ABSTRACT
Introduction: Dengue is a prevalent disease in tropical and subtropical countries with an estimated 400 million people infected annually. While significant advancement has been made in the chase for an effective dengue vaccine, the recently licensed Sanofi vaccine was, in contrast to in vitro data, only partially protective.
Areas covered: This suggests that our understanding of the serological correlates for dengue is currently inadequate. With growing evidence supporting the role of fragment crystalizable gamma receptors (FcγRs) in antibody-mediated neutralization or antibody-dependent enhancement (ADE) of dengue virus (DENV) infection, FcγR-expressing cells have been increasingly used for measuring neutralizing antibody responses elicited by dengue vaccines. Here, we review the mechanisms of how FcγRs modulates both DENV neutralization and enhanced infections via its interactions with antibodies.
Expert commentary: This review provides insights on the importance of factoring FcγRs for in vitro neutralization assays. Bridging the gap between in vitro and clinical observations would allow researchers to more accurately predict in vivo vaccine efficacy.
1. Introduction
Dengue is the most common mosquito-borne viral disease, which spreads in over 100 countries and represents a serious threat to global community. The disease results from infection with dengue virus (DENV), which is a single positive-stranded RNA virus that belongs to Flavivirus genus. DENV consists of four antigenically distinct serotypes, namely DENV-1, DENV-2, DENV-3, and DENV-4. While infection with DENV can be asymptomatic, disease manifestation can range from dengue fever (DF) to severe, life-threatening dengue hemorrhagic fever (DHF), or dengue shock syndrome (DSS). Based on the new World Health Organiztion (WHO) classification system, severe dengue is characterized by either severe plasma leakage, severe bleeding, or severe organ involvement [Citation1–Citation4]. Using cartographic approaches, a recent study estimates that DENV infects more than 390 million people every year with 96 million exhibiting clinically apparent diseases [Citation5]. According to estimation by WHO, half a million of severe dengue cases require hospitalizations every year [Citation6].
Human activities such as urbanization, migration, and travelling have resulted in geographical expansion of DENV and its primary mosquito vector, Aedes aegypti. Furthermore, residential environments have been proven to be an effective breeding habitat for the Aedes aegypti as its larvae has been frequently found in common areas such as ant traps, earthenware jars, bowls, tanks, tin cans, and drums [Citation7]. A current promising entomological strategy to combat the spread of mosquito vectors is to release modified Aedes aegypti infected with the intracellular bacterium Wolbachia, which have been shown to have a reduction in its ability to transmit dengue [Citation8]. However, it will take several years post implementation to assess the effectiveness of these methods in disrupting the transmission of DENV. Furthermore, effective vector control can be difficult especially for developing countries as such programs depend on availability of resources and trained personnel for execution [Citation9,Citation10]. Given the challenges with vector control, the development of a safe and potent tetravalent dengue vaccine is necessary to eradicate dengue. Among all the potential dengue vaccines, only Sanofi Pasteur’s tetravalent chimeric yellow-fever dengue (CYD) vaccine passed the phase IIb and phase III vaccine trials. Although the CYD vaccine showed clinical efficacy against DENV-3 and DENV-4, efficacies against DENV-1 and DENV-2 were found to be significantly weaker in the clinical trials [Citation11,Citation12]. Interestingly, these observations are in contrast to preclinical studies in mice and monkeys, where protection against DENV-1 and DENV-2 were observed after administration of the CYD vaccine [Citation13].
The discrepancies observed in the pre-clinical studies and clinical evaluation is still largely unknown. A possibility is that DENV-1 and DENV-2 are genetically more diverse compared to the other DENV serotypes [Citation14]. Another possibility is the lack of a good surrogate of protection, indicating a requirement to better define dengue immune correlates of protection, so as to identify the relevant parameters required for future vaccine potency tests. Besides dengue, the recent Zika outbreak that resulted in an associated increase in Guillain–Barre syndrome (GBS) [Citation15–Citation17] and microcephaly [Citation18,Citation19] also warrants the need of a Zika vaccine that prevents these adverse reactions. The knowledge gained from the understanding of immune correlates of vaccine protection would therefore aid in accelerating development vaccine candidates for dengue and other medically important flaviviruses.
1.1. Antibodies – friend or foe?
Similar to many viruses, the measurement of neutralizing antibody responses is one of the most relevant endpoints when testing for efficacy of a dengue vaccine. However, antibodies against DENV is potentially a double-edge sword. While serotype-specific neutralizing antibodies can confer life-long protection [Citation12], cross-reactive antibodies targeted against the other DENV serotypes only protect for approximately 3–6 months [Citation13]. Moreover, as levels of cross-reactive antibodies wane with time, these antibodies can potentially result in increased pathogenicity. The most widely accepted hypothesis to explain for this phenomenon is the antibody-dependent enhancement (ADE), where sub-neutralizing levels of antibodies can form immune complexes that interact with FcγRs in monocytes, macrophages and dendritic cells, leading to increased uptake and infection [Citation20]. In addition to dengue, ADE has been shown in vitro with other viruses such as influenza virus [Citation21], coxsackievirus B virus [Citation22], respiratory syncytial virus (RSV) [Citation23], ebola virus [Citation24], human immunodeficiency virus (HIV) [Citation25], and zika virus [Citation26]. Despite a wealth of in vitro studies [Citation27–Citation29] and an elegant mice study proving the existence of ADE using maternal transferred antibodies [Citation30], the role of ADE has only been recently authenticated clinically, where cross-reactive antibodies can result in enhanced live-attenuated vaccine infection, resulting in prolonged viraemia [Citation31]. Given the significance of FcγRs in mediating neutralization and ADE, a better understanding of the molecular mechanisms involved in these processes could potentially lead to the development of tools to assess neutralizing and non-neutralizing antibody responses produced by vaccines. This review hence focuses on describing the current knowledge of the key mechanisms involved in antibody-dependent neutralization and enhancement of DENV infection in FcγR-bearing cells, which would be necessary for interpretation of antibody responses following administration of dengue candidate vaccines in humans.
1.2. Antibody responses to dengue virus infection
The viral envelope of DENV consists of three main proteins – envelope (E) membrane (M), and pre-membrane (prM). During infection, the E protein facilitates DENV binding to cellular receptors and mediates intracellular fusion of DENV and host membranes [Citation32]. Following replication, immature virions consisting of E and prM are assembled in the endoplasmic reticulum (ER) and subsequently pass through the trans-golgi network (TGN) [Citation33]. In the acidic environment of the TGN, an endoprotease furin cleaves prM to M [Citation34,Citation35]. Peptide pr then dissociates from the virion upon secretion of the virus. However, cleavage of prM to M is not efficient [Citation36], thus resulting in the secretion of high levels of prM-immature viruses. Although fully immature viruses are unable to cause infection due to its inability to present the E protein for host cell attachment, studies have shown that antibodies targeting prM can rescue their infectious properties via ADE [Citation37]. Thus, potent neutralizing antibodies must prevent ADE by either blocking viral attachment to cells or prevent fusion of viral and host membranes.
