ABSTRACT
During the production and processing of coal, burning it for energy production may cause a series of environmental pollution due to the amount of sulphur in it. When the consumed sulphur in coal is in the form of sulphur dioxide at the end of combustion, it reacts with water at high altitudes. Moreover, it goes down to the earth in the form of sulphuric acid, as well as damaging plants. The corrosive harmful impact on industrial plants is the removal of the sulphur in the coal when it is environmental. Microorganisms that use sulphur as food to eliminate the anxiety of clean production brought about by the chemical method. This review determined the study contains information about which microorganism is effective in removing sulphur in coal and also the types of organisms that should be according to the organic sulphur type in coal.
1. Introduction
It is thought that coal will remain one of the important actors in meeting the increasing demand for global energy demand. Various technological solutions are available for the environmentally friendly use of this fossil fuel resource. For example, dehydration processes such as pre-treatment, preparation, catalytic treatment, gasification, refining of liquefied products are widely studied. While evaluating the environmental performance of coal-based thermal power plants; parameters such as the type of coal, high humidity, low calorific value, oxygen, high humidity, ash content, oxygen content are evaluated. Another important parameter of these is sulphur. The basis for the use of microorganisms in desulphurisation from coal derives from the role played by bacteria in the sulphur cycle. Oxidation of sulphur, which is generally found in the coal structure, is in question (Gredilla et al. Citation2019; Gasparotto et al. Citation2019; Ferrari et al. Citation2019; Duarte et al. Citation2019; Akinyemi et al. Citation2019; Silva et al. Citation2021; Silva, Crissien, Sampaio, et al. Citation2020; Silva, Crissien, Tutikian, et al. Citation2020; Saikia et al. Citation2020; Oliveira, Saikia, et al. Citation2019).
Technologies for desulphurisation and others cleaning process from coal have several advantages and disadvantages (Saikia et al. Citation2016; Wilcox et al. Citation2015; Civeira, Ramos, et al., Citation2016; León-Mejía et al. Citation2016; Sehn et al. Citation2016; Dalmora et al. Citation2016; Rodriguez-Iruretagoiena et al. Citation2016). Its biggest advantage is that it takes place under low pressure and temperature conditions. Compared to the chemical desulphurisation method, there are more environmentally friendly process steps in the biological desulphurisation method. The disadvantages of the biological desulphurisation method are that conditions such as storage and clean production are provided under difficult conditions. The excess of these intermediate processes not only increases the cost of the process but also lowers the calorific value of the fuel. Among the advantages of the hydro sulphurisation method, the process is simple and the necessary H2 is easily provided. The biggest disadvantage is that it requires high temperature and pressure. The cost of the catalyst used is also high. In the desulphurisation method with adsorption, as in the biological method, low reaction temperature and pressure are required. The process is simple. The disadvantage of this process is that the process is limited according to the activation of the selected sorbent. The amount of sorbent required for the process is high. In the oxidative desulphurisation method, there is no need to use any catalyst. The required raw material cost is low. Its methodology can be easily studied for low-rate results. Its reaction rate is high. H2 is not needed. However, in large-scale systems that require the use of H2O2, the cost is high. There is not enough work for bitumen. Intense desulphurisation requires a catalyst. When using H2SO4, undesirable products may be obtained as a result of the process. Extractive desulphurisation with ionic liquids requires low pressure and temperature. Another advantage is that the refining process is simple. The raw material does not have any effect. The solvents used can be easily recycled. However, the efficiency of the process is limited depending on the solvent type. High efficiency is achieved by the oxidation of sulphur (Almashjary et al. Citation2018). Desulphurisation methods from coal have their advantages and disadvantages. Especially desulphurisation by chemical method takes a long time. Minerals used for desulphurisation contribute to ash formation in coal. This situation affects the calorific value negatively. Ultrasonic methods, on the other hand, are an expensive process due to the high amount of energy consumed. The flotation process, which is used for sulphur removal, is preferred as a more suitable method in the stages of reducing ash and demineralising coal. The petroleum agglomeration process has advantages over chemical methods in terms of reducing coal ash, removing sulphur, and increasing calorific value. In this process, the mineral matter is separated from the coal by applying foamy flotation. While the hydrophobic feature of the oil used in the process increases in the coal, the decomposition of mineral substances becomes easier (Ken and Nandi Citation2019). The need for fine-grained coal in coal separation brings the cost to the fore in removing sulphur. In particular, in the removal of organic sulphur, which will be explained in the following sections, inorganic minerals must be separated after the drying and grinding of the coal first. Along with these costly processes, sulphur can be removed by the microwave process. All these stages bring an additional cost, as mentioned above, both in terms of its type and the amount of energy it consumes (Yu et al. Citation2019). These disadvantages can be reduced by the rapid penetration of bacteria from the coal surface in the aqueous environment. The most important financial issue in the biodesulphurisation method of coal is the operation of enzymatic reactions at certain temperatures. Increasing the reaction efficiency depends on the determination of an accurate microbial culture based on the sulphur type. Reducing the reaction time depends on the application of a microbial culture enzyme with a single enzyme in mind (Saikia et al. Citation2016; Wilcox et al. Citation2015; Civeira, Ramos, et al. Citation2016; León-Mejía et al. Citation2016; Sehn et al. Citation2016; Dalmora et al. Citation2016; Rodriguez-Iruretagoiena et al. Citation2016; Civeira, Oliveira, et al. Citation2016).
In Malani et al.’s compilation study, the main species used in microbial desulphurisation were bacillius, brevibacillus, arthobactor, agrobacterium, desulphobacterium, corynebacterium, gordonia, microbacterium, lysinibacillus, mycobacterium, pantoea, paenibacillus, pseudomonas, rhodococcus, shewanella, serraastia, sphingomona (Malani et al. Citation2021).
Ye and his colleagues studied desulphurisation with the ‘indigenous and exotic microorganism’ of the ‘raw fat coal’ and the ‘bio-leached coal’ in the Inner Mongolia China region and, unlike other studies, they found that the two organisms they tried had positive effects on the kaolinite in the coal (Ye et al. Citation2018). They have summarised these studies in .
Figure 1. Summary of the study (Ye et al. Citation2018).
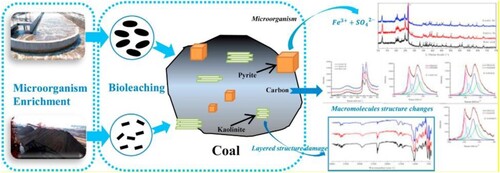
As the methods to be applied while removing sulphur from coal by biological method, Singh et al. said that there are physical technologies such as microwave radiation, microwave oxidation, ultrasonic-microwave, ultrasonication, flotation, separation by weight, pyrolysis, and separation by weight in iron powder separation. However, such as nitric acid treatment, KOH, and HCl solution curing, HNO3 leaching, acid remediation flotation process, cupric chlorite remediation, NaOH/HCI treatment, alkali treated-solvent extraction, hydrogen peroxide (H2O2) treatment, sodium hypochlorite, and sodium hydroxide cure have reported chemical methods (Singh et al. Citation2021).
Alkaline reagents used in coal desulphurisation methods; Xu et al. reported that there are chemicals such as HCl, H2SO4, HNO3, H2O2, nitric-acid solution, acetic acid, NaCIO4, NaOH, Hac-H2O2 (Xu et al. Citation2021).
Saha et al. conducted a compilation study on the effectiveness of desulphurisation depending on the surface chemistry of adsorbents such as metal oxide, zeolite, mesopore materials, metal-organic materials used in the adsorptive desulphurisation method. In this study, they concluded that the acid-base, π-complexation, metal-sulphur, and Van der Waals forces on the adsorbent surface of sulphur molecules are effective (Saha et al. Citation2021).
Although coal is not a renewable energy source, it is still the primary energy source in many countries in terms of meeting energy needs. On the other hand, fossil fuels such as coal and oil should not be considered only as energy raw materials. It is also the raw material of various industrial institutions such as cosmetics, paint, pharmacy, plastic, aluminium and iron, and steel. Among the non-renewable energy sources, the percentage of raw material coverage of coal due to energy requirement is 75. The most significant increase in energy resources in the European Union energy demand in 2020 comes from coal. The based estimates are on the information that lignite-based coal will run out of 160 years of natural gas and 60 years of oil reserves compared to today’s consumption. Coal is the one with the highest energy security compared to other energy sources. Therefore, especially in countries where natural gas and oil have low reserves, coal will always remain current as an energy source. Globally, considering the known coal quality is that they have 1–3% sulphur content. However, sulphur-based environmental regulations require the restriction of chemical reactions caused by sulphur and the particles and gases released after combustion. Since the response of oxygen and sulphur in the structure of coal, produced products in the form of SO2 and SO3 take part in the formation of H2SO4 (H2SO4 is the primary source of acid rain). Acid rains are significant for both living things and the environment. These losses also have financial consequences. Especially in the industry, it increases both maintenance and operating costs due to its corrosive effects on metal in thermal power plants and steel production. They form leaf spots on plants. Plants that cannot perform photosynthesis die and lead to negative consequences, such as stopping the production of natural and agricultural products. In humans, SO2 invites skin and respiratory diseases. In terms of human and environmental health, preventing adverse effects of SO2 should be in due to the industrial cost increases in the country’s economy (Abdollahy et al. Citation2006; Asmatulu et al. Citation1998; Belyakov Citation2019; Berkowitz Citation1979; Olszewska Citation2011, Citation2012). Many countries that are aware of the issue rather than technological solutions have started work on reducing sulphur-based emissions with various sanctions by law (Klein Citation1998). In our country, it is also essential to use natural resources most effectively without polluting the environment with an emphasis on domestic and national policies. The main issues that make up a country’s focused energy policy are reliability, efficiency, sustainability, and minimising the adverse effects on the environment. These titles require that the method to be applied to the domestic energy source should be both economical and environmentally friendly. The implementation of these titles should pave the way for the development and social development of the country’s economy based on energy planning. The national energy policy must come into effect with the topics mentioned above once the implementation of these titles is a measure of the development of that country. These titles seem only possible with the adoption of a continuous energy policy. These energy policies are realised only with the application of clean energy and high efficiency, with the production of energy from national leading energy sources. In other words, sustainability is an application that covers the evaluation of fossil-based energy production with environmentally friendly technologies – for example, considering the energy released as waste in one energy cycle as an input to another period and supporting these applications with economic growth (Klein Citation1998; Fairbairn and Bushell Citation1992; Constantí et al. Citation1996; Haddadin et al. Citation1995; Kargi Citation1982; Chakrabarti Citation1978; Huffman et al. Citation1993; Chapter Citation1989 – Physical Desulphurisation of Coal Citation1989; Yang and Chen Citation1993).
