ABSTRACT
Introduction
Tuberculosis (TB) is a chronic infectious disease caused by mycobacterium tuberculosis (Mtb) that poses a major threat to human health.
Areas covered
Herein, we aim to review the alteration of the microbiota in gut and respiratory during TB development, the potential function and mechanisms of microbiota in the pathogenesis of Mtb infection, and the impact of antibiotic treatment on the microbiota. In addition, we discuss the potential new paradigm for the use of microbiota-based treatments such as probiotics and prebiotics in the treatment of TB.
Expert opinion
Studies have shown that trillions of micro-organisms live in the human gut and respiratory tract, acting as gatekeepers in maintaining immune homeostasis and respiratory physiology and playing a beneficial or hostile role in the development of TB. Anti-TB antibiotics may cause microecological imbalances in the gut and respiratory tract, and microbiome-based therapeutics may be a promising strategy for TB treatment. Appropriate probiotics and prebiotics supplementation, along with antimycobacterial treatment, will improve the therapeutic effect of TB treatment and protect the gut and respiratory microbiota from dysbiosis.
KEYWORDS:
1. Introduction
Tuberculosis (TB) remains a major health problem of global proportions. Although the estimated number of TB is on a downward trend compared to the past against the backdrop of the COVID-19 worldwide pandemic, there are still 10.6 million people who became ill with TB, and 1.6 million died of TB in 2021 [Citation1]. China ranks as one of the top countries with a high prevalence of TB, with approximately 8.5% of worldwide incident cases, second only to India. In addition, the emergence of drug-resistant tuberculosis poses a serious threat to public health, there were more than one hundred thousand cases of multidrug-resistant TB (MDR) or rifampin-resistant TB (RR-TB). Therefore, new TB drugs and strategies are urgently needed.
TB is the result of invasion by Mycobacterium tuberculosis (Mtb), and Mtb determines the development and progression of TB through complex host–pathogen interactions. Recent evidence has confirmed that the composition and function of the gut and respiratory microbiota are integral to the biology of the host and that their alteration contributes to the development of TB. Finely tuned interaction of the host microbiota with the immune system can lead to dysbiosis and impaired immune defenses, thereby increasing the risk of TB occurring [Citation2]. Meanwhile, TB treatment leads to extensive side effects, including liver damage [Citation3], acoustic nerve damage, hematological abnormalities, and gastrointestinal reactions [Citation4], which are probably related to microbial dysbiosis. Evidence is mounting that both the gut and respiratory microbiota should be taken into consideration in the pathogenesis and the treatment of TB. In this review, we provide an overview of the latest advances regarding the TB microbiota, focusing on the gut and respiratory axis micro-ecosystem during TB development and treatment. Finally, we discuss microbiota-based therapeutic intervention strategies for TB.
2. Microbiota in humans
The human microbiome, composed of various microbiota, is affected by both internal and external factors. Diet (high fiber, high sugar, and high protein), age, alcohol, smoking, infectious diseases, lifestyle habits, antibiotics, and genetic factors have been linked to dysbiosis and microbiome-associated pathologies. In the host body, the gut microbiota play a role in absorbing nutrients from food, then delivering the appropriate vitamins to the body, and providing a barrier function against exogenous pathogens. However, factors (diet, broad-spectrum antibiotics, inflammation state) that overload the body increase intestinal permeability and lead to the leakage of exogenous pathogens, finally cause a microbial dysbiosis [Citation5,Citation6]. Lifelong microbial dysbiosis affects gut and lung homeostasis by altering metabolic, physiological, and immunological status, thereby increasing susceptibility to infection and pathology.
It can be roughly divided into the oral, gastrointestinal, reproductive tract, and respiratory microbiota according to the different spaces occupied by normal microbiota in the micro-ecosystem [Citation7]. In this paper, we mainly focus on the gut and lung microbiota, which are closely associated with respiratory tract health and disease. Complex respiratory tract microbial communities not only interact with invading pathogens but also regulate the immune system. Respiratory microbiota protects the body from pathogens by producing and preserving immune cells or by secreting various antimicrobial peptides in the mucosal surface [Citation8–10]. Gut microbiota can also regulate the local immune homeostasis in the lung by exposing structural ligands and secreting metabolites [Citation11]. Dysbiosis of the gut and lung microbiota, which means a loss in richness and biodiversity of microbiota [Citation12], makes the host more susceptible to infection or drug resistance [Citation13]. For example, respiratory dysbiosis may promote cells in the lower respiratory tract being exposed to bacterial LPS and then stimulate immune system response, ultimately leading to steroid resistance in Asthma [Citation14,Citation15]. On the other hand, diseases can be inhibited by microbiota system restoration. For instance, improving the gut microbiota diversity can enhance the response to anti-PD1 immunotherapy [Citation16], as well as improve the effect of chemotherapy treatment in lung cancer [Citation17].