The main surface antigen on DENV, the E protein, which consists of three domains (EDI, EDII, and EDIII), is a major target of neutralizing antibodies [Citation38]. Antibodies that target E protein or its quaternary epitopes can neutralize by inhibiting virus attachment or preventing viral uncoating. While humans can produce neutralizing antibodies to epitopes similar to those recognized by murine EDIII antibodies, in a natural primary infection, only a small subset of the antibody repertoire produced presents serotype specificity and potent neutralizing properties [Citation39–Citation41]. Anti-EDIII antibodies, for instance 1H9, 14A4, and 8A1 have been shown to neutralize DENV infections [Citation42–Citation45]. Broadly neutralizing anti-EDIII monoclonal antibodies such as 1A1D-2 can also potently neutralize DENV-1, DENV-2, and DENV-3 at 37°C by inhibiting attachment of DENV to host cells [Citation46]. Furthermore, using the structural framework of EDIII, Robinson et al. developed a unique EDIII potent neutralizing antibody that binds to the epitope–paratope interface on EDIII of all DENV serotypes and potently neutralizes DENV by preventing viral fusion [Citation47], reinforcing the notion that EDIII antibodies can be highly neutralizing. In addition, antibodies that target complex quaternary structures of DENV can be highly neutralizing. For instance, antibodies such as 5J7 and 2D22 that binds across E proteins are highly neutralizing and can neutralize DENV by inhibiting viral fusion [Citation48,Citation49]. In contrast, cross-reactive antibodies that target the EDII fusion peptide are predominantly non-neutralizing [Citation50], and can potentially lead to ADE of DENV and ZIKV infections [Citation26]. Nonetheless, a broadly neutralizing human antibody 1C19 that targets a unique cross-reactive epitope of EDII has been shown to be a potent neutralizing antibody across all four DENV serotypes [Citation51]. Altogether, these studies suggest that although neutralizing monoclonal antibodies have been mapped to all three domains, antibodies that target the EDIII and the complex quaternary epitopes (such as the hinge) are likely to be most neutralizing in humans [Citation39,Citation52–Citation55].
While high levels of EDIII targeting antibodies can be potentially neutralizing, these antibodies would be most suitable for therapeutic use, as EDIII targeting antibodies are only induced at low levels following a natural infection. This is clearly demonstrated in depletion experiments, where studies have shown that depletion of EDIII antibodies did not significantly change the neutralization potency of sera [Citation56,Citation57]. On the other hand, antibodies that target the complex quaternary epitopes are generated following a natural infection and are highly neutralizing. Therefore, besides assessing antibody titers, the epitopes that the human antibodies target should be an important consideration when assessing for neutralizing antibody responses against DENV. With the knowledge that cross-reactive antibodies neutralizes poorly in humans, a method that employs depleting the sera of cross-reactive antibodies with other DENV serotypes before assessing the amount of serotype-specific antibodies present in the sera by ELISA or PRNT will likely be informative in the assessment of the levels of neutralizing antibodies induced by potential dengue vaccine candidates [Citation39,Citation58].
1.3. Determinants of neutralization or enhancement of dengue virus infection
Two models exist to understand the mechanisms of neutralizing antibodies; the (1) ‘single hit’ and (2) ‘multiple hit’ hypothesis. The ‘single hit’ hypothesis posits that a critical neutralization site exists on a virion, thus suggesting that blocking of these functionally important regions will result in neutralization [Citation59]. However, with the diversity of virion structures and the complexity of viral fusion, to expect neutralization with a single binding event is unlikely. The ‘multiple hit’ hypothesis posits that neutralization requires binding of antibodies to the virion with a stoichiometry that exceeds the threshold required for virus neutralization. In this model, both antibody affinity and epitope accessibility are important determinants to assessing the concentrations of antibodies needed to bind and neutralize the virion. Antibody affinity correlates strongly with neutralizing activity. However, affinity alone is insufficient as binding sites must be available for docking of antibodies, as not all epitopes may be accessible for antibody binding. Steric constraints, structural dynamics and changes in virion surface conformation that affects epitope accessibility has also been demonstrated to affect the stoichiometric requirements for DENV neutralization [Citation60,Citation61].
To assess if antibodies meet the stoichiometry requirements for antibody neutralization, plaque reduction neutralization test (PRNT) on baby hamster kidney (BHK-21) or Vero cells are traditionally used. PRNT measures an antibody’s ability to inhibit infection either by blocking virus entry or preventing virus uncoating [Citation62,Citation63]. However, fibroblast and epithelial cells are not the primary targets of dengue infection. Moreover, they do not express FcγRs, which are abundantly expressed in human monocytes; the primary targets of DENV infection [Citation64,Citation65]. Given the role of FcγRs in modulating neutralization and enhancement of DENV immune complexes, stoichiometric requirements for antibody neutralization should be determined in FcγR-bearing cells.
1.4. FcγRs and their role in antibody-dependent uptake of dengue immune complexes
The association of severe disease and ADE was uniquely demonstrated in young infants who developed DHF due to waning concentrations of maternal antibodies to DENV [Citation66,Citation67]. Ventura and colleagues were the first to observe DENV antibodies crossing the placenta barrier either through the placenta via neonatal Fc receptor (FcRn) or through breast-feeding [Citation68]. As neonatal and adult monocytes exhibit similar expression of FcγRI and FcγRIIA [Citation69], DENV opsonized with sub-neutralizing levels of maternal DENV antibodies can result in ADE of dengue infection. T-cells, on the other hand, cannot directly pass through the placenta barrier, suggesting that T-cells are unlikely to be involved in severe disease of young infants. Hence, besides viral factors [Citation70,Citation71], ADE of dengue infection can potentially explain for the epidemiological observations of severe disease in young infants. On the other hand, the contribution of ADE in severe disease in adults is less clear, as cytotoxic T-cells that are partially protective may lead to release of cytokines that leads to enhanced inflammation and tissue damage [Citation72]. Nonetheless, we have clinically demonstrated that the levels of pre-existing cross-reactive antibodies can directly affect vaccine viraemia and subsequent immunogenicity of live-attenuated vaccines [Citation31]. Taken together, these studies emphasize the need to consider the involvement of FcγR when assessing for antibody-mediated neutralization and enhancement of DENV infection.