A tried environmental health is to be controlled by international agreements. However, chemical processes commonly applied to sulphur removal show some disruptions in terms of both cost and target orientation. The first one is whether the method employed is environmentally friendly. It becomes attractive in biological processes that include the ways of using sulphur of bacteria or other species (fungi, etc.) that use sulphur as a considered food source, which is as process waste, as a continuation of the sustainability mentioned above concept. Important issues such as shortening time in biological methods, increasing efficiency, and reducing brought operating costs may also be up. Bioremediation; is a natural treatment method. In this method, the disposal of environmental pollutants and toxic chemicals is involved. Also, it is a used living organism. A done much work is to perform a low-cost and high-efficiency process (Fairbairn and Bushell Citation1992; Constantí et al. Citation1996; Haddadin et al. Citation1995; Kargi Citation1982; Chakrabarti Citation1978; Huffman et al. Citation1993; Chapter Citation1989 – Physical Desulphurisation of Coal Citation1989; Yang and Chen Citation1993; Ernst WHO Citation1998; Behrouzifar et al. Citation2016; Çelik et al. Citation2019; Wierzchowski et al. Citation2020; Soltanali et al. Citation2008; Bubenick et al. Citation1983).
Industrial microbiology science has made significant progress in other technologies, such as high technology. At this stage, not only living things but also enzymes they produce are used. These technologies cause essential changes not only in technological terms but also in socio-economic conditions (Fairbairn and Bushell Citation1992; Constantí et al. Citation1996; Haddadin et al. Citation1995; Kargi Citation1982; Chakrabarti Citation1978; Huffman et al. Citation1993; Chapter Citation1989 – Physical Desulphurisation of Coal Citation1989; Yang and Chen Citation1993; Singh et al. Citation2012).
Widely, used fungi (especially white-rot fungi) are in bio-healing. In particular, they have enzymes effective in breaking down the organic structure. Therefore, they have an essential role in the disintegration of fragmentation-resistant compounds (Kargi Citation1982; Gu et al. Citation2017).
The thing is that this book chapter study will prepare an academic ground for sulphur removal methods with a realised biological process that follows the titles of ‘Clean energy clean environment clean technology’ envisaged as one of the harmonisation technologies of the European Union in our country. Besides, the idea is that these considered methods, which are in developed countries in recent years, may be useful in advancing research and development studies. Also, we firmly believe that our research will contribute to biotechnology science in environmental and chemical biotechnology (Klein Citation1998; Singh et al. Citation2012).
2. Methodology
In this review, the sulphur emissions of fossil fuel power plants, which lead the global world sanctions due to environmental concerns, should be taken under certain limits. For this reason, the type of coal that various microorganisms that are consumers of given natural sulphur are useful is in the light of different current data from the literature in the following sections.
3. The structure of coal
Coal is an organic rock formed by the swamp conditions created as a result of the fossilisation of plants and the natural substances accumulated there. Coal, which has a complex polymeric structure, also has a more porous macromolecular network structure than other fossil fuels such as oil and natural gas. An introduced organic material is in these pores. The formed heteroatoms and aliphatic chains are with naphthenic and aromatic rings. In addition to the seen covalent bonds is that hydroxyl-bonded polar groups are effectively involved in the primary structure together with oxygen (Kabe et al. Citation2004; Yao and Liu Citation2019; Chou Citation2012; Bressler et al. Citation1997).
3.1. The coal composition
The information obtained from the petrographic perspective regarding the structural analysis of coal is given below. For petrographic analysis, the sample (coal) is immersed in oil and/or classified as huminite, vitrinite, liptinite, and inertinite according to ‘maceral’ molecules expressing physical optical property.
3.2. Huminite/vitrinite maceral group
It first gels with bacterial action and then comes from lignocellulosic tissues after charring with its geological evolution. Huminite macerals are identified in low-grade coals and these macerals are considered to be the precursor of vitrinite macerals in higher-grade coals. The huminite/vitrinite group, which is mainly aromatic and hydroaromatic in nature, is the most abundant maceral group. These macerals are mostly found in coals from the northern hemisphere. The vitrinite feature of coal is an important maceral that determines the desulphurisation feature from coal (Yao and Liu Citation2019; Ahamed et al. Citation2019; Dai, Bechtel, et al. Citation2020; Dai, Hower, et al. Citation2020; Li et al. Citation2019; Moldoveanu Citation2021; Pashin et al. Citation2020; Sen and Dash Citation2020; Tangstad et al. Citation2019; Valentim Citation2020).
3.3. Liptinite maceral group
Liptinite maceral group of organic substances that are relatively poor in oxygen, such as spores, algae, cuticles, pollens, and secretions. In this group, aliphatic structures and hydrogen content are high. During the charring of coal, most liptinite is lost due to adventitious thermal transformation or develops optical properties similar to vitrinite (in the bituminous stage). During the carbonisation process, the liptinite group gives off large amounts of tar and gas due to its high hydrogen content. In this group of macerals, there are macerals with high calorific values (Yao and Liu Citation2019; Ahamed et al. Citation2019; Dai, Bechtel, et al. Citation2020; Dai, Hower, et al. Citation2020; Li et al. Citation2019; Moldoveanu Citation2021; Pashin et al. Citation2020; Sen and Dash Citation2020; Tangstad et al. Citation2019; Valentim Citation2020).
3.4. Inertinite maceral group
Inertinite maceral group is formed as a result of chemical reaction with oxidation of cellulosic structure in coal. Although it is an aromatic structure with low volatile matter and hydrogen in structure, it has a high carbon content. During combustion, active molecules with different characters are produced depending on the coal grade and the type of inertinite (Yao and Liu Citation2019; Ahamed et al. Citation2019; Dai, Bechtel, et al. Citation2020; Dai, Hower, et al. Citation2020; Li et al. Citation2019; Moldoveanu Citation2021; Pashin et al. Citation2020; Sen and Dash Citation2020; Tangstad et al. Citation2019; Valentim Citation2020).
The macerals listed above can also be seen in . Macerals cannot be isolated. Microlithotypes can hold different macerals together and they can also contain inorganic components. The diversity of the components of the microlithotypes is an important parameter affecting the efficiency of desulphurisation. The organic structure of coal consists of oxygen, hydrogen, carbon, sulphur, and nitrogen. The amounts of carbon, hydrogen, and oxygen are roughly 97–99% by mass of all coal except the inorganic part. With the increase of the coal grade from 65% to 95%, the coal grading up to anthracite occurs. However, as the carbon ratio increases with the degree of carbonisation, the hydrogen content decreases. For example, while this value is 30% in lignite, it is less than 1% in anthracite level. It is known that nitrogen content is between 0.3 and 15% and the nitrogen content of organic sulphur is between 0.3 and 2% (Yao and Liu Citation2019; Ahamed et al. Citation2019; Dai, Bechtel, et al. Citation2020; Dai, Hower, et al. Citation2020; Li et al. Citation2019; Moldoveanu Citation2021; Pashin et al. Citation2020; Sen and Dash Citation2020; Tangstad et al. Citation2019; Valentim Citation2020).
4. Inorganic composition
Mineral substances are an important component of coal. However, the percentages found are variable. The main of these substances are dissolved salts in the coal pores. It is also found in inorganic elements found in the macros of coal. Inorganic substances are of two types, crystalline, and non-crystalline. The mineral substance content of the coals with a low carbonisation degree is high. Coals contain rock fragments and minerals mixed with coal. This mineral is partially removed by pre-cleaning in the mine. In the processes of desulphurisation from coal, the types of non-mineral inorganics in the macerals can be examined together with the minerals mixed with the coal. Major minerals are clay minerals, quartz, feldspars, titanium minerals, chlorite, sulphur, chloride minerals, carbonate, barytes, calcium sulphates, iron, limonite, and mica. Sulphur is the most important inorganic. Other minerals occur in all coals below 1% (Artanto et al. Citation2000; Basu Citation2018; Benson et al. Citation2005; Guo et al. Citation2004; Laumb et al. Citation2008; Naudé et al. Citation2013; Wüst et al. Citation2002; Zygarlicke et al. Citation1992).
5. Sulphur types in coal
Sulphur is found in two primary forms in coal, organic and inorganic. Sulphur in a natural way, which is in the form of benzothiophene, or especially dibenzothiophene, is inorganically present in FeS2 form. Also available in sulphate and sulphite sulphur. Sulphide minerals are compounds such as sphalerite (ZnS), pyrite (FeS2), arsenopyrite (FeAsS), and galena (PbS). The found sulphate minerals are in the form of gypsum (CaSO4.2H2O), barite (BaSO4), many iron sulphate compounds, and anhydride calcium sulphate (CaSO4). The most common dominant inorganic sulphur is pyrite in coal. Pyrite pieces spread randomly in crystal form in all coal, but are not bound in the coal structure. However, organic sulphur depends on the complex structure of coal. Unlike inorganic sulphur and pyrite, organic sulphur is more challenging to remove from the structure. The studied organic sulphur of coal is in four classes aromatic, aliphatic, and heterocyclic compounds (Behrouzifar et al. Citation2016; Çelik et al. Citation2019; Wierzchowski et al. Citation2020; Soltanali et al. Citation2008; Chou Citation2012; Gorbaty et al. Citation1991; Parekh Citation2003; Nam and Park Citation2004).
Aromatic or aliphatic thiols (thiophene, mercaptans)
Mixed sulphur, aromatic or aliphatic sulphur (thioethers)
Mixed disulphides, aliphatic and aromatic sulphur (thioethers)
Thiophene type and heterocyclic compounds (dibenzothiophene) (Chou Citation2012; Gorbaty et al. Citation1991).
6. Sulphur removal technologies
It is said that SO2, which is an important component of air pollution today, originates from the sulphur in the structure of coal in the combustion of coal. Sulphur removal techniques can be chemical, biological, or physical (Behrouzifar et al. Citation2016; Çelik et al. Citation2019; Wierzchowski et al. Citation2020; Soltanali et al. Citation2008; Singh et al. Citation2012; Parekh Citation2003; Nam and Park Citation2004; Clark and Olson Citation1993; Weills Citation1976; Chadwick and Lindman Citation1982; Liu et al. Citation2016, Citation2017; Demirbas and Balat Citation2004).
7. Chemical methods in sulphur removal
These methods are air oxidation, carbonisation in different environments, Meyers process, moisture oxidation, sodium hydroxide, chlorination, ethanol solutions, copper chloride extractions. However, in the pre-treatment of stubborn sulphidic minerals such as arseno-pyritic and pyrite, with bases, there are methods such as leaching, which are soluble by filtration. Another technique is chlorination with gamma rays. The removed sulphur is with water. In this method called hydrodesulphurisation, sulphur is transformed by a converted process that takes place at high temperature (about 200–415oC) and pressure (about 10–17 atm pressure), where it is to hydrogen sulphide (Chou Citation2012; Nam and Park Citation2004; Longwell et al. Citation1995; Tang et al. Citation2020; Cliffe and Syed Citation1993; Holst et al. Citation1998; Kuenen and Robertson Citation1992).
High reaction rates occur in both the above-mentioned and desulphurisation method (10% organic sulphur removal, 90% inorganic sulphur removal). However, these processes are costly, and there might be dangerous by-products. Also, the process affects the structural integrity of coal. Therefore, these methods of removing organic sulphur compounds are not particularly useful in eliminating heterocyclic polyaromatic sulphur compounds (Chou Citation2012; Bressler et al. Citation1997; Longwell et al. Citation1995; Tang et al. Citation2020; Cliffe and Syed Citation1993).
The conventional hydrodesulphurisation approach requires temperatures and high pressures. Alternative Petroleum Technologies Corporation has established an oxidative desulphurisation pilot plant, call as SuffexTM, which can mitigate sulphur from liquid hydrocarbons at near-ambient temperatures and pressures. Company headquarters declared that the need for high temperatures and pressures eliminating cuts, both operating and capital costs for sulphur removal by at least 50% (Technologies AP Citationn.d.).
