ABSTRACT
Introduction
Nontuberculous mycobacteria infect patients who have structural lung disease or those who are immunocompromised. Nontuberculous mycobacterial pulmonary disease (NTM-PD) is increasing in prevalence. Treatment guidelines for Mycobacterium avium complex (MAC) pulmonary disease involve a three-drug regimen with azithromycin, ethambutol, and rifampin, and those of Mycobacterium abscessus complex (MAB) pulmonary disease involve a combination of three or more antimicrobials including macrolides, amikacin, and a β-lactam or imipenem. However, these regimens are poorly tolerated and generally ineffective.
Areas covered
SPR720 is a novel therapeutic agent that has demonstrated activity against a range of NTM species, including MAC and MAB. Encouraging in vitro and pre-clinical data demonstrate that SPR720 is active both alone and in combination with standard-of-care agents, with no evidence of cross-resistance to such agents. It is generally well tolerated with mainly gastrointestinal and headache adverse events of mild or moderate severity.
Expert opinion
Management of NTM-PD is challenging for many reasons including length of therapy, poor efficacy, drug intolerance, recurrence, and resistance development. The current antimicrobial management options for NTM-PD are limited in number and there exists a large unmet need for new treatments. SPR720 has encouraging data that warrant further study in the context of a multidrug regimen.
1. Introduction
Nontuberculous mycobacteria (NTM) are a diverse group of organisms that are ubiquitous in the environment [Citation1]. More than 200 NTM species and subspecies have been identified to date [Citation2] but only a small percentage are recognized to cause human infection. The vast majority (80–85%) of NTM pulmonary disease (NTM-PD) is caused by Mycobacterium avium complex (MAC), with Mycobacterium abscessus complex (MAB) and Mycobacterium kansasii comprising the remainder [Citation3], and species such as Mycobacterium malmoense and Mycobacterium xenopi prevalent in other regions of the world (namely Europe and Canada) [Citation4,Citation5].
NTM-PD prevalence is increasing worldwide [Citation3,Citation6], and given that risk is greater in older individuals [Citation7–10], it is expected that larger numbers of patients will acquire these infections from the environment as the population ages. Underlying bronchiectasis is the strongest risk factor for NTM-PD, although immunosuppression and underlying architectural lung disease such as chronic obstructive pulmonary disease, pneumonia, and asthma also predispose to disease [Citation8,Citation11–15].
Treatment of MAC pulmonary disease (MAC-PD) is well articulated by guidelines [Citation16,Citation17]; however, success of therapy and tolerability are unsatisfactory. A three-drug regimen with azithromycin, ethambutol, and rifampin is recommended for all patients initiating therapy. ‘Cure’ is elusive, particularly in the setting of bronchiectasis, and only 60–70% of individuals convert their sputum cultures to negative within the first six months of therapy [Citation18,Citation19]. Further, even after treatment success has been achieved (defined as completion of 12 months treatment post-culture conversion to negative), approximately half of those will become culture positive within three years [Citation20]. This return to culture positivity represents both new infection from the environment as well as relapse of the original infection [Citation21–23]. The tolerability of this regimen is poor, and population-based data suggest that less than half of patients who start triple-drug therapy are still using this regimen at six months after treatment initiation [Citation24]; further attrition is evident and early therapy discontinuation is common [Citation24–28].
Replacement for these recommended three drugs is limited, as only clofazimine has observational data supporting its use in this context [Citation29–31]. Additionally, access to this drug is limited in the United States as it is only available within a managed access program offered by the manufacturer. Fluoroquinolones have little to no activity against MAC and are rarely useful in the treatment of MAC-PD [Citation32]. Intravenous (IV) and inhaled forms of amikacin, including amikacin liposome inhalation suspension (ALIS), have good activity against MAC and are used initially in the treatment of cavitary disease (IV amikacin) or after failure of culture conversion (‘refractory disease’) within six months of therapy [Citation16,Citation17]. The need for new therapies against MAC, as well as MAB, for which there are essentially no active oral therapies for most isolates beyond clofazimine, cannot be understated.
The treatment armamentarium for NTM-PD includes a very limited number of therapeutic options and regimens are poorly tolerated and generally ineffective. Beyond clinicians, regulatory bodies and industry have realized the need for therapeutic development within the indication of NTM-PD. The FDA convened a workshop in April 2019 to discuss challenges and considerations related to the treatment of NTM-PD and the expert panelists agreed that there was a great need for new treatment options [Citation33]. Limited therapeutic options for specific NTM species and the emergence of multidrug-resistant strains pose challenges in achieving successful treatment outcomes. Addressing these limitations requires ongoing research and development efforts to improve treatment outcomes for NTM-PD. This includes the development of novel oral antimicrobial agents with improved safety, tolerability, spectrum of activity, efficacy over shorter time periods, and minimal drug-drug interactions [Citation34,Citation35]. Drugs that are currently under development for NTM-PD that have reached clinical trials include ALIS, clofazimine, omadacycline, epetraborole, and SPR720 (). This review focuses on the attributes of SPR720 and a proposed development pathway in the treatment of NTM-PD.
