperature. The components in this type of refrigerant will evaporate and condense together as one. There is no glide to worry about with this type of mixture. Azeotrope mixture cannot be separated by simple distillation. An example of an azeotropic refrigerant is R507.
4.1.4. Very wide boiling zeotropes
A very wide boiling zeotropes having glide greater than 6 K will fall under this category. They might have favourable properties such as lower flammability, better thermophysical properties, better oil solubility, etc.
4.2. Technical difficulties in using blends and mixture
Leakage of refrigerants leads to shift in composition of the mixed refrigerant resulting in change of temperature, pressure, efficiency and capacity (Rajapaksha Citation2007).
The occurrence of pinch points in the condenser and evaporator during phase change. This occurs due to nonlinear variation in refrigerant properties results in reduction in effectiveness of condenser and evaporator (Venkatarathnam and Srinivasa Murthy Citation2017).
Non-linearity could influence or limit the potential for decreasing the temperature differences at the inlet/outlet and may lead to an increased heat transfer area requirement to achieve a desired capacity (Rajapaksha Citation2007).
Perfect glide matching requires the use of shell and tube, counter flow, concentric tubes and flat plate heat exchanger.
Mixed refrigerant draws attention towards the new method of heat exchanger design.
Nonlinearity of the refrigerant mixture effects to decrease the difference at inlet and outlet temperature (Mohanraj, Jayaraj, and Muraleedharan Citation2009a).
Mixed refrigerants need suction line accumulator and liquid receiver as composition of mixture change during phase change.
Component selection from manufacturers catalogue will become uncertain because of non-isothermal behaviour of the refrigerant mixture.
4.3. Risk in using HFO and their blends
HFO are mildly flammable. R1234yf, 1234ze are graded as mildly flammable. Their usage in Mobile Air Conditioning [MAC] system could be a potential system. Manufacturing of most HFO molecules is costly as the synthesis of molecules involves more than single process (Calm, Didion, and Didion Citation1997). The products of degradation of HFO depend on the identity of the HFO (Fleet et al. Citation2017). In case of fire, HFO-1234yf will decompose into hydrogen fluoride (HF) and carbonyl fluoride (COF2) which are extremely toxic. Also, in case of leakage in the atmosphere, HFOs breakdown into trifluoroacetic acid (TFA), which has a potential to accumulate and adversely affect ecosystems by increasing their acidity (Hanson et al. Citation2002). To conclude the effect of TFA, several knowledge gaps need to be addressed. The cycle of TFA in the atmosphere and hydrosphere is not fully understood. There is a lack of information on the potential source of TFA and the amount of TFA usage in global ().
Table 6. Decomposition products of HFOs (David Fleet Dr James Hanlon Dr Kate Osborne Max La Vedrine Paul Ashford (Anthesis-Caleb Citation2017).
4.4. Nano refrigerants
The addition of solid nanoparticles in conventional fluid is one of the promising methods to enhance the thermo physical properties of working fluids in thermodynamic cycle. Researchers have showed that nanofluids have better thermal conductivity than host fluid. These nano refrigerants will have better thermal, rheological and tribological properties. The nanoparticles such as copper oxide, titanium dioxide, silicon dioxide, aluminium oxide, copper titanium will be added in conventional refrigerant.
5. Compatibility of low GWP refrigerants
The long-term consistency of HVAC&R system depends on thermal stability of the refrigerant/lubricant and their compatibility with material of construction. The chemistries of new low GWP refrigerants are designed such a way that molecules disintegrate in the atmosphere in days rather than years. This decreased stability in the atmosphere may present possibility of less stability with HVAC&R materials and contaminants. The HFO refrigerant chemistries also present the potential for different interaction between materials of construction, lubricants and also with process chemicals, compatibility of refrigerants are important design parameters to ensure that products are operable for at least 10–25 years ().
It is very important to understand the suitability of low GWP refrigerant with the system material, in this respect two areas need to be investigated and understood. First compatibility of the system material when it is in contact with the fluids and second chemical stability of the fluid when exposed to system materials (Majurin, Gilles, and Staats Citation2014). Extensive work has been done to study fluid properties, thermodynamic properties, and energy efficiency of the low GWP refrigerants. Only few studied material compatibilities with low GWP refrigerants.
Material compatibility and lubricant compatibility for low GWP refrigerants are extensively studied by American Air conditioning heating and refrigeration institute (AHRI). Honeywell and Chemours have published various guides to select suitable material and lubricant for various low GWP refrigerant.
5.1. Material compatibility
For the studies, materials that are currently in use or that are assessed for use in the HVAC&R system are chosen as a sample, while the motor varnish, varnish pucks are used. The testing samples were cut to size and exposed in their received state for testing.
Foe assessing refrigerant chemical accessibility, refrigerant decomposition pathways along with the parameter or conditions that influence decomposition needs to be understood. These considerations then applied to risk assessment. For single component refrigerant, the process of testing is simple straight forward. For refrigerant blends, components and contaminants, the process will be more complex. For contaminant study, oxides of copper and iron, iron chloride with and without water are considered. Testing samples were made to expose for temperature range and observed for specific period. Post evaluation will be done for changes in physical properties such weight, volume, appearance, hardness and tensile performance depending on sample. The material compatibility was presented for new low GWP refrigerants in .
