ABSTRACT
Introduction
Moxidectin is a milbemycin endectocide recently approved for the treatment of human onchocerciasis. Onchocerciasis, earmarked for elimination of transmission, is a filarial infection endemic in Africa, Yemen, and the Amazonian focus straddling Venezuela and Brazil. Concerns over whether the predominant treatment strategy (yearly mass drug administration (MDA) of ivermectin) is sufficient to achieve elimination in all endemic foci have refocussed attention upon alternative treatments. Moxidectin’s stronger and longer microfilarial suppression compared to ivermectin in both phase II and III clinical trials indicates its potential as a novel powerful drug for onchocerciasis elimination.
Areas covered
This work summarizes the chemistry and pharmacology of moxidectin, reviews the phase II and III clinical trials evidence on tolerability, safety, and efficacy of moxidectin versus ivermectin, and discusses the implications of moxidectin’s current regulatory status.
Expert opinion
Moxidectin’s superior clinical performance has the potential to substantially reduce times to elimination compared to ivermectin. If donated, moxidectin could mitigate the additional programmatic costs of biannual ivermectin distribution because, unlike other alternatives, it can use the existing community-directed treatment infrastructure. A pediatric indication (for children <12 years) and determination of its usefulness in onchocerciasis–loiasis co-endemic areas will greatly help fulfill the potential of moxidectin for the treatment and elimination of onchocerciasis.
1. Introduction
Human onchocerciasis is a neglected tropical disease (NTD) caused by the filarial nematode Onchocerca volvulus (Filarioidea: Onchocercidae) and transmitted via the bites of Simulium (Diptera: Simuliidae) blackfly vectors. Onchocerciasis is the second leading cause of infectious blindness globally after trachoma, but it also causes skin lesions, depigmentation (leopard skin), and debilitating ‘troublesome itch’ in addition to excess mortality in those heavily infected, partly because of its association with epilepsy [Citation1,Citation2]. Given the clustering of disease near rivers, where the blackflies breed, the disease is colloquially termed ‘river blindness’ [Citation1,Citation2].
The lifecycle of O. volvulus comprises a human stage and a vector stage, with the blackfly vector having its own distinct (holometabolous) lifecycle. Humans are the definitive host of the parasite, harboring adult worm stages known as macrofilariae, with lifespans (mean age to death) of approximately 10 years (with some worms potentially living for at least 20 years). Macrofilariae are dioecious, with distinct sexual dimorphism. Adult female worms must mate with male worms to begin production of the progeny stage, termed microfilariae, which live, on average, for approximately 9–12 months and are responsible for the pathology associated with onchocerciasis. Blackflies can ingest skin-dwelling microfilariae when taking a blood meal on an infected human by virtue of their pool-feeding mechanism. Microfilariae ingested during a bloodmeal migrate through the blackfly’s peritrophic matrix, abdominal epithelium, and hemocoel to reach the thoracic muscles, where, in competent vectors, they develop into infective (L3) larvae in about a week, depending on environmental conditions. L3 larvae are released from the fly’s mouth-parts upon a subsequent blood meal, into the wound created by the feeding blackfly, and develop into adult worms (in about 12–18 months) to complete the cycle [Citation1].
Estimates of the number of O. volvulus-infected people globally range from 17 to 37 million, with 99% of the cases in 31 countries of Sub-Saharan Africa. The disease is also endemic in Yemen and the Amazonian focus straddling Venezuela and Brazil, and has been eliminated from other foci in Meso (Mexico, Guatemala) and South America (northern Venezuela, Colombia, Ecuador) [Citation3]. The burden of disease caused by onchocerciasis has been significantly reduced from 1,442,000 (95% uncertainty interval = 835,000–2,289,000) disability-adjusted life years (DALYs) in 2005 to 1,340,000 (639,000–2,370,000) in 2017 (a reduction of 7%) due to large-scale interventions [Citation4]. The first control program in Africa, the Onchocerciasis Control Programme in West Africa (OCP, 1974–2002), averted an estimated 600,000 cases of blindness in 11 countries [Citation1]. The African Programme for Onchocerciasis Control (APOC, 1995–2015), extended control efforts to the remaining 20 endemic countries in Africa not covered by the OCP, averting a further 200,000 cases of blindness between 1995 and 2011 [Citation5]. Upon APOC’s closure in December 2015, the Expanded Special Project for the Elimination of Neglected Tropical Diseases (ESPEN) was created, to provide technical support to the endemic countries in their efforts to eliminate onchocerciasis [Citation2]. In January 2012, and on occasion of the London Declaration on NTDs, the World Health Organization (WHO) launched a roadmap for accelerating progress against NTDs [Citation6] and proposed the goal of eliminating onchocerciasis where feasible by 2020. This was later revised by the Joint Action Forum (JAF) of APOC to become elimination in 80% of endemic countries by 2025 [Citation7]. In 2019, the WHO launched a consultation process for revising the roadmap toward 2030 and beyond [Citation8].
Since 1989, onchocerciasis control has relied on the mass drug administration (MDA) of (donated) ivermectin (Mectizan®), a broad-spectrum antiparasitic macrocyclic lactone. Ivermectin’s effects on O. volvulus include a microfilaricidal (killing of microfilariae), an embryostatic (temporary inhibition of microfilarial release from female worms), a (modest) permanent sterilizing, and a (partial) macrofilaricidal (killing of macrofilariae) action [Citation9,Citation10] (see 2.3. Pharmacodynamics and antiparasitic effects in onchocerciasis). Distribution of ivermectin in Africa is predominantly by annual community-directed treatment with ivermectin (aCDTI), whereby trained members of the local community act as community drug distributors (CDDs) year on year. Despite elimination successes in some foci of Mali, Senegal, Nigeria, Sudan, and Uganda [Citation11,Citation12] (some under biannual, 6-monthly, treatment), others are not on track to reach the proposed elimination goals with aCDTI or exhibit ‘sub-optimal’ responses (SOR) to the drug [Citation2,Citation11] (see 4.1.4. Settings that have reported sub-optimal responses to ivermectin for a discussion on these).
Consequently, attention has focused on identifying alternative treatment strategies (ATS) aimed at accelerating progress toward elimination of transmission [Citation13]. ATS include (not mutually exclusively) enhancing existing CDTI by increasing geographic and therapeutic coverage as well as treatment adherence; increasing treatment frequency (e.g. to biannual CDTI (bCDTI) or quarterly (3-monthly) treatment where necessary); deploying ground-based focal vector control (in contrast with the logistically demanding and large-scale aerial vector larviciding operations undertaken by the OCP); and using novel therapeutics [Citation14]. Moxidectin represents a prime example of the latter, having been recently been approved by the US Food & Drug Administration (FDA) for the treatment of humans with onchocerciasis due to O. volvulus [Citation15]. Hailing from the field of veterinary medicine, moxidectin is also a macrocyclic lactone and a potent microfilaricide, with a seemingly more prolonged microfilaricidal and/or embryostatic effect than ivermectin. Phase II and III clinical trials have demonstrated moxidectin’s superior clinical performance compared to ivermectin [Citation16,Citation17], indicating its potential to accelerate and enhance the feasibility of onchocerciasis elimination.
This review presents an overview of the chemistry, pharmacology, tolerability, safety, and efficacy of moxidectin for the treatment of human onchocerciasis. It outlines unmet therapeutic needs, summarizes the regulatory status of moxidectin for human use, and provides an expert commentary on how moxidectin could be used within the current landscape of onchocerciasis treatment, control, and elimination.
2. Overview of moxidectin
2.1. Chemistry
Moxidectin is a semi-synthetic macrocyclic lactone of the milbemycin class of macrolide antibiotics. It is derived by chemical optimization of F-alpha or nemadectin (LL F-2924α), a fermentation product of the bacterium Streptomyces cyanogriseus ssp. noncyanogenus, through the addition of a methoxime moiety at carbon 23 [Citation18]. Moxidectin differs from ivermectin (also a macrocyclic lactone but of the avermectin family) in the absence of a disaccharide attached to carbon 13, the presence of an olefinic side chain at carbon 25, and a methoxime moiety at carbon 23. The chemical structures of ivermectin and moxidectin are compared in [Citation18].
2.2. Pharmacokinetics and metabolism
Moxidectin is classified as a Biopharmaceutics Classification System Class II (high permeability, low solubility) compound. Absorption is fast, with a time to peak concentration (tmax) of 3–4 hours [Citation19–21]. Peak plasma concentration (Cmax) and total area under the concentration–time curve (AUC0–∞) showed a linear increase with a dose within the range (3–36 mg) investigated [Citation19,Citation20]. A liquid formulation of moxidectin has approximately 30% higher Cmax and AUC0–∞, and a tmax nearly an hour earlier than a tablet formulation [Citation21], although the liquid formulation resulted in only modestly increased bioavailability (1.29 fold) relative to the tablet formulation and marginally more rapid absorption. A high-fat breakfast (co-administration with lipids) is associated with increased exposure and a delayed tmax [Citation19,Citation22], but the increases are not clinically relevant and moxidectin can be dosed without regard to food. Once absorbed, moxidectin has a large apparent volume of distribution and a half-life of 20–43 days in human beings [Citation19,Citation21], compared to approximately 1 day for ivermectin [Citation23]. Moxidectin is also more lipophilic than ivermectin (partition coefficient of logP = 6 for moxidectin and logP = 4.8 for ivermectin), which may result in greater retention in adipose tissue, which may help explain moxidectin’s long half-life [Citation24]. In vitro studies using mammalian liver microsomes suggested that moxidectin is a limited substrate of CYP-metabolism (cytochrome P450 3A and cytochrome P450 2B [Citation25]), with no evidence for non-CYP-mediated metabolism. In humans, moxidectin is minimally metabolized within the body and did not affect the pharmacokinetics of midazolam, a sensitive CYP3A4 substrate [Citation26]. Moxidectin, therefore, exhibits no clinically relevant cytochrome P450-related drug–drug interactions.