Table 1. NTM treatments under investigation.
2. SPR720
SPR720 is an oral agent that was granted Qualified Infectious Disease Product (QIDP), fast track, and orphan drug designations by the FDA, and is being developed initially for the treatment of NTM-PD due to MAC. It is a chemically stable phosphate ester prodrug that is converted rapidly in vivo to SPR719, which is the active moiety. The chemical formulae of SPR720 and SPR719 are C21H26FN6O6P and C21H25FN6O3, respectively, and the structural formulae can be found in . SPR719 is a novel aminobenzimidazole antibiotic that inhibits in mycobacteria the ATPase activity of DNA gyrase B (GyrB) [Citation36–39], a subunit of the tetrameric gyrase A2B2 bacterial topoisomerase that catalyzes ATP-dependent negative DNA supercoiling and DNA decatenation [Citation40–43]. DNA gyrase () is the sole complex with topoisomerase activity in mycobacteria, so inhibiting ATPase activity can impede the growth of a range of these pathogens [Citation44]. As such, DNA gyrase is recognized as a clinically validated target for NTM infections [Citation38,Citation45].
Figure 2. Bacterial DNA gyrase is composed of two GyrA subunits (light blue) and two GyrB subunits, which contain an ATPase (yellow), a transducer domain (green), and a TOPRIM domain (dark blue). A double stranded DNA segment (black) is captured, and, after ATP binding, the ATPase domains dimerize and the DNA strand gets trapped. ATP hydrolysis, double-strand cleavage, and strand translocation follow and then strand religation and dissociation of the ATPase domains occurs to reset the enzyme for future activity (Stokes, Vemula et al. 2020).
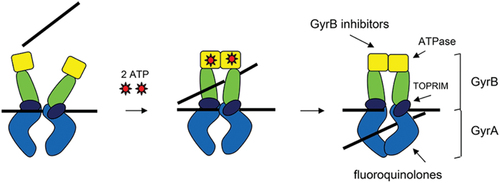
The mechanism of action of SPR719 is distinct from that of fluoroquinolones, which inhibit DNA gyrase A (GyrA) [Citation38,Citation46]. As such, there is no cross-resistance with fluoroquinolones or other standard-of-care (SOC) agents; this has been corroborated with in vitro surveillance data from NTM clinical isolates [Citation43].
2.1. In vitro activity of SPR719 against clinically relevant mycobacteria
Several studies have shown that SPR719 is active in vitro against isolates from some of the major NTM organisms including MAC and MAB with a MIC range of 0.002–4 µg/mL and 0.03–8 µg/mL, respectively [Citation36,Citation47,Citation48], as shown in . Its potent antimycobacterial activity is comparable or better than a range of other antimicrobials, however, not all are universally used for NTM infections. The comparator agents were clarithromycin, rifabutin, rifampin, amikacin, doxycycline, linezolid, trimethoprim/sulfamethoxazole, moxifloxacin, and ciprofloxacin across clinically relevant mycobacterial species in vitro, including MAC (M. avium, Mycobacterium intracellulare, Mycobacterium chimaera), MAB (subspecies M. abscessus, Mycobacterium massiliense, M. massiliense hybrid), M. kansasii, Mycobacterium ulcerans, Mycobacterium marinum, and M. chimaera, with no evidence of cross-resistance to other SOC agents [Citation36,Citation43,Citation48,Citation50]. SPR719 demonstrates potent activity against MAC (MIC50 of 0.5–1 µg/mL and MIC90 of 1–2 µg/mL) and M. kansasii (MIC50 of <0.015 µg/mL and MIC90 of 0.03 µg/mL) and good activity against MAB (MIC50 of 2 µg/mL and MIC90 of 2–4 µg/mL) [Citation43]; its activity was concentration-dependent and considered bacteriostatic for MAC and MAB and bactericidal for M. kansasii [Citation49].
Table 2. MIC ranges, MIC50 values, and MIC90 values of SPR719 against different NTM speciesa.
Studies also demonstrate activity against contemporary clinical isolates resistant to current therapeutics such as clarithromycin, fluoroquinolones, amikacin, cefoxitin, and imipenem [Citation36,Citation43,Citation47,Citation51]. Furthermore, the potential for combination therapy with ethambutol against MAC and M. kansasii or synergy with clarithromycin against M. abscessus (mean fractional inhibitory concentration index of 0.8 for each) has been demonstrated [Citation49].