Monocytes are the FcγR bearing cells that have been shown to be a major reservoir for DENV infection and replication [Citation64,Citation65]. Primates have a complex FcγR system consisting of FcγRI family (FcγRIA, FcγRIB, FcγRIC), FcγRII family (FcγRIIA, FcγRIIBI, FcγRIIBII, FcγRIIBIII, FcγRIIC), and FcγRIII family (FcγRIIIA, FcγRIIIB). Each FcγR subtype has its own distinct properties and thus have different effects on DENV neutralization or enhancement [Citation73]. As the majority of the monocytes express FcγRI, FcγRIIA, and FcγRIIB, these are the main FcγRs that have an impact on antibody-opsonized DENV infection or neutralization (). Kwissa et al. recently suggested that DENV infection induces the expansion of FcγRIII expressing monocytes to stimulate plasmablast differentiation [Citation74]. FcγRIII bearing monocytes can also play an important pro-inflammatory role during disease, stimulating the release of cytokines such as TNFα, CCL2, CCL3, and CCL4 [Citation75]. However, since FcγRIII is only present on 10% of monocytes under healthy conditions and has little impact on DENV neutralization or enhancement [Citation76], this review will focus on FcγRI, FcγRII and their impact on the assessment of neutralizing antibody efficiency against DENV.
Table 1. FcγRs effects on antibody-dependent dengue neutralization and enhancement.
FcγRIA has high affinity to monomeric IgG and immune complexes while FcγRIIA and FcγRIIIA have higher affinity to immune complexes but not monomeric IgG. Both FcγRIA and FcγRIIIA are activating FcγRs and have an accessory immunoreceptor tyrosine-based activation motif (ITAM) bearing gamma-chain for signal transduction. By contrast, FcγRIIA has an ITAM motif in its own cytoplasmic domain, thereby eliminating the need for a gamma chain. Compared to FcγRIIA, FcγRI requires lesser antibodies to neutralize DENV, suggesting that FcγRI plays a more predominant role in DENV neutralization. This could be attributed to its role in internalization of neutralized immune complexes when both FcγRs are expressed [Citation29]. On the other hand, although both activating FcγRs can mediate ADE, FcγRIIA has been shown to be more effective compared to FcγRI [Citation28] ().
The physiological role of activating FcγRs in professional phagocytes is to internalize immune complexes via FcγR-mediated endocytosis or phagocytosis to clear immune complexes in blood circulation [Citation81]. IgG engagement to FcγRs initiates a signaling cascade beginning with the phosphorylation of tyrosines within its ITAM [Citation82]. This results in the recruitment and activation of protein and lipid kinases such as proto-oncogene tyrosine-protein kinase (Src) and spleen tyrosine kinase (Syk) [Citation83]. Members of the Src tyrosine-kinase family (Fyn, Hck, Lyn, Yes, Src), is postulated to be important for early phosphorylation events after initial engagement of FcγRs [Citation84–Citation86] along with increased synthesis of signaling molecules phosphatidylinositol (3,4,5)-trisphosphate (PIP3), phosphatidylinositol 4,5-bisphosphate (PI(4,5)P2) and diacylglycerol (DAG). However, a degree of redundancy is likely present, as Src–/– macrophages exhibited no alternations in phagocytosis. Phosphorylated ITAMs allows for docking, phosphorylation and activation of Syk, which subsequently stimulates multiple signaling cascades such as phosphoinositide 3-kinase (PI3K), phosphoinositide phospholipase C (PLCγ), phospholipase D (PLD), and protein kinase C (PKC) [Citation87,Citation88]. In addition, engagement of FcγRs triggers membrane ruffling and the formation of actin protrusions [Citation89], resulting in FcγR-mediated phagocytosis of immune complexes.
However, whether FcγRs functionally mediates DENV entry or serves only to aid in DENV attachment to the cell surface remains an unsolved question [Citation90]. Using DENV with triple mutations in its E protein, Chotiwan et al. showed that DENV opsonized with monoclonal antibody 4G2 was unable to bypass the viral attachment defect [Citation91]. This suggests that post-FcγR engagement and interactions with other cellular receptors are required for successful entry of the DENV immune complexes [Citation91]. Hence, FcγRs can enhance uptake of DENV immune complexes via FcγR-phagocytosis or improved interaction with DENV receptors ().
Figure 1. Hypothesized mechanisms of extrinsic ADE. (1) DENV immune complexes internalization is mediated by the engagement of activating Fc?Rs (red) on the cell surface triggering the phosphorylation of cytosolic ITAM by Src kinases. This subsequently leads to the phosphorylation of Syk and PI3 K that triggers a signaling cascade for Fc?R-mediated phagocytosis. (2) Alternatively, activating Fc?Rs (red) can concentrate immune complexes on the surface of the cell. The virion is then able to bind to its receptor to enter the cell via receptor-mediated endocytosis. These processes result in increased internalization of the virus, thus resulting in ADE. Full color available online.
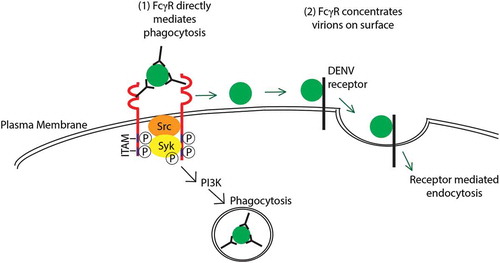
Given the role of activating FcγRs in uptake of immune complexes, potent neutralizing antibodies against DENV must be able to neutralize DENV intracellularly by inhibiting viral fusion. In contrast, non-neutralizing antibodies against DENV would result in enhanced DENV uptake via activating FcγRs and intracellular fusion, thus leading to ADE. Through mechanisms that are largely unclear, these virions bound by sub-neutralizing concentrations of antibodies are able to evade degradation in the phagolysosme and are unable to prevent E-protein mediated fusion of the virion. This allows the release of its genome in the host cytosol and subsequently establishes a productive infection. Indeed, we have previously shown that weakly cross-reactive antibodies can only neutralize at concentrations high enough to form viral aggregates that co-ligate the lowly expressed inhibitory Fc-receptor, FcγRIIB [Citation78]. FcγRIIB has an immunoreceptor tyrosine-based inhibition motif (ITIM), which can recruit and activate phosphatases Src homology region 2 domain containing phosphatase-1 (SHP-1) and phosphatidylinositol-3,4,5-trisphosphate 5-phosphatase 1 (SHIP-1) to shut down signaling mediated by activating FcγRs, thereby inhibiting FcγR-mediated phagocytosis [Citation78,Citation92]. As the mode of neutralization mediated by serotype-specific and cross-reactive antibodies differs fundamentally in FcγR-expressing cells monocytes, these cells can be used to assess the type of antibodies that are induced by DENV. Indeed, using 30 blinded convalescent sera, Wu et al. observed that this method correctly identifies DENV serotype of infection in 93.3% of the cases as compared to 76.7% with PRNT [Citation79]. These studies support that neutralizing antibodies against DENV would be able to inhibit viral fusion even in the presence of FcγR-mediated phagocytosis and this can potentially be used to assess antibody responses triggered by future dengue vaccines.