Research Triangle Park (RTI) has underdeveloped the Warm-gas Desulphurisation Process (WDP), and is now commercially available through RTI’s partner Casale S.A., for over ten years. In raw syngas with sulphur concentrations as high as 14000 parts per million (ppm), the WDP technology consistently reduced entering total sulphur by 99.8–99.9% (RTI. stated). Furthermore, according to RTI, integration of WDP with CO2− capture systems can reduce sulphur to parts per billion (ppb) levels, which renders the syngas suitable for fuel manufacturing processes, fertiliser, and chemicals (Clariant to Supply Unique Sorbent to RTI International for Its Warm Gas Desulfurization Technology Citation2018).
8. Biological methods in sulphur removal
The evaluated biological methods are in a different sense than the below-described physical techniques present. According to the chemical process, less harmful formed products are in the biological method. It takes place under more moderate conditions than the chemical method, and the main structure of coal is not affected. In this sense, as a result of microbial sulphur removal, which we can call desulphurisation, sulphur in coal turns into a compound like a sulphate as a result of oxidation. This method is a biochemical reaction catalysed by aerobic microorganisms () (Speight and El-Gendy Citation2018). In general, naturally, coal is healed by removing both the carbon matrix and pyritic sulphur. These methods include fewer energy costs and simple process processes than the chemical method (Soleimani et al. Citation2007; Benito et al. Citation1995; Martínez et al. Citation1995; Kargi Citation1986; Kilbane Citation1989; Aliebrahimi et al. Citation2015; Bayram et al. Citation2002; Gürü et al. Citation2006; Nazari et al. Citation2017; Raheb et al. Citation2011, Citation2012; Raheb and Hajipour Citation2011a, Citation2011b, Citation2012; Xu et al. Citation2020).
9. Physical methods in sulphur removal
In a physical process, the coal is ground and then washed. The removed known inorganic sulphur is that total emission decreased by 20–30% as a result of coal burning. Physical processing on organic sulphur has no positive effect on (Brothers and Starzomski Citation1976; Yaman and Küçükbayrak Citation1997; Uzun and Özdoğan Citation2006; Kumar and Kumar Citation2018).
Using Thiopaq O&G technology, Paques Holding B.V. and Shell Global Solutions cooperated to form biodesulphurisation technology for gas and oil. The process started in 2011 and operated from the Watercampus in Leeuwarden, Amsterdam (Kazemi et al. Citation2014; Mokhatab and Poe Citation2012). The technology installed works as follows; Ph is reacted irreversibly with H2S from acid gas or flue gas to form hydrosulphide ions (HS−) between an alkaline solution between 8 and 9 and an absorbent. The solution containing HS-ions separated from the poured absorber is from a low-pressure flash tank for the synthesis of dissolved gases. Hydrosulphide (HS−) is then oxidised to elemental sulphur under controlled conditions in the presence of microorganisms after flowing into a bioreactor operating at atmospheric pressure. These oxygen-consuming (aerobic) organisms use the energy released from sulphur oxidation for metabolic processes. The produced elemental sulphur is with a hydrophilic structure, is separated from the aqueous waste in a three-phase separator, and then the renewed solution is fed back to absorbance to repeat the previous reaction steps (Kazemi et al. Citation2014; Mokhatab and Poe Citation2012; Baatar et al. Citation2017; Dehkordi et al. Citation2013; Gürü Citation2007; Hussein and Fadhil Citation2021).
10. Biological removal methods
10.1. Biological removal mechanism of inorganic sulphur
The use of inorganic sulphur with different microorganisms for the last 30 years has been tried and proven experimentally. In this method, with the presence of mesophilic and thermophilic organisms, the environment of separation from minerals is created in coal inorganic salt solutions (Deriase et al. Citation2012; Saed et al. Citation2014; Singh et al. Citation2018). There are two methods in the oxidation process of pyrite in coal with various microorganisms. These are direct and indirect oxidation. It was shown by Silverman in 1967 that there is a physical interaction between the sulphuric mineral in the structure of coal in the bacterial culture, and the formation of metal sulphate occurs entirely by biological mechanisms (Tripathi et al. Citation2019; Liu et al. Citation2020).
Indirect oxidation, in the first stage, pyrite is oxidised directly without any intermediate mechanisms. This oxidation is about direct physical contact between bacteria and pyrite. As a result of the bacteria cells injecting enzymes into the surface of the crystalline sulphide molecule, thin-film corrosion occurs between the bacterial outer membrane and the sulphide. Here the process takes place in a second phase (Tripathi et al. Citation2019; Liu et al. Citation2020).
In 2019, the methane production potential of various bacterial species was investigated on the coal bed by Beckmann et al. In the study, the results were obtained in three stages bacterial culture, acetate media, calcium peroxide media, and only coal surface, according to the bacterial species () (Tripathi et al. Citation2019; Liu et al. Citation2020; Beckmann et al. Citation2019).
The fact that microorganisms are too large to enter the coal pores formally is one of the most important factors preventing the oxidation of pyrite in the coal structure. Therefore, oxidation of pyrite with an enzyme secreted from a microorganism, which we can call indirectly, is active () (Jiang et al. Citation2007). While oxidised pyrite is with enzymes, Fe +3 ions act as an oxidiser. In this process, enzymes reduce Fe + 2 to Fe + 3 in the oxidation of pyrite. Oxidises pyrite in Fe +3 () (Jiang et al. Citation2007). Iron deposits such as jarosite inhibit oxidation. Thermophilic bacteria oxidise pyrite more quickly. However, after this process requires high temperatures, the probability of formation of deposited jarosite from oil is on the coal surface as a residue in the specific washing stage, increases. However, high temperature also reduces the dissolution amount of ferric ions. Therefore, correct operating conditions are essential for the cultivation of bacterial cultures for indirect oxidation of pyrite (Ye et al. Citation2018; Tripathi et al. Citation2019; Liu et al. Citation2020; McIlwain and Dugan Citation1990).
Figure 6. Different resolution images (SEM (a, b, and c), Fluorescent staining image (d)) of pyrite surface oxidised due to Acidithiobacillus ferrooxidans effect for 39 days.
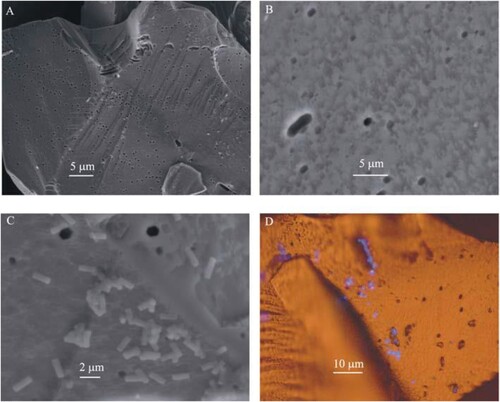
11. Biological removal mechanism of organic sulphur
The removal of organic sulphur is not as easy as inorganic sulphur. The most challenging type of sulphur to remove fossil fuels is dibenzothiophene (DBT) () (Speight and El-Gendy Citation2018). Pseudomonas and Brevibacterium species use DBT as a source of sulphur, energy, and carbon. DBT is an integral part of thiophenes sulphur in most fuels. Rhodococcus erythropolis ATCC 53968 is one of the standard methods used in DBT removal in isolation and characterisation studies. A technique called 4S represents DBT, DBT-sulphoxide, DBT-sulphone, DBT-sulphinate, hydroxyphenyl (HBP), and sulphide sequenced metabolism in this method developed as a sulphur-specific metabolic pathway. The most significant advantage of the 4S route is that bacteria selectively oxidise sulphur without touching the C–C bonds. In the Arthrobakter type, dibenzothiophene removes sulphur on sulphone (Liu et al. Citation2020; Benedik et al. Citation1998; Wackett Citation2000; Chakrabarty Citation1985).
12. Types of microorganisms used in sulphur removal from coal
The first species of microorganisms used in sulphur removal from coal are sulpholobus acidocaldarius, thiobacilus thioxidans, thiobasilus ferrooxidans, and acidian brierinine species. Thiobacillus ferrooxinases are the most common among them. This type of chemotroph is anaerobic respiration bacteria. They play a role in the oxidation of the reduced iron and other sulphur components of pyrite (Kilbane Citation1989, Citation1990; Tang et al. Citation2018; Gogoi and Bezbaruah Citation2002; Kalia et al. Citation2003).
While bacteria are not active on sulphur when alone, they can break down pyrite in their interactions with more than one species. For example, while Leptospirilum bacteria are not active on pyrite alone, they can break down pyrite (symbiosis) with the combination of Thiobasilus organoparus, T. thioxidasn, and T. acidophilus (Kilbane Citation1989, Citation1990; Tang et al. Citation2018; Gogoi and Bezbaruah Citation2002).
While mesoacidophilic bacteria are useful on inorganic sulphur, it is not very active on organic sulphur. Sulpholobus and Pseudomonas species are beneficial in organic sulphur removal. The bacterial species that are effective for both types of sulphur are Rhodokokus species (R. eritropolis, R. eritropolis ATCC 53968, R. eritropolis H-2, and R. sp ECRD-1). Moreover, the most active species among them is R. eritropolis ATCC 53968. Gordonia alkanivornas bacterium is active in both types of sulphur. The tried is in species with more effective enzymes than thiobacillus species. Metallosifera sedula bacterium is a thermosidofil species and is especially useful in metal sulphide removal (Kilbane Citation1989, Citation1990; Tang et al. Citation2018; Gogoi and Bezbaruah Citation2002).
Pretreatment of sulphidic minerals (arsenopyrite and pyritic species) in coal has a positive effect on the biological sulphur removal method. Sodium carbonate and ammonium hydroxide are treated with coal before natural treatment, allowing easier separation of the sulphur bond means a yield of about 11% over usual biological treatment (Kilbane Citation1989, Citation1990; Tang et al. Citation2018; Gogoi and Bezbaruah Citation2002).
Sulphur removal efficiency varies according to coal types. The most common type of coal that needs sulphur removal is lignite. Lignites are preferred because the degree of carbonisation is low, and the morphology of sulphur is more prone to removal. So pyrite bonds can be broken more easily. For example, thiobacillus ferrooxidans have higher sulphur removal in lignite than other coal types (Kilbane Citation1989, Citation1990; Tang et al. Citation2018; Gogoi and Bezbaruah Citation2002).
Although it is not more common in fungi other than used bacteria, widely, is to remove sulphur from coal. Fungi have the property of breaking down hydrocarbons using Cytochrome P-450 status and anthropogenic chemicals with extracellular enzymes. The powerful extracellular enzymes of fungi have a useful shredding property on high molecular weight organic molecules and turn them into valuable products (Kilbane Citation1989, Citation1990; Tang et al. Citation2018; Gogoi and Bezbaruah Citation2002).
Microorganisms have essential roles in performing processes such as selectively breaking C–S bonds, destroying the entire molecule, and removing sulphur atoms from organic sulphur compounds. For example, studies in acidophilic bacteria that oxidise sulphur such as acidithiobacillus have reported that such an encountered problem is not when working with fungi such as aspergillus, while precipitates such as jarosite, which are ferric iron and sulphates that inhibit the reaction (Kilbane Citation1989, Citation1990; Chakrabarty Citation1985; Tang et al. Citation2018; Gogoi and Bezbaruah Citation2002).