Table 7. The compatibility of the ASHRAE referred low GWP refrigerants with different construction material and Lubricant.
5.2. Oil compatibility
The relationship between the lubricant and the refrigerant is important especially in sealed system, to facilitate the return of oil to the compressor, it is very important that oil and refrigerant should have good miscibility and solubility (Canter Citation2009). Compatibility of HFO, natural refrigerant was available in standard guide released by various refrigerant company. This serves as the basis for selecting the material rather than proof, as materials of different grade are available in the market. lists the compatibility of newly proposed refrigerants with construction material and lubricants. Unsaturated nature of HFO and their blends call for much detailed study (Sebastian et al. Citation2017). Newly introduced HFO molecules compatibility tests are done and the results are available in the open literature whereas for the blends, compatibility with construction material and lubricants are not known much.
lists some of the results for compatibility of new low GWP refrigerants with respect to various oils.
6. Retrofit options for low GWP refrigerants
The Montreal protocol banned use of CFC and introduced HFCs. These HFC are well suited with the CFC systems with small changes in material and lubricant. With retrofit procedure, many CFC systems without change in processes, skill of workers, production line, many system manufacturers switched to HFC. Many studies are done to retrofit HFC/HCFC system into low GWP refrigerant. Both HFO and natural refrigerants are studied for the replacement of high GWP refrigerants.
R134a: R 134a is the most widely used refrigerant in various HVAC sector in medium and high temperature for both commercial and domestic applications.
The saturation pressure of R1234yf is similar to R134a and the 100-year GWP of R1234yf is only 4 (). Low-GWP refrigerant R1234yf as a drop-in replacement for R134a was tested under different heat load conditions in a typical mobile air conditioning system by Zhao et al. (Citation2012). In all working conditions, the cooling capacity of the R134a system was found to be 12.4% larger than that of the R1234yf system, and the COP of the R134a system was only 9% larger. Author suggested redesigning of expansion valve may improve the performance of R1234yf system.
The system performance (COP) with HFO-1234yf as ‘drop in’ is higher than the base case, especially at ambient temperature greater than 30°C when tested for AC of an automotive system and cooling ability is also high when compared to base Refrigerant. This could be a potential to reduce the evaporator size (Mathur Citation2011).
R1234yf and R513a were tested for the replacement of R134a in a split heat pump and exhibited comparable performances to R134a (Pardo and Mondot Citation2018). The discharge temperature is lower than the base refrigerant. Author suggested both R1234yf and R513A can be used as alternative to R134a without significant performance change.
The refrigerants R-1234yf and R-1234ze were tested as drop in refrigerant for R134a in domestic refrigerator by Karber, Abdelaziz, and Vineyard (Citation2012) and Karber, Abdelaziz, and Vineyard (Citation2021) demonstrated that R1234yf had shown higher energy consumption than base refrigerant R134a and R1234ze shows lower energy consumption than R134A and suggested R1234yf can be replacement for R134A whereas to use R1234ze lower capacity needs to be addressed.
The refrigerants HFO R1234yf and HC R290 and R600a are tested in household refrigerator for R12 and R134a (Hmooda et al. Citation2017). The investigation reveals that hydrocarbon (R600a) refrigerant consumes less power than the other two. The COP was better for R600a, than R290 and R1234yf. Hydrocarbon has a flammability issue determined usage as alternative refrigerants. Hence R1234yf, which had high energy consumption, low COP was chosen as a substitute for R134a.
Refrigerant R290/R600a refrigerant mixture was tested and compared as drop-in replacement with CFC12 and HFC134a (Mani and Selladurai Citation2008). The experiment reveals that the refrigerant R290/R600a had 19.9% to 50.1% higher refrigerating capacity than R12 and 28.6% to 87.2% than R134a. The COP of R290/R600a (68/32 by wt%) mixture was higher in the range 3.9–25.1% in the lower evaporating temperatures and 11.8–17.6% higher in the higher evaporating temperatures than R12. With increase in condensing and evaporating temperature efficiency of the refrigerant is found to be increasing and safe during test. Refrigerants R290/R600a (68/32 by wt%) mixture suggested as a drop – in replacement for both R134 and R12.
The performance of a propane/isobutane (R290/R600a) mixture in the composition range of 0.2–0.6 mass mixture was tested as drop – in for domestic refrigerators R12/R134a and found to be suitable for long-term candidate as it yields increased COP of about 2–3% (Jung et al. Citation2000). During testing refrigeration components stayed the same throughout the tests, except for the length of the capillary tube and amount of charge was changed.
Hydrocarbon refrigerant R270, R290, R1270 and Di Methyl Ether (DME) are tested as alternate for R134a in domestic refrigerator and found to be better replacement for CFC based refrigerants and have excellent performance (Parashurama et al. Citation2019). The refrigerant R270 suggested for direct substitute. Refrigerants R290, R1270 are recommended as alternatives to R134a with lower displacement compressor. The DME can be used with higher displacement compressor.
The hydrocarbon mixtures of R290/R600 and R290/R600a were experimentally investigated as a drop-in alternatives to replace R134a in 297 L domestic refrigerator designed to work with R134a (Mohanraj et al. Citation2009b). The results showed better freezer air temperature, cabinet air temperature, discharge temperature, coefficient of performance, lower pressure ratio and lower electrical power.