Characterization of in vitro activity is a first step in the profiling of resistance properties of novel drug candidates. Susceptibility studies have confirmed that SPR719 exhibited consistent activity against both MAC and MAB isolates that were resistant to SOC agents. Laboratory studies have identified spontaneous mutants in the ATPase domain of GyrB of MAC displaying resistance to SPR719 at low frequency (10−8/CFU) at concentrations 2 × MIC of SPR719 but not at higher concentrations. For MAB, but not for MAC, a second resistance mechanism at a frequency of 10−6/CFU, associated with frameshift mutations in the transcriptional repressor MAB_4384, was observed. Finally, resistant mutants did not exhibit cross-resistance to clarithromycin or moxifloxacin, indicating that resistance is specific to SPR719 and not due to a multidrug-resistance mechanism [Citation51]. In the clinic, this will be further de-risked knowing that NTM-PD is treated with combination agents.
2.2. Various nonclinical models support the efficacy of SPR719/SPR720 in NTM-PD
SPR719 showed significant microbial kill in a hollow fiber system model of pulmonary intracellular MAC using a pharmacokinetic (PK) profile that supports once-daily dosing of SPR720 in humans [Citation52]. Penetration of SPR719 into THP-1 monocytes was demonstrated. Moreover, SPR720 doses that are currently being evaluated in the ongoing Phase 2a clinical trial were predicted to achieve exposures associated with bactericidal effect in 95% of patients.
In a 60-day chronic C3HeBFeJ mouse infection model, SPR720 monotherapy caused a dose-dependent reduction in pulmonary M. avium ATCC 700898 burden at 10–100 mg/kg/day in comparison to the untreated control group. The combination of SPR720, clarithromycin, and ethambutol demonstrated the greatest reduction in the average mycobacterial burden in the lungs, spleen, and liver in this model [Citation53].
SPR720 was also evaluated in a 17-day acute prolonged severe combined immunodeficiency (SCID) mouse model of infection with M. abscessus subspecies boletti 103. SPR720 significantly reduced the mycobacterial burden in the lungs, spleen, and liver after oral dosages of 25–400 mg/kg/day for 16 days. While dose-dependent efficacy was observed, a dose level of 100 mg/kg/day resulted in the greatest reduction in M. abscessus burden in the lungs, spleen, and liver compared with that in the control group [Citation48].
Finally, the efficacy of SPR720 dosed at 25, 50, and 100 mg/kg/day, and comparator agents clarithromycin, amikacin, and clofazimine, dosed both alone and in combination with SPR720, was assessed in a 60-day chronic murine model of pulmonary and systemic infection caused by M. abscessus subspecies bolletii 1513. The SPR720 treatment group of 100 mg/kg/day resulted in the greatest bacterial reduction in the lung when given as a single agent, but the greatest bacterial reduction in the lung was achieved when SPR720 was combined with clarithromycin, amikacin, and clofazimine [Citation47].
2.3. Phase 1 randomized, double-blind, placebo-controlled trial
SPR720 was evaluated in a phase 1, randomized, double-blind, placebo-controlled, single-center, first-in-human study (NCT03796910) to assess safety, tolerability, and PK [Citation54]. A total of 96 healthy males and females aged 18–55 years participated in this two-part, multi-cohort study. Each subject was assigned to only one cohort and each cohort enrolled eight subjects, randomized (3:1) to receive SPR720 or placebo as single ascending doses (SAD) ranging from 100 to 2000 mg daily, including a second 500-mg dose group of healthy elderly subjects aged ≥65 years (n = 56) or multiple ascending doses doses (MAD) ranging from 500 to 1500 mg daily for seven or 14 days (n = 40).
The primary objective was to investigate the safety and tolerability in both the SAD and MAD cohorts. A full list of treatment-emergent adverse events (TEAEs) can be found in . TEAEs were reported by 42.9% of subjects who received SPR720 across the SAD cohorts. Higher doses of SPR720 led to higher reporting of TEAEs compared to lower doses (e.g. 2000 mg dose, 100%; 1500 mg, 66.7%; 1000 mg, 33.3%; 100 mg, 16.7%). Across the MAD cohorts, 60.0% of SPR720 recipients reported TEAEs. The proportion of subjects reporting TEAEs in the seven-day dose cohorts (500 mg, 66.7%; 1000 mg, 100%; 1500 mg, 66.7%) was greater than in the 14-day cohorts (500 mg, 16.7%; 1000 mg, 50.0%), which likely represents improved tolerability with continued dosing. All TEAEs were mild or moderate in severity and tended to be either gastrointestinal in nature (nausea, vomiting, and diarrhea) or headaches. No serious TEAEs were reported, and no deaths occurred.