Moxidectin is excreted, mostly unmetabolized [Citation27], in feces. A small amount of moxidectin is excreted into the breast milk of lactating women (relative infant dose ~9% of the maternal dose), who otherwise have identical pharmacokinetics to healthy males [Citation28]. Moxidectin is a poor substrate for P-glycoprotein (P-gp) transporters [Citation29,Citation30], being mostly excreted via a P-gp-independent pathway into the intestine [Citation31]. Intestinal, biliary, and mammary excretion is thought to be through Breast Cancer Resistance Protein (BCRP or ABCG2) [Citation32] unlike ivermectin, for which P-gp transporters appear to be important for both biliary and intestinal secretion [Citation31]. However, moxidectin is effluxed by P-gp transporters at the blood–brain barrier [Citation31], suggesting that tissue-specific P-gp transporters interact with moxidectin differently. Drug efflux at the blood–brain barrier, and/or lower intrinsic activity than ivermectin on mammalian GABA receptors seemingly lower the neurotoxicity of moxidectin compared to ivermectin in dogs and mice [Citation33,Citation34]. The elimination (or lack thereof) of moxidectin through P-gp or similar routes is likely to contribute to the drug’s long half-life and overall efficacy, with the potential for parasites’ (cumulative) exposure to moxidectin far exceeding that of ivermectin. Despite the long half-life, systemic exposure is relatively low from 72 hours post-treatment, with plasma concentrations lower than 3 ng/mL after an 8 mg oral dose.
Nematodes express P-gp in the pharynx, intestine, head neurons, and the uteri of female worms [Citation35,Citation36]. The compelling, albeit indirect, evidence that nematodes P-gp transporters can efflux macrocyclic lactones [Citation37–39], with P-gp expression increasing after in vivo parasites’ exposure to macrocyclic lactones [Citation40,Citation41], is implicated in macrocyclic lactone resistance [Citation42]. It has been hypothesized that P-gp transporters act to limit drug concentrations within the parasite and can alter the drug’s efficacy [Citation42]. Therefore, moxidectin’s limited interaction with P-gp may play a role in its increased efficacy [Citation29]. However, there still is no direct evidence of macrocyclic lactone efflux by nematode P-gp transporters, and the pharmacokinetics within the parasites remain far from clear.
2.3. Pharmacodynamics and antiparasitic effects in onchocerciasis
Moxidectin exerts both stronger and longer suppression of O. volvulus microfilaridermia than ivermectin [Citation16,Citation17]. Its microfilaricidal effect leads to near-complete clearance of skin microfilariae, with peak clearance at around 1 month after treatment, and little variability in inter-individual responses. Ivermectin clears, on average, 98–99% of skin microfilariae, with maximum clearance at 1–2 months after treatment [Citation9], with more pronounced inter-individual variability. Microfilariae are thought to be paralyzed by ivermectin and transported from the skin to the sub-epidermal layers and other organs (e.g. spleen), where they are killed by the body’s immune system [Citation43]; however, the occurrence of Mazzotti reactions suggests some microfilarial killing in the skin. Other proposed mechanisms include disruption of the excretory-secretory apparatus of microfilariae which leads to disruption of the host–pathogen molecular negotiation via suppression of parasite protein secretion [Citation44].
Similar studies have yet to be conducted for moxidectin to ascertain the body sites where processing of the microfilariae takes place and to assess its effect on the excretory-secretory apparatus of microfilariae. With moxidectin, low skin microfilarial loads are sustained, with many individuals having no detectable microfilariae (by skin-snip microscopy) 6 months after treatment [Citation16]. Ivermectin has a similar, albeit weaker and shorter, suppression of microfilariae that is attributed to an ‘embryostatic’ effect (suppression of microfilarial release from female worms in treated individuals). Adult female worms gradually resume the release of microfilariae 3–4 months after ivermectin treatment [Citation9]. Given the relative similarity of the two drugs, and the observed dynamics of skin repopulation, it has been conjectured that moxidectin’s longer suppression of skin microfilariae suggests a more potent and prolonged embryostatic effect [Citation45], although other mechanisms cannot be ruled out. presents the microfilarial dynamics following a single dose of ivermectin ()) or moxidectin ()).Repeated doses of ivermectin are postulated (through modeling of data from Ghana [Citation46]) to effect a cumulative (and irreversible) sterilizing effect on adult worms, estimated at around 30–35% reduction in microfilarial productivity per dose [Citation47]. However, field studies in Cameroon [Citation48] and modeling of data from Guatemala [Citation49] and Cameroon [Citation10] reported little evidence of a cumulative effect of repeated ivermectin doses on female worm fecundity. A macrofilaricidal effect of (annual and quarterly) ivermectin treatments has been documented in field trials [Citation50] and its magnitude (50% and 70% reduction in adult worm life-expectancy, respectively) has been estimated through modeling [Citation10]. A recent analysis of the data collected in [Citation50] suggests that quarterly ivermectin treatments may reduce the incidence of new nodules compared to annual treatments [Citation51]. We emphasize that the estimation of both cumulative and macrofilaricidal effects of ivermectin required modeling analysis of longitudinal data on repeated (annual, biannual, quarterly) treatments [Citation46,Citation50,Citation52]. Such data have not yet been collected and modeled for moxidectin and, therefore, there is currently no direct evidence of moxidectin’s potential sterilizing and/or macrofilaricidal effects. The more prolonged embryostatic effect of moxidectin that has been used in modeling studies [Citation45] has been assumed by analogy with the observed microfilarial pharmacodynamics after a single (standard, 150 µg/kg of body weight) dose of ivermectin [Citation9,Citation45]. A prophylactic effect of monthly and quarterly ivermectin and moxidectin treatments on O. ochengi (the closest relative of O. volvulus) has been documented in its cattle host [Citation53].
Figure 1. The observed and modeled skin microfilarial dynamics following a single dose of: (a) ivermectin (150 µg/kg) and (b) moxidectin (8 mg)
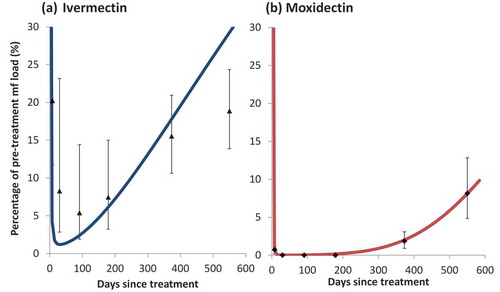
The principal mechanism of antiparasitic action for moxidectin is thought to be as for ivermectin’s, mediated by glutamate-gated chloride channels (GluCls) of the target helminths [Citation54]. This binding acts to increase channel permeability, with the influx of chloride ions resulting in dysfunction of the excretory pore, flaccid paralysis, and death of the parasite through host response. X-ray crystallography showed ivermectin to be capable of binding to the transmembrane domain of GluCl in the Caenorhabditis elegans (free-living) nematode model system [Citation55]. Although research has not mostly focused on O. volvulus (for which no satisfactory animal model exists), GluCl genes are highly conserved across filarial nematodes [Citation56–58]. Homology modeling (based on Brugia malayi Bm-GluCl), used together with computer-based docking of moxidectin’s 3-dimensional ligand site, suggests that GluCl binding should be conserved with moxidectin [Citation58], with in vitro studies showing that moxidectin increases GluCl channel activity [Citation59,Citation60]. Although no direct binding has been observed between moxidectin and ligand-gated chloride channels, in vitro assays suggest that worm paralysis requires concentrations of ivermectin and moxidectin [Citation61] 2–3 orders of magnitude higher than the peak plasma levels achieved in vivo [Citation23,Citation62]. At such high concentrations (well above pharmacologically relevant concentrations), macrocyclic lactones can act as agonists for gamma-aminobutyric acid (GABA)-gated chloride channels, that could contribute to worm paralysis in motility assays [Citation54]. The lack of effect on parasite motility at pharmacologically relevant concentrations suggests that parasite paralysis in the skin may not be a macrocyclic lactones’ mechanism of action. Other potential mechanisms of action include altering the secretion of immunomodulatory proteins and molecules reducing the parasite’s ability to evade host immune responses [Citation44]. In Brugia malayi, ivermectin has been demonstrated also to disrupt the function of the excretory-secretory apparatus of microfilariae, supporting the argument that ivermectin treatment in human filariases, including onchocerciasis, leads to disruption of the host–pathogen molecular negotiation via suppression of parasite protein secretion [Citation44]. Such mechanisms may be responsible for differences in drug efficacy between in vitro and in vivo studies.
Note that macrocyclic lactones also have activity against invertebrate ectoparasites (such as ticks), as well as other parasitic nematodes [Citation24]. Discussion of these is beyond the scope of this review, but we recommend Prichard et al. [Citation24] for a detailed review of these topics.
2.4. Resistance
Resistance to ivermectin has not been documented in humans. However, studies in Ghana of populations repeatedly treated with ivermectin have reported so-called ‘suboptimal’ responses (SOR) to ivermectin, phenotypically characterized by faster (poor responses) than expected (good responses) rates of skin repopulation by O. volvulus microfilariae [Citation63,Citation64]. SORs have been proposed to occur because of a reduction in ivermectin’s embryostatic effect (manifested as an earlier resumption of microfilarial production by female worms). Worms extracted from thus characterized ‘poor’ and ‘good’ responders in Ghana and Cameroon populations have been analyzed with genome-wide approaches to understand the genetic basis of this apparent loss of ivermectin sensitivity [Citation65]. According to the SOR definition, however, and in the moxidectin vs. ivermectin clinical trials (see 3. Clinical trials of moxidectin), individuals who were ivermectin naïve showed SORs following ivermectin but not moxidectin treatment [Citation17], indicating that a high degree of inter-individual variability in responses to ivermectin is present before wide-spread and prolonged treatment [Citation66]. Smaller levels of inter-individual variability in responses to moxidectin suggest that the drug could be useful in communities with increased frequency of ivermectin SORs.