The natural Refrigerant Propane and their mixture Propane/Isobutane are tested for R134a in large Capacity Chest Freezer tested by (He et al. Citation2014). During testing it is found that the COP of R290 lower than that of R134a, but volumetric efficiency of R290 is higher than R134a. The power consumption of R290 is lower by 26.7% than R134a during test. The COP of R290 was lower than R600a. The authors suggested the right mixture of R290/R600a would increase the energy conversion efficiency and recommended a proportion of R290/R600a is 93.75/6.25 wt%, which considerably deviates from the theoretical ratio of 30/70 wt%.
R410a: R410a is an HFC blend designed for new R22 applications. R410A is the dominant refrigerant in residential heat pumps. Even though refrigerant R410A does not contribute to the depletion of the ozone layer it has a GWP (AR5) of 1924. The refrigerant permits to reduce the GWP from 2090 kg eq CO2 to 675 kg eq CO2. Pure synthetic and natural refrigerants such as R32 or propane (R290) can be used as alternate refrigerant, but their thermodynamic properties are different and a mixture of HFC/HFO mixture R452B and R454B are proposed as alternate refrigerant. The performance test was done on multiple options like Split air conditioner, Water-to-air heat pump, Ducted heat pump, Roof top Air conditioner using Fixed Scroll compressor and thermal expansion valve.
The Performance test of DR-55(R452B) as a drop-in replacement for R-410A in a high efficiency rooftop air conditioning unit proved 5% higher efficiency. DR-55(R452B) also showed significantly better high ambient performance from 95°F to 125°F and proposed as a direct design-compatible replacement for R410A (Shen, Abdelaziz, and Liudahl Citation2016).
The performance of R452B and R454B in an R410a liquid-to-water heat pump was done (Sieres et al. Citation2021). The lower GWP refrigerants showed present higher discharge temperature, lower heating, and compression power. It is shown in the experiment that low GWP refrigerant requires less refrigerant charge than the R410A in drop in performance analysis.
R459A (R134A, R1234yf/ and 1234ze in proportion of 68%/26%/6%), R454B (R32/ R1234yf in proportion of 68.9%/31.1%), R447A (R32/R1234ze(E)/R125 in proportion of (68/28.5/3.5%wt)), HPR2A (R32/R134a/R1234ze in proportion (76/6/18%wt)) and R32 were investigated for the drop-in replacement of R410A in air to-water reversible heat pump. R454B and R459A exhibited the best performances. R32 can be considered as drop-in replacement for R410A, but it showed higher discharge temperature than base refrigerant (Pardo and Mondot Citation2018).
The performance parameter such as cooling/heating abilities and EER/COP values depends on the testing unit and operating conditions. From the research, it is clear that ability of lower GWP refrigerants somehow lower than 410A and EER and COP values are slightly higher or like R410A. The compressor discharge temperature is higher for Alternative than R410A (Mota-Babiloni et al. Citation2018). Refrigerants R454C and R455A are found to be alternate refrigerant for R410A for warm countries.. The authors also suggest that the usage of IHX is not recommended with the alternatives due to the increased discharge temperature and the low energy performance benefit.
The experimental study conducted by Mota-Babiloni et al. (Citation2016) demonstrates the cooling capacity of the replacement refrigerant R1234ze is slightly lower than R404A, and the Coefficient of Performance (COP) of the proposed refrigerant is 10–15% higher than that of R404A, at higher condensation temperatures. The authors also suggest that the usage of IHX is not recommended with the alternatives due to the increased discharge temperature and the low energy performance benefit.
Retrofit test of R-410A in Air to Water Heat Pumps with low GWP blends ARM70a (R32/R134a/R1234yf by mass in percentage (50/10/40)) and DR5(Zoughaib and Besbes). Test results show that heating capacity is more with the DR-5(R32/R1234yf by mass in percentage (72.5/27.5)) about 10% compared to the R-410A. ARM-70a gives equivalent to higher heating capacities compared to R-410A. For both alternative low GWP refrigerants, system COP is improved by 5–10%.
Drop-in tests were carried out to assess the heat pump performance of 8 low-GWP alternative refrigerants for R134a, R410A and R407c were evaluated (Pardo and Mondot Citation2018). The refrigerants R459A, R454B, R447A, HPR2A and R32 were investigated for the drop-in replacement of R410A. The HFC/HFO mixtures posed no problem and the performance almost equivalent (±10%) to that with R410A and R32 suggested as a drop in refrigerant. R454C was assessed as a possible alternative to R407C. R454C offered equivalent or greater capacities (−0.5% to +7.1%) and lower or equivalent COP (−5 to – 0.6%) than R407C. The refrigerant R1234yf and R513A were tested for the replacement of R134a in a split heat pump water heater and both the refrigerant showed similar performance and suggested as alternative refrigerant for R134a.
The Drop-in Tests of Refrigerant R-32 in Single Packaged Vertical Heat Pump for R410A (Wuesthoff and Antonio Citation2015). The alternate refrigerant was like the R410A system, with no major equipment changes. Subcooling of the outdoor circuit with tuning of metering device may improve the capacity and COP of the system with drop in refrigerant. Optimising refrigerant charge is not done.