13. Coal bio-solubilisation
Chemical and clean fuel, which are useful in liquefying coal by the microbial process, is produced. A considered this process is as economically attractive and environmentally safe. The process consists of transforming complex organic compounds into products with lower molecular weight. A proven product was that in the 1980s, coal could be broken down by microbial methods and even used in this process in fungus. A used Trichoderma atroviride and Fusarium occiporum species are useful in the bio-solubilisation mechanism of coal in filamentous fungi. These fungi effectively cleaved coal bonds with extracellular oxidase and peroxidase enzymes. The surface culture studies of fungus have proven to play an active role in digesting coal macromolecules in metabolic events. It can depolymerise, polymerise and decolourise macromolecules from low-quality coal in oxidase enzyme secreted by white-rot fungi (Behrouzifar et al. Citation2016; Çelik et al. Citation2019; Wierzchowski et al. Citation2020; Soltanali et al. Citation2008; Benedik et al. Citation1998; Wackett Citation2000; Matsumura Citation2005; Klasson et al. Citation1993; Crawford and Gupta Citation1991; Olson and Brinckman Citation1986; Andrews and Noah Citation1997; Faison Citation1991).
14. The potential of coal bio-desulphurisation
A declared fact is that the excessive polymeric structure in the coal compared to the oil makes the bacteria more difficult in the sulphur removal process () (Matsumura Citation2005). Here, the physical size of the bacterium plays an important role. For this reason, associated coal is with many factors such as particle size, pH, nutrient composition, temperature, reactor design, and ventilation. The mud leaching system and the leaching system are examples.
Table 1. Structure of the main chain polymer and specific enzyme suitable for the structure.
Thermophilic bacteria are more effective than mesophilic bacteria in non-biological oxidation of pyrite (Kilbane Citation1989, Citation2006; Benedik et al. Citation1998; Chakrabarty Citation1985; Andrews and Noah Citation1997; Faison Citation1991; Natarajan Citation2018; Biology and Fuel Science Citation1995; Yang and Ge Citation2016).
The selection and design of the appropriate based reactor are on continuous systems due to the high volume of biolysing and bio-oxidation process. It is essential to ensure the autocatalytic growth of the microorganism in the bioreactor design (Selvaraj et al. Citation1997). Proposed Pachuca tank reactors are for coal desulphurisation. These reactors are cylindrical, conical bottom, and three phases. Suitable conditions in the reactor are possible by providing subjects such as pH value, mass transfer, and temperature conditions for microorganisms that oxidise pyrite. Still, limited industrial-scale applications are due to the prevalence of chemical methods and the economic and technological preferability of the bio-method. A discussed sustainability of the procedure is since the results obtained for a fixed bacterial culture are variable. However, the suitability of the reactor design is essential for the sustainability of the process. In the reactor design, different parameters such as the first substrate type, concentration, growth temperature, mixing speed, initial pH value, and percentage of inoculum are essential. Among the batch and continuous bioreactors, the most efficient type is the feed-through continuous bioreactor (Kilbane Citation1989, Citation2006; Benedik et al. Citation1998; Wackett Citation2000; Chakrabarty Citation1985; Kalia et al. Citation2003; Andrews and Noah Citation1997; Natarajan Citation2018; Biology and Fuel Science Citation1995; Yang and Ge Citation2016).
15. Types of microorganisms used for coal bio-desulphurisation
15.1. White rot fungus
White rot fungus; fungus produces enzymes that break down almost all of the large molecular-weight structures, especially lignin of vegetable origin. A divided Fungi are into two according to their wood shredding properties. Brown fungus do not touch lignin while separating hemicellulose and cellulose. Laetiporus portentosus, Serpula lacrymans, and Fomitopsis lilacino-gilva are examples of these species. White fungus, on the other hand, can break down polysaccharides such as hemicellulose and cellulose, as well as ligninide. Trametes Versicolor, Chrysosporium lignorium, Phanerochaete chrysosporium, Pleurotus ostreatus, Armillaria lutebubalina, Stereum hirsutum, Hebeloma crustuliniform, Daldinia concentrica, and Schizophyllum commune are examples of these species. The most common among these are P. chrysosporium and T. Versicolor. Also, the used white rotting fungus is in the paper industry for wastewater discharges containing trinitrotoluene (TNT) and for the decomposition of chlorinated lignin waste and various phenol compounds in pesticide production. Among the extracellular enzymes of these species, lignin peroxidase, manganese peroxidase, and laccase enzymes can break down secondary metabolites, polychlorinated biphenyl and dioxins, and many chlorinated phenolic compounds. Besides, these used enzymes are in the colour removal of textile dyes (Constantí et al. Citation1996; Olson and Brinckman Citation1986; Shirkavand et al. Citation2016; Ali and Zhao Citation2020; Asghari et al. Citation2013; Arnold et al. Citation2017; Alcalde Citation2015).
In general, we can divide white rotten fungi into three according to the enzymes they produce. The first one has the enzyme that breaks down wood (cellulose, hemicellulose, and lignin). The second group includes glyoxal oxidase and superoxide dismutase enzymes. This group alone cannot act on the woody substrate without the first group. The third type comprises aryl alcohol oxidase, glucose 1-oxidase, pyranose 2-oxidase, and cellobiose dehydrogenase enzymes. These break down the lignin (Klein Citation1998; Constantí et al. Citation1996; Olson and Brinckman Citation1986; Shirkavand et al. Citation2016; Ali and Zhao Citation2020; Asghari et al. Citation2013; Alcalde Citation2015).
15.2. Phanerochaete chrysoporium
Phanerochaete chrysoporium; belongs to the family of Cırticiaceae. They have fructification organs in a flat structure that have a shell structure with a thickness of less than 0.23 mm, consisting of basidiocarpals in the range of white to salmon. The shell has no distinctive feature on the living species. P. chrysosporium shows both heterotality bipolar and homotality sexuality. Observed thin-walled intercalar chlamydospores and pure split fibres with a diameter of 3–9 µm are at the age of 50–60 µm (Asghari et al. Citation2013; Hofrichter Citation2002; Harayama Citation1997; Janda Citation2015).
P. chrysosporium is a type of fungus that breaks both soft and hardwood stumps and branches, but they live in forests with temperate climates all over North America, Europe, and Iran. This type, which is responsible for the successful disintegration of lignin and its components, has attracted considerable interest in the paper industry, especially as an environmentally friendly and alternative method for the chemical bleaching of pulp. Due to the low selectivity of the peroxidase-based ligninolytic of P. chrysosporium, it has made it possible to use the by-products of many waste-resistant organic wastes in biological treatment as a result of biodegradation (Asghari et al. Citation2013; Hofrichter Citation2002; Harayama Citation1997; Janda Citation2015).
Many extracellular enzymes of P. chrysosporium are manganese peroxidase (MnP), lignin peroxidase (MnP), cellobiohydrolase, B-glucosidase, endoglucanase, glyoxal oxidase, xylosidase, xylanase, α-galactosidase, superoxide dismutase, pyranose 2-oxidase, and mannose-6-phosphatase (Asghari et al. Citation2013; Hofrichter Citation2002; Harayama Citation1997; Janda Citation2015).
Among these, used MnP and LiP enzymes are for biological removal of much organic waste by-products including polyethylene, textile dyes, pesticides, polyaromatic hydrocarbons (PAH), dynamite, dioxins, and petroleum contaminated soils (Asghari et al. Citation2013; Hofrichter Citation2002; Harayama Citation1997; Janda Citation2015). There are synonyms and systematic locations in (Brambl and Marzluf Citation2013; Ruckenstein et al. Citation2019).
Table 2. The synonym properties and systematic places of Phanerochaete chrysoporium.
15.3. Trames Versicolor
Also known as Turkey tail fungus, Trames is a member of Versicolor basidiomycete. The fructification organ consists of a dark-light brown band structure. The colour distribution is due to genetic polymorphism and varies from yellowish-brown, white, blue, orange, and even chestnut colour. Basidiocarp has a fan-shaped geometry; it can reach 0.5 cm thickness and 10 cm diameter and is in the form of a shelf in a row. Its surface is in the way of dark and light concentric bands. Its surface is velvety or flat. There are 3–5 spores on the surface in the circular, small or angular form in 20 mm age. Mature basidiospores are up to 2 × 6 µm in dice structure. The vegetative range of the trimitic hif system has 3–10 µm length. The anamorphic phase of T. Versicolor consists of chlamydospores and oodiospores. This species is widespread in subtropic and tropical forests. Its enzyme is stable and can disrupt the lignin structure of hardwood trees. It can also cause wood rot in live hosts. It is an essential type of white fungus. The most important enzymes are the five isoforms of Mangan peroxidase (MnP), 16 isoforms of lignin peroxidase (LiP), laccase, aviselase, carboxymethyl cellulose, and cellobiose dehydrogenase. Proteases play a role in and out of the cell play an active role in the regulation of peroxidase and laccase activity. They regulate the production of laccase by controlling the intracellular presence of cofactor enzymes such as CtaA and tahA. T. Versicolor stabilises Mn (III) and MnP with oxalic acid to chelate toxic metals (Center USDoETI, Scientific USDoEOo, Information T, Citation1990; United States Department of Energy PETC Citation1987; Elke Hammer et al. Citation2001; Doble and Kumar Citation2005; Grogan Citation2012; Chozhavendhan et al. Citation2020; Zhang et al. Citation2020).
A used the laccase polychlorinated biphenyls (PCB) produced by T. Versicolor is to break down many new biodegradable synthetic chemicals (xenobiotics) such as polyaromatic hydrocarbons (PAH) and textile dyes. Besides, used extracellular enzymes are in the paper industry. A used organism is in the biological method for bleaching the pulp. These species are also used effectively in the synthesis or degradation of antibiotics. It has an essential role in the synthesis of aromatic compounds (especially carotene). They are involved in the biodegradation of high-weight compounds of oil (Center USDoETI, Scientific USDoEOo, Information T, Citation1990; United States Department of Energy PETC Citation1987; Elke Hammer et al. Citation2001; Doble and Kumar Citation2005; Grogan Citation2012; Chozhavendhan et al. Citation2020; Zhang et al. Citation2020).
There are synonyms and systematic locations in (Beck et al. Citation2018).
Table 3. Synonym properties and systematic locations of Trames versicolor.
The highlights of the outcomes are outlined below:
Physical methods remove inorganic sulphur in coal
Bacteria in symbiosis relationship effects mainly pyrite sulphur in coal
Mesoacidophilic bacteria are useful on inorganic sulphur in coal
Fungus enzymes are active on organic sulphur in coal
Chemical methods primarily effective on inorganic sulphur in coal.
16. Conclusions
The following studies can be suggested:
Establishing a microbial library that removes organic and inorganic sulphur
To present the availability of these cultures in natural environments (geological research)
Registration of isolates from each culture
Determining to what extent each microbial culture should be applied to which type of coal (rank, etc.)
Determination of species that appear dominant by molecular techniques while determining microbial culture
Biooxidation, bioleaching, selective metal retention, and use in enzymatic processes with the isolated library created within the scope of the study.