Resistance to moxidectin (and to ivermectin) is common in parasitic nematodes of livestock and companion animals [Citation67]. Even in resistant populations, moxidectin retains a higher efficacy than the avermectins, but continued exposure can result in therapeutic failure and cross-resistance between moxidectin and ivermectin [Citation67]. The genetic analysis of O. volvulus taken from poor and good responders in Ghana and Cameroon found that decreased ivermectin efficacy in human onchocerciasis may be determined by (geographically different) quantitative trait loci, mostly unrelated to the few candidate genes and mechanisms that had been proposed in resistance studies of veterinary parasitic nematodes [Citation66]. In Haemonchus contortus, a major quantitative trait locus has been established to be associated with ivermectin resistance [Citation68], suggesting that the mechanisms of ivermectin resistance in parasites of humans and farmed ruminants may be somewhat distinct. However, if moxidectin were widely adopted for large-scale treatment of onchocerciasis and other endo/ectoparasitic infections of humans, it would be essential to regularly monitor its efficacy, and understand its impact on the genetic diversity and structure of O. volvulus (and other parasite) populations. This is particularly important for onchocerciasis for which the ultimate goal is global elimination (i.e. reducing the potentially rescuing effect that untreated refugia could have on the spread of SORs, but see [Citation69] for a review of the conventional wisdom of, and challenges to the concept of refugia in mitigating anthelmintic resistance).
3. Clinical trials of moxidectin
3.1. Safety and tolerability
Multiple phase I studies, first by Cotreau et al. [Citation19] and subsequently by Korth-Bradley et al. [Citation21,Citation22,Citation26,Citation28] and Kinrade et al. [Citation20], established that the safety and tolerability of moxidectin are very similar to placebo in healthy volunteers. Moxidectin doses ranging from 3 to 36 mg were well tolerated with no severe adverse events (SAEs) and mild/moderate adverse events (AEs) such as headache. There was no increase in incidence, nature, or severity of AEs with increasing dose [Citation19–21]. Up to the maximum 36 mg dose tested, moxidectin did not prolong the QT interval [Citation20]. (A drug’s influence on cardiac repolarization is assessed by detecting prolongation of the QT interval, which represents the duration of ventricular depolarization and subsequent repolarization; QT prolongation is a biomarker for a drug’s risk of causing arrhythmias [Citation70].)
In the treatment of onchocerciasis patients, moxidectin results in the same risk management profile as ivermectin. The incidence and severity of AEs were similar between moxidectin and ivermectin. Rapid microfilarial death in infected patients can result in AEs, including pruritus, rash, tachycardia, and orthostatic hypotension as part of the so-called Mazzotti reactions (complex, acute inflammatory responses mounted by the immune system after administration of microfilaricides). Both phase II and phase III clinical trials monitored basic vital signs as well as monitoring of AEs and SAEs including Mazzotti reactions. Nearly all patients in either treatment arm in both phase II and III trials had some form of Mazzotti reaction but events were transient and self-limiting. Nearly all non-Mazzotti AEs and all SAEs were unrelated to the drug administration. Treatment groups with higher microfilarial loads were more likely to suffer reactions [Citation16,Citation17], unsurprising given that Mazzotti reactions are correlated with skin microfilarial density [Citation71]. In the phase II trial, the 8 mg moxidectin group showed a statistically significant (p < 0.05) increase in the occurrence of rash and pruritus, as well as increased pulse rate and hypotension compared to ivermectin. There was no significant difference between ivermectin and moxidectin 2 mg, although 4 mg showed a significant increase in hypotension compared to ivermectin. In the much larger phase III trial, the frequency, nature, and severity of Mazzotti reactions were very similar between ivermectin and 8 mg moxidectin. Moxidectin significantly increased (Onchocerciasis Chemotherapy Research Centre grade 4) Mazzotti reactions (p < 0.01), primarily attributed to increased rates of hypotension. No patient in either trial required major clinical intervention for a Mazzotti reaction. In the phase II trial, all rashes resolved without intervention with only one severely infected participant treated with 2 mg moxidectin requiring chlorpheniramine to relieve pruritus symptoms. Hypotension, in both phase II and III trials, required no treatment beyond lying down for a few minutes.
To date, no study has been conducted to investigate the safety of using moxidectin in O. volvulus–Loa loa co-infected individuals. Ivermectin can cause SAEs, including potentially fatal encephalopathy, in individuals with high L. loa microfilaremia [Citation72]. The pathophysiology of such SAEs is poorly understood but it appears to have an immunological basis, involving interleukin (IL)5-driven eosinophilia and eosinophil activation (positively correlated with pre-treatment microfilarial count) in the mechanism of microfilarial killing by ivermectin in loiasis [Citation73]. Given moxidectin’s strong microfilaricidal effect and overall similarity to ivermectin, it will be crucial to determine the safety of moxidectin for the treatment of onchocerciasis in loiasis co-endemic areas, its efficacy for the treatment of loiasis, and the immunological mechanisms involved in the killing of L. loa microfilariae and any subsequent AEs.
3.2. Clinical efficacy against O. volvulus
3.2.1. Phase II trial
The phase II trial compared 2, 4, and 8 mg doses of moxidectin to the standard ivermectin dose (150 µg/kg, translating into 6, 9, or 12 mg doses according to the weight range of participants in the 8 mg moxidectin arm) [Citation16,Citation45]. Male and female participants between the ages of 18 and 60 years and with O. volvulus infection (determined by identification of microfilariae in skin snips) were recruited from the River Tordzi basin in South-eastern Ghana, from communities with no previous history of vector control or ivermectin MDA.
Notable exclusion criteria included pregnancy or breastfeeding, anti-filarial therapy within the previous 5 years, and loiasis co-infection. A total of 172 patients were recruited with 166 (96.5%) completing the study. The trial used a 3∶1 moxidectin∶ivermectin randomization ratio, such that the ivermectin control arm and the 3 moxidectin dose arms each contained approximately 45 patients. Patients were further stratified by pre-treatment skin microfilarial load as: mild (<10 microfilariae/mg skin and no ocular involvement), moderate (10–20 microfilariae/mg skin and low ocular involvement), and severe (>20 microfilariae/mg skin and with or without ocular involvement), with approximately 50% of recruited patients in the severe infection category. Drug efficacy was monitored based primarily on skin microfilarial density using a total of 4 skin snips taken from the right and left iliac crests and calves before and 8 days and 1, 2, 3, 6, 12, and 18 months after treatment.
All three moxidectin doses (2, 4, and 8 mg) achieved both faster and considerably more complete skin microfilarial clearance than ivermectin. For all three moxidectin doses, the number of patients with undetectable levels of skin microfilariae was statistically significantly greater than with ivermectin, with the difference reaching significance as soon as 8 days after treatment with 4 and 8 mg moxidectin. For the 8 mg dose, the proportion of participants with undetectable skin microfilariae was significantly higher than that for 2 and 4 mg moxidectin at 12 and 18 months after treatment. The average annual reduction in skin microfilarial density was 88% for ivermectin, compared to 97%, 98%, and 98% for 2, 4, 8 mg moxidectin, respectively. Microfilaridermia began increasing after 2 months for ivermectin, after 3 months for 2 mg moxidectin, after 6 months for 4 mg, and after 12 months for 8 mg moxidectin.
The trial also looked at the proportion of live female macrofilariae in (a total of 214) onchocercomata (O. volvulus nodules) excised from 126 patients (all treatment groups) at 18 months after treatment and reported this to be 50% (n = 92 female worms, 95% confidence interval (95% CI) = 40–60%) for ivermectin; 64% (n = 115, 95% CI = 54–72%) for 2 mg moxidectin; 68% (n = 90, 95% CI = 58–77%) for 4 mg, and 72% (n = 65, 95% CI = 60–82%) for 8 mg moxidectin. Although these proportions are statistically significantly different between ivermectin and moxidectin 4 mg (p = 0.0147), and ivermectin and moxidectin 8 mg (p = 0.0051), they are not significantly different between ivermectin and 2 mg moxidectin or between the three moxidectin dose groups. The authors of the trial questioned the true significance of their adult worm analysis given the small sample size, pre-treatment imbalance between treatment groups and the possibility that the excised macrofilariae may not have been truly representative of an individual’s total macrofilarial load [Citation16]. They concluded that their analysis was insufficiently powered to quantify treatment effects on macrofilariae. Interestingly, however, the proportion of live female worms in the ivermectin group (50%) and the three moxidectin groups (64–72%) falls within the range of variation modeled by Walker et al. [Citation10] when estimating the macrofilaricidal effect of one (annual) round of ivermectin in the field trial conducted by Gardon et al. [Citation50] in Cameroon [Citation10].
3.2.2. Phase III trial
The Phase III trial was a randomized, double-blind trial directly comparing the efficacy of 8 mg moxidectin vs. 150 μg/kg ivermectin [Citation17] (see 3.2.1. Phase II trial for a range of ivermectin doses in mg). Male and female participants aged ≥12 years and infected with O. volvulus infection were recruited from communities in Ghana, Liberia, and the Democratic Republic of the Congo (DRC), none of which had received CDTI. Notable exclusion criteria included: pregnancy or breastfeeding; harboring <10 microfilariae/mg as assessed by skin snip; co-infection with lymphatic filariasis or loiasis, and having received anthelmintic treatment in the past 6 months. After screening, a total of 1,472 participants were randomized at a 2:1 ratio to the moxidectin or ivermectin control arms. The primary measure of efficacy was again skin microfilarial density (by four skin snips as described above in the phase II trial) 1, 6, 12, and 18 months after treatment compared to microfilarial density before treatment. Moxidectin 8 mg resulted in lower skin microfilarial loads than ivermectin at the 1-, 6-, 12- and 18-month follow-up times. At 12 months, the (geometric) mean microfilarial load in the moxidectin group (0.6 microfilariae/mg, 95% CI = 0.3–1.0) was statistically significantly lower than that in the ivermectin group (4.5 microfilariae/mg, 95% CI = 3.5–5.9; p < 0.0001; treatment difference 86.7%), and this reduction was independent of sex, but greater (93%) in those with ≥20 microfilariae/mg before treatment than in those with <20 microfilariae/mg (76%) before treatment. Significantly more participants maintained undetectable skin microfilariae in the moxidectin group (360/938 = 38%) than in the ivermectin group (7/478 = 1.5%) (p < 0.0001) between 1 and 12 months after treatment.
summarizes AEs of moxidectin in comparison to ivermectin from the results of the phase II and III clinical trials described above, and presents the efficacy against O. volvulus of moxidectin vs. ivermectin in those trials.