The refrigerant HC-290 was tested as a drop-in substitute to HCFC-22 in a window air conditioner. The refrigerant HC-290 had 6.6% lower cooling capacity for the lower operating conditions and 9.7% lower for the higher operating conditions with respect to HCFC-22. The coefficient of performance for HC290 was 7.9% higher for the lower operating conditions and 2.8% higher for the higher operating conditions and found to be suitable refrigerant for R22 in all terms like condenser, evaporator exit temperature, energy consumption, pressure ratio and found to have lower condenser and evaporator capacity when compared to R22.
The drop-in performance analysis of refrigerant R1234yf for R134A was revealed superheat of the R1234yf was more, which necessitates the redesigning of expansion valve (Zhao et al. Citation2012).
It is clear from the review, drop in analysis of low GWP refrigerant alone not sufficient to judge the overall performance of alternative refrigerant as thermodynamic and transport properties of refrigerant may require different heat exchanger configuration such as cross flow, Parallel flow, different arrangement of expansion valve (Shen, Abdelaziz, and Shrestha Citation2008). Many authors have not shared these details. Drop-in analysis of low GWP refrigerant analysis can be summarised as below.
With the usage of low GWP refrigerant, amount of refrigerant charge reduced when compared to base refrigerant.
Hydrocarbon refrigerant consumes less energy when compared to HFO.
Flammability issue of hydrocarbon addressed properly, then it will be a better choice than HFO as hydrocarbon refrigerant performance for R134a as an alternate refrigerant in domestic refrigerator found to be better replacements for CFC based refrigerant (Jung et al. Citation2000; Sattar, Saidur, and Masjuki Citation2007; Agrawal, Patil, and Nanda Citation2017)
COP of few low GWP refrigerants was less than base refrigerant.
Tuning of expansion valve, revising the length of capillary tube, right heat exchanger configuration might improve the performance.
Right proportion mixture composition improves the conversion efficiency.
Optimising refrigerant charge mixture proportion is a tedious task, optimised proportion given by experimental analysis considerably deviates from the theoretical analysis.
Most of the retrofit test are done to study the energy consumption, COP, cooling capacity, and compressor exit temperature. Compatibility test with Structure material, lubricant stability analysis are not done much for blends.
The refrigerants ammonia (R717) and carbon dioxide (R744) can only be considered in new refrigeration facilities, not as direct drop-ins, due to the properties of the refrigerants, i.e. material compatibility and high-pressure operating conditions.
7. Result and discussion
Much focus is needed in addressing safety and environmental concern for compounds with minimal undesirable effect instead of phaseout. Random elimination of whole group of compounds, without regard to compensating the benefits of low concern refrigerant, enforce fewer desirable compromises later.
Emission of refrigerant can be reduced by recovery of refrigerant, recycling and reclamation. This helps in prompt phase out of halogenated refrigerants.
Fluorocarbons Life Cycle Management needs initiative to proper disposal of fluorocarbons.
The leakage rate of large supermarket and mobile air conditioning may be fixed by incorporating proper design modification.
Most of the new low GWP refrigerants falls under A2L (mildly flammable) category and requires lower charge, restricts the usage of its kind in large installations.
Flammability of a natural refrigerant can be tackled through proper product design and maintenance of equipment. This calls for keeping a safety levels and minimising risks. Standards, codes and legislation should be adapted to technological progress and allow the use of more climate-friendly alternatives.
Hydrocarbon refrigerants can be used in small and medium commercial applications with following a firm rule. Heat pumps which are located outdoors can be used hydrocarbon-based refrigerants safely. In other systems, the use of hydrocarbon refrigerants is challenged by cost of mechanical and electrical safety setting.
Establishing a fund for aiding and organisation of trainings on employing natural refrigerants in the developing countries may help in usage of natural refrigerants.
8. Conclusion
Scientific findings, regulatory requirement and market pressure to reduce GHG emission along with low ODP gave rise to the new refrigerants. This new generation refrigerants bound to reduce global warming and should possess short atmospheric life along with energy efficiency. The thermodynamic and thermophysical properties influence the efficiency of thermodynamic cycle, performance of refrigeration device and compressors. Refrigerant choice should be made considering energy efficiency, life cycle cost along with environmental cost.
For residential HVAC sector, selecting an energy efficient system will reduce the global warming while for the commercial refrigeration system, moving towards low GWP refrigerant and minimising the leakage rate will contribute more to reduce global warming.
Impact of fluorinated refrigerant on climate change triggered a swing to the development of new refrigerants in agreement with change in policies. Montreal protocol sets the time frame for phase out of CFC, HCFC and HFC refrigerants. The HFO refrigerants and their blends have appeared as a replacement for these refrigerants. The decomposition products of HFO and their blends include TFA which has a potential to accumulate. TFA effect on life on environment needs further investigation.
Blends have a technical issue such as occurrence of pinch point in the condenser and evaporator, nonlinear variation of refrigerant properties. This problem can be overcome by implementing suitable design modifications and incorporating added component such as accumulator and receiver.
The use of natural refrigerants such as carbon dioxide, ammonia and hydrocarbons in vapour compression system should be entertained as they are more environmentally friendly refrigerants, however, their flammability issue requires safe equipment design, safe handling, servicing and transportation practices along with safe refrigerant charge.