Table 3. Incidence of treatment-emergent adverse events following oral administration of single and multiple ascending doses of SPR720 in healthy volunteers.
Secondary objectives were to investigate the PK as well as any effects of food or age on PK in the SAD groups. The mean plasma SPR719 concentrations increased with increasing dose of SPR720 (). The median SPR719 Tmax ranged from 2.8–8.0 hours across cohorts, and the mean t1/2 ranged from 2.9–4.5 hours. Cmax was independent of dose. Dosing with a high-fat meal decreased SPR719 plasma exposure (Cmax, AUClast, and AUCinf) by approximately 20% compared to the fasting state () and there was also a trend toward greater exposure of SPR719 in healthy subjects ≥65 years versus younger subjects.
Figure 3. Geometric mean plasma SPR719 concentration-time curves following single ascending doses of SPR720.
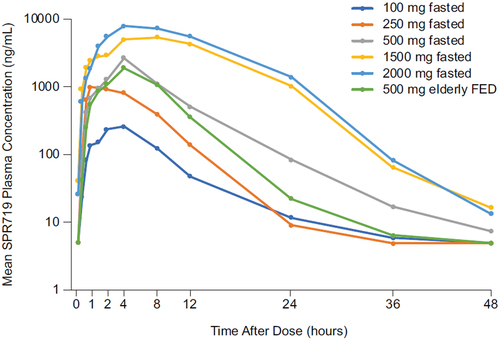
Table 4. Food effect on the PK of SPR719 following single oral 1000 mg doses of SPR720 in healthy volunteers.a
In the MAD cohorts, SPR719 plasma concentrations increased with increasing dose of SPR720. SPR719 plasma Cmax and AUC 0–24 increased in a greater-than-dose-proportional manner with increasing doses of SPR720 (). SPR719 plasma exposure declined approximately 40% between days 1 and 7, suggesting induction of an elimination pathway. However, this seemed to stabilize after day 7 as AUC0–24 was comparable between days 7 and 14. SPR719 trough plasma concentrations also stabilized by the end of dosing ().
Table 5. Summary of SPR719 PK parameters following oral administration of multiple ascending doses of SPR720 in healthy volunteers.
Table 6. Trough concentrations of SPR719 by dose following oral administration of multiple ascending doses of SPR720 in healthy volunteers.
SPR720 was rapidly and extensively converted to SPR719 in vivo. Overall, these results suggest that predicted therapeutic exposures of SPR719 can be attained with a once-daily oral administration of SPR720 [Citation54].
2.4. Phase 2a dose-ranging clinical trial
SPR720 is currently being evaluated in a phase 2a, randomized, double-blind, placebo-controlled, multicenter, dose-ranging study (NCT05496374) to evaluate the efficacy, safety, tolerability, and PK of SPR720 for the treatment of adult patients with MAC-PD. Double-blind treatment assignments will be SPR720 500 mg, SPR720 1000 mg, or placebo orally once daily for 56 days, in addition to one open-label intensive PK arm with a treatment of SPR720 1000 mg orally once daily for 56 days. The estimated enrollment is 31 patients.
3. Conclusion
NTM infection is increasingly common especially among individuals with structural lung disease or immunocompromised conditions. NTM-PD may be due to a range of mycobacterial species with MAC being the most common. Management of this disease can be difficult due to limited effectiveness, poor tolerability, the long duration of SOC treatment, and the paucity of anti-NTM drugs in development. Currently SPR720 shows promise based on its activity against a range of NTM species including strains resistant to SOC agents. Clinical trials are ongoing.
4. Expert opinion
NTM-PD is increasing in prevalence, especially among those with underlying structural lung disease such as bronchiectasis, COPD, or cystic fibrosis, and those with compromised immune systems. Expert Societies have published guidelines on the management of this disease. Treatment for MAC-PD involves a three-drug regimen including a macrolide and ethambutol for a minimum of 12 months after sputum cultures convert from positive to negative, whereas treatment of MAB-PD involves a combination of three or more antimicrobials including macrolides, amikacin, and a β-lactam or imipenem. Despite treatment guidelines, only half of diagnosed patients begin guideline-based therapy with only 18% continuing at 12 months. Adherence rates decline further at 18 months. The occurrence of a wide range of adverse events have been reported by patients with NTM-PD. The complexity of the regimen can lead to challenges identifying which drug incites these events. It is also important to note that the occurrence of these events is often a factor of age. Further, several of the currently available drugs are metabolized in such a way that drug-drug interactions can be common and manifest as other adverse effects.