Table 1. Summary of adverse events (as a percentage of participants with AEs or SAEs) for ivermectin and moxidectin from phase II and III clinical trials
Table 2. Summary of efficacy on Onchocerca volvulus microfilariae for ivermectin and moxidectin from phase II and III clinical trials
4. Overview of unmet needs regarding the treatment of onchocerciasis
Given the superior microfilaricidal efficacy of moxidectin, as demonstrated in the above-described clinical trials (section 3.2. Clinical efficacy against O. volvulus), it is important to consider what would be the role that moxidectin could play in helping to fill unmet therapeutic needs for the treatment, control, and elimination of onchocerciasis. Therefore, we describe below the epidemiological scenarios in which ivermectin distribution would be insufficient and how moxidectin could help achieve or accelerate elimination of transmission goals [Citation8]. We also discuss the development of novel macrofilaricides, particularly those based on anti-Wolbachia action, which could potentially be combined with moxidectin to harness superior macro- and microfilaricidal activity. For a review of research on new drugs for the elimination of onchocerciasis in Africa see [Citation14].
4.1. Major impediments to onchocerciasis elimination
4.1.1. Settings with a long-term history of community-directed treatment with ivermectin (CDTI)
Some communities have received many years of CDTI but have made somewhat limited progress toward elimination [Citation74–76]. This may be due to low levels of therapeutic coverage (proportion of the total population taking the drug on a given treatment round) and/or high levels of systematic non-adherence (proportion of the population never taking treatment) due to programmatic constraints [Citation77], with untreated (or infrequently treated) individuals maintaining microfilarial loads that contribute to transmission. This situation has been documented in a number of communities across Africa [Citation78–81]. In others, however, although acceptable coverage and adherence may be reached and sustained, very high rates of transmission (due to high blackfly biting rates and/or intense exposure to blackfly bites) can result in substantial inter-treatment transmission even if microfilarial loads are low. Despite the initial rapid microfilaridermia reduction that follows ivermectin, adult female worms gradually resume microfilarial production 3–4 months after treatment [Citation9]. Therefore, as microfilariae repopulate the skin during the period between rounds of aCDTI (or even bCDTI), transmission to vectors can be considerable in communities with high blackfly biting rates. Mathematical modeling studies of onchocerciasis transmission suggest that both high endemicity and unsatisfactory coverage, adherence, and frequency can hinder elimination [Citation82,Citation83]. Moxidectin, delivered through community-directed treatment approaches, could replace ivermectin in areas of persistent transmission, but it would also necessitate reaching and sustaining high levels of therapeutic coverage and adherence. Its superior efficacy and more prolonged suppression of microfilaridermia levels would help, however, to curtail inter-treatment transmission more effectively than ivermectin. Modeling studies have suggested that annual community-directed treatment with moxidectin (aCDTM) may have a similar epidemiological impact to that of bCDTI and mitigate against the increased cost of biannual distribution [Citation45].
4.1.2. Settings with no or very little community-directed treatment with ivermectin (CDTI)
Some endemic communities, mostly of low (hypo-)endemicity, have remained largely untreated. Within APOC, foci delineated as hypoendemic (nodule prevalence <20%, corresponding to a skin microfilarial prevalence <35–40% [Citation84]) were not prioritized for treatment, partly because of their limited contribution to onchocerciasis-associated morbidity, and because of uncertainty on their ability to maintain autochthonous (endemic) transmission [Citation85]. Thus, it was proposed that eliminating O. volvulus in higher endemicity communities would precipitate elimination in (epidemiologically coupled) hypoendemic foci. Most communities delineated as hypoendemic occur in vast rural areas, with very little detailed information on transmission intensity or endemicity [Citation85,Citation86]. Yet, in-depth epidemiological evaluations of areas previously delineated as hypoendemic have revealed that some areas contained pockets of higher endemicity or were wrongly classified as hypoendemic when reassessed using a more sensitive diagnostic [Citation87]. There also exist higher endemicity (meso- and hyperendemic) communities that should have received treatment (nodule prevalence ≥20%, microfilarial prevalence ≥35–40% [Citation84]) but which have received none or very few rounds of CDTI because of political and social instability (e.g. programs in the DRC, Sierra Leone, and South Sudan) [Citation2]. Although ivermectin distribution may have started in some of these communities, progress is far behind compared to elsewhere in Africa. Therefore, implementation of aCDTM or biannual CDTM (bCDTM) from a very early stage could help accelerate progress toward elimination (in hypoendemic–mesoendemic settings) or increase its feasibility (in hyperendemic areas).
4.1.3. Settings that are hypoendemic for onchocerciasis but co-endemic with loiasis
A major limitation to the implementation of ivermectin MDA in communities that are hypoendemic for onchocerciasis but co-endemic with loiasis (another filarial infection, caused by Loa loa, and distributed in the forest belt of central Africa) is the risk of SAEs (e.g. encephalitis, coma, death), following microfilaricidal treatment of individuals with heavy L. loa microfilaremia [Citation72]. Although Gardon et al. [Citation72] found a significantly increased risk of developing prolonged functional impairment above 8,000 L. loa microfilariae/mL of blood, and an increased risk of encephalopathy above 30,000 microfilariae/mL, others have used ≥20,000 as the SAE threshold [Citation88]. Hypoendemic onchocerciasis–loiasis co-endemic areas with L. loa prevalence >20% (where it is expected that 2–9% of individuals harbor ≥30,000 microfilariae/mL) would be excluded from CDTI [Citation13]. In these situations, test-and-not-treat (TNT) protocols have been successfully piloted [Citation88] which test for L. loa microfilaremia levels in community residents, and for those harboring levels above those associated with SAEs, albendazole (or anti-Wolbachia therapies, see 4.2. Other compounds in clinical development), but not ivermectin, may be offered. Although clinical trials have yet to be conducted to assess the safety of moxidectin in onchocerciasis–loiasis co-endemic areas, it could potentially be used in TNT modalities of drug distribution, after having established safe (L. loa) microfilaremia thresholds for treatment. Once a safe microfilaremia threshold is established, it would also be important to determine the efficacy of moxidectin for treating (low to moderate) L. loa infection (see [Citation89] for a meta-analysis of studies investigating the effect of ivermectin on L. loa), and to determine the need to re-test individuals for levels of loiasis microfilaremia following initiation of TNT with moxidectin.
In areas of meso- and hyperendemic onchocerciasis and co-endemic loiasis, it has been considered that the benefits of CDTI for onchocerciasis treatment/elimination outweigh the risks of SAEs (provided that enhanced surveillance is in place for their prompt identification and management [Citation13]), although this judgment has attracted criticism and may have contributed to the low levels of coverage and adherence to treatment in certain settings because of the fears of SAEs [Citation80]. If moxidectin were to replace ivermectin in these co-endemic settings, it would be essential to evaluate the relative benefits and risks of CDTM.
4.1.4. Settings that have reported sub-optimal responses to ivermectin
The fourth (and least well-understood) group of potential impediments to onchocerciasis elimination refers to the occurrence of so-called sub-optimal responses (SORs) to ivermectin [Citation63–66], which have been described and discussed in 2.4. Resistance. Here we re-iterate the imperative to monitor the efficacy of moxidectin should it widely replace ivermectin for the treatment, control, and elimination of onchocerciasis, and to better understand the impact of MDA on the population genetic structure of O. volvulus [Citation65].
4.2. Other compounds in clinical development
Other than moxidectin, the drugs furthest along in clinical and pre-clinical development are anti-Wolbachia therapies, targeting the endosymbiotic Wolbachia pipientis bacterium within the parasite [Citation90–92]. Depletion of Wolbachia (by >90%), following treatment with tetracycline antibiotics such as doxycycline, exerts a potent but protracted macrofilaricidal activity, reducing the adult worms’ life-expectancy by 70–80%, from approximately 10 years to 2–3 years [Citation93]. This slow-killing action contributes to its safety and tolerability profile [Citation91,Citation93]. Although not directly microfilaricidal, microfilaridermia loads decrease progressively as a result of female worm sterilization by inhibition of the parasite’s embryogenesis [Citation90]. Furthermore, microfilariae from doxycycline-treated individuals exhibit retarded development to the infective, L3 stage, if ingested by blackfly vectors for at least 5 months after treatment compared to microfilariae from placebo-treated patients [Citation94]. Data from animal models of filariasis also show that, during the course of treatment, larvae entering the vertebrate host do not establish as adult worms, thereby indicating a prophylactic effect [Citation95]. To achieve the critical efficacy threshold of >90% Wolbachia depletion [Citation90], a 4–6-week course of 100–200 mg of doxycycline is required [Citation93]. As L. loa does not harbor W. pipientis, the parasite is unaffected by anti-Wolbachia therapies [Citation96], which can, therefore, be safely used to treat onchocerciasis in loiasis co-endemic areas [Citation97].
High levels of coverage (74%) and adherence (98%) with a 6-week (100 mg daily) course (for those aged ≥12 years) were documented in a feasibility trial of community-directed treatment with doxycycline (CDTD) in communities of Cameroon co-endemic with loiasis [Citation98]. A significant impact on epidemiological indicators (microfilarial prevalence and load) was recorded 3–4 years after treatment in doxycycline-treated individuals from these communities [Citation99]. The sterilizing and macrofilaricidal efficacy of doxycycline has also been demonstrated in communities with documented SORs to ivermectin [Citation100]. Treatment with doxycycline in combination with ivermectin is highly effective in exerting a prolonged (18-month) suppression of microfilarial loads in clinical trials [Citation101]. To date, no trial has evaluated the efficacy of moxidectin and doxycycline in combination.
The Cameroon CDTD feasibility field trial of Wanji et al. [Citation98] employed pre-treatment advocacy meetings and daily monitoring of patients throughout the treatment course delivered by community health implementers trained to deliver doxycycline MDA. Such strong community engagement undoubtedly contributed to improved coverage and adherence, with a cost estimated at USD 2.5 per course per person [Citation98] compared to approximately USD 0.50 per treatment for CDTI [Citation102]. A preliminary modeling study [Citation103] indicated that CDTD would be approximately twice as effective in preventing prevalent cases of O. volvulus infection compared to CDTI, and more than twice as effective in reducing levels of transmission. Moreover, CDTD would be about as cost-effective as CDTI in loiasis co-endemic areas, partly because CDTD could be delivered less frequently than CDTI (e.g. biennially (every 2 years) rather than annually), and partly because it would avoid the increased costs associated with monitoring and surveillance of people treated with ivermectin (in meso- and hyperendemic onchocerciasis–loiasis co-endemic areas) to minimize SAEs [Citation13].