Material and oil compatibility test for low GWP refrigerants blends are to be done on priority.
Retrofit experiment analysis reveals even HFO refrigerant requires component modification to enhance the efficiency.
Glossary of terms
Glide: | = | Glide is the difference between the boiling point of the most volatile component compared to the least volatile component within the refrigerant blend. |
Dew point: | = | The hottest temperature of the temperature glide is known as the dew point. |
Bubble point: | = | The lowest temperature of the temperature glide is known as the bubble point. |
PGS 13 directive: | = | This is the directive for the safe use of ammonia in refrigeration installations and heat pumps. The directive sets requirements for the preparation, management, and maintenance of the installation. |
ASHRAE: | = | American Society of Heating, Refrigerating and Air Conditioning Engineers |
AB: | = | Alkyl benzene |
BV: | = | Burning Velocity |
COP: | = | Co-efficient of performance |
EPDM: | = | Ethylene-Propylene-Diene Rubber |
EU: | = | European Union |
FIC: | = | Fluoro Iodo Carbons |
FKM: | = | fluoro rubber |
GHG: | = | Global House Gas |
GWP: | = | Global Warming Potential |
HC: | = | Hydrocarbons |
HCFC: | = | Hydrochlorofluorocarbon |
HFC: | = | Hydrofluorocarbon |
HFO: | = | Hydrofluoroolefin |
HoC: | = | Heat of combustion |
HVAC: | = | Heating, Ventilation, Air Conditioning |
LCCP: | = | Life Cycle Climate Performance |
LFL: | = | Lower Flammability Limit |
NBR: | = | Nitrile Butadiene Rubber |
PAG: | = | Poly Alkylene Glycol |
PAO: | = | Poly-alpha-olefin |
POE: | = | polyol ester |
PTFE: | = | polytetrafluoroethylene |
PVE: | = | polyvinyl ether |
VCR: | = | Vapour Compression Refrigeration |
VOF: | = | Volatile organic compounds |
TEWI: | = | Total Equivalent warming Impact |
TFA: | = | Trifluoro acetic acid |
Disclosure statement
No potential conflict of interest was reported by the author(s).
References
- Agrawal, Neeraj, Shriganesh Patil, and Prasant Nanda. 2017. “Experimental Studies of a Domestic Refrigerator Using R290/R600a Zeotropic Blends.” Energy Procedia 109 (November 2016): 425–430. doi:https://doi.org/10.1016/j.egypro.2017.03.051
- Ally, Moonis R, Vishaldeep Sharma, and Kashif Nawaz. 2019. “Analysis of the P-T and T-S Phase Boundary in Discriminating Suitable Low Global Warming Potential and Low Flammability Refrigerants 25th IIR International Congress of Refrigeration (ICR 2019), Montreal, Canada.
- Beshr, M., V. Aute, V. Sharma, O. Abdelaziz, B. Fricke, R. Radermacher, Oak Ridge, Transportation Science Division. 2014. “A Comparative Study on the Environmental Impact of Supermarket Refrigeration Systems Using low GWP Refrigerants”.
- Bhatkar, V. W., V. M. Kriplani, and G. K. Awari. 2013. “Alternative Refrigerants in Vapour Compression Refrigeration Cycle for Sustainable Environment: A Review of Recent Research.” International Journal of Environmental Science and Technology 10 (4): 871–880. doi:https://doi.org/10.1007/s13762-013-0202-7
- Bobbo, Sergio, Giovanni Di Nicola, Claudio Zilio, J. Steven Brown, and Laura Fedele. 2018. “Low GWP Halocarbon Refrigerants: A Review of Thermophysical Properties.” International Journal of Refrigeration 90: 181–201. Elsevier Ltd. doi:https://doi.org/10.1016/j.ijrefrig.2018.03.027.
- Bolaji, B. O., and Z. Huan. 2013. “Ozone Depletion and Global Warming: Case for the Use of Natural Refrigerant – A Review.” Renewable and Sustainable Energy Reviews 18: 49–54. doi:https://doi.org/10.1016/j.rser.2012.10.008
- Calm, James M. 2008. “The Next Generation of Refrigerants – Historical Review, Considerations, and Outlook.” International Journal of Refrigeration 31: 1123–1133.
- Calm, James M., David A. Didion, Pe A David Didion, and Pe Fellow ASHRAE. 1997. “Trade-Offs in Refrigerant Selections: Past, Present, and Future.” Refrigerants for the 21st Century ASHRAE/NIST Refrigerants Conference, 1–16. https://www.sciencedirect.com/science/article/pii/S0140700797000893
- Canter, Dr Neil. 2009. “Lubricants : Transitioning to New Refrigerants.” Tribology and Lubrication Technology 30 (December): 30–39.
- Cho, Honghuyun, and Piotr A Domanski. 2016. “Optimized Air-to-Refrigerant Heat Exchanger with Low-GWP Refrigerants.” 12th IIR Gustav Lorentzen Conference on Natural Working Fluids, 290:1–8.