In addition to the physiological and mental burden associated with NTM-PD, it is not surprising that patients struggle with therapeutic strategies. Over-arching these individual complexities, resistance to some of the recommended agents is emerging which can lead to uncertainty regarding empirical management. Moreover, even in patients who receive ‘appropriate’ therapy, the clinical success of multidrug therapy can be unpredictable. Among those patients with susceptible strains, sputum conversion can occur in less than two-thirds of patients, while in those with resistant isolates conversion occurs in less than 20%. Recurrence can occur even in those whose sputum converted with two subgroups of recurrence, genuine relapse and reinfection.
In summary, the current antimicrobial management options for NTM-PD are limited in number, with poor tolerability, adherence, and efficacy contributing to the challenges of therapy. There exists a large unmet need with regard to NTM therapeutics, as NTM-PD continues to increase in prevalence. The aminobenzimidazole, SPR720, has demonstrated potential activity in in vitro and pre-clinical models and is currently in phase 2 proof-of-concept study with MAC-PD. If a clinically meaningful signal is detected, then further study incorporating this agent in the context of a multidrug regimen should be undertaken.
Article highlights
Nontuberculous mycobacteria, including MAC and MAB, can cause pulmonary infections that are increasing in prevalence.
Underlying lung disease such as bronchiectasis or chronic obstructive pulmonary disease, immunosuppression, and older age are the biggest risk factors.
Management of NTM-PD is clinically challenging due to the long duration of complex multidrug antibiotic regimens, poor efficacy, recurrence, and resistance development.
Current therapies are poorly tolerated, which often leads to early treatment discontinuation.
SPR720 is a novel oral aminobenzimidazole that inhibits in mycobacteria the ATPase activity of DNA gyrase B.
This agent has demonstrated activity against MAC and MAB both alone and in combination with standard-of-care agents in vitro and in pre-clinical models.
SPR720 was well tolerated in a phase 1 randomized, double-blind, placebo-controlled trial with mainly mild or moderately severe gastrointestinal or headache adverse events.
SPR720 is currently in phase 2 clinical studies.
Declaration of interest
KL Winthrop has received research support from AN2 Therapeutics, Insmed, Paratek Pharmaceuticals, RedHill Biopharma, Renovion, and Spero Therapeutics, and is a consultant for AN2 Therapeutics, Insmed, Paratek Pharmaceuticals, RedHill Biopharma, Renovion, and Spero Therapeutics. P Flume has received grant support from AbbVie, AN2 Therapeutics, Armata Pharmaceuticals, AstraZeneca, Cystic Fibrosis Foundation Therapeutics, Insmed, Janssen Pharmaceuticals, National Institutes of Health, Novovax, RedHill Biopharma, Sound Pharmaceuticals, Spero Therapeutics, Synchrony Bio, and Vertex Pharmaceuticals; and is a consultant for AN2 Therapeutics, Chiesi Farmaceutici, Eloxx Pharmaceuticals, Insmed, Ionis Pharmaceuticals, Janssen Research & Development, McKesson, Sionna Therapeutics, Spero Therapeutics, and Vertex Pharmaceuticals. KA Hamed is an employee of Spero Therapeutics. The authors have no other relevant affiliations or financial involvement with any organization or entity with a financial interest in or financial conflict with the subject matter or material discussed in the manuscript apart from those disclosed.
Reviewer disclosures
Peer reviewers on this manuscript have no relevant financial or other relationships to disclose.