Carrying out desulphurisation at the fermentor scale
Biological improvement in acidic mine drains according to the isolates determined.
The emphasised technological studies are that biological methods should be applied more in future applications due to the high temperature and pressure that determine the cost of chemical methods. In the anaerobic degradation process used in coal, Ece and Koyunoğlu have developed a method of improvement that provides the slag that occurs in the combustion boiler as a result of the combustion of the coal in the process of healing the coal and earlier ignition of the coal (Cemil Koyunoğlu Citation2017). A started technological means such as biomethane production is by adding the solvent used in this process to the procedures specified above, especially by injecting enzymes into the coal deposits whose mineral process has been completed.
This study made specific determinations to carry out private research different from previous studies in terms of revealing which biological structure is suitable for which sulphur type. There is a need for important genetic studies to realise each sulphur species with a specific bacterial culture. DNA studies of a particular bacterial culture are important in this sense to shorten the completion times of enzymatic reactions. Expanding the desulphurisation of coal by the microbial method is an important alternative in the rapid solution of the global climate problem. There is some information that serious genetic studies are carried out to reduce the reaction. In our next study, evaluations will be made on the application of new technologies, especially with genetic studies, and the increase of sulphur removal efficiency with enzyme kinetic mechanisms
Disclosure statement
No potential conflict of interest was reported by the authors.
References
- Abdollahy, M., A.Z. Moghaddam, and K. Rami. 2006. “Desulfurization of Mezino Coal Using Combination of ‘Flotation’ and ‘Leaching with Potassium Hydroxide/Methanol’.” Fuel 85 (7): 1117–1124. https://doi.org/10.1016/j.fuel.2005.10.011.
- Ahamed, M.A.A., M.S.A. Perera, S.K. Matthai, et al. 2019. “Coal Composition and Structural Variation with Rank and its Influence on the Coal-Moisture Interactions Under Coal Seam Temperature Conditions – A Review Article.” Journal of Petroleum Science and Engineering 180: 901–917. https://doi.org/10.1016/j.petrol.2019.06.007.
- Akinyemi, S.A., W.M. Gitari, L.F. Petrik, et al. 2019. “Environmental Evaluation and Nano-Mineralogical Study of Fresh and Unsaturated Weathered Coal fly Ashes.” Science of The Total Environment 663: 177–188. https://doi.org/10.1016/j.scitotenv.2019.01.308.
- Alcalde, M. 2015. “Engineering the Ligninolytic Enzyme Consortium.” Trends in Biotechnology 33 (3): 155–162. https://doi.org/10.1016/j.tibtech.2014.12.007.
- Ali, A., and C. Zhao. 2020. “Direct Liquefaction Techniques on Lignite Coal: A Review.” Chinese Journal of Catalysis 41 (3): 375–389. https://doi.org/10.1016/S1872-2067(19)63492-3.
- Aliebrahimi, S., J. Raheb, G. Ebrahimipour, et al. 2015. “Designing a New Recombinant Indigenous Klebsiella oxytoca ISA4 by Cloning of dsz Genes.” Energy Sources, Part A: Recovery, Utilization, and Environmental Effects 37 (19): 2056–2063. doi:10.1080/15567036.2012.662266.
- Almashjary, K.H., M. Khalid, S. Dharaskar, et al. 2018. “Optimisation of Extractive Desulfurization Using Choline Chloride-Based Deep Eutectic Solvents.” Fuel 234: 1388–1400. https://doi.org/10.1016/j.fuel.2018.08.005.
- Andrews, G.F., and K.S. Noah. 1997. “The Slurry-Column Coal Beneficiation Process.” Fuel Processing Technology 52 (1): 247–266. https://doi.org/10.1016/S0378-3820(97)00033-7.
- Arnold, S., K. Moss, M. Henkel, et al. 2017. “Biotechnological Perspectives of Pyrolysis Oil for a Bio-Based Economy.” Trends in Biotechnology 35 (10): 925–936. https://doi.org/10.1016/j.tibtech.2017.06.003.
- Artanto, Y., W.R. Jackson, P.J. Redlich, et al. 2000. “Liquefaction Studies of Some Indonesian low Rank Coals.” Fuel 79 (11): 1333–1340. https://doi.org/10.1016/S0016-2361(99)00275-6.
- Asghari, I., S.M. Mousavi, F. Amiri, et al. 2013. “Bioleaching of Spent Refinery Catalysts: A Review.” Journal of Industrial and Engineering Chemistry 19 (4): 1069–1081. https://doi.org/10.1016/j.jiec.2012.12.005.
- Asmatulu, R., B. Ipekoglu, and Y. Dasdemir. 1998. “The Effects of Coal Based Power Stations on The Environment in Turkey.” In Chemistry, Energy and the Environment, edited by C. A. C. Sequeira, and J. B. Moffat, 5–15. Estoril: Woodhead Publishing.
- Baatar, B., T. Gan-Erdene, M. Myekhlai, et al. 2017. “Desulfurization of Coal Using the Electrochemical Technique in Neutral and Alkaline Media.” Energy Sources, Part A: Recovery, Utilization, and Environmental Effects 39 (15): 1610–1616. doi:10.1080/15567036.2017.1356886.
- Basu, P. 2018. “Chapter 7 – Gasification Theory.” In Biomass Gasification, Pyrolysis and Torrefaction. 3rd ed., edited by P. Basu, 211–262. San Diego: Academic Press.
- Bayram, Z., T. Bozdemir, T. Durusoy, et al. 2002. “Biodesulfurization of Mengen Lignite with Rhodoccocus rhodochrous: Effects of Lignite Concentration and Retreatment.” Energy Sources 24 (7): 625–631. doi:10.1080/00908312.2002.11877437.
- Beck, S., E. Berry, S. Duke, et al. 2018. “Characterization of Trametes Versicolor Laccase-Catalyzed Degradation of Estrogenic Pollutants: Substrate Limitation and Product Identification.” International Biodeterioration & Biodegradation 127: 146–159. https://doi.org/10.1016/j.ibiod.2017.11.020.
- Beckmann, S., A.W.S. Luk, M.-L. Gutierrez-Zamora, et al. 2019. “Long-Term Succession in a Coal Seam Microbiome During in situ Biostimulation of Coalbed-Methane Generation.” The ISME Journal 13 (3): 632–650. doi:10.1038/s41396-018-0296-5.
- Behrouzifar, A., S. Rowshanzamir, Z. Alipoor, et al. 2016. “Application of a Square Wave Potentiometry Technique for Electroreductive Sulfur Removal from a Thiophenic Model Fuel.” International Journal of Environmental Science and Technology 13 (12): 2883–2892. doi:10.1007/s13762-016-1121-1.
- Belyakov, N. 2019. “Chapter 8 – Efficient and Clean Combustion of Fossil Fuels Within Boiler Island.” In Sustainable Power Generation, edited by N. Belyakov, 179–200. London: Academic Press.
- Benedik, M.J., P.R. Gibbs, R.R. Riddle, et al. 1998. “Microbial Denitrogenation of Fossil Fuels.” Trends in Biotechnology 16 (9): 390–395. https://doi.org/10.1016/S0167-7799(98)01237-2.
- Benito G.G., G. Osorio, and D. Bonilla. 1995. “Biological Sulphur Removal by Thiobacillus Thiooxidans in Fine Coal Coming from a Flotation Washing Plant.” In Vol. 24 of Coal Science and Technology, edited by J. A. Pajares, and J. M. D. Tascón, 1745–1748. Lexington: Elsevier.
- Benson, S.A., J.D. Laumb, C.R. Crocker, et al. 2005. “SCR Catalyst Performance in Flue Gases Derived from Subbituminous and Lignite Coals.” Fuel Processing Technology 86 (5): 577–613. https://doi.org/10.1016/j.fuproc.2004.07.004.
- Berkowitz, N. 1979. “Chapter 15 – Environmental Aspects of Coal Utilization.” In An Introduction to Coal Technology, edited by N. Berkowitz, 323–335. Academic Press.
- Biology and Fuel Science. 1995. Fuel Processing Technology 43 (2): 177. https://doi.org/10.1016/0378-3820(95)90027-6.
- Brambl, R., and G.A. Marzluf. 2013. Biochemistry and Molecular Biology. Berlin Heidelberg: Springer.
- Bressler, D.C., J.A. Norman, and P.M. Fedorak. 1997. “Ring Cleavage of Sulfur Heterocycles: How Does It Happen?” Biodegradation 8 (5): 297–311. doi:10.1023/A:1008283207090.
- Brothers, J.A., and J.J. Starzomski. 1976. “Reaction of Pyritic Sulphur in Coal with Lime and Calcined Dolomite During the Coking Process.” Fuel 55 (2): 105–108. https://doi.org/10.1016/0016-2361(76)90004-1.
- Bubenick, D.V., F.A. Record, and R.J. Kindya. 1983. “Acid Rain – an Overview of the Problem.” Environmental Progress 2 (1): 15–32. doi:10.1002/ep.670020107.
- Çelik, P.A., DÖ Aksoy, S. Koca, et al. 2019. “The Approach of Biodesulfurization for Clean Coal Technologies: A Review.” International Journal of Environmental Science and Technology 16 (4): 2115–2132. doi:10.1007/s13762-019-02232-7.
- Cemil Koyunoğlu, E.P. 2017. “Biological Improvement of Coal: Formica Rufa Enzymes Over Mining Fluid and Rumen Liquid.” Advances in Energy and Power 5 (4): 58–62. doi:10.13189/aep.2017.050403.
- Center USDoETI, Scientific USDoEOo, Information T. 1990. Energy Research Abstracts. Technical Information Center, U.S. Department of Energy (15. c.,11. no.).
- Chadwick, M. J., and N. Lindman, eds. 1982. “Chapter 9 – Impacts of Coal on Natural Environmental Systems.” In Environmental Implications of Expanded Coal Utilization: New York, Pergamon, 230–272.
- Chakrabarti, J.N. 1978. “Chapter 9 – Analytical Procedures for Sulfur in Coal Desulfurization Products.” In Analytical Methods for Coal and Coal Products, edited by C. Karr, 279–322. New York: Academic Press.
- Chakrabarty, A.M. 1985. “Genetically-manipulated Microorganisms and Their Products in the Oil Service Industries.” Trends in Biotechnology 3 (2): 32–39. https://doi.org/10.1016/0167-7799(85)90056-3.
- Chapter 11 – Physical Desulphurisation of Coal. 1989. The Problems of Sulphur, 65–78. London: Butterworth-Heinemann.
- Chou, C.-L. 2012. “Sulfur in Coals: A Review of Geochemistry and Origins.” International Journal of Coal Geology 100: 1–13. https://doi.org/10.1016/j.coal.2012.05.009.
- Chozhavendhan, S., G. Karthiga Devi, B. Bharathiraja, et al. 2020. “Chapter 9 – Assessment of Crude Glycerol Utilization for Sustainable Development of Biorefineries.” In Refining Biomass Residues for Sustainable Energy and Bioproducts, edited by R. P. Kumar, E. Gnansounou, and J. K. Raman, et al., 195–212. London: Academic Press.