- Choudhari, C. S., and S. N. Sapali. 2017. “Performance Investigation of Natural Refrigerant R290 as a Substitute to R22 in Refrigeration Systems.” Energy Procedia 109 (November 2016): 346–352.doi:https://doi.org/10.1016/j.egypro.2017.03.084
- Ciconkov, Risto. 2018. “Refrigerants: There Is Still No Vision for Sustainable Solutions.” International Journal of Refrigeration 86: 441–448. doi:https://doi.org/10.1016/j.ijrefrig.2017.12.006
- Devecioglu, Atilla Gencer, and Vedat Oruc. 2015. “Characteristics of Some New Generation Refrigerants with Low GWP.” Energy Procedia 75: 1452–1457. doi:https://doi.org/10.1016/j.egypro.2015.07.258
- Devin, Eric. 2015. Refrigerant Containment Study.
- Ding, Guo liang. 2007. “Recent Developments in Simulation Techniques for Vapour-Compression Refrigeration Systems.” International Journal of Refrigeration. doi:https://doi.org/10.1016/j.ijrefrig.2007.02.001
- Domanski, Piotr A., Riccardo Brignoli, J. Steven Brown, Andrei F. Kazakov, and Mark O. McLinden. 2017. “Low-Gwp Refrigerants for Medium and High-Pressure Applications.” International Journal of Refrigeration 84: 198–209. doi:https://doi.org/10.1016/j.ijrefrig.2017.08.019.LOW-GWP
- EDGE Guidance Document for. 2017. Refrigerant Selection to Reduce Climate Impact.
- Eyerer, Sebastian, Peter Eyerer, Markus Eicheldinger, and Beatrice Tübke. 2018. “Theoretical Analysis and Experimental Investigation of Material Compatibility Between Refrigerants and Polymers Sebastian.” Energy Elsevier Ltd 10: 1–23. doi:https://doi.org/10.1016/j.energy.2018.08.142
- Fleet, David, Dr James Hanlon, Dr Kate Osborne, Max La Vedrine, and Paul Ashford. 2017. Study on Environmental and Health Effects of HFO Refrigerants (Publication Number: M-917|2017).
- Fleet Dr, David. 2020. Study on environmental and health effects of HFO refrigerants Other Info.
- Goetzler, W., T. sutherland, M. Rassi, and J. Burgos. 2014. Roadmap for Next-Generation Low Global Warming Potential Refrigerants.
- Grand view Research. 2021. HVAC Systems Market Size | Industry Analysis Report, 2020-2027. Accessed March 15. https://www.grandviewresearch.com/industry-analysis/hvac-equipment-industry.
- Hamza, Ameer, and Tauseef Aized Khan. 2020. “Comparative Performance of Low-GWP Refrigerants as Substitutes for R134a in a Vapor Compression Refrigeration System.” Arabian Journal for Science and Engineering 45 (7): 5697–5712. doi:https://doi.org/10.1007/s13369-020-04525-3
- Hanson, Mark L., Paul K. Sibley, Scott A. Mabury, Keith R. Solomon, and Derek C.G. Muir. 2002. “Trichloroacetic Acid (TCA) and Trifluoroacetic Acid (TFA) Mixture Toxicity to the Macrophytes Myriophyllum Spicatum and Myriophyllum Sibiricum in Aquatic Microcosms.” Science of the Total Environment 285 (1–3): 247–259. doi:https://doi.org/10.1016/S0048-9697(01)00955-X
- He, Mao Gang, Xin Zhou Song, Huan Liu, and Ying Zhang. 2014. “Application of Natural Refrigerant Propane and Propane/Isobutane in Large Capacity Chest Freezer.” Applied Thermal Engineering 70 (1): 732–736. doi:https://doi.org/10.1016/j.applthermaleng.2014.05.097
- Hmooda, Kamel S., H. Pop, V. Apostol, and Ahmed Q. Ahmed. 2017. “Refrigerants Retrofit as Alternative for R12 and R134a in Household Refrigerators.” American Scientific Research Journal for Engineering, Technology, and Sciences (ASRJETS) 35 (1): 251–265.
- Ikem, A. Ikem, Paschal A Ubi, Matthew I Ibeh, Saviour E Ofem, and Assam T. Assam. 2018. “Review of Refrigerants for Steam Compression Refrigeration Machines.” International Journal of Engineering and Technology 10 (4): 1172–1180. doi:https://doi.org/10.21817/ijet/2018/v10i4/181004056
- ISHRAE. 2015. ISHRAE-Position-Documents-Ref.Pdf.
- Juhasz, Jason R. 2017. “Novel Working Fluid, HFO-1336mzz (E), for Use in Waste Heat Recovery Application.” 12th IEA Heat Pump Conference, 1–10.
- Jung, Dongsoo, Chong Bo Kim, Kilhong Song, and Byoungjin Park. 2000. “Testing of Propane/Isobutane Mixture in Domestic Refrigerators.” International Journal of Refrigeration 23 (7): 517–527. doi:https://doi.org/10.1016/S0140-7007(99)00084-5
- Karber, Kyle M, Omar Abdelaziz, and Edward Allan Vineyard. 2012. “Experimental Performance of R-1234yf and R-1234ze as Drop-in Replacements for R-134a in Domestic Refrigerators.” International Refrigeration and Air Conditioning Conference at Purdue, West Lafayette, IN, USA, July 16–19: 1–10.