Additional information
Funding
References
- Falkinham JO. 3rd. Environmental sources of nontuberculous mycobacteria. Clin Chest Med. 2015 Mar;36(1):35–41. doi: 10.1016/j.ccm.2014.10.003
- LPSN. Genus Mycobacterium Sudbury, Massachusetts2023. [cited 2023 Jun 6]. Available from: https://lpsn.dsmz.de/genus/mycobacterium
- Prevots DR, Marras TK. Epidemiology of human pulmonary infection with nontuberculous mycobacteria: a review. Clin Chest Med. 2015 Mar;36(1):13–34. doi: 10.1016/j.ccm.2014.10.002
- Hoefsloot W, van Ingen J, Andrejak C, et al. The geographic diversity of nontuberculous mycobacteria isolated from pulmonary samples: an NTM-NET collaborative study. Eur Respir J. 2013 Dec;42(6):1604–1613. doi: 10.1183/09031936.00149212
- Zweijpfenning SMH, Ingen JV, Hoefsloot W. Geographic distribution of nontuberculous mycobacteria isolated from clinical specimens: a systematic review. Semin Respir Crit Care Med. 2018 Jun;39(3):336–342. doi: 10.1055/s-0038-1660864
- Dahl VN, Molhave M, Floe A, et al. Global trends of pulmonary infections with nontuberculous mycobacteria: a systematic review. Int J Infect Dis. 2022 Dec;125:120–131. doi: 10.1016/j.ijid.2022.10.013
- Marras TK, Campitelli MA, Kwong JC, et al. Risk of nontuberculous mycobacterial pulmonary disease with obstructive lung disease. Eur Respir J. 2016 Sep;48(3):928–931. doi: 10.1183/13993003.00033-2016
- Winthrop KL, Marras TK, Adjemian J, et al. Incidence and prevalence of nontuberculous mycobacterial lung disease in a large U.S managed care Health plan, 2008-2015. Ann Am Thorac Soc. 2020 Feb;17(2):178–185. doi: 10.1513/AnnalsATS.201804-236OC
- Wang PH, Pan SW, Wang SM, et al. The impact of nontuberculous mycobacteria species on mortality in patients with nontuberculous mycobacterial lung disease. Front Microbiol. 2022;13:909274. doi: 10.3389/fmicb.2022.909274
- Huang HL, Cheng MH, Lu PL, et al. Epidemiology and predictors of NTM pulmonary infection in Taiwan - a retrospective, five-year multicenter study. Sci Rep. 2017 Nov 24;7(1):16300. doi: 10.1038/s41598-017-16559-z
- Zhu YN, Xie JQ, He XW, et al. Prevalence and clinical characteristics of nontuberculous mycobacteria in patients with Bronchiectasis: a systematic review and meta-analysis. Respiration. 2021;100(12):1218–1229. doi: 10.1159/000518328
- Chu H, Zhao L, Xiao H, et al. Prevalence of nontuberculous mycobacteria in patients with bronchiectasis: a meta-analysis. Arch Med Sci. 2014 Aug 29;10(4):661–668. doi: 10.5114/aoms.2014.44857
- Zhou Y, Mu W, Zhang J, et al. Global prevalence of non-tuberculous mycobacteria in adults with non-cystic fibrosis bronchiectasis 2006-2021: a systematic review and meta-analysis. BMJ Open. 2022 Aug 1;12(8):e055672. doi: 10.1136/bmjopen-2021-055672
- Griffith DE, Aksamit T, Brown-Elliott BA, et al. An official ATS/IDSA statement: diagnosis, treatment, and prevention of nontuberculous mycobacterial diseases. Am J Respir Crit Care Med. 2007 Feb 15;175(4):367–416. doi: 10.1164/rccm.200604-571ST
- Chan ED, Iseman MD. Underlying host risk factors for nontuberculous mycobacterial lung disease. Semin Respir Crit Care Med. 2013 Feb;34(1):110–123. doi: 10.1055/s-0033-1333573
- Daley CL, Iaccarino JM, Lange C, et al. Treatment of nontuberculous mycobacterial pulmonary disease: an official ATS/ERS/ESCMID/IDSA clinical practice guideline. Clin Infect Dis. 2020 Aug 14;71(4):905–913. doi: 10.1093/cid/ciaa1125
- Daley CL, Iaccarino JM, Lange C, et al. Treatment of nontuberculous mycobacterial pulmonary disease: an official ATS/ERS/ESCMID/IDSA clinical practice guideline. Eur Respir J. 2020 Jul;56(1):2000535. doi:10.1183/13993003.00535-2020
- Im Y, Hwang NY, Kim K, et al. Impact of time between diagnosis and treatment for nontuberculous mycobacterial pulmonary disease on culture conversion and all-cause mortality. Chest. 2022 May;161(5):1192–1200. doi: 10.1016/j.chest.2021.10.048
- Diel R, Nienhaus A, Ringshausen FC, et al. Microbiologic outcome of interventions against Mycobacterium avium complex pulmonary disease: a systematic review. Chest. 2018 Apr;153(4):888–921. doi: 10.1016/j.chest.2018.01.024
- Jarand J, Davis JP, Cowie RL, et al. Long-term follow-up of Mycobacterium avium complex lung disease in patients treated with regimens including clofazimine and/or rifampin. Chest. 2016 May;149(5):1285–1293. doi: 10.1378/chest.15-0543
- Wallace RJ Jr., Brown-Elliott BA, McNulty S, et al. Macrolide/Azalide therapy for nodular/bronchiectatic mycobacterium avium complex lung disease. Chest. 2014 Aug;146(2):276–282. doi: 10.1378/chest.13-2538
- Boyle DP, Zembower TR, Qi C. Relapse versus reinfection of Mycobacterium avium complex pulmonary disease. Patient characteristics and Macrolide susceptibility. Ann Am Thorac Soc. 2016 Nov;13(11):1956–1961. doi: 10.1513/AnnalsATS.201605-344BC