Despite these favorable arguments, the length of an efficacious (at least 5-week) doxycycline course – with which adherence is key – together with treatment contra-indications in pregnant women and children aged <8 years (but see [Citation104,Citation105]), and the potential risk of increasing antimicrobial resistance in other concomitant infections, represent substantial barriers to the widespread implementation of doxycycline for community-directed treatment. Therefore, a test-and-treat with doxycycline (TTd) strategy (with and without focal 10-week vector control through ground larviciding of blackfly breeding sites) has been trialed in comparison to routine aCDTI in South West Cameroon by the COUNTDOWN Consortium [Citation106]. Preliminary modeling results indicate that not only is achieving high levels of therapeutic coverage and adherence essential (the ‘Td’ component of the strategy), but crucially, the coverage of screening for O. volvulus microfilariae (the pre-requisite ‘T’ component) must also be high [Citation107].
The anti-Wolbachia consortium (A·WOL) was established to find anti-Wolbachia drugs at least as efficacious as doxycycline but requiring considerably shorter treatment courses and without its contra-indications [Citation91,Citation92]. Other anti-Wolbachia drugs that have been trialed in humans include rifampicin and moxifloxacin [Citation108] (results yet to be published), and minocycline [Citation109]. Other compounds screened by A∙WOL that have shown great promise in pre-clinical development, are high-dose rifampicin [Citation110], rifampicin plus albendazole [Citation111], and an optimized azaquinazoline (AWZ1066S) [Citation112]. Other compounds (in the Drugs for Neglected Diseases initiative (DNDi) filariasis portfolio) [Citation113] include oxfendazole (for which a phase I trial is being planned through the Helminth Elimination Platform (HELP)), emodepside (nearing phase II trial in Ghana for safety, tolerability, and dose/regimen selection), and TylAMacTM (ABBV-4083, developed by A∙WOL in partnership with AbbVie [Citation114]), for which a phase II proof-of-concept trial is being prepared in the DRC [Citation113].
compares the characteristics, pharmacokinetics, pharmacodynamics, modalities of distribution in onchocerciasis-endemic communities, and regulatory status of ivermectin, moxidectin, and doxycycline for the treatment and control of O. volvulus infection.
Table 3. Characteristics of ivermectin, moxidectin, and doxycycline
5. Regulatory status of moxidectin
5.1. Approval by the USA Food and Drug Administration (FDA)
On 13 June 2018, the FDA approved the use of moxidectin for the treatment of human onchocerciasis in patients aged 12 years and older [Citation15]. The posology is an 8 mg dose (specifically four 2-mg tablets), not dependent on the weight of the recipient and without regard to food. (By contrast, ivermectin can be used for those aged ≥5 years, and doxycycline for patients aged ≥8 years.) The FDA approval does not give any explicit contra-indications for moxidectin but notes the lack of sufficient data to establish its safety during pregnancy, lactation, and ages less than 12 years, and provides warnings of Mazzotti reactions, worsening of onchodermatitis, symptomatic orthostatic hypotension, and SAEs in patients with loiasis. The FDA approval package for the drug outlined two post-marketing requirements for moxidectin, namely: (i) evaluation of pre- and post-natal toxicity assessment in rats, and (ii) conduction of a prospective, randomized, ivermectin-controlled trial of repeated doses of 8 mg moxidectin for onchocerciasis control [Citation15].
5.2. Future trials
The National Institutes of Health (NIH) indicate registration of three trials for the treatment of onchocerciasis in preparatory stages, aimed at: (i) assessing the safety and efficacy of biannual doses of moxidectin [Citation116], (ii) establishing the appropriate dose and formulation of moxidectin for treatment of children aged 4 to 11 years [Citation117], and (iii) evaluating the safety and efficacy of 2 mg moxidectin in patients with L. loa microfilaremia [Citation118].
6. Conclusions
Moxidectin represents a powerful drug, recently incorporated into our armory, for the fight against human onchocerciasis both in clinical and community settings. To realize its full potential in the latter context, and particularly as a drug suitable for MDA, repeated doses and/or increased treatment frequency may be necessary. It will also be essential to extend the indication to include children under 12. Clinical trials have been registered and are in the process of being designed or conducted to accomplish these requirements [Citation116,Citation117]. Where not possible for MDA use, as in onchocerciasis–loiasis co-endemic areas, trials establishing moxidectin’s safety and efficacy for treatment of individuals with loiasis microfilaremia have also been registered [Citation118]. The analysis of the clinical trials that are planned can be assisted by mathematical modeling [Citation10,Citation93] to ascertain more robustly the anti-filarial properties and any macrofilaricidal effect that moxidectin may exert. Economic considerations such as its manufacturing and distribution costs, as well as the feasibility of its (probably time-limited) donation (see 7. Expert opinion) will require further investigation [Citation102]. Given moxidectin’s superior efficacy compared to ivermectin, moxidectin clearly has the potential to revolutionize the treatment, control, and elimination of onchocerciasis due to O. volvulus.
7. Expert opinion
Phase II and III clinical trials have demonstrated the superiority of moxidectin compared to ivermectin, with stronger and longer microfilaridermia suppression, very little difference in side effects, smaller inter-individual variability in responses to treatment, and absence of suboptimal responders [Citation16,Citation17]. Given that microfilariae are mostly responsible for onchocerciasis-associated morbidity and possibly also excess mortality [Citation1], moxidectin provides a better therapeutic option in clinical settings. From the epidemiological perspective of the treatment of populations, a modeling study predicted that moxidectin (delivered as CDTM) has the potential to reduce substantially times to elimination (or specifically, to an operational microfilarial prevalence threshold taken as a proxy of elimination) across a range of endemicity settings [Citation45]. Therefore, moxidectin represents an excellent ATS drug to fill some crucial unmet needs for the treatment, control, and elimination of onchocerciasis. In communities with very high transmission due to high vector biting rates and/or exposure to vector bites, moxidectin’s complete microfilarial suppression 6 months after treatment, and very low microfilarial loads even 12 months after treatment, has the prospect to reduce considerably the magnitude of inter-treatment transmission (from humans to vectors) compared to ivermectin, particularly if high levels of therapeutic coverage and adherence can be reached and sustained [Citation45]. In settings with low–moderate coverage foci, it is anticipated that even individuals who are not treated at every round may harbor lower microfilarial loads for longer, hopefully reducing the impact of missed treatment rounds (although parasite population biology processes enhancing transmission at low infection levels [Citation1] will counteract this somewhat [Citation45]). High levels of systematic non-adherence would still hamper moxidectin’s epidemiological impact, emphasizing the need to understand and minimize the factors leading to reduced adherence [Citation78–83].
Regarding community treatment, it is envisaged that moxidectin will offer major advantages over other proposed ATS. Given that no data yet exist to evaluate the effect on O. volvulus transmission and incidence of repeated moxidectin treatment rounds, transmission dynamic modeling studies can be used to project infection trends in human and vector populations according to endemicity levels and programmatic factors such as treatment duration, frequency, coverage, and adherence, among others. The only study published to date used phase II clinical trial data to calibrate the (EPIONCHO) model, and suggested that aCDTM can be as effective as bCDTI in shortening times to reach given microfilarial prevalence thresholds [Citation45]. Given that biannual ivermectin distribution incurs a greater (yet not simply double) cost than aCDTI [Citation102], aCDTM may represent an economically superior choice compared to increasing ivermectin treatment frequency to bCDTI. This modeling study [Citation45] assumed that CDTM could be deployed to the same age groups as CDTI, namely to those aged ≥5 years. Given that the current FDA approval is for those aged ≥12 years [Citation15], the determination of an appropriate dose for the treatment of children under 12 is a pressing need [Citation117]. The health economic analysis presented in [Citation45] assumed that moxidectin would be donated on the same terms as ivermectin (for as long as necessary wherever needed). Currently, it remains unclear whether moxidectin will be donated on these terms. However, given that [Citation45] suggests that aCDTM could achieve substantial in-country cost savings (30–40% reductions in program costs when compared to ivermectin), accelerate onchocerciasis elimination compared to aCDTI, and be less influenced by transmission and drug distribution seasonal patterns, some of the drug costs of moxidectin could be mitigated. Further work on economic evaluations of not only moxidectin, but all proposed ATS is an important area of research [Citation102]. When comparing moxidectin to other (non-ivermectin-based) ATS [Citation13], such as test-and-treat with doxycycline (TTd) and/or localized vector control [Citation106], moxidectin would be able to capitalize upon the community-directed treatment framework already in place in endemic foci in Africa [Citation2,Citation11], particularly if it were donated as is ivermectin. Thus, in principle, CDTI could transition to CDTM across Africa without much anticipated disruption to existing drug distribution platforms. Some extra training of CDDs may be needed to ensure the delivery of the correct moxidectin dose, as there are some differences compared to the posology of ivermectin: 150 µg/kg of body weight to those aged ≥5 years (which necessitates the use of dosing height poles and translates into a variable number of tablets) compared to four 2-mg tablets (regardless of weight and presently for those aged ≥12 years). However, as CDDs should be re-trained yearly or every 2 years, this should not incur a greatly increased cost.
In areas hypoendemic for onchocerciasis and co-endemic with loiasis at ≥20% prevalence, MDA is not advisable [Citation13], but after establishing safe dosage and thresholds of L. loa microfilaremia levels [Citation118], moxidectin could be used in TNT modalities of delivery that may potentially be more effective than using ivermectin (due to the enhanced curtailing of transmission that would result from more prolonged suppression of microfilaridermia). Doxycycline (already available) and other anti-Wolbachia macrofilaricides (in pre-clinical and clinical development [Citation110–114]) offer an exciting prospect, as TTd for the former, and ideally with much shorter treatment courses for the latter, albeit requiring high rates of O. volvulus screening, therapeutic coverage, and adherence [Citation107]. Large-scale and long-term (at least 14 years) larviciding of vector breeding sites was effective for vector control in the OCP [Citation1] but unlikely to be implemented elsewhere. Therefore, the feasibility, cost, environmental considerations, and optimal localization and duration of ground-based focal larviciding [Citation106] or of non-insecticidal community-directed approaches [Citation119] need to be further explored. The epidemiological impact of superior microfilaricidal (moxidectin) plus macrofilaricidal (anti-Wolbachia or direct-acting) combination therapies also warrants further investigation.