3. The gut and lung microbiota of TB
At present, many studies have revealed that TB is intimately intertwined with the ecology of the human gut and respiratory microbiota [Citation18,Citation19]. The gut microbiota acts as a multiplicity of roles in maintaining balance in the host, such as detoxification, regulation of immunity and metabolism [Citation20]. Some researchers suggest that the pathogenesis of TB may not only be caused by a single causative agent, Mycobacterium tuberculosis (Mtb) disruption of microbial dynamics and the balance of their composition and abundance in the healthy gut and respiratory resulting in altered susceptibility to TB [Citation7]. Microbial dysregulation leads to a significant increase in the bacterial load in the lungs, promoting the spread of Mtb to the spleen and liver. In addition, the gut microbiota and the respiratory tract can fight against Mtb by interacting with the human body’s immune response [Citation8,Citation21,Citation22]. Specifically, Neisseria and Haemophilus in TB patients may influence the Th1 immune response, which is essential to help defend against viruses, intracellular bacteria (such as Mtb), and cancer cells [Citation23].
Both intestinal microbiota and lung microbiota play a structural role in the body and participate in local immunity. Gut-lung axis communicates with each other or transmits signals through the blood and lymphatic systems to participate in the body’s immune response. Therefore, it is necessary to reveal the composition of the intestinal and lung microbiota between TB and normal conditions, and fully understand which major microbiota are involved in the body reaction [Citation10]. Human gut microbiota is linked with the immune system and maintains the development of immune functions by providing signals and affecting the systemic pro-inflammatory response in the body [Citation24]. Compared with TB, the dominant genera in the gut of (healthy controls) HC are the genera Neisseria, Fusobacterium, Escherichia, and Bacteroides [Citation25]. In comparison, the lung microbiota was mainly composed of the genus Streptococcus, Haemophilus, Veillonella, and Prevotella [Citation26,Citation27]. Microbiota also influences the ability to fight against respiratory infection [Citation28]. For example, in the respiratory tract, an expanding collection of healthy bacteria, such as the genus Prevotella, Streptococcus, and Veillonella, promotes the differentiation of anti-inflammatory macrophages, which leads to anabolic remodeling [Citation29]. Haemophilus parainfluenzae enables Toll-like receptor (TLR) 4 to activate the pro-inflammatory and prohibit the corticosteroid-related pathway, whereas Haemophilus can activate inflammatory Th2 pathways and lead to bronchial high responsiveness [Citation30].
Individuals’ gut and lung microbiome variability may affect their response to vaccines. A study found that gut dysbiosis reduced the efficacy of Bacillus Calmette – Guérin (BCG) vaccination in animals, as evidenced by a decrease in effector and memory T cells generation and an increase in colony forming units (CFUs) in the lungs of Mtb infected mice. This suggests that gut dysbiosis can significantly impact vaccine endurance and protective efficacy [Citation31]. Additionally, a recent study showed that parenteral BCG vaccination causes time-dependent changes to the intestinal microbiome, barrier function and microbial metabolites, and subsequent changes in circulating and lung metabolites, leading to the induction of memory macrophages and trained immunity in the lung. The results suggest that part of the protection conferred by BCG vaccination occurs through modulation of the lung-gut axis [Citation32]. Furthermore, fecal transplants from healthy animals to those with disrupted guts restored immunological responses toward Mtb confirming that gut flora plays a crucial role in modulating TB vaccine efficacy.