- Civeira, M., M.L. Oliveira, J.C. Hower, et al. 2016. “Modification, Adsorption, and Geochemistry Processes on Altered Minerals and Amorphous Phases on the Nanometer Scale: Examples from Copper Mining Refuse, Touro, Spain.” Environmental science and pollution research international 23 (7): 6535–45. doi:10.1007/s11356-015-5816-5.
- Civeira, M.S., C.G. Ramos, M.L.S. Oliveira, et al. 2016. “Nano-Mineralogy of Suspended Sediment during the Beginning of Coal Rejects Spill.” Chemosphere 145: 142–147. https://doi.org/10.1016/j.chemosphere.2015.11.059.
- Clariant to Supply Unique Sorbent to RTI International for Its Warm Gas Desulfurization Technology. 2018. Focus on Catalysts 2018 (2): 6. https://doi.org/10.1016/j.focat.2018.01.043.
- Clark, T.R., and G.J. Olson. 1993. “Biodepyritization of Pittsburgh Seam Coal: Mechanism and Implications for Process Design.” In Vol. 21 of Coal Science and Technology, edited by B. K. Parekh, and J. G. Groppo, 323–330. Kentucky: Elsevier.
- Cliffe, K.R., and M.M. Syed. 1993. “Low Temperature Oxidation of Sulphur.” In Vol. 21: of Coal Science and Technology, edited by B. K. Parekh, and J. G. Groppo, 297–304. Kentucky: Elsevier.
- Constantí, M., J. Giralt, and A. Bordons. 1996. “Degradation and Desulfurization of Dibenzothiophene Sulfone and Other Sulfur Compounds by Agrobacterium MC501 and a Mixed Culture.” Enzyme and Microbial Technology 19 (3): 214–219. https://doi.org/10.1016/0141-0229(95)00236-7.
- Crawford, D.L., and R.K. Gupta. 1991. “Influence of Cultural Parameters on the Depolymerization of a Soluble Lignite Coal Polymer by Pseudomonas Cepacia DLC-07.” Resources, Conservation and Recycling 5 (2): 245–254. https://doi.org/10.1016/0921-3449(91)90028-M.
- Dai, S., A. Bechtel, C.F. Eble, et al. 2020. “Recognition of Peat Depositional Environments in Coal: A Review.” International Journal of Coal Geology 219: 103383. https://doi.org/10.1016/j.coal.2019.103383.
- Dai, S., J.C. Hower, R.B. Finkelman, et al. 2020. “Organic Associations of Non-mineral Elements in Coal: A Review.” International Journal of Coal Geology 218: 103347. https://doi.org/10.1016/j.coal.2019.103347.
- Dalmora, A.C., C.G. Ramos, M.L.S. Oliveira, et al. 2016. “Chemical Characterization, Nano-Particle Mineralogy and Particle Size Distribution of Basalt Dust Wastes.” Science of The Total Environment 539: 560–565. https://doi.org/10.1016/j.scitotenv.2015.08.141.
- Dehkordi, A.M., M.A. Sobati, and M.A. Nazem. 2013. “An Experimental Investigation on the Oxidative Desulfurization of Kerosene Feedstock.” Energy Sources, Part A: Recovery, Utilization, and Environmental Effects 35 (3): 226–234. doi:10.1080/15567036.2010.509087.
- Demirbas, A., and M. Balat. 2004. “Coal Desulfurization via Different Methods.” Energy Sources 26 (6): 541–550. doi:10.1080/00908310490429669.
- Deriase, S.F., N.S. El-Gendy, and H.N. Nassar. 2012. “Enhancing Biodegradation of Dibenzothiophene by Bacillus sphaericus HN1 Using Factorial Design and Response Surface Optimization of Medium Components.” Energy Sources, Part A: Recovery, Utilization, and Environmental Effects 34 (22): 2073–2083. doi:10.1080/15567036.2010.497801.
- Doble, M., and A. Kumar. 2005. “Chapter 24 – Petroleum Hydrocarbon Pollution.” In Biotreatment of Industrial Effluents, edited by M. Doble, and A. Kumar, 241–253. Burlington: Butterworth-Heinemann.
- Duarte, A.L., K. DaBoit, M.L.S. Oliveira, et al. 2019. “Hazardous Elements and Amorphous Nanoparticles in Historical Estuary Coal Mining Area.” Geoscience Frontiers 10 (3): 927–939. https://doi.org/10.1016/j.gsf.2018.05.005.
- Elke Hammer, Wolf-Dieter, Ulrike Lindequist Jülich, Annett Schäfer, and Frieder Schauer. 2001. Biotransformation of Biologically Active Compounds Made of Various Classes of Chemical Substances by Means of Laccase and Manganese Peroxidase Enzymes.
- Ernst WHO. 1998. “Sulfur Metabolism in Higher Plants: Potential for Phytoremediation.” Biodegradation 9 (3): 311–318. doi:10.1023/A:1008250827209.
- Fairbairn, D.J., and M.E. Bushell. 1992. “Evaluation of Continuous Enrichment as a Method for Isolating Microbial Cultures Capable of Coal Desulfurization.” Enzyme and Microbial Technology 14 (1): 48–52. https://doi.org/10.1016/0141-0229(92)90025-J.
- Faison, B.D. 1991. “Microbial Conversions of Low Rank Coals.” Bio/Technology 9 (10): 951–956. doi:10.1038/nbt1091-951.
- Ferrari, V., S.R. Taffarel, E. Espinosa-Fuentes, et al. 2019. “Chemical Evaluation of by-Products of the Grape Industry as Potential Agricultural Fertilizers.” Journal of Cleaner Production 208: 297–306. https://doi.org/10.1016/j.jclepro.2018.10.032.
- Gasparotto, J., P.R. Chaves, K. da Boit Martinello, et al. 2019. “Obesity Associated with Coal Ash Inhalation Triggers Systemic Inflammation and Oxidative Damage in the Hippocampus of Rats.” Food and Chemical Toxicology 133: 110766. https://doi.org/10.1016/j.fct.2019.110766.
- Gogoi, B.K., and R.L. Bezbaruah. 2002. “Microbial Degradation of Sulfur Compounds Present in Coal and Petroleum.” In Vol. 36 of Progress in Industrial Microbiology, edited by Ved Pal S, and S. Raymond D, 427–456. Netherland: Elsevier.
- Gorbaty, M.L., S.R. Kelemen, and G.N. George. 1991. “Characterization and Chemistry of Organically Bound Sulfur during Coal Conversion.” In 1991 International Conference on Coal Science Proceedings, edited by International Energy Agency Coal Research L, 961–964. London: Butterworth-Heinemann.
- Gredilla, A., Fdez-Ortiz de Vallejuelo S, A. Rodriguez-Iruretagoiena, et al. 2019. “Evidence of Mercury Sequestration by Carbon Nanotubes and Nanominerals Present in Agricultural Soils from a Coal Fired Power Plant Exhaust.” Journal of Hazardous Materials 378: 120747. https://doi.org/10.1016/j.jhazmat.2019.120747.
- Grogan, G. 2012. “7.14 Oxidation: Asymmetric Enzymatic Sulfoxidation.” In Comprehensive Chirality, edited by E. M. Carreira, and H. Yamamoto, 295–328. Amsterdam: Elsevier.
- Gu, X.Y., J.W.C. Wong, and R.D. Tyagi. 2017. “Chapter 11 – Bioleaching of Heavy Metals from Sewage Sludge for Land Application.” In Current Developments in Biotechnology and Bioengineering, edited by J. W. C. Wong, R. D. Tyagi, and A. Pandey, 241–265. Amsterdam: Elsevier.
- Guo, R., J. Yang, and Z. Liu. 2004. “Influence of Heat Treatment Conditions on Release of Chlorine from Datong Coal.” Journal of Analytical and Applied Pyrolysis 71 (1): 179–186. https://doi.org/10.1016/S0165-2370(03)00086-X.
- Gürü, M. 2007. “Oxidative Desulfurization of Aşkale Coal by Nitric Acid Solution.” Energy Sources, Part A: Recovery, Utilization, and Environmental Effects 29 (5): 463–469. doi:10.1080/009083190966054.
- Gürü, M., M. Cubuk, S. Dursun, et al. 2006. “Biodesulfurization of Cayirhan Lignites.” Energy Sources, Part A: Recovery, Utilization, and Environmental Effects 28 (6): 559–565. doi:10.1080/009083190928056.
- Haddadin, J., C. Dagot, and M. Fick. 1995. “Models of Bacterial Leaching.” Enzyme and Microbial Technology 17 (4): 290–305. https://doi.org/10.1016/0141-0229(94)00032-8.
- Harayama, S. 1997. “Polycyclic Aromatic Hydrocarbon Bioremediation Design.” Current Opinion in Biotechnology 8 (3): 268–273. https://doi.org/10.1016/S0958-1669(97)80002-X.
- Hofrichter, M. 2002. “Review: Lignin Conversion by Manganese Peroxidase (MnP).” Enzyme and Microbial Technology 30 (4): 454–466. https://doi.org/10.1016/S0141-0229(01)00528-2.
- Holst, O., B. Stenberg, and M. Christiansson. 1998. “Biotechnological Possibilities for Waste Tyre-Rubber Treatment.” Biodegradation 9 (3): 301–310. doi:10.1023/A:1008337708006.
- Huffman, G.P., N. Shah, F.E. Huggins, et al. 1993. “Further Sulfur Speciation Studies by Sulfur K-Edge XANES Spectroscopy.” In Vol. 21 of Coal Science and Technology, edited by B. K. Parekh, and J. G. Groppo, 1–13. Kentucky: Elsevier.
- Hussein, A.A., and A.B. Fadhil. 2021. “Kinetics and Isothermal Evaluations of Adsorptive Desulfurization of Dibenzothiophene Over Mixed Bio-Wastes Derived Activated Carbon.” Energy Sources, Part A: Recovery, Utilization, and Environmental Effects, 1–20. doi:10.1080/15567036.2021.1895372.
- Janda, K. 2015. “6.13 – Petroleum Products.” In Handbook of Material Biodegradation, Biodeterioration, and Biostablization. 2nd ed., edited by M. Falkiewicz-Dulik, K. Janda, and G. Wypych, 257–375. Toronto: ChemTec Publishing.
- Jiang, L., H. Zhou, and X. Peng. 2007. “Bio-Oxidation of Pyrite, Chalcopyrite and Pyrrhotite by Acidithiobacillus Ferrooxidans.” Chinese Science Bulletin 52 (19): 2702–2714. doi:10.1007/s11434-007-0352-4.
- Kabe, T., A. Ishihara, E. W. Qian, et al., eds. 2004. “Chapter 2 – Chemical and Macromolecular Structure of Coal.” In Vol. 150 of Studies in Surface Science and Catalysis, 81–126. Louvain-la-Neuve: Elsevier.
- Kalia, V.C., S. Lal, R. Ghai, et al. 2003. “Mining Genomic Databases to Identify Novel Hydrogen Producers.” Trends in Biotechnology 21 (4): 152–156. https://doi.org/10.1016/S0167-7799(03)00028-3.
- Kargi, F. 1982. “Microbiological Coal Desulphurization.” Enzyme and Microbial Technology 4 (1): 13–19. https://doi.org/10.1016/0141-0229(82)90004-7.
- Kargi, F. 1986. “Microbial Methods for Desulfurization of Coal.” Trends in Biotechnology 4 (11): 293–297. https://doi.org/10.1016/0167-7799(86)90232-5.