Article highlights
Moxidectin is a milbemycin endectocide that has been used to treat nematode and ectoparasitic infections of livestock and companion animals and has recently been approved by the FDA for the treatment of onchocerciasis in humans aged ≥12 years.
Moxidectin (8 mg) exerts a potent microfilaricidal effect (killing of Onchocerca volvulus microfilariae) and a prolonged suppression of microfilaridermia, which may result from a strong embryostatic effect (temporary inhibition of microfilarial production by adult female worms), although other mechanisms cannot be ruled out.
Moxidectin is not considered to be curative or macrofilaricidal (killing of adult worms), but data on repeated treatments with long follow-up times are currently lacking to rigorously evaluate this.
In healthy volunteers, moxidectin was well tolerated in the range of 3–36 mg investigated in phase I clinical trials; this range includes the recommended 8 mg therapeutic dose against human onchocerciasis. In O. volvulus-infected patients, moxidectin treatment was associated with an increased incidence of adverse events associated with microfilaricidal activity compared to ivermectin, but nearly all were mild to moderate, did not require medical intervention, and were self-limiting.
Moxidectin showed impressive efficacy in both phase II and phase III clinical trials. Compared to ivermectin, the current gold-standard treatment for onchocerciasis, moxidectin achieved significantly more complete and sustained microfilaridermia clearance.
Other alternative treatments for onchocerciasis include (curative) anti-Wolbachia therapies. Unlike moxidectin, which could be delivered through established community-directed treatment platforms in Africa, currently available anti-Wolbachia therapies (e.g. doxycycline) require a 4–6-week course of 100 mg per day, complicating their use in community treatment programs. Other anti-Wolbachia compounds in pre-clinical development may be efficacious with shorter (1–2-week) courses. Combination therapies using anti-Wolbachia drugs plus moxidectin could, respectively, deliver macrofilaricidal and microfilaricidal efficacy superior to the combination with ivermectin.
Moxidectin represents a promising alternative to ivermectin for the treatment and control of onchocerciasis, capable of accelerating or increasing the feasibility of elimination in endemic areas. To fulfil this potential, it is imperative that an appropriate pediatric dose and formulation of moxidectin be developed and approved.
Declaration of interest
The authors have no relevant affiliations or financial involvement with any organization or entity with a financial interest in or financial conflict with the subject matter or materials discussed in the manuscript. This includes employment, consultancies, honoraria, stock ownership or options, expert testimony, grants or patents received or pending, or royalties.
Reviewer disclosures
Peer reviewers on this manuscript have no relevant financial or other relationships to disclose.
Medicines Development for Global Health (MDGH) provided a scientific accuracy review at the request of the journal editor.
Additional information
Funding
References
- Basáñez MG, Pion SDS, Churcher TS, et al. River blindness: a success story under threat? PLoS Med. 2006;3(9):e371.
- Colebunders R, Basáñez MG, Siling K, et al. From river blindness control to elimination: bridge over troubled water. Infect Dis Poverty. 2018;7(1):21.
- Sauerbrey M, Rakers LJ, Richards FO Jr. Progress toward elimination of onchocerciasis in the Americas. Int Health. 2018;10(Suppl 1):i71–i78.
- Kyu HH, Abate D, Abate KH, et al. Global, regional, and national disability-adjusted life-years (DALYs) for 359 diseases and injuries and healthy life expectancy (HALE) for 195 countries and territories, 1990–2017: a systematic analysis for the global burden of disease study 2017. Lancet. 2018;392(10159):1859–1922.
- Fobi G, Yameogo L, Noma M, et al. Managing the fight against onchocerciasis in Africa: APOC experience. PLoS Negl Trop Dis. 2015;9(5):e0003542.
- World Health Organization. Accelerating work to overcome the global impact of neglected tropical diseases – a roadmap for implementation; 2012 [cited 2020 June 27]. Available from: https://www.who.int/neglected_diseases/NTD_RoadMap_2012_Fullversion.pdf
- African Programme for Onchocerciasis Control. Final Communiqué of the 18th session of the joint action forum (JAF) of APOC. Bujumbura, Burundi; 2012 Dec 11–13 [cited 2020 June 27]. Available from: https://www.who.int/apoc/about/structure/jaf/Final_Communique_JAF_18_English_final_with_annexes.pdf?ua=1
- World Health Organization. WHO launches global consultations for a new roadmap on neglected tropical diseases; 2019 [cited 2020 June 27]. Available from: https://www.who.int/neglected_diseases/news/WHO-launches-global-consultations-for-new-NTD-Roadmap/en/; https://www.who.int/neglected_diseases/news/NTD-Roadmap-targets-2021-2030.pdf?ua=1
- Basáñez MG, Pion SDS, Boakes E, et al. Effect of single-dose ivermectin on Onchocerca volvulus: a systematic review and meta-analysis. Lancet Infect Dis. 2008;8(5):310–322.
- Walker M, Pion SDS, Fang H, et al. The macrofilaricidal efficacy of repeated doses of ivermectin for the treatment of river blindness. Clin Infect Dis. 2017;65(12):2026–2034.
- Dadzie Y, Amazigo UV, Boatin BA, et al. Is onchocerciasis elimination in Africa feasible by 2025: a perspective based on lessons learnt from the African control programmes. Infect Dis Poverty. 2018;7(1):63.
- Katabarwa MN, Walsh F, Habomugisha P, et al. Transmission of onchocerciasis in Wadelai focus of northwestern Uganda has been interrupted and the disease eliminated. J Parasitol Res. 2012;748540:2012.
- Boussinesq M, Fobi G, Kuesel AC. Alternative treatment strategies to accelerate the elimination of onchocerciasis. Int Health. 2018;10(suppl_1):i40–8.
- Kuesel AC. Research for new drugs for elimination of onchocerciasis in Africa. Int J Parasitol Drugs Drug Resist. 2016;6(3):272–286.
- FDA. US Food & Drug Administration. Drug approval package: moxidectin; 2018 [cited 2020 June 27]. Available from: https://www.accessdata.fda.gov/drugsatfda_docs/nda/2018/210867Orig1s000TOC.cfm; https://www.accessdata.fda.gov/drugsatfda_docs/label/2018/210867lbl.pdf
- Awadzi K, Opoku NO, Attah SK, et al. A randomized, single-ascending-dose, ivermectin-controlled, double-blind study of moxidectin in Onchocerca volvulus infection. PLoS Negl Trop Dis. 2014;8(6):e2953.
- Opoku NO, Bakajika DK, Kanza EM, et al. Single dose moxidectin versus ivermectin for Onchocerca volvulus infection in Ghana, Liberia, and the Democratic Republic of the Congo: a randomised, controlled, double-blind phase 3 trial. Lancet. 2018;392(10154):1207–1216.
- Verdú JR, Cortez V, Martinez-Pinna J, et al. First assessment of the comparative toxicity of ivermectin and moxidectin in adult dung beetles: sub-lethal symptoms and pre-lethal consequences. Sci Rep. 2018;8(1):14885.
- Cotreau MM, Warren S, Ryan JL, et al. The antiparasitic moxidectin: safety, tolerability, and pharmacokinetics in humans. J Clin Pharmacol. 2003;43(10):1108–1115.
- Kinrade SA, Mason JW, Sanabria CR, et al. Evaluation of the cardiac safety of long-acting endectocide moxidectin in a randomized concentration-QT study. Clin Transl Sci. 2018;11(6):582–589.
- Korth-Bradley JM, Parks V, Patat A, et al. Relative bioavailability of liquid and tablet formulations of the antiparasitic moxidectin. Clin Pharmacol Drug Dev. 2012;1(1):32–37.
- Korth-Bradley JM, Parks V, Chalon S, et al. The effect of a high-fat breakfast on the pharmacokinetics of moxidectin in healthy male subjects: a randomized phase I trial. Am J Trop Med Hyg. 2012;86(1):122–125.
- González Canga A, Sahagún Prieto AM, Diez Liébana MJ, et al. The pharmacokinetics and interactions of ivermectin in humans–a mini-review. AAPS J. 2008;10(1):42–46.
- Prichard R, Ménez C, Lespine A. Moxidectin and the avermectins: consanguinity but not identity. Int J Parasitol Drugs Drug Resist. 2012;2:134–153.
- Dupuy J, Escudero E, Eeckhoutte C, et al. In vitro metabolism of 14C-moxidectin by hepatic microsomes from various species. Vet Res Commun. 2001;25(5):345–354.
- Korth-Bradley JM, Parks V, Wagner F, et al. Effect of moxidectin on CYP3A4 activity as evaluated by oral midazolam pharmacokinetics in healthy subjects. Clin Pharmacol Drug Dev. 2014;3(2):151–157.
- Hennessy DR, Alvinerie MR. Pharmacokinetics of the macrocyclic lactones: conventional wisdom and new paradigms. In: Vercruysse J, Rew RS, editors. Macrocyclic lactones in antiparasitic therapy. Wallingford, Oxon, UK: CAB International; 2002. p. 97–123.
- Korth-Bradley JM, Parks V, Chalon S, et al. Excretion of moxidectin into breast milk and pharmacokinetics in healthy lactating women. Antimicrob Agents Chemother. 2011;55(11):5200.
- Lespine A, Martin S, Dupuy J, et al. Interaction of macrocyclic lactones with P-glycoprotein: structure–affinity relationship. Eur J Pharm Sci. 2007;30(1):84–94.
- Griffin J, Fletcher N, Clemence R, et al. Selamectin is a potent substrate and inhibitor of human and canine P-glycoprotein. J Vet Pharmacol Ther. 2005;28(3):257–265.
- Kiki-Mvouaka S, Menez C, Borin C, et al. Role of P-glycoprotein in the disposition of macrocyclic lactones: a comparison between ivermectin, eprinomectin, and moxidectin in mice. Drug Metab Dispos. 2010;38(4):573–580.
- Perez M, Blazquez AG, Real R, et al. In vitro and in vivo interaction of moxidectin with BCRP/ABCG2. Chem Biol Interact. 2009;180(1):106–112.
- Janko C, Geyer J. Moxidectin has a lower neurotoxic potential but comparable brain penetration in P-glycoprotein-deficient CF-1 mice compared to ivermectin. J Vet Pharmacol Ther. 2013;36(3):275–284.