3.1. The gut microbiota of TB
Numerous studies have found that Mtb infection could change microbiota composition in mice, macaques, and humans [Citation33–37]. In the gut of TB, Ruminococcacea, Enetrobactericeae, Erysipelotrichaceae, Bifidobacterium, and so on, were the major genera [Citation34]. Hu et al. reported that pulmonary Mtb infection contributed to a slight decrease in the α diversity of the gut microbiota, compared with HC, particularly the genus Bacteroides [Citation28]. As gut commensals, Bacteroides can provide nutrients to other microbial residents of the gut through their polysaccharide utilization loci (PULs) and maintain gut homeostasis through its outer membrane vesicle (OMV). Moreover, Bacteroides can take part in immunity and defense, by the manner of an independent contract that secretes antimicrobial toxins and involves interference competition [Citation28,Citation38]. Another research recruited a cohort in Xinjiang, a multi-ethnic region, and reported a contrasting result that the abundance of the genus Bacteroides increased in TB compared with Latent tuberculosis infection (LTBI) and HC group [Citation39]. These different results might be influenced by geographical variation, types of populations, and hereditary characteristics. In addition, a study showed natural colonization of the digestive tract with Helicobacter hepaticus in mice, which was concomitant with modification of the gut microbiota that Bacteroidaceae enriched and Firmicutes decreased, drastically impaired immune control of the growth of subsequently administered M. tuberculosis, suggesting the potential role of Bacteroidaceae in the susceptibility of Mtb in mouse models [Citation40].
Wang, S indentified the changes in the relative abundance of specific genera that were associated with significant alterations in fecal metabolites [Citation41]. At the genus level, Bacteroides, Parabacteroides, Fusobacterium, and Lachnoclostridium enriched in PTB patients, while Blautia, Roseburia, Bifidobacterium, unidentified Ruminococcaceae, Fusicatenibacter, and Romboutsia enriched in healthy people [Citation41]. This dysregulation was reported to decrease the production of short-chain fatty acids (SCFAs), especially acetic, propionic, and butyric acids, which influence inflammatory inhibition [Citation41,Citation42].
One following study is focused specifically on children’s gut microbiota in individuals with TB [Citation43]. Although the overall structure of the children’s gut microbial community remained similar between those with TB and HC, the study observed differences in the relative abundance of certain families, genera, and species. Pediatric patients with TB showed an upregulation of Prevotella (known as a pro-inflammatory bacterium) and Enterococcus (known as an opportunistic pathogen); while downregulation of beneficial bacteria such as Bifidobacteriaceae, Ruminococcaceae, and prausnitzii [Citation43]. The researchers hypothesized that intestinal microbial homeostasis in patients with PTB may affect the pathogenesis of PTB by regulating the host immune status through the gut-lung axis.
In addition, a clinical trial linked clinical outcomes in TB to the microbiota for the first time, suggesting that maintaining gut microbiota homeostasis may be beneficial for host recovery and prevention of recurrent Mtb infection [Citation44]. Compared to HC, gut microbiota diversity index was significantly increased in patients with new and recurrent TB (ACE and Chao1 diversity index). The relative abundance of the phyla Bacteroidetes (containing a variety of beneficial commensal organisms) was reduced in patients with new and recurrent TB. Conversely, Proteobacteria (containing many pathogenic species) were significantly enriched in the gut compared to HC. CD4+ lymphocytes are the most important adaptive immune cells for the infectious response. In the clinical trial, another interesting finding was that the genus Prevotella and Lachnospira decreased in both new and recurrent TB groups. They correlated positively with the peripheral CD4 + cells in the new TB group, whereas the results were reversed in the recurrent TB group [Citation44]. These data suggest that specific gut microbes may be associated with host immune status, which in turn affected prognosis and outcome of TB.
3.2. The lung microbiota of TB
In TB, lung and the entire lower respiratory tract (LRT) have specific microbial features. Ding, L collected bronchoalveolar lavage fluid from 123 patients with TB to understand the lung microbiota and found that Mtb, Staphylococcus aureus, and Kluyveromyces lactis were enriched in bacteriologically confirmed group. In contrast, Haemophilus parainfluenzae was enriched in the lungs of the bacteriologically negative group [Citation45]. A study reported that TB patients had a decreased diversity of alveolar microbiota compared with HC, characterized by a reduction in genus Streptococcus and Fusobacterium and an increase in mycobacterial abundance. These changes might be influenced by the inflammatory environment, and Mtb could release virulence factors, ESAT-6, and CFP-10 to compete for its nutrients, which suppressed the macrophage response [Citation46].