1.5. FcγRs and their role in modification of antiviral pathways
Activating FcγR signaling is known to induce interferon-stimulated genes (ISGs) production unless co-ligated with an inhibitory receptor such as FcγRIIB [Citation93]. However, under ADE conditions, antibodies are not present at sufficiently high levels to form immune complex aggregates that facilitates co-ligation of FcγRIIB [Citation78]. For ADE of DENV infection to occur, we have recently elucidated that DENV immune complexes can co-ligate the inhibitory receptor leukocyte-immunoglobulin like receptor B1 (LILRB1) that activates phosphatase SHP-1 to downregulate antiviral responses triggered by Syk phosphorylation from activating FcγR signaling [Citation27] ().
Figure 2. Hypothesized mechanisms of intrinsic ADE. Immune complexes engage activating Fc?R (red) that results in the phosphorylation of Syk. This subsequently leads to the activation of STAT1 that drives the transcription of interferon-stimulated genes (ISG). However, co-ligation of LILRB1 mediated by DENV immune complexes under ADE conditions can reduce ISG responses by phosphorylation of SHP-1 that attenuates Syk and STAT-1 signaling. At longer time periods, co-ligation of Fc-gamma receptor can also signal for IL-10 induction that inhibit IFN and subsequent STAT-1 and ISG induction. Besides intracellular signaling for evasion of host immune responses, virus fusion activity can be increased under ADE conditions. Together, these intrinsic pathways lead to suppression of the anti-viral responses thus resulting in enhanced DENV infection. Full color available online.
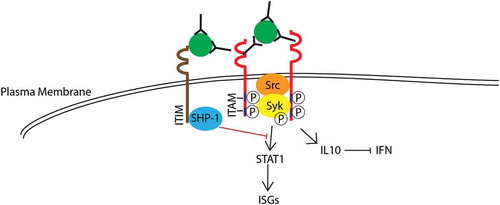
Furthermore, as postulated by Halstead et al., after Fc-receptor mediated phagocytosis [Citation94], DENV immune complexes is thought to induce innate immune responses suppression by driving a bias toward a T-helper-2 (Th2) response, repress IL12, IFNγ, and TNF-α [Citation95,Citation96] and induce IL10 [Citation97] and suppressor of cytokine signaling 1 (SOCS-1) [Citation98], thus leading to increased production of infectious particles in infected cells (). Other downstream intracellular signaling, including signal transducer and activator of transcription 1 (STAT-1) and interferon regulatory factor 1 (IRF1) have also been observed to be suppressed after infection with DENV opsonized with antibodies [Citation96], creating an immunosuppressive intracellular environment that is considered favorable for DENV replication. However, it is important to note that in human primary monocytes, suppression of IFN under ADE conditions is not observed [Citation99], suggesting that there could be involvement of other intrinsic factors that accounts for the increased DENV replication observed during ADE. Interestingly, a recent study by Flipse et al. showed using primary human macrophages that entry of DENV immune complexes does not affect binding, internalization, or immunosuppressive signaling but instead, appeared to have enhanced fusion activity per cell, leading to increased viral progeny production [Citation100]. While the exact mechanism remains to be determined, it is likely that antibody-opsonized DENV traffics DENV into cellular compartments different from DENV only infection. Altogether, these studies suggest that the success of ADE infection relies on the immune complexes ability to increase binding, internalization, fusion and evasion of host cell immune responses during the early course of infection. Future dengue vaccine candidates should hence aim to induce neutralizing antibody responses that would inhibit DENV fusion, thereby circumventing DENV replicate even if the immune complexes are internalized via FcγR-mediated phagocytosis.
1.6. Considerations when assessing antibody responses in fcγR-bearing cells
There are many FcγR-bearing cell lines that can be used to assess neutralization and ADE of DENV infection. However, of the FcγR-bearing cell lines available, THP-1, K562 and U937 are most commonly used. Others have also used HEK-293, COS or BHK-21 cell lines transfected with FcγRs required for ADE. For example, Moi et al. used 18 secondary dengue patients’ sera and compared PRNT results in both FcγR-negative BHK-21 cells and FcγR-positive BHK-21 cells. Neutralization titers obtained using FcγR-negative BHK-21 cells were found to be significantly higher than BHK-21 cells that over-express of FcγR [Citation101]. However, the limitation to using transfected cells is the differences in the expression of activating and inhibitory FcγRs in these cells compared to monocytes, which may cause differences in the stoichiometric requirement for DENV neutralization (). To potentially reduce these variabilities, FcγR-bearing monocytic cell lines can be used to assess neutralization (). In such cases, the important consideration when using these cell lines are the types and the expression levels of FcγRs that are present in these cell lines, which could influence outcome of DENV neutralization or enhancement. For instance, as K562 is FcγRI deficient, the stoichiometric requirement required for DENV neutralization in K562 is fourfold higher than what is obtained for THP-1, where both FcγRI and FcγRII are present [Citation29]. In our experiment, we have observed that antibody neutralization titer observed in THP-1 closely mimics the antibody titers in primary monocytes. However, due to heterogeneity in cancer cell lines, it is always recommended to validate results obtained from cell lines with primary monocytes.
Table 2. FcγR-expressing cell lines commonly used to assess antibody-dependent enhancement and neutralization of dengue infection.
Although human primary monocytes are known to express FcγRs, the single-nucleotide polymorphism (SNPs) and expression of FcγRs can influence outcome of antibody-mediated neutralization. Therefore, detection of FcγR SNPs in human monocytes is recommended for accurate interpretation of neutralization titers. An important consideration is the SNPs present in FcγRIIA. Common single-nucleotide polymorphisms in FcγRIIA gene at position 131 (rs1801274) changes the amino acid at position 131 (His131Arg). This site is polymorphic for binding of human IgG2 but not IgG1, with H131 form of FcγRIIA the only class of FcγRs that interacts well with IgG2. Thus His131Arg potentially leads to significant reduction in binding to IgG, which could potentially account for the reduced ADE and reduced risk of dengue severity associated with this polymorphism [Citation103,Citation104]. The frequency of rs1801274 varies between ethnic groups as Osborne et al. found that Japanese and Chinese have an increased frequency of H131 as compared to Caucasians [Citation105]. Polymerase chain reaction with confronting two-pair primers method can be used to access polymorphism of the receptors [Citation27].