- Karber, Kyle Melvin, Omar Abdelaziz, and Edward A Vineyard. 2021. Experimental Performance of R-1234yf and R-1234ze as Drop-in Replacements for R-134a in Domestic Refrigerators. Academia.Edu. Accessed February 9. https://www.academia.edu/download/30415507/pub36081.pdf.
- Longo, Giovanni A. 2008. “Refrigerant R134a Condensation Heat Transfer and Pressure Drop Inside a Small Brazed Plate Heat Exchanger.” International Journal of Refrigeration 31 (5). doi:https://doi.org/10.1016/j.ijrefrig.2007.11.017.
- Longo, Giovanni A., Giulia Righetti, and Claudio Zilio. 2019. “Heat-Transfer Assessment of the Low GWP Substitutes for Traditional HFC Refrigerants.” International Journal of Heat and Mass Transfer 139: 31–38. doi:https://doi.org/10.1016/j.ijheatmasstransfer.2019.04.144
- Majurin, Julie A, William Gilles, and Steven J Staats. 2014. “Materials Compatibility of HVACR System Materials with Low GWP Refrigerants.” International Refrigeration and Air Conditioning and Air Conditioning conference, 2132–2141.
- Majurin, Julie, Steven J Staats, Elyse Sorenson, William Gilles, Julie Majurin, Steven J Staats, Elyse Sorenson, et al. 2015. “Material Compatibility of HVAC&R System Materials with low Global Warming Potential Refrigerants.” Science and Technology for the Built Environment 21: 491–501. doi:https://doi.org/10.1080/23744731.2015.1009353
- Makhnatch, Pavel. 2014. “Refrigerants with Low GWP and Cost and Energy Efficiency Optimization of Vapor Compression Systems.” Thesis, no. June.
- Makhnatch, Pavel. 2019. “New Refrigerants for Vapour Compression Refrigeration and Heat Pump Systems”.
- Mani, K., and V. Selladurai. 2008. “Experimental Analysis of a New Refrigerant Mixture as Drop-in Replacement for CFC12 and HFC134a.” International Journal of Thermal Sciences 47 (11): 1490–1495. doi:https://doi.org/10.1016/j.ijthermalsci.2007.11.008
- Mathur, Gursaran D. 2011. “Experimental Investigation of the Performance of a Laminate Evaporator with HFO-1234yf as the Working Fluid.” SAE International Journal of Materials and Manufacturing 4 (1): 1231–1243. doi:https://doi.org/10.4271/2011-01-1170
- McLinden, Mark O., J. Steven Brown, Riccardo Brignoli, Andrei F. Kazakov, and Piotr A. Domanski. 2017. “In Situ Click Chemistry Generation of Cyclooxygenase-2 Inhibitors.” Nature Communications 8: 1–9. Nature Publishing Group. doi:https://doi.org/10.1038/ncomms14476
- Mohanraj, M., S. Jayaraj, and C. Muraleedharan. 2009a. “Environment Friendly Alternatives to Halogenated Refrigerants—A Review.” International Journal of Greenhouse Gas Control 3: 108–119. doi:https://doi.org/10.1016/j.ijggc.2008.07.003
- Mohanraj, M., S. Jayaraj, C. Muraleedharan, and P. Chandrasekar. 2009b. “Experimental Investigation of R290/R600a Mixture as an Alternative to R134a in a Domestic Refrigerator.” International Journal of Thermal Sciences 48 (5): 1036–1042. doi:https://doi.org/10.1016/j.ijthermalsci.2008.08.001
- Mota-Babiloni, Adrián, Jorge Haro-Ortuño, Joaquín Navarro-Esbrí, and Ángel Barragán-Cervera. 2018. “Experimental Drop-in Replacement of R404A for Warm Countries Using the Low GWP Mixtures R454C and R455A.” International Journal of Refrigeration 91 (July): 136–145. doi:https://doi.org/10.1016/j.ijrefrig.2018.05.018
- Mota-Babiloni, Adrián, Joaquín Navarro-Esbrí, Francisco Molés, Ángel Barragán Cervera, Bernardo Peris, and Gumersindo Verdú. 2016. “A Review of Refrigerant R1234ze(E) Recent Investigations.” Applied Thermal Engineering. doi:https://doi.org/10.1016/j.applthermaleng.2015.09.055
- Nimitz, J., and L. Lankford. 2021. Purdue E-Pubs Refrigerants Containing Fluoroiodocarbons (FICs). Accessed February 23. http://docs.lib.purdue.edu/iracc/256.
- Palm, Björn. 2011. “Refrigerants of the Future.” 10th IEA Heat Pump Conference, 1–12.
- Parashurama, S., M. S. Govindegowda, Vaibhav Jain, and S. B. Anjappa. 2019. “Hydrocarbons as Alternate Refrigerants in Domestic Refrigerators- A n Overview.” Indian Journal of Environmental Protection 39 (12): 1119–1124.
- Pardo, Pierre, and Michèle Mondot. 2018. “Experimental Evaluation of R410A, R407C and R134a Alternative Refrigerants in Residential Heat Pumps.” International Refrigeration and Air Conditioning Conference, Paper 2498. https://docs.lib.purdue.edu/cgi/viewcontent.cgi?article=2990&context=iracc
- Pham, Hung, and Sachs Harvey. 2010. “Next Generation Refrigerants : Standards and Climate Policy Implications of Engineering Constraints ACEEE , summer Study on Energy Efficiency in Buildings.California, USA.15-20 Aug.