In another study, four major genera, Haemophilus, Neisseria, Streptococcus, and Veillonella, were selected for culture to determine microbiota differences in the lower respiratory tract microbiota in TB and lung cancer. It was concluded that only Streptococcus was significantly increased in TB and that the Th1-response in TB group may be influenced by Neisseria and Haemophilus [Citation23]. Compared with healthy volunteers, Vázquez-Pérez, J demonstrated a significant reduction of the Streptococcus genus and was associated with increased Mtb abundance [Citation46]. The trends that were not consistent might be influenced by the study population. Meanwhile, Sala et al. studied whether different geographical settings would contribute to the composition divergence of microbiota in TB and control patients. The results showed no different enrichment characteristics in the group of TB patients and controls recruited in Switzerland, Italy, and Bangladesh [Citation47].
From another aspect, different sample types of the respiratory tract can also affect the results of lung microbiota as the Bronchoalveolar lavage (BAL) samples had a lower abundance and diversity of the microbiota compared with those of the throat swab samples. Under normal circumstances, Klebsiella pneumoniae, Staphylococcus aureus, and Pasteurella multocida were the three most common species in BAL samples, while Roxella mucilis is the most common species of throat swab samples. In contrast, in the untreated PTB group, Staphylococcus aureus and Neisseria gonorrhoeae were enriched in BALF samples [Citation48]. A survey using sputum samples from India found that phyla Bacteroidetes and Proteobacteria were enriched in Chinese TB patients and healthy Indian people. In contrast, phyla Firmicutes were enriched in healthy Chinese people and Indian TB patients. In addition, among the 16 major genera, Streptococcus was the most dominant genus in both TB and HC groups, and Neisseria and Veillonella were the other two dominant genera in the TB group. Moreover, the opportunistic pathogen Rothia mucilaginosa was widespread in TB patients [Citation49]. A particular study using nasopharyngeal swab samples revealed that Mtb infection significantly changed microbiota composition. Phyla Proteobacteria and Gammaproteobacteria increased in TB compared to LTBI. The Phyla Pseudomonadales and Moraxellaceae increased, while phyla Bacillales and Lachnospiraceae decreased in TB compared to HC. Furthermore, Corynebacterium was less in the TB group compared with HC and LTBI, and the relative abundance of Corynebacterium may be related to nutritional status, immune-related molecules, and inflammation-related indicators [Citation50].
4. The potential function and mechanism of gut microbiota in TB
Study described that peripheral CD4+ cells in new and recurrent TB patients correlated with gut microbiota [Citation44]. Prevotella and peripheral CD4+ cells in new TB patients had a positive correlation but the opposite in patients with recurrent TB (). It means that specific microbiota may make contact with the host immune system during TB. Several researchers have studied the function of the microbiota in animal models and concluded that microbiota-associated non-coding RNAs are important mediators in the regulation of anti-tuberculosis immunity. Yang, F et al. analyzed the role of gut microbiota in Mtb-infected mice by using antibiotics to destroy the intestinal microbiota and found that the intestinal microbiota could modulate host TB susceptibility and pulmonary inflammatory responses through gut bacteria-regulated lncRNA (lncRNA-CGB) [Citation51]. The study revealed that the gut microbiota Bacteroides fragilis (B. fragilis) was a vital regulator of the lncRNA-CGB expression profile. lncRNA-CGB could interact with EZH2 and negatively control H3K27Me3, ultimately rousting the protective immunity against Mtb by inducing IFN-γ expression (). Another study by Yang’s team found that microRNA could also influence the ability to control Mtb infection in the lung. In this study, gut dysbacteriosis could remotely increase the expression of miRNA-21 in the lung, specifically reducing the production of IFN-γ, thus weakening anti-TB immunity () [Citation25].
Figure 1. (A) Prevotella and peripheral CD4+ cells were positively correlated in new-onset TB patients, whereas showed a negative correlation in relapsed TB patients. (B) Bacteroides fragilis upregulated the lncRNA-CGB, which then contacts EZH2 of histone methyltransferase PRC2, negatively controlling the epigenetic regulation of H3K27Me3, leading to increased expression of IFN-γ and a robust capacity to against Mtb. (C) miRNA-21 was predicted to interact with IFN-γ during the dysbiosis of the gut microbiota after constructing a “loss-of-function” model. Then the IFN-γ expression was decreased, which in turn leads to a weakened anti-TB immune function. (D) Anaerostipes may actively engage with “interferon signalling”, “Nur77 signalling” and “inflammasome” pathways, all of which are aimplicated in the pro-inflammatory responses to TB, and therefore it may predict pro-inflammatory responses. (E) The colonization of Helicobacter in mice promoted changes in the gut microbiota, outbreak of inflammation,reduced innate immunity and accumulation of activated T cells, all of which resulted in a loss of the immunity to suppress Mtb infection.