- Kazemi, A., M. Malayeri, A. Gharibi Kharaji, et al. 2014. “Feasibility Study, Simulation and Economical Evaluation of Natural Gas Sweetening Processes – Part 1: A Case Study on a low Capacity Plant in Iran.” Journal of Natural Gas Science and Engineering 20: 16–22. https://doi.org/10.1016/j.jngse.2014.06.001.
- Ken, B.S., and B.K. Nandi. 2019. “Desulfurization of High Sulfur Indian Coal by Oil Agglomeration Using Linseed Oil.” Powder Technology 342: 690–697. https://doi.org/10.1016/j.powtec.2018.10.045.
- Kilbane, J.J. 1989. “Desulfurization of Coal: The Microbial Solution.” Trends in Biotechnology 7 (4): 97–101. https://doi.org/10.1016/0167-7799(89)90007-3.
- Kilbane, J.J. 1990. “Sulfur-specific Microbial Metabolism of Organic Compounds.” Resources, Conservation and Recycling 3 (2): 69–79. https://doi.org/10.1016/0921-3449(90)90046-7.
- Kilbane, J.J. 2006. “Microbial Biocatalyst Developments to Upgrade Fossil Fuels.” Current Opinion in Biotechnology 17 (3): 305–314. https://doi.org/10.1016/j.copbio.2006.04.005.
- Klasson, K.T., M.D. Ackerson, E.C. Clausen, et al. 1993. “Biological Conversion of Coal and Coal-Derived Synthesis Gas.” Fuel 72 (12): 1673–1678. https://doi.org/10.1016/0016-2361(93)90354-5.
- Klein, J. 1998. “Technological and Economic Aspects of Coal Biodesulfurisation.” Biodegradation 9 (3): 293–300. doi:10.1023/A:1008282216016.
- Kuenen, J.G., and L.A. Robertson. 1992. “The Use of Natural Bacterial Populations for the Treatment of Sulphur-Containing Wastewater.” Biodegradation 3 (2): 239–254. doi:10.1007/BF00129086.
- Kumar, D., and D. Kumar. 2018. “Chapter 10 – High-Sulphur Coal Washing.” In Sustainable Management of Coal Preparation, edited by D. Kumar, and D. Kumar, 231–241. Duxford: Woodhead Publishing.
- Laumb, J.D., B.C. Folkedahl, and C.J. Zygarlicke. 2008. “Chapter 4 – Characteristics and Behavior of Inorganic Constituents.” In Combustion Engineering Issues for Solid Fuel Systems, edited by B. G. Miller, and D. A. Tillman, 133–170. Burlington: Academic Press.
- León-Mejía, G., L.F. Silva, M.S. Civeira, et al. 2016. “Cytotoxicity and Genotoxicity Induced by Coal and Coal Fly Ash Particles Samples in V79 Cells.” Environmental Science and Pollution Research International 23 (23): 24019–24031. 10.1007/s11356-016-7623-z.
- Li, J., S. Lu, D. Liu, et al. 2019. “Chapter 14 – Dynamic Gas Flow in Coals and Its Evaluation.” In Petrophysical Characterization and Fluids Transport in Unconventional, edited by J. Cai, and X. Hu, 277–300. Reservoirs: Elsevier.
- Liu, T., J. Hou, and Y. Peng. 2016. “Bacterial Removal of Sulfur from the China Lignite by a Newly Isolated Bacterium, Sinomonas flava XL4.” Environmental Progress & Sustainable Energy 35 (2): 374–379. doi:10.1002/ep.12230.
- Liu, T., J.-H. Hou, and Y.-L. Peng. 2017. “Biodesulfurization from the High Sulfur Coal with a Newly Isolated Native Bacterium, Aspergillus sp.” DP06. Environmental Progress & Sustainable Energy 36 (2): 595–599. doi:10.1002/ep.12464.
- Liu, F., Y. Lei, J. Shi, et al. 2020. “Effect of Microbial Nutrients Supply on Coal Bio-Desulfurization.” Journal of Hazardous Materials 384: 121324. https://doi.org/10.1016/j.jhazmat.2019.121324.
- Longwell, J.P., E.S. Rubin, and J. Wilson. 1995. “Coal: Energy for the Future.” Progress in Energy and Combustion Science 21 (4): 269–360. https://doi.org/10.1016/0360-1285(95)00007-0.
- Malani, R.S., A.H. Batghare, J.B. Bhasarkar, et al. 2021. “Kinetic Modelling and Process Engineering Aspects of Biodesulfurization of Liquid Fuels: Review and Analysis.” Bioresource Technology Reports 14: 100668. https://doi.org/10.1016/j.biteb.2021.100668.
- Martínez, O., A. Aller, J. Alonso, et al. 1995. “Biodesulphurization of Coals from the North of León (Spain). Optimization of Process Variables.” In Vol. 24 of Coal Science and Technology, edited by J. A. Pajares, and J. M. D. Tascón, 1749–1752. Kentucky: Elsevier.
- Matsumura, S. 2005. “Chapter 14 – Mechanism of Biodegradation.” In Biodegradable Polymers for Industrial Applications, edited by R. Smith, 357–410. New York: Woodhead Publishing.
- McIlwain, M.E., and P.R. Dugan. 1990. “Introduction and Overview of the Third Workshop on Bioprocessing of Coals.” Resources, Conservation and Recycling 3 (2): 53–57. https://doi.org/10.1016/0921-3449(90)90044-5.
- Mokhatab, S., and W.A. Poe. 2012. “Chapter 8 – Sulfur Recovery and Handling.” In Handbook of Natural Gas Transmission and Processing. 2nd ed., edited by S. Mokhatab, and W. A. Poe, 291–316. Boston: Gulf Professional Publishing.
- Moldoveanu, S.C. 2021. “Chapter 11 – Analytical Pyrolysis of Several Organic Geopolymers.” In Vol. 20 of Analytical Pyrolysis of Natural Organic Polymers. 2nd ed., edited by S. C. Moldoveanu, 403–425. Amsterdam: Elsevier.
- Nam, Y.-W., and K.-S. Park. 2004. “Thermal and Chemical Desulfurization of low Sulfur Coals.” Korean Journal of Chemical Engineering 21 (2): 370–377. doi:10.1007/BF02705422.
- Natarajan, K.A. 2018. “Chapter 10 – Microbially Induced Mineral Beneficiation.” In Biotechnology of Metals, edited by K. A. Natarajan, 243–304. Amsterdam: Elsevier.
- Naudé, G., J. Hoffman, S.J. Theron, et al. 2013. “The Use of X-Ray Computed Tomography in the Characterisation of Coal and Associated Char Reductants.” Minerals Engineering 52: 143–154. https://doi.org/10.1016/j.mineng.2013.05.012.
- Nazari, F., M.-E. Kefayati, and J. Raheb. 2017. “Isolation, Identification, and Characterization of a Novel Chemolithoautotrophic Bacterium with High Potential in Biodesulfurization of Natural or Industrial Gasses and Biogas.” Energy Sources, Part A: Recovery, Utilization, and Environmental Effects 39 (10): 971–977. doi:10.1080/15567036.2016.1263255.
- Oliveira, M.L.S., M. Izquierdo, X. Querol, et al. 2019. “Nanoparticles from Construction Wastes: A Problem to Health and the Environment.” Journal of Cleaner Production 219: 236–243. https://doi.org/10.1016/j.jclepro.2019.02.096.
- Oliveira, M.L.S., B.K. Saikia, K. da Boit, et al. 2019. “River Dynamics and Nanopaticles Formation: A Comprehensive Study on the Nanoparticle Geochemistry of Suspended Sediments in the Magdalena River, Caribbean Industrial Area.” Journal of Cleaner Production 213: 819–824. https://doi.org/10.1016/j.jclepro.2018.12.230.
- Olson, G.J., and F.E. Brinckman. 1986. “Bioprocessing of Coal.” Fuel 65 (12): 1638–1646. doi:https://doi.org/10.1016/0016-2361(86)90262-0.
- Olszewska, D. 2011. “Application of Modified Montmorillonite for Desulfurization During the Combustion of Hard Coal.” Fuel Processing Technology 92 (12): 2412–2419. https://doi.org/10.1016/j.fuproc.2011.08.022.
- Olszewska, D. 2012. “Application of XPS Method in the Research Into Ni ion-Modified Montmorillonite as a SO2 Sorbent.” Fuel Processing Technology 95: 90–95. https://doi.org/10.1016/j.fuproc.2011.11.023.
- Parekh, B.K. 2003. “Coal Desulfurization: High Efficiency Preparation Methods: by S. Komar Kawatara and Timothy C. Eisele. Taylor and Francis Inc., New York, NY, 360 pp.” International Journal of Coal Geology 55 (1): 71. https://doi.org/10.1016/S0166-5162(03)00065-X.
- Pashin, J.C., et al. 2020. “Chapter 2 – Geology of North American Coalbed Methane Reservoirs.” In Coal Bed Methane. 2nd ed., edited by P. Thakur, S. J. Schatzel, and K. Aminian, 35–64. Oxford: Elsevier.
- Raheb, J., S. Ghaffari, M.J. Hajipour, et al. 2012. “The in vitro Rhamnolipid Biosurfactant Reduced Energy Consuming in Biodesulfurization Activity of Genetically Engineered Strain Pseudomonas aeruginosa ATCC9027.” Energy Sources, Part A: Recovery, Utilization, and Environmental Effects 34 (14): 1318–1325. doi:10.1080/15567031003735261.
- Raheb, J., and M.J. Hajipour. 2011a. “The Effect of Elimination of the dszC Gene in Energy Consuming of Biodesulfurization in Broken 4S Pathway.” Energy Sources, Part A: Recovery, Utilization, and Environmental Effects 33 (19): 1814–1821. doi:10.1080/15567030903419455.
- Raheb, J., and M.J. Hajipour. 2011b. “The Stable Rhamnolipid Biosurfactant Production in Genetically Engineered Pseudomonas Strain Reduced Energy Consumption in Biodesulfurization.” Energy Sources, Part A: Recovery, Utilization, and Environmental Effects 33 (22): 2113–2121. doi:10.1080/15567030903503167.
- Raheb, J., and M.J. Hajipour. 2012. “The Characterization of Biosurfactant Production Related to Energy Consumption of Biodesulfurization in Pseudomonas aeruginosa ATCC9027.” Energy Sources, Part A: Recovery, Utilization, and Environmental Effects 34 (15): 1391–1399. doi:10.1080/15567036.2010.481659.
- Raheb, J., B. Memari, and M.J. Hajipour. 2011. “Gene-Manipulated Desulfurizing Strain Pseudomonas Putida Reduced Energy Consuming in the Biodesulfurization Process.” Energy Sources, Part A: Recovery, Utilization, and Environmental Effects 33 (21): 2018–2026. doi:10.1080/15567030903515054.
- Rodriguez-Iruretagoiena, A., S.F.-O. de Vallejuelo, A. de Diego, et al. 2016. “The Mobilization of Hazardous Elements After a Tropical Storm Event in a Polluted Estuary.” Science of The Total Environment 565: 721–729. https://doi.org/10.1016/j.scitotenv.2016.05.024.