- Koh WJ, Moon SM, Kim SY, et al. Outcomes of Mycobacterium avium complex lung disease based on clinical phenotype. Eur Respir J. 2017 Sep;50(3):1602503. doi: 10.1183/13993003.02503-2016
- Ku JH, Henkle E, Aksamit TR, et al. Treatment of nontuberculous mycobacterial (NTM) pulmonary infection in the US bronchiectasis and NTM registry: treatment patterns, adverse events, and adherence to American thoracic society/infectious disease society of America treatment guidelines. Clin Infect Dis. 2023 Jan 13;76(2):338–341. doi: 10.1093/cid/ciac788
- Morimoto K, Namkoong H, Hasegawa N, et al. Macrolide-resistant Mycobacterium avium complex lung disease: analysis of 102 consecutive cases. Ann Am Thorac Soc. 2016 Nov;13(11):1904–1911. doi: 10.1513/AnnalsATS.201604-246OC
- Kwak N, Park J, Kim E, et al. Treatment outcomes of Mycobacterium avium complex lung disease: a systematic review and meta-analysis. Clin Infect Dis. 2017 Oct 1;65(7):1077–1084. doi: 10.1093/cid/cix517
- Kwon YS, Kwon BS, Kim OH, et al. Treatment outcomes after discontinuation of ethambutol due to adverse events in Mycobacterium avium complex lung disease. J Korean Med Sci. 2020 Mar 9;35(9):e59. doi: 10.3346/jkms.2020.35.e59
- Balavoine C, Blanc F-X, Lanotte P, et al. Adverse events during treatment of nontuberculous mycobacterial lung disease: do they really matter? Eur Respir J. 2018;52(suppl 62):PA2664. doi: 10.1183/13993003.congress-2018.PA2664
- Kim DH, Kim BG, Kim SY, et al. In vitro activity and clinical outcomes of clofazimine for nontuberculous mycobacteria pulmonary disease. J Clin Med. 2021 Oct 3;10(19):4581. doi: 10.3390/jcm10194581
- Pfaeffle HOI, Alameer RM, Marshall MH, et al. Clofazimine for treatment of multidrug-resistant non-tuberculous mycobacteria. Pulm Pharmacol Ther. 2021 Oct;70:102058.
- McGuffin SA, Pottinger PS, Harnisch JP. Clofazimine in nontuberculous mycobacterial infections: a growing niche. Open Forum Infect Dis. 2017 Summer;4(3):ofx147. doi: 10.1093/ofid/ofx147.
- Shuto H, Komiya K, Goto A, et al. Efficacy and safety of fluoroquinolone-containing regimens in treating pulmonary Mycobacterium avium complex disease: a propensity score analysis. PLoS One. 2020;15(7):e0235797. doi: 10.1371/journal.pone.0235797
- Flume PA, Griffith DE, Chalmers JD, et al. Development of drugs for nontuberculous mycobacterial disease: clinicians’ interpretation of a US food and drug administration workshop. Chest. 2021 Feb;159(2):537–543. doi: 10.1016/j.chest.2020.08.2055
- Wu ML, Aziz DB, Dartois V, et al. NTM drug discovery: status, gaps and the way forward. Drug Discov Today. 2018 Aug;23(8):1502–1519. doi: 10.1016/j.drudis.2018.04.001
- Ruth MM, van Ingen J. New insights in the treatment of nontuberculous mycobacterial pulmonary disease. Future Microbiol. 2017 Oct;12(13):1109–1112. doi: 10.2217/fmb-2017-0144
- Locher CP, Jones SM, Hanzelka BL, et al. A novel inhibitor of gyrase B is a potent drug candidate for treatment of tuberculosis and nontuberculosis mycobacterial infections. Antimicrob Agents Chemother. 2015 Mar;59(3):1455–1465. doi: 10.1128/AAC.04347-14
- Grossman TH, Bartels DJ, Mullin S, et al. Dual targeting of GyrB and ParE by a novel aminobenzimidazole class of antibacterial compounds. Antimicrob Agents Chemother. 2007 Feb;51(2):657–666. doi: 10.1128/AAC.00596-06
- Durcik M, Tomasic T, Zidar N, et al. ATP-competitive DNA gyrase and topoisomerase IV inhibitors as antibacterial agents. Expert Opin Ther Pat. 2019 Mar;29(3):171–180. doi: 10.1080/13543776.2019.1575362
- Henderson SR, Stevenson CEM, Malone B, et al. Structural and mechanistic analysis of ATPase inhibitors targeting mycobacterial DNA gyrase. J Antimicrob Chemother. 2020 Oct 1;75(10):2835–2842. doi: 10.1093/jac/dkaa286
- Chaudhari K, Surana S, Jain P, et al. Mycobacterium tuberculosis (MTB) GyrB inhibitors: an attractive approach for developing novel drugs against TB. Eur J Med Chem. 2016 Nov 29;124:160–185. doi: 10.1016/j.ejmech.2016.08.034
- Charifson PS, Grillot AL, Grossman TH, et al. Novel dual-targeting benzimidazole urea inhibitors of DNA gyrase and topoisomerase IV possessing potent antibacterial activity: intelligent design and evolution through the judicious use of structure-guided design and structure-activity relationships. J Med Chem. 2008 Sep 11;51(17):5243–5263. doi: 10.1021/jm800318d
- Finn J. Evaluation of WO2012177707 and WO2012097269: vertex’s phosphate prodrugs of gyrase and topoisomerase antibacterial agents. Expert Opin Ther Pat. 2013 Sep;23(9):1233–1237. doi: 10.1517/13543776.2013.820707
- Brown-Elliott BA, Rubio A, Wallace RJ Jr. In vitro susceptibility testing of a novel benzimidazole, SPR719, against nontuberculous mycobacteria. Antimicrob Agents Chemother. 2018 Nov;62(11). doi: 10.1128/AAC.01503-18.