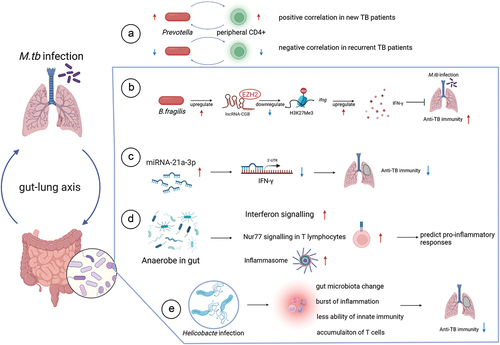
In addition to bacteria, Anaerostipes has also been reported to be involved in the anti-TB immune response and could be used to predict pro-inflammatory responses in TB. Mechanistically, Anaerostipes were found to positively interact with the interferon signaling, Nur77 signaling, and inflammasome pathways, all of which are associated with pro-inflammatory responses in TB () [Citation52].
Co-infection with other microbes may affect the microbiota in TB patients. Helminths are predicted to mediate immune tolerance to TB through the gut microbiota [Citation53]. Microbiota diversity is known to decrease during Mtb infection or anti-TB therapy. However, in animal models, helminth infection has been shown to increase gut microbiota diversity. Helminth infection might impact TB, but the underlying mechanism remains unknown. One possibility is that helminthic infection elicits a robust type 2 immune response that may contribute to TB tolerance by suppressing both type 1 and type 3 immune responses, thereby reducing inflammation and pathology. Additionally, Helicobacter is another microbe that changes microbiota in the host. Majlessi L et al. found that chronic or acute infections, and consequent immune responses, can affect host susceptibility to unrelated pathogens [Citation40]. Symptomatic or asymptomatic microbial cloning can be a critical factor in TB. Helicobacter hepaticus (H. hepaticus), one of the most common bacteria associated with human biliary and liver disease, can alter the gut microbiota, increasing susceptibility to Mtb in mice [Citation40]. The colonization of H. hepaticus in mice stimulated the loss of immune control to inhibit Mtb infection by reducing innate immunity and accumulating activated T cells () [Citation40]. Helicobacter pylori, another species, also proved that its colonization changes the risk of Mtb infection. H. pylori is associated with increased IFN-γ and Th1-like responses to specific TB antigens. Researchers have observed that infected household contacts are significantly more likely to have concurrent H. pylori infection than TB cases, and H. pylori-infected animals are significantly less likely to develop active disease than uninfected animals [Citation54].
Taken together, the above studies suggest that during Mtb infection, the gut microbiota may interact with the body’s immune system by regulating the expression of non-coding RNAs, influencing the inflammatory pathway, or combining with the effects of helminth and Helicobacter hepaticus infection to fight TB. When it comes to lung microbiota studies in TB patients, most studies look at changes in the composition of the microbiota. The results are unable to determine whether the changes identified are a cause or a consequence of Mtb infection. Due to the limited evidence in the current studies, the potential function and mechanisms of the lung microbiota need to be further investigated.
5. Effect of antibiotic therapy on gut and lung microbiology in TB
Many studies have shown that the administration of anti-tuberculosis drugs, including isoniazid (INH), rifampicin (RIF), pyrazinamide (PZA), and ethambutol (EMB), can cause changes in the gut and lung microbiota (). Patients diagnosed with TB should receive the above drug treatment for at least 6 months until the disease is ruled out. After the long-term anti-TB treatment, there was a significant decrease in microbiome diversity [Citation57]. Both Mtb infection and anti-tuberculosis treatment lead to dysbiosis of the gut microbiota, but Mtb infection causes only minor changes in the alpha diversity of the microbiota, whereas anti-tuberculosis treatment leads to more significant and rapid changes in the community structure [Citation28]. During treatment, some microorganisms belonging to the genus Clostridiales of the phylum Firmicutes were significantly decreased in the gut, e.g. Firmicutes Ruminococcus sp. 39BFAA, Ruminococcus gnavus, and Faecalibacterium (OTU 15). Meanwhile, many members of the genus Bacteroides were increased, such as Bacteroides fragilis and Bacteroides OTU230. In addition, OTU8 and OTU2972, belonging to the family Erysipelotrichaceae, and Enterococcus, belonging to the family Enterococcaceae of the phylum Firmicutes, increased significantly 1 week