Expression of FcγRs in primary monocytes can vary between individuals. For instance, differential levels of cytokines can influence FcγR expression. FcγRI expression has been shown to be upregulated by interferon γ (IFNγ) which can affect stoichiometry required for DENV neutralization [Citation73,Citation106,Citation107]. In contrast, interleukin-4, interleukin-10 and transforming growth-factor-B (TGB-B) downregulate activating FcγRs but enhances the inhibitory FcγRIIB [Citation108,Citation109] which can potentially impact on the neutralization of immune complexes mediated by cross-reactive antibodies [Citation102]. Expression of FcγRs can also vary between ethnic and age groups. It has been previously shown that groups of African descents have increased expression of FcγRI [Citation110]. Seidler et al. suggest that monocytes subsets changes considerably with age, with CD14+CD16+ subset increasing with age [Citation111]. Therefore, for consistency, we should take into consideration FcγR SNPs and expression when accessing the stoichiometric requirements for DENV neutralization and enhancement in human monocytes. Alternatively, primary monocytes obtained from different donors can be used to reduce the effects from these confounding factors.
2. Conclusion
Defining correlates of protection induced by dengue vaccines can be potentially challenging. However, it is crucial that antibody responses elicited by dengue vaccines should neutralize all four DENV serotypes without increasing the risk of ADE, which highlights the relevance of using FcγR-bearing cells for the assessment of humoral responses generated by these vaccines.
3. Expert commentary
The inconsistencies observed clinically compared to in vitro data has highlighted the necessity for assays that reliably predicts either immunity or the increased susceptibility to severe disease. Given the different neutralization efficiency mediated by different types of antibodies, it is essential to develop neutralization assays that can differentiate between serotype-specific antibodies that result in long-term protection from cross-reactive antibodies that can cause ADE of dengue infection, for accurate assessment of protective immune responses elicited by dengue vaccines. With the current knowledge of the role of FcγRs in neutralization and enhancement of DENV, it has become increasingly clear that traditional methods of measuring DENV neutralization and protective antibodies are inadequate in predicting vaccine efficacy. We hence reason that neutralizing antibody titers obtained from FcγR-expressing cells will likely be more indicative of protection in humans.
4. Five-year view
Neutralizing antibody responses depend on multiple parameters, including antibody titer, antibody avidity index, and antibody–antigen stoichiometry. In addition, with increasing evidence clarifying the importance of FcγRs in DENV neutralization and enhancement, antibody assays that reliably assess the neutralization capacity of antibodies in FcγR-expressing cells will be instrumental to determine the level of protection elicited by future candidate dengue vaccines.
Key issues
Dengue is disease of global significance. The current Sanofi vaccine provides only partial protection against DENV-1 and DENV-2.
Traditional methods of assessing immunogenicity do not accurately predict in vivo protection.
Antibodies targeting EDIII and the complex quaternary epitopes (such as the hinge) of DENV are neutralizing, as compared to anti-EDII antibodies that target near the fusion loop.
FcγRs are essential for antibody-mediated DENV neutralization and ADE of DENV infection
The type and levels of FcγRs expressed affect the stoichiometric requirement of DENV neutralization Neutralizing antibodies inhibit DENV infections by preventing virus fusion.
Non-neutralizing antibodies enhance DENV infection by increasing virus entry, fusion and evasion of host immune responses.
Neutralizing antibody responses determined in FcγR-expressing cells will likely be more indicative of vaccine protection than in traditional assays, which uses epithelial cells.
Declaration of interest
The authors have no relevant affiliations or financial involvement with any organization or entity with a financial interest in or financial conflict with the subject matter or materials discussed in the manuscript. This includes employment, consultancies, honoraria, stock ownership or options, expert testimony, grants or patents received or pending, or royalties.
Additional information
Funding
References
- Kabra SK, Jain Y, Singhal T, et al. Dengue hemorrhagic fever: clinical manifestations and management. Indian J Pediatr. 1999;66(1):93–101.
- Kalayanarooj S. Clinical manifestations and management of dengue/DHF/DSS. Trop Med Health. 2011;39(4 Suppl):83–87.
- Gubler DJ. Dengue and dengue hemorrhagic fever. Clin Microbiol Rev. 1998;11(3):480–496.
- Dietz V, Gubler DJ, Ortiz S, et al. The 1986 dengue and dengue hemorrhagic fever epidemic in Puerto Rico: epidemiologic and clinical observations. P R Health Sci J. 1996;15(3):201–210.
- Bhatt S, Gething PW, Brady OJ, et al. The global distribution and burden of dengue. Nature. 2013;496(7446):504–507.
- World Health Organization. Prevention and control of dengue and dengue haemorrhagic fever: comprehensive guidelines. SEARO technical publication series. World Health Organization; 2011.
- Aedes albopictus (Skuse) in Singapore City, Chan YC, Ho BC, Chan KL, et al. 5. Observations in relation to dengue haemorrhagic fever. Bull World Health Organ. 1971;44(5):651–657.
- Walker T, Johnson PH, Moreira LA, et al. The wMel Wolbachia strain blocks dengue and invades caged Aedes aegypti populations. Nature. 2011;476(7361):450–453.
- Erlanger TE, Keiser J, Utzinger J. Effect of dengue vector control interventions on entomological parameters in developing countries: a systematic review and meta-analysis. Med Vet Entomol. 2008;22(3):203–221.
- Horstick O, Runge-Ranzinger S, Nathan MB, et al. Dengue vector-control services: how do they work? A systematic literature review and country case studies. Trans R Soc Trop Med Hyg. 2010;104(6):379–386.
- Capeding MR, Tran NH, Hadinegoro S. Clinical efficacy and safety of a novel tetravalent dengue vaccine in healthy children in Asia: a phase 3, randomised, observer-masked, placebo-controlled trial. The Lancet. 2014; 384:1358-1365.
- Sabchareon A, Wallace D, Sirivichayakul C, et al. Protective efficacy of the recombinant, live-attenuated, CYD tetravalent dengue vaccine in Thai schoolchildren: a randomised, controlled phase 2b trial. The Lancet. 2012;380(9853):1559–1567.
- Guy B, Lang J, Saville M, et al. Vaccination against dengue: challenges and current developments. Annu Rev Med. 2016;67(1):387–404.
- Pyke AT, Moore PR, Taylor CT, et al. Highly divergent dengue virus type 1 genotype sets a new distance record. Scientific …. 2016;6:22356.
- Ioos S, Mallet H-P, Leparc Goffart I, et al. Current Zika virus epidemiology and recent epidemics. Med Mal Infect. 2014;44(7):302–307.
- Santos Dos T, Rodriguez A, Almiron M, et al. Zika Virus and the Guillain–Barré Syndrome — Case Series from Seven Countries. N Engl J Med. 2016;375:1598–1601.