- Ruckenstein, E., H. Li, and C. Cheng. 2019. Concentrated Emulsion Polymerization. New York: CRC Press.
- Saed, D., H.N. Nassar, N.S. El-Gendy, et al. 2014. “The Enhancement of Pyrene Biodegradation by Assembling MFe3O4 Nano-sorbents on the Surface of Microbial Cells.” Energy Sources, Part A: Recovery, Utilization, and Environmental Effects 36 (17): 1931–1937. doi:10.1080/15567036.2014.889782.
- Saha, B., S. Vedachalam, and A.K. Dalai. 2021. “Review on Recent Advances in Adsorptive Desulfurization.” Fuel Processing Technology 214: 106685. https://doi.org/10.1016/j.fuproc.2020.106685.
- Saikia, B.K., A.C. Dalmora, R. Choudhury, et al. 2016. “Effective Removal of Sulfur Components from Brazilian Power-Coals by Ultrasonication (40kHz) in Presence of H2O2.” Ultrasonics Sonochemistry 32: 147–157. https://doi.org/10.1016/j.ultsonch.2016.03.007.
- Saikia, M., T. Das, N. Dihingia, et al. 2020. “Formation of Carbon Quantum Dots and Graphene Nanosheets from Different Abundant Carbonaceous Materials.” Diamond and Related Materials 106: 107813. https://doi.org/10.1016/j.diamond.2020.107813.
- Sehn, J.L., F.B. de Leão, K. da Boit, et al. 2016. “Nanomineralogy in the Real World: A Perspective on Nanoparticles in the Environmental Impacts of Coal Fire.” Chemosphere 147: 439–443. https://doi.org/10.1016/j.chemosphere.2015.12.065.
- Selvaraj, P.T., M.H. Little, and E.N. Kaufman. 1997. “Analysis of Immobilized Cell Bioreactors for Desulfurization of Flue Gases and Sulfite/Sulfate-Laden Wastewater.” Biodegradation 8 (4): 227–236. doi:10.1023/A:1008200411998.
- Sen, K., and P.S. Dash. 2020. “Quantum Chemical Perspective of Coal Molecular Modeling: A Review.” Fuel 279: 118539. https://doi.org/10.1016/j.fuel.2020.118539.
- Shirkavand, E., S. Baroutian, D.J. Gapes, et al. 2016. “Combination of Fungal and Physicochemical Processes for Lignocellulosic Biomass Pretreatment – A Review.” Renewable and Sustainable Energy Reviews 54: 217–234. https://doi.org/10.1016/j.rser.2015.10.003.
- Silva, L.F.O., T.J. Crissien, C.H. Sampaio, et al. 2020. “Occurrence of Carbon Nanotubes and Implication for the Siting of Elements in Selected Anthracites.” Fuel 263: 116740. https://doi.org/10.1016/j.fuel.2019.116740.
- Silva, L.F.O., T.J. Crissien, B.F. Tutikian, et al. 2020. “Rare Earth Elements and Carbon Nanotubes in Coal Mine Around Spontaneous Combustions.” Journal of Cleaner Production 253: 120068. https://doi.org/10.1016/j.jclepro.2020.120068.
- Silva, L.F.O., M. Santosh, M. Schindler, et al. 2021. “Nanoparticles in Fossil and Mineral Fuel Sectors and Their Impact on Environment and Human Health: A Review and Perspective.” Gondwana Research 92: 184–201. https://doi.org/10.1016/j.gr.2020.12.026.
- Singh, A.K., A. Kumar, P.K. Singh, et al. 2018. “Bacterial Desulphurization of low-Rank Coal: A Case Study of Eocene Lignite of Western Rajasthan, India.” Energy Sources, Part A: Recovery, Utilization, and Environmental Effects 40 (10): 1199–1208. doi:10.1080/15567036.2018.1476608.
- Singh, V.K., R. Singh, A. Kumar, et al. 2021. “Chapter 14 – Perspectives in Desulfurization of Coal Using Microbes.” In Microbe Mediated Remediation of Environmental, edited by A. Kumar, V. K. Singh, and P. Singh, et al., 141–155. Contaminants: Woodhead Publishing.
- Singh, A., B. Singh, and O. Ward. 2012. “Potential Applications of Bioprocess Technology in Petroleum Industry.” Biodegradation 23 (6): 865–880. doi:10.1007/s10532-012-9577-2.
- Soleimani, M., A. Bassi, and A. Margaritis. 2007. “Biodesulfurization of Refractory Organic Sulfur Compounds in Fossil Fuels.” Biotechnology Advances 25 (6): 570–596. https://doi.org/10.1016/j.biotechadv.2007.07.003.
- Soltanali, S., Z. Shams Hagani, and M. Pazouki Yaftabadi. 2008. “Economic Evaluation for air Pollution Control Technologies Selection in Power Plants Processes.” International Journal of Environmental Science & Technology 5 (4): 555–564. doi:10.1007/BF03326053.
- Speight, J.G., and N.S. El-Gendy. 2018. “Chapter 6 – Biocatalytic Desulfurization.” In Introduction to Petroleum Biotechnology, edited by J. G. Speight, and N. S. El-Gendy, 165–227. Boston: Gulf Professional Publishing.
- Tang, L., S. Chen, D. Gui, et al. 2020. “Effect of Removal Organic Sulfur from Coal Macromolecular on the Properties of High Organic Sulfur Coal.” Fuel 259: 116264. doi:10.1016/j.fuel.2019.116286.
- Tang, L., S. Wang, X. Zhu, et al. 2018. “Feasibility Study of Reduction Removal of Thiophene Sulfur in Coal.” Fuel 234: 1367–1372. https://doi.org/10.1016/j.fuel.2018.08.016.
- Tangstad, M., J.P. Beukes, J. Steenkamp, et al. 2019. “Chapter 14 – Coal-Based Reducing Agents in Ferroalloys and Silicon Production.” In New Trends in Coal Conversion, edited by I. Suárez-Ruiz, M. A. Diez, and F. Rubiera, 405–438. Duxford: Woodhead Publishing.
- Technologies AP. n.d. Sulfex™ – Desulfurization Simplified Reno, Nevada 89502, USA2018 [cited 2020]. https://www.altpetrol.com/contact/.
- Tripathi, N., R.S. Singh, and C.D. Hills. 2019. “Microbial Removal of Sulphur from Petroleum Coke (Petcoke).” Fuel 235: 1501–1505. https://doi.org/10.1016/j.fuel.2018.08.072.
- United States Department of Energy PETC. 1987. Quaterly Technical Progress Report. Pittsburgh: Pittsburgh Energy Technology Center. p. 183.
- Uzun, D., and S. Özdoğan. 2006. “Sulfur Removal from Original and Acid Treated Lignites by Pyrolysis.” Fuel 85 (3): 315–322. https://doi.org/10.1016/j.fuel.2005.06.012.
- Valentim, B. 2020. “Petrography of Coal Combustion Char: A Review.” Fuel 277: 118271. https://doi.org/10.1016/j.fuel.2020.118271.
- Wackett, L.P. 2000. “Environmental Biotechnology.” Trends in Biotechnology 18 (1): 19–21. https://doi.org/10.1016/S0167-7799(99)01399-2.
- Weills, J.T. 1976. “Impacts of the Coal Fuel Cycle in Power Generation.” In Energy and the Environment Cost-Benefit Analysis: Georgia, Pergamon, edited by R. A. Karam, and K. Z. Morgan, 297–317.
- Wierzchowski, K., B. Białecka, J. Calus Moszko, et al. 2020. “Characterization of Unburned Carbon Separated from Power Plant Slag.” International Journal of Environmental Science and Technology 17 (5): 2499–2510. doi:10.1007/s13762-020-02655-7.
- Wilcox, J., B. Wang, E. Rupp, et al. 2015. “Observations and Assessment of Fly Ashes from High-Sulfur Bituminous Coals and Blends of High-Sulfur Bituminous and Subbituminous Coals: Environmental Processes Recorded at the Macro- and Nanometer Scale.” Energy & Fuels 29 (11): 7168–7177. doi:10.1021/acs.energyfuels.5b02033.
- Wüst, R.A.J., C.R. Ward, R.M. Bustin, et al. 2002. “Characterization and Quantification of Inorganic Constituents of Tropical Peats and Organic-Rich Deposits from Tasek Bera (Peninsular Malaysia): Implications for Coals.” International Journal of Coal Geology 49 (4): 215–249. https://doi.org/10.1016/S0166-5162(01)00064-7.
- Xu, Y., Y. Liu, Y. Bu, et al. 2021. “Review on the Ionic Liquids Affecting the Desulfurization of Coal by Chemical Agents.” Journal of Cleaner Production 284: 124788. https://doi.org/10.1016/j.jclepro.2020.124788.
- Xu, J., X. Liu, C. Song, et al. 2020. “Biodesulfurization of High Sulfur Coal from Shanxi: Optimization of the Desulfurization Parameters of Three Kinds of Bacteria.” Energy Sources, Part A: Recovery, Utilization, and Environmental Effects 42 (18): 2297–2315. doi:10.1080/15567036.2019.1675821.
- Yaman, S., and S. Küçükbayrak. 1997. “Sulfur Removal from Lignite by Oxydesulfurization Using fly ash.” Fuel 76 (1): 73–77. https://doi.org/10.1016/S0016-2361(96)00167-6.
- Yang, J.-K., and S.-F. Chen. 1993. “Chemical Desulfurization of Coal Using Microwave Irradiation.” In Vol. 21 of Coal Science and Technology, edited by B. K. Parekh, and J. G. Groppo, 317–322. Kentucky: Elsevier.
- Yang, L., and X. Ge. 2016. “Chapter 3 – Biogas and Syngas Upgrading.” In Vol. 1 of Advances in Bioenergy, edited by Y. Li, and X. Ge, 125–188. Elsevier.
- Yao, Y., and J. Liu. 2019. “Chapter 2 – Petrophysical Characterization of the Pore Structure of Coal.” In Petrophysical Characterization and Fluids Transport in Unconventional, edited by J. Cai, and X. Hu, 21–36. Reservoirs: Elsevier.
- Ye, J., P. Zhang, G. Zhang, et al. 2018. “Biodesulfurization of High Sulfur Fat Coal with Indigenous and Exotic Microorganisms.” Journal of Cleaner Production 197: 562–575. doi:10.1016/j.jclepro.2018.02.061.
- Yu, X., Z. Luo, and D. Gan. 2019. “Desulfurization of High Sulfur Fine Coal Using a Novel Combined Beneficiation Process.” Fuel 254: 115603. doi:https://doi.org/10.1016/j.fuel.2019.06.011.
- Zhang, J., H. Zhou, D. Liu, et al. 2020. “Chapter 2 – Pretreatment of Lignocellulosic Biomass for Efficient Enzymatic Saccharification of Cellulose.” In Lignocellulosic Biomass to Liquid, edited by A. Yousuf, D. Pirozzi, and F. Sannino, 17–65. Biofuels: Academic Press.
- Zygarlicke, C.J., D.P. McCollor, S.A. Benson, et al. 1992. “Ash Particle Size and Composition Evolution During Combustion of Synthetic Coal and Inorganic Mixtures.” Symposium (International) on Combustion 24 (1): 1171–1177. doi:https://doi.org/10.1016/S0082-0784(06)80138-4.