- Stokes SS, Vemula R, Pucci MJ. Advancement of GyrB inhibitors for treatment of infections caused by Mycobacterium tuberculosis and non-tuberculous mycobacteria. ACS Infect Dis. 2020 Jun 12;6(6):1323–1331. doi: 10.1021/acsinfecdis.0c00025
- Nagaraja V, Godbole AA, Henderson SR, et al. DNA topoisomerase I and DNA gyrase as targets for TB therapy. Drug Discov Today. 2017 Mar;22(3):510–518. doi: 10.1016/j.drudis.2016.11.006
- Hooper DC, Jacoby GA. Topoisomerase inhibitors: fluoroquinolone mechanisms of action and resistance. Cold Spring Harb Perspect Med. 2016 Sep 1;6(9):a025320. doi: 10.1101/cshperspect.a025320
- Cotroneo N, Stokes SS, Pucci MJ, et al. In vitro activity and in vivo efficacy against non-tuberculous mycobacteria of SPR719, the active moiety of the novel oral benzimidazole prodrug SPR720. J Antimicrob Chemother. 2023. [manuscript submitted].
- Rubio A, Stapleton M, Verma D, et al. Potent activity of a novel gyrase inhibitor (SPR719/SPR720) in vitro and in a prolonged acute Mycobacterium abscessus mouse model of infection [poster SUN-539]. (WA) DC: ASM Microbe/American Society for Microbiology; 2018.
- Pennings LJ, Ruth MM, Wertheim HFL, et al. The benzimidazole SPR719 shows promising concentration-dependent activity and synergy against nontuberculous mycobacteria. Antimicrob Agents Chemother. 2021 Mar 18;65(4). doi: 10.1128/AAC.02469-20
- Pidot SJ, Porter JL, Lister T, et al. In vitro activity of SPR719 against Mycobacterium ulcerans, Mycobacterium marinum and Mycobacterium chimaera. PLoS Negl Trop Dis. 2021 Jul;15(7):e0009636. doi: 10.1371/journal.pntd.0009636
- Aragaw WW, Cotroneo N, Stokes S, et al. In vitro resistance against DNA gyrase inhibitor SPR719 in Mycobacterium avium and Mycobacterium abscessus. Microbiol Spectr. 2022 Feb 23;10(1):e0132121. doi: 10.1128/spectrum.01321-21
- Deshpande D, Kuret D, Cirrincione K, et al. Pharmacokinetics/Pharmacodynamics of the novel gyrase inhibitor SPR719/SPR720 and clinical dose selection to treat pulmonary Mycobacterium avium-complex disease. Open Forum Infect Dis. 1659 2020 Dec 31;7(Suppl 1):S817. doi: 10.1093/ofid/ofaa439.1837
- Verma D, Peterson C, Stokes S, et al. SPR720, a novel aminobenzimidazole gyrase inhibitor, demonstrates potent efficacy against Mycobacterium avium ATCC 700898 in a chronic C3HeBFeJ mouse infection model [poster 1637]. Arlington VA: IDWeek/IDSA; 2020.
- Talley AK, Thurston A, Moore G, et al. First-in-human evaluation of the safety, tolerability, and pharmacokinetics of SPR720, a novel oral bacterial DNA gyrase (GyrB) inhibitor for mycobacterial infections. Antimicrob Agents Chemother. 2021 Oct 18;65(11):e0120821. doi: 10.1128/AAC.01208-21