- Arias A, Torres-Tobar L, Hernández G, et al. Guillain-Barré syndrome in patients with a recent history of Zika in Cúcuta, Colombia: A descriptive case series of 19 patients from December 2015 to March 2016. J Crit Care. 2016;37:19–23.
- Mlakar J, Korva M, Tul N, et al. Zika virus associated with microcephaly. N Engl J Med. 2016;374(10):951–958.
- Štrafela P, Vizjak A, Mraz J, et al. Zika virus-associated micrencephaly: a thorough description of neuropathologic findings in the fetal central nervous system. Arch Pathol Lab Med. 2016;arpa.2016–0341–SA.
- Halstead SB, O’Rourke EJ. Antibody-enhanced dengue virus infection in primate leukocytes. Nature. 1977;265(5596):739–741.
- Tamura M, Webster RG, Ennis FA. Subtype cross-reactive, infection-enhancing antibody responses to influenza A viruses. J Virol. 1994;68(6):3499–3504.
- Sauter P, Hober D. Mechanisms and results of the antibody-dependent enhancement of viral infections and role in the pathogenesis of coxsackievirus B-induced diseases. Microbes Infect. 2009;11(4):443–451.
- Krilov LR, Anderson LJ, Marcoux L, et al. Antibody-mediated enhancement of respiratory syncytial virus infection in two monocyte/macrophage cell lines. J Infect Dis. 1989;160(5):777–782.
- Takada A, Feldmann H, Ksiazek TG, et al. Antibody-dependent enhancement of Ebola virus infection. J Virol. 2003;77(13):7539–7544.
- Willey S, Aasa-Chapman MMI, O’Farrell S, et al. Extensive complement-dependent enhancement of HIV-1 by autologous non-neutralising antibodies at early stages of infection. Retrovirology. 2011;8(1):16.
- Dejnirattisai W, Supasa P, Wongwiwat W, et al. Dengue virus sero-cross-reactivity drives antibody-dependent enhancement of infection with zika virus. Nat Immunol. 2016;17(9):1102–1108.
- Chan KR, Ong EZ, Tan HC, et al. Leukocyte immunoglobulin-like receptor B1 is critical for antibody-dependent dengue. Proc Natl Acad Sci U S A. 2014;111(7):2722–2727.
- Rodrigo WWSI, Jin X, Blackley SD, et al. Differential enhancement of dengue virus immune complex infectivity mediated by signaling-competent and signaling-incompetent human Fcgamma RIA (CD64) or FcgammaRIIA (CD32). J Virol. 2006;80(20):10128–10138.
- Chawla T, Chan KR, Zhang SL, et al. Dengue virus neutralization in cells expressing Fc gamma receptors. PLoS ONE. 2013;8(5):e65231.
- Ng JKW, Zhang SL, Tan HC, et al. First experimental in vivo model of enhanced dengue disease severity through maternally acquired heterotypic dengue antibodies. PLoS Pathog. 2014;10(4):e1004031.
- Chan KR, Wang X, Saron WAA, et al. Cross-reactive antibodies enhance live attenuated virus infection for increased immunogenicity. Nat Microbiol. 2016;1:16164.
- Perera R, Kuhn RJ. Structural proteomics of dengue virus. Curr Opin Microbiol. 2008;11(4):369–377.
- Mukhopadhyay S, Kuhn RJ, Rossmann MG. A structural perspective of the flavivirus life cycle. Nat Rev Microbiol. 2005;3(1):13–22.
- Stadler K, Allison SL, Schalich J, et al. Proteolytic activation of tick-borne encephalitis virus by furin. J Virol. 1997;71(11):8475–8481.
- Li L, Lok SM, Yu I-M, et al. The flavivirus precursor membrane-envelope protein complex: structure and maturation. Science. 2008;319(5871):1830–1834.
- Junjhon J, Edwards TJ, Utaipat U, et al. Influence of pr-M cleavage on the heterogeneity of extracellular dengue virus particles. J Virol. 2010;84(16):8353–8358.
- Richter MKS, Da Silva Voorham JM, Torres Pedraza S, et al. Immature dengue virus is infectious in human immature dendritic cells via interaction with the receptor molecule DC-SIGN. PLoS ONE. 2014;9(6):e98785.
- Modis Y, Ogata S, Clements D, et al. A ligand-binding pocket in the dengue virus envelope glycoprotein. Pnas. 2003;100(12):6986–6991.
- De Alwis R, Smith SA, Olivarez NP, et al. Identification of human neutralizing antibodies that bind to complex epitopes on dengue virions. Proc Natl Acad Sci U S A. 2012;109(19):7439–7444.
- De Alwis R, Beltramello M, Messer WB, et al. In-depth analysis of the antibody response of individuals exposed to primary dengue virus infection. PLoS Negl Trop Dis. 2011;5(6):e1188.
- Smith SA, De Alwis AR, Kose N, et al. Isolation of dengue virus-specific memory B cells with live virus antigen from human subjects following natural infection reveals the presence of diverse novel functional groups of antibody clones. J Virol. 2014;88(21):12233–12241.
- Zhou Y, Austin SK, Fremont DH, et al. The mechanism of differential neutralization of dengue serotype 3 strains by monoclonal antibody 8A1. Virology. 2013;439(1):57–64.
- Wahala WMPB, Donaldson EF, De Alwis R, et al. Natural strain variation and antibody neutralization of dengue serotype 3 viruses. PLoS Pathog. 2010;6(3):e1000821.
- Serafin IL, Aaskov JG. Identification of epitopes on the envelope (E) protein of dengue 2 and dengue 3 viruses using monoclonal antibodies. Arch Virol. 2001;146(12):2469–2479.
- Matsui K, Gromowski GD, Li L, et al. Characterization of a dengue type-specific epitope on dengue 3 virus envelope protein domain III. J Gen Virol. 2010;91(Pt 9):2249–2253.
- Lok SM, Kostyuchenko V, Nybakken GE, et al. Binding of a neutralizing antibody to dengue virus alters the arrangement of surface glycoproteins. Nat Struct Mol Biol. 2008;15(3):312–317.
- Robinson LN, Tharakaraman K, Rowley KJ, et al. Structure-guided design of an anti-dengue antibody directed to a non-immunodominant epitope. Cell. 2015;162(3):493–504.
- Fibriansah G, Tan JL, Smith SA, et al. A highly potent human antibody neutralizes dengue virus serotype 3 by binding across three surface proteins. Nat Commun. 2015;6:6341.
- Fibriansah G, Ibarra KD, Ng T-S, et al. DENGUE VIRUS. Cryo-EM structure of an antibody that neutralizes dengue virus type 2 by locking E protein dimers. Science. 2015;349(6243):88–91.
- Crill WD, Chang G-J-J. Localization and characterization of flavivirus envelope glycoprotein cross-reactive epitopes. J Virol. 2004;78(24):13975–13986.