- Geyer J, Janko C. Treatment of MDR1 mutant dogs with macrocyclic lactones. Curr Pharm Biotechnol. 2012;13(6):969–986.
- Zhao Z, Sheps JA, Ling V, et al. Expression analysis of ABC transporters reveals differential functions of tandemly duplicated genes in Caenorhabditis elegans. J Mol Biol. 2004;344(2):409–417.
- Godoy P, Lian J, Beech RN, et al. Haemonchus contortus P-glycoprotein-2: in situ localisation and characterisation of macrocyclic lactone transport. Int J Parasitol. 2015;45(1):85–93.
- Janssen IJI, Krücken J, Demeler J, et al. Genetic variants and increased expression of Parascaris equorum P-glycoprotein-11 in populations with decreased ivermectin susceptibility. PLoS One. 2013;8(4):e61635.
- Janssen IJI, Krücken J, Demeler J, et al. Transgenically expressed Parascaris P-glycoprotein-11 can modulate ivermectin susceptibility in Caenorhabditis elegans. Int J Parasitol Drugs Drug Resist. 2015;5(2):44–47.
- Ardelli BF, Prichard RK. Inhibition of P-glycoprotein enhances sensitivity of Caenorhabditis elegans to ivermectin. Vet Parasitol. 2013;191(3–4):264–275.
- Maté L, Ballent M, Cantón C, et al. Assessment of P-glycoprotein gene expression in adult stage of Haemonchus contortus in vivo exposed to ivermectin. Vet Parasitol. 2018;264:1–7.
- Lloberas M, Alvarez L, Entrocasso C, et al. Measurement of ivermectin concentrations in target worms and host gastrointestinal tissues: influence of the route of administration on the activity against resistant Haemonchus contortus in lambs. Exp Parasitol. 2012;131(3):304–309.
- Lespine A, Ménez C, Bourguinat C, et al. P-glycoproteins and other ABC transporters in the pharmacology of anthelmintics: prospects for reversing transport-dependent anthelmintic resistance. Int J Parasitol Drugs Drug Resist. 2012;2:58–75.
- Knab J, Darge K, Büttner DW. Immunohistological studies on macrophages in lymph nodes of onchocerciasis patients after treatment with ivermectin. Trop Med Int Health. 1997;2(12):1156–1169.
- Moreno Y, Nabhan JF, Solomon J, et al. Ivermectin disrupts the function of the excretory-secretory apparatus in microfilariae of Brugia malayi. Proc Natl Acad Sci U S A. 2010;107(46):20120–20125.
- Turner HC, Walker M, Attah SK, et al. The potential impact of moxidectin on onchocerciasis elimination in Africa: an economic evaluation based on the Phase II clinical trial data. Parasit Vectors. 2015;8:167.
- Alley ES, Plaisier AP, Boatin BA, et al. The impact of five years of annual ivermectin treatment on skin microfilarial loads in the onchocerciasis focus of Asubende, Ghana. Trans R Soc Trop Med Hyg. 1994;88(5):581–584.
- Plaisier AP, Alley ES, Boatin BA, et al. Irreversible effects of ivermectin on adult parasites in onchocerciasis patients in the onchocerciasis control programme in West Africa. J Infect Dis. 1995;172(1):204–210.
- Pion SDS, Nana-Djeunga HC, Kamgno J, et al. Dynamics of Onchocerca volvulus microfilarial densities after ivermectin treatment in an ivermectin-naïve and a multi-treated population from Cameroon. PLoS Negl Trop Dis. 2013;7:e2084.
- Bottomley C, Isham V, Collins RC, et al. Rates of microfilarial production by Onchocerca volvulus are not cumulatively reduced by multiple ivermectin treatments. Parasitology. 2008;135(13):1571–1581.
- Gardon J, Boussinesq M, Kamgno J, et al. Effects of standard and high doses of ivermectin on adult worms of Onchocerca volvulus: a randomised controlled trial. Lancet. 2002;360(9328):203–210.
- Campillo JT, Chesnais CB, Pion SDS, et al. Individuals living in an onchocerciasis focus and treated three-monthly with ivermectin develop fewer new onchocercal nodules than individuals treated annually. Parasit Vectors. 2020;13(1):258.
- Collins RC, Gonzales-Peralta C, Castro J, et al. Ivermectin: reduction in prevalence and infection intensity of Onchocerca volvulus following biannual treatments in five Guatemalan communities. Am J Trop Med Hyg. 1992;47(2):156–169.
- Njongmeta LM, Nfon CK, Gilbert J, et al. Cattle protected from onchocerciasis by ivermectin are highly susceptible to infection after drug withdrawal. Int J Parasitol. 2004;34(9):1069–1074.
- Wolstenholme AJ, Maclean MJ, Coates R, et al. How do the macrocyclic lactones kill filarial nematode larvae? Invert Neurosci. 2016;16(3):7.
- Hibbs RE, Gouaux E. Principles of activation and permeation in an anion-selective Cys-loop receptor. Nature. 2011;474(7349):54–60.
- Wolstenholme A, Rogers A. Glutamate-gated chloride channels and the mode of action of the avermectin/milbemycin anthelmintics. Parasitology. 2005;131(S1):S85–S95.
- Li BW, Rush AC, Weil GJ. High level expression of a glutamate-gated chloride channel gene in reproductive tissues of Brugia malayi may explain the sterilizing effect of ivermectin on filarial worms. Int J Parasitol Drugs Drug Resist. 2014;4(2):71–76.
- Verma M, Pathak M, Shahab M, et al. Moxidectin causes adult worm mortality of human lymphatic filarial parasite Brugia malayi in rodent models. Folia Parasitol (Praha). 2014;61(6):561–570.
- Njue AI, Hayashi J, Kinne L, et al. Mutations in the extracellular domains of glutamate-gated chloride channel α3 and β subunits from ivermectin-resistant Cooperia oncophora affect agonist sensitivity. J Neurochem. 2004;89(5):1137–1147.
- Forrester SG, Beech RN, Prichard RK. Agonist enhancement of macrocyclic lactone activity at a glutamate-gated chloride channel subunit from Haemonchus contortus. Biochem Pharmacol. 2004;67(6):1019–1024.
- Storey B, Marcellino C, Miller M, et al. Utilization of computer processed high definition video imaging for measuring motility of microscopic nematode stages on a quantitative scale: ‘The Worminator’. Int J Parasitol Drugs Drug Resist. 2014;4(3):233–243.
- Lallemand E, Lespine A, Alvinerie M, et al. Estimation of absolute oral bioavailability of moxidectin in dogs using a semi-simultaneous method: influence of lipid co-administration. J Vet Pharmacol Ther. 2007;30(5):375–380.
- Awadzi K, Attah SK, Addy ET, et al. Thirty-month follow-up of sub-optimal responders to multiple treatments with ivermectin, in two onchocerciasis-endemic foci in Ghana. Ann Trop Med Parasitol. 2004;98(4):359–370.
- Frempong KK, Walker M, Cheke RA, et al. Does increasing treatment frequency address suboptimal responses to ivermectin for the control and elimination of river blindness? Clin Infect Dis. 2016;62(11):1338–1347.
- Doyle SR, Bourguinat C, Nana-Djeunga HC, et al. Genome-wide analysis of ivermectin response by Onchocerca volvulus reveals that genetic drift and soft selective sweeps contribute to loss of drug sensitivity. PLoS Negl Trop Dis. 2017;11(7):e0005816.
- Churcher TS, Pion SDS, Osei-Atweneboana MY, et al. Identifying sub-optimal responses to ivermectin in the treatment of River Blindness. Proc Natl Acad Sci U S A. 2009;106(39):16716–16721.
- Prichard RK, Geary TG. Perspectives on the utility of moxidectin for the control of parasitic nematodes in the face of developing anthelmintic resistance. Int J Parasitol Drugs Drug Resist. 2019;10:69–83.
- Doyle SR, Illingworth CJR, Laing R, et al. Population genomic and evolutionary modelling analyses reveal a single major QTL for ivermectin drug resistance in the pathogenic nematode, Haemonchus contortus. BMC Genomics. 2019;20(1):218.
- Hodgkinson JE, Kaplan RM, Kenyon F, et al. Refugia and anthelmintic resistance: concepts and challenges. Int J Parasitol Drugs Drug Resist. 2019;10:51–57.
- Brody T. Clinical trials. Second edition. Study design, endpoints and biomarkers, drug safety, and FDA and ICH guidelines. Academic Press, Elsevier; 2016.
- Francis H, Awadzi K, Ottesen E. The Mazzotti reaction following treatment of onchocerciasis with diethylcarbamazine: clinical severity as a function of infection intensity. Am J Trop Med Hyg. 1985;34(3):529–536.
- Gardon J, Gardon-Wendel N. Demanga-Ngangue, et al. Serious reactions after mass treatment of onchocerciasis with ivermectin in an area endemic for Loa loa infection. Lancet. 1997;350(9070):18–22.
- Herrick JA, Legrand F, Gounoue R, et al. Posttreatment reactions after single-dose diethylcarbamazine or ivermectin in subjects with Loa loa infection. Clin Infect Dis. 2017;64(8):1017–1025.
- Katabarwa MN, Eyamba A, Nwane P, et al. Fifteen years of annual mass treatment of onchocerciasis with ivermectin have not interrupted transmission in the West region of Cameroon. J Parasitol Res. 2013;420928:2013.
- Eisenbarth A, Achukwi MD, Renz A. Ongoing transmission of Onchocerca volvulus after 25 years of annual ivermectin mass treatments in the Vina du Nord River Valley, in North Cameroon. PLoS Negl Trop Dis. 2016;10(2):e0004392.
- Komlan K, Vossberg PS, Gantin RG, et al. Onchocerca volvulus infection and serological prevalence, ocular onchocerciasis and parasite transmission in northern and central Togo after decades of Simulium damnosum s.l. vector control and mass drug administration of ivermectin. PLoS Negl Trop Dis. 2018;12(3):e0006312.
- Duamor CT, Datchoua-Poutcheu FR, Ndongmo WPC, et al. Programmatic factors associated with the limited impact of Community-Directed Treatment with Ivermectin to control onchocerciasis in three drainage basins of South West Cameroon. PLoS Negl Trop Dis. 2017;11(11):e0005966.