- Rajapaksha, Leelananda. 2007. “Influence of Special Attributes of Zeotropic Refrigerant Mixtures on Design and Operation of Vapour Compression Refrigeration and Heat Pump Systems.” Energy Conversion and Management 48 (2): 539–545. doi:https://doi.org/10.1016/j.enconman.2006.06.001
- Sarbu, Ioan, and Emilian Stefan Valea. 2014. “Ecological Refrigerants Used in Refrigeration, Air- Conditioning and Heat Pump Systems.” Proceedings of the 2014 International Conference on power systems, energy environment, 178–184.
- Sattar, M. A., R. Saidur, and H. H. Masjuki. 2007. “Butane, Isobutane and Their Mixtures as an Alterantives to R-134a in Domestic Refrigerator R-134a IN DOMESTIC REFRIGERATOR,” no. December 2013.
- Sebastian, Eyerer, Peter Eyerer, Markus Eicheldinger, Sebastian Sax, Christoph Wieland, and Hartmut Spliethoff. 2017. “Material Compatibility of ORC Working Fluids with Polymers.” Energy Procedia 129: 137–144. doi:https://doi.org/10.1016/j.egypro.2017.09.189
- Shen, Bo, Omar Abdelaziz, and Lane Liudahl. 2016. “Assessment of DR-55 as a Drop-in Replacement for R-410A.” 16th International Refrigeration and Air Conditioning Conference at purdue. http://docs.lib.purdue.edu/iracc%0Ahttp://docs.lib.purdue.edu/iracc/1708
- Shen, Bo, Omar Abdelaziz, and Som Shrestha. 2008. “Performance Optimization of Alternative Lower Global Warming Potential Refrigerants in Mini-Split Room Air Conditioners”.
- Siddegowda, Parashurama, Govindegowda Mundur Sannappagowda, Vaibhav Jain, and Satheesh Javare Gowda. 2019. “Revue Des Composites et Des Matériaux Avancés Hydrocarbons as Alternate Refrigerants to Replace R134a in Domestic Refrigerators.” International Information Engineering Technology Association 29 (2): 95–99.
- Sieres, Jaime, Ignacio Ortega, Fernando Cerdeira, and Estrella Álvarez. 2021. “Drop-in Performance of the Low-GWP Alternative Refrigerants R452B and R454B in an R410A Liquid-to-Water Heat Pump.” Applied Thermal Engineering 182 (July 2020). doi:https://doi.org/10.1016/j.applthermaleng.2020.116049
- Sivasakthivel, T., and K. K. Siva Kumar Reddy. 2011. “Ozone Layer Depletion and Its Effects : A Review.” International Journal of Environmental Science and Development 2 (1): 30–37.
- Troch, Sarah, Hoseong Lee, Yunho Hwang, and Reinhard Radermacher. 2016. “Harmonization of the Life Cycle Climate Performance Methodology.” International Refrigeration and Air Conditioning Conference 2016 (January): 1–8. https://docs.lib.purdue.edu/iracc/1724.
- UNEP. 2019. Refrigeration, Air Conditioning and Heat Pumps Technical Options Committe 2018 Assessment Report. https://ozone.unep.org/sites/default/files/2019-04/RTOC-assessment-report-2018_0.pdf.
- Venkatarathnam, G., and S. Srinivasa Murthy. 2012. “Refrigerants for Vapour Compression Refrigeration Systems.” Resonance 17 (February): 139–162. doi:https://doi.org/10.1201/9781315368184-16
- Venkatarathnam, G., and S. Srinivasa Murthy. 2017. “Effect of Mixture Composition on the Formation of Pinch Points in Condensers and Evaporators for Zeotropic Refrigerant Mixturess.” International Journal of Refrigeration 55 (11): 205–215.
- Wuesthoff, Ed, and San Antonio. 2015. System Drop-in Tests of Refrigerant R-32 in Single Packaged Vertical Heat Pump (SPVH).
- Wypior, Markus. 2014. “Refrigerators and Air-Conditioners with Natural Refrigerants – a Safe, Energy Efficient and Climate Friendly Solution.” Centre for Science and environment’s media briefing workshop.
- Zhang, Ming, and Jan Muehlbauer. 2012. “Life Cycle Climate Performance Model for Residential Heat Pump Systems.” International Refrigeration and Air Conditioning Conference. Paper 1311. http://docs.lib.purdue.edu/iracc/1311/
- Zhao, Yu, Zhaogang Qi, Jiangping Chen, Baixing Xu, and Bin He. 2012. “Experimental Analysis of the Low-GWP Refrigerant R1234yf as a Drop-in Replacement for R134a in a Typical Mobile Air Conditioning System.” Proceedings of the Institution of Mechanical Engineers, Part C: Journal of Mechanical Engineering Science 226 (11): 2713–2725. doi:https://doi.org/10.1177/0954406211435583
- Zoughaib, Assaad, and Karim Besbes. “Retrofit of R-410A in Air to Water Heat Pumps: Test of Two Low GWP Candidates,” 1–6.