- Smith SA, De Alwis AR, Kose N, et al. The potent and broadly neutralizing human dengue virus-specific monoclonal antibody 1C19 reveals a unique cross-reactive epitope on the bc loop of domain II of the envelope protein. MBio. 2013;4(6):e00873–13.
- Gromowski GD, Barrett ADT. Characterization of an antigenic site that contains a dominant, type-specific neutralization determinant on the envelope protein domain III (ED3) of dengue 2 virus. Virology. 2007;366(2):349–360.
- Sukupolvi-Petty S, Austin SK, Purtha WE, et al. Type- and subcomplex-specific neutralizing antibodies against domain III of dengue virus type 2 envelope protein recognize adjacent epitopes. J Virol. 2007;81(23):12816–12826.
- Shrestha B, Brien JD, Sukupolvi-Petty S, et al. The development of therapeutic antibodies that neutralize homologous and heterologous genotypes of dengue virus type 1. PLoS Pathog. 2010;6(4):e1000823.
- Teoh EP, Kukkaro P, Teo EW, et al. The structural basis for serotype-specific neutralization of dengue virus by a human antibody. Sci Transl Med. 2012;4(139):139ra83–139ra83.
- Wahala WMPB, Kraus AA, Haymore LB, et al. Dengue virus neutralization by human immune sera: role of envelope protein domain III-reactive antibody. Virology. 2009;392(1):103–113.
- Williams KL, Wahala WMPB, Orozco S, et al. Antibodies targeting dengue virus envelope domain III are not required for serotype-specific protection or prevention of enhancement in vivo. Virology. 2012;429(1):12–20.
- Wahala WMPB, Silva AM de. The human antibody response to dengue virus infection. Viruses. 2011;3(12):2374–2395.
- Mandel B. Neutralization of poliovirus: a hypothesis to explain the mechanism and the one-hit character of the neutralization reaction. Virology. 1976;69(2):500–510.
- Diamond MS, Pierson TC, Fremont DH. The structural immunology of antibody protection against West Nile virus. Immunol Rev. 2008;225(1):212–225.
- Dowd KA, Pierson TC. Antibody-mediated neutralization of flaviviruses: a reductionist view. Virology. 2011;411(2):306–315.
- Putnak JR, La Barrera De R, Burgess T, et al. Comparative evaluation of three assays for measurement of dengue virus neutralizing antibodies. Am J Trop Med Hyg. 2008;79(1):115–122.
- Roehrig JT, Hombach J, Barrett ADT. Guidelines for plaque-reduction neutralization testing of human antibodies to dengue viruses. Viral Immunol. 2008;21(2):123–132.
- Kou Z, Quinn M, Chen H, et al. Monocytes, but not T or B cells, are the principal target cells for dengue virus (DV) infection among human peripheral blood mononuclear cells. J Med Virol. 2008;80(1):134–146.
- Pham AM, Langlois RA, TenOever BR. Replication in cells of hematopoietic origin is necessary for dengue virus dissemination. PLoS Pathog. 2012;8(1):e1002465.
- Simmons CP, Chau TNB, Thuy TT, et al. Maternal antibody and viral factors in the pathogenesis of dengue virus in infants. J Infect Dis. 2007;196(3):416–424.
- Perret C, Chanthavanich P, Pengsaa K, et al. Dengue infection during pregnancy and transplacental antibody transfer in Thai mothers. J Infect. 2005;51(4):287–293.
- Ventura AK, Ehrenkranz NJ, Rosenthal D. Placental passage of antibodies to dengue virus in persons living in a region of hyperendemic dengue virus infection. J Infect Dis. 1975;131(Suppl):S62–8.
- Maeda M, Van SCHIE RCAA, Yüksel B, et al. Differential expression of Fc receptors for IgG by monocytes and granulocytes from neonates and adults. Clin Exp Immunol. 1996;103(2):343–347.
- Manokaran G, Finol E, Wang C, et al. Dengue subgenomic RNA binds TRIM25 to inhibit interferon expression for epidemiological fitness. Science. 2015;350(6257):217–221.
- Pozo-Aguilar JO, Monroy-Martínez V, Díaz D, et al. Evaluation of host and viral factors associated with severe dengue based on the 2009 WHO classification. Parasit Vectors. 2014;7(1):243.
- Duangchinda T, Dejnirattisai W, Vasanawathana S, et al. Immunodominant T-cell responses to dengue virus NS3 are associated with DHF. Proc Natl Acad Sci U S A. 2010;107(39):16922–16927.
- Chan KR, Ong EZ, Ooi EE. Therapeutic antibodies as a treatment option for dengue fever. Expert Rev Anti Infect Ther. 2013;11(11):1147–1157.
- Kwissa M, Nakaya HI, Onlamoon N, et al. Dengue virus infection induces expansion of a CD14(+)CD16(+) monocyte population that stimulates plasmablast differentiation. Cell Host Microbe. 2014;16(1):115–127.
- Wong KL, Chen W, Balakrishnan T, et al. Susceptibility and response of human blood monocyte subsets to primary dengue virus infection. PLoS ONE. 2012;7(5):e36435.
- Van De Winkel JG, Anderson CL. Biology of human immunoglobulin G Fc receptors. J Leukoc Biol. 1991;49(5):511–524.
- Rodrigo WWSI, Block OKT, Lane C, et al. Dengue virus neutralization is modulated by IgG antibody subclass and Fcgamma receptor subtype. Virology. 2009;394(2):175–182.
- Chan KR, Zhang SL-X, Tan HC, et al. Ligation of Fc gamma receptor IIB inhibits antibody-dependent enhancement of dengue virus infection. Proc Natl Acad Sci U S A. 2011;108(30):12479–12484.
- Wu RSL, Chan KR, Tan HC, et al. Neutralization of dengue virus in the presence of Fc receptor-mediated phagocytosis distinguishes serotype-specific from cross-neutralizing antibodies. Antiviral research. 2012;96(3):340–343.
- Boonnak K, Slike BM, Donofrio GC, et al. Human FcγRII cytoplasmic domains differentially influence antibody-mediated dengue virus infection. J Immunol. 2013;190(11):5659–5665.
- Amigorena S, Bonnerot C. Fc receptor signaling and trafficking: a connection for antigen processing. Immunol Rev. 1999;172:279–284.
- Isakov N. Immunoreceptor tyrosine-based activation motif (ITAM), a unique module linking antigen and Fc receptors to their signaling cascades. J Leukoc Biol. 1997;61(1):6–16.
- Lowell CA. Src-family and Syk kinases in activating and inhibitory pathways in innate immune cells: signaling cross talk. Cold Spring Harb Perspect Biol. 2011;3(3):a002352–a002352.