- Brieger WR, Okeibunor JC, Abiose AO, et al. Compliance with eight years of annual ivermectin treatment of onchocerciasis in Cameroon and Nigeria. Parasit Vectors. 2011;4(1):1.
- Osue HO. Field-based evidence of single and few doses of annual ivermectin treatment efficacy in eliminating skin microfilaria load after a decade of intervention. Ethiop J Health Sci. 2017;27(2):129–138.
- Wanji S, Kengne-Ouafo JA, Esum ME, et al. Relationship between oral declaration on adherence to ivermectin treatment and parasitological indicators of onchocerciasis in an area of persistent transmission despite a decade of mass drug administration in Cameroon. Parasit Vectors. 2015;8(1):667.
- Kamga GR, Dissak-Delon FN, Nana-Djeunga HC, et al. Audit of the community-directed treatment with ivermectin (CDTI) for onchocerciasis and factors associated with adherence in three regions of Cameroon. Parasit Vectors. 2018;11(1):356.
- Turner HC, Churcher TS, Walker M, et al. Uncertainty surrounding projections of the long-term impact of ivermectin treatment on human onchocerciasis. PLoS Negl Trop Dis. 2013;7(4):e2169.
- Stolk WA, Walker M, Coffeng LE, et al. Required duration of mass ivermectin treatment for onchocerciasis elimination in Africa: a comparative modelling analysis. Parasit Vectors. 2015;8(1):552.
- Prost A, Hervouet JP, Thylefors B. Les niveaux d’endémicité dans l’onchocercose. Bull World Health Organ. 1979;57(4):655–662.
- Katabarwa MN, Eyamba A, Chouaibou M, et al. Does onchocerciasis transmission take place in hypoendemic areas? A study from the North Region of Cameroon. Trop Med Int Health. 2010;15(5):645–652.
- Kelly-Hope LA, Unnasch TR, Stanton MC, et al. Hypo-endemic onchocerciasis hotspots: defining areas of high risk through micro-mapping and environmental delineation. Infect Dis Poverty. 2015;4(1):36.
- Cantey PT, Roy SL, Boakye D, et al. Transitioning from river blindness control to elimination: steps toward stopping treatment. Int Health. 2018;10(Suppl 1):i7–13.
- Kamgno J, Pion SD, Chesnais CB, et al. A test-and-not-treat strategy for onchocerciasis in Loa loa-endemic areas. N Engl J Med. 2017;377(21):2044–2052.
- Pion SD, Tchatchueng-Mbougua JB, Chesnais CB, et al. Effect of a single standard dose (150–200 μg/kg) of ivermectin on Loa loa microfilaremia: systematic review and meta-analysis. Open Forum Infect Dis. 2019;6(4):ofz019.
- Tamarozzi F, Halliday A, Gentil K, et al. Onchocerciasis: the role of Wolbachia bacterial endosymbionts in parasite biology, disease pathogenesis, and treatment. Clin Microbiol Rev. 2011;24(3):459–468.
- Taylor MJ, Hoerauf A, Townson S, et al. Anti-Wolbachia drug discovery and development: safe macrofilaricides for onchocerciasis and lymphatic filariasis. Parasitology. 2014;141(1):119–127.
- Sulaiman WAW, Kamtchum-Tatuene J, Mohamed MH, et al. Anti-Wolbachia therapy for onchocerciasis & lymphatic filariasis: current perspectives. Indian J Med Res. 2019;149(6):706–714.
- Walker M, Specht S, Churcher TS, et al. Therapeutic efficacy and macrofilaricidal activity of doxycycline for the treatment of river blindness. Clin Infect Dis. 2015;60(8):1199–1207.
- Albers A, Esum ME, Tendongfor N, et al. Retarded Onchocerca volvulus L1 to L3 larval development in the Simulium damnosum vector after anti-wolbachial treatment of the human host. Parasit Vectors. 2012;5:12.
- Bosshardt SC, McCall JW, Coleman SU, et al. Prophylactic activity of tetracycline against Brugia pahangi infection in jirds (Meriones unguiculatus). J Parasitol. 1993;79(5):775–777.
- Taylor MJ, Bandi C, Hoerauf A. Wolbachia bacterial endosymbionts of filarial nematodes. Adv Parasitol. 2005;60:245–284.
- Turner JD, Tendongfor N, Esum M, et al. Macrofilaricidal activity after doxycycline only treatment of Onchocerca volvulus in an area of Loa loa co-endemicity: a randomized controlled trial. PLoS Negl Trop Dis. 2010;4(4):e660.
- Wanji S, Tendongfor N, Nji T, et al. Community-directed delivery of doxycycline for the treatment of onchocerciasis in areas of co-endemicity with loiasis in Cameroon. Parasit Vectors. 2009;2(1):39.
- Tamarozzi F, Tendongfor N, Enyong PA, et al. Long term impact of large scale community-directed delivery of doxycycline for the treatment of onchocerciasis. Parasit Vectors. 2012;5:53.
- Debrah AY, Specht S, Klarmann-Schulz U, et al. Doxycycline leads to sterility and enhanced killing of female Onchocerca volvulus worms in an area with persistent microfilaridermia after repeated ivermectin treatment: a randomized, placebo-controlled, double-blind trial. Clin Infect Dis. 2015;61(4):517–526.
- Hoerauf A, Mand S, Adjei O, et al. Depletion of Wolbachia endobacteria in Onchocerca volvulus by doxycycline and microfilaridermia after ivermectin treatment. Lancet. 2001;357(9266):1415–1416.
- Turner HC, Walker M, Pion SDS, et al. Economic evaluations of onchocerciasis interventions: a systematic review and research needs. Trop Med Int Health. 2019;24(7):788–816.
- Walker M, Churcher TS, Wanji S, et al. The cost-effectiveness of doxycycline therapy for the control of human onchocerciasis in areas coendemic with loiasis. Am J Trop Med Hyg. 2012;87(5 Suppl):144. Presented at: American Society of Tropical Medicine and Hygiene 61st Annual Meeting; 2012 November 11 –15;Atlanta.
- Tan KR, Magill AJ, Parise ME, et al. Doxycycline for malaria chemoprophylaxis and treatment: report from the CDC expert meeting on malaria chemoprophylaxis. Am J Trop Med Hyg. 2011;84(4):517–531.
- Gaillard T, Briolant S, Madamet M, et al. The end of a dogma: the safety of doxycycline use in young children for malaria treatment. Malar J. 2017;16(1):148.
- Wanji S, Nji TM, Hamill L, et al. Implementation of test-and-treat with doxycycline and temephos ground larviciding as alternative strategies for accelerating onchocerciasis elimination in an area of loiasis co-endemicity: the COUNTDOWN consortium multi-disciplinary study protocol. Parasit Vectors. 2019;12(1):574.
- Edwards H. Use of an individual-based onchocerciasis transmission model (EPIONCHO-IBM) to predict the impact of a doxycycline test-and-treat intervention on infection prevalence and intensity in endemic communities of south-western Cameroon. MRes Biomedical Sciences thesis, Imperial College London; 2018.
- International Standard Randomised Controlled Trials Number (ISRCTN) Registry. The efficacy of rifapentine plus moxifloxacin against onchocerciasis: a randomized, open label pilot trial. ISRCTN; 2018 [cited 2020 June 27]. DOI:10.1186/ISRCTN43697583
- Klarmann-Schulz U, Specht S, Debrah AY, et al. Comparison of doxycycline, minocycline, doxycycline plus albendazole and albendazole alone in their efficacy against onchocerciasis in a randomized, open-label, pilot trial. PLoS Negl Trop Dis. 2017;11(1):e0005156.
- Aljayyoussi G, Tyrer HE, Ford L, et al. Short-course, high-dose rifampicin achieves Wolbachia depletion predictive of curative outcomes in preclinical models of lymphatic filariasis and onchocerciasis. Sci Rep. 2017;7(1):210. Erratum in Sci Rep. 8(1),1384 (2018).
- Turner JD, Sharma R, Al Jayoussi G, et al. Albendazole and antibiotics synergize to deliver short-course anti-Wolbachia curative treatments in preclinical models of filariasis. Proc Natl Acad Sci U S A. 2017;114(45):E9712–21.
- Hong WD, Benayoud F, Nixon GL, et al. AWZ1066S, a highly specific anti-Wolbachia drug candidate for a short-course treatment of filariasis. Proc Natl Acad Sci U S A. 2019;116(4):1414–1419. .
- Drugs for Neglected Diseases initiative (DNDi). R&D portfolio in review: Filaria – river blindness (2020); 2019 [cited 2020 June 27]. Available from: https://dndi.org/news/2020/filarialdiseases-riverblindness-rnd-portfolio-update/
- Taylor MJ, von Geldern TW, Ford L, et al. Preclinical development of an oral anti-Wolbachia macrolide drug for the treatment of lymphatic filariasis and onchocerciasis. Sci Transl Med. 2019;11(483):pii: eaau2086.
- Agwuh KN, MacGowan A. Pharmacokinetics and pharmacodynamics of the tetracyclines including glycylcyclines. J Antimicrob Chemother. 2006;58(2):256–265.
- US National Library of Medicine. Safety and efficacy of annual or biannual doses of moxidectin or ivermectin for onchocerciasis. National Institutes of Health; 2020 [cited 2020 June 27]. Available from: https://clinicaltrials.gov/ct2/show/NCT03876262
- US National Library of Medicine. A pharmacokinetic and safety study of moxidectin to identify an optimal dose for treatment of children 4 to 11 years. National Institutes of Health; 2019 [cited 2020 June 27]. Available from: https://clinicaltrials.gov/ct2/show/NCT03962062
- US National Library of Medicine. Clinical trial evaluating the safety and efficacy of moxidectin 2 mg ivermectin-controlled in loa loa microfilaremic patients (EOLoa). National institutes of Health; 2019 [cited 2020 June 27]. Available from: https://clinicaltrials.gov/ct2/show/NCT04049851
- Jacob BG, Loum D, Lakwo TL, et al. Community-directed vector control to supplement mass drug distribution for onchocerciasis elimination in the Madi mid-North focus of Northern Uganda. PLoS Negl Trop Dis. 2018;12(8):e0006702.