- Ibarrola I, Vossebeld PJ, Homburg CH, et al. Influence of tyrosine phosphorylation on protein interaction with FcgammaRIIa. Biochim Biophys Acta. 1997;1357(3):348–358.
- Ezumi Y, Shindoh K, Tsuji M, et al. Physical and functional association of the Src family kinases Fyn and Lyn with the collagen receptor glycoprotein VI-Fc receptor gamma chain complex on human platelets. J Exp Med. 1998;188(2):267–276.
- Ghazizadeh S, Bolen JB, Fleit HB. Physical and functional association of Src-related protein tyrosine kinases with Fc gamma RII in monocytic THP-1 cells. J Biol Chem. 1994;269(12):8878–8884.
- Melendez AJ, Harnett MM, Allen JM. FcγRI activation of phospholipase Cγ1 and protein kinase C in dibutyryl cAMP-differentiated U937 cells is dependent solely on the tyrosine-kinase activated form of phosphatidylinositol-3-kinase. Immunology. 1999;98(1):1–8.
- Rankin BM, Yocum SA, Mittler RS, et al. Stimulation of tyrosine phosphorylation and calcium mobilization by Fc gamma receptor cross-linking. Regulation by the phosphotyrosine phosphatase CD45. J Immunol. 1993;150(2):605–616.
- Ayala-Nunez NV, Hoornweg TE, Van De Pol DPI, et al. How antibodies alter the cell entry pathway of dengue virus particles in macrophages. Sci Rep. 2016;6:28768.
- Mady BJ, Erbe DV, Kurane I, et al. Antibody-dependent enhancement of dengue virus infection mediated by bispecific antibodies against cell surface molecules other than Fc gamma receptors. J Immunol. 1991;147(9):3139–3144.
- Chotiwan N, Roehrig JT, Schlesinger JJ, et al. Molecular determinants of dengue virus 2 envelope protein important for virus entry in FcγRIIA-mediated antibody-dependent enhancement of infection. Virology. 2014;456-457:238–246.
- Huang Z-Y, Hunter S, Kim M-K, et al. The effect of phosphatases SHP-1 and SHIP-1 on signaling by the ITIM- and ITAM-containing Fcgamma receptors FcgammaRIIB and FcgammaRIIA. J Leukoc Biol. 2003;73(6):823–829.
- Dhodapkar KM, Banerjee D, Connolly J, et al. Selective blockade of the inhibitory Fcgamma receptor (FcgammaRIIB) in human dendritic cells and monocytes induces a type I interferon response program. J Exp Med. 2007;204(6):1359–1369.
- Halstead SB, Mahalingam S, Marovich MA, et al. Intrinsic antibody-dependent enhancement of microbial infection in macrophages: disease regulation by immune complexes. Lancet Infect Dis. 2010;10(10):712–722.
- Yang KD, Yeh WT, Yang MY, et al. Antibody-dependent enhancement of heterotypic dengue infections involved in suppression of IFNgamma production. J Med Virol. 2001;63(2):150–157.
- Chareonsirisuthigul T, Kalayanarooj S, Ubol S. Dengue virus (DENV) antibody-dependent enhancement of infection upregulates the production of anti-inflammatory cytokines, but suppresses anti-DENV free radical and pro-inflammatory cytokine production, in THP-1 cells. J Gen Virol. 2007;88(Pt 2):365–375.
- Tsai -T-T, Chuang Y-J, Lin Y-S, et al. Antibody-dependent enhancement infection facilitates dengue virus-regulated signaling of IL-10 production in monocytes. PLoS Negl Trop Dis. 2014;8(11):e3320.
- Ubol S, Phuklia W, Kalayanarooj S, et al. Mechanisms of immune evasion induced by a complex of dengue virus and preexisting enhancing antibodies. J Infect Dis. 2010;201(6):923–935.
- Kou Z, Lim JYH, Beltramello M, et al. Human antibodies against dengue enhance dengue viral infectivity without suppressing type I interferon secretion in primary human monocytes. Virology. 2011;410(1):240–247.
- Flipse J, Diosa-Toro MA, Hoornweg TE, et al. Antibody-dependent enhancement of dengue virus infection in primary human macrophages; balancing higher fusion against antiviral responses. Sci Rep. 2016;6:29201.
- Moi ML, Lim C-K, Chua KB, et al. Dengue virus infection-enhancing activity in serum samples with neutralizing activity as determined by using FcγR-expressing cells. PLoS Negl Trop Dis. 2012;6(2):e1536.
- Tridandapani S, Siefker K, Teillaud J-L, et al. Regulated expression and inhibitory function of Fcgamma RIIb in human monocytic cells. J Biol Chem. 2002;277(7):5082–5089.
- García G, Sierra B, Pérez AB, et al. Asymptomatic dengue infection in a cuban population confirms the protective role of the RR variant of the FcgammaRIIa polymorphism. Am J Trop Med Hyg. 2010;82(6):1153–1156.
- Noecker CA, Amaya-Larios IY, Galeana-Hernández M, et al. Contrasting associations of polymorphisms in FcγRIIa and DC-SIGN with the clinical presentation of dengue infection in a Mexican population. Acta Trop. 2014;138:15–22.
- Osborne JM, Chacko GW, Brandt JT, et al. Ethnic variation in frequency of an allelic polymorphism of human Fc gamma RIIA determined with allele specific oligonucleotide probes. J Immunol Methods. 1994;173(2):207–217.
- Chan KR, Ong EZ, Mok DZ, et al. Fc receptors and their influence on efficacy of therapeutic antibodies for treatment of viral diseases. Expert Rev Anti Infect Ther. 2015;13(11):1351–1360.
- Sittisombut N, Maneekarn N, Kanjanahaluethai A, et al. Lack of augmenting effect of interferon-gamma on dengue virus multiplication in human peripheral blood monocytes. J Med Virol. 1995;45(1):43–49.
- Pricop L, Redecha P, Teillaud JL, et al. Differential modulation of stimulatory and inhibitory Fc gamma receptors on human monocytes by Th1 and Th2 cytokines. J Immunol. 2001;166(1):531–537.
- Tridandapani S, Wardrop R, Baran CP, et al. TGF-beta 1 suppresses [correction of supresses] myeloid Fc gamma receptor function by regulating the expression and function of the common gamma-subunit. J Immunol. 2003;170(9):4572–4577.
- Moxey-Mims MM, Frank MM, Lin EY, et al. Increased expression of Fc gamma RI on isolated PMN from individuals of African descent. Clin Immunol Immunopathol. 1993;69(1):117–121.
- Seidler S, Zimmermann HW, Bartneck M, et al. Age-dependent alterations of monocyte subsets and monocyte-related chemokine pathways in healthy adults. BMC Immunol. 2010;11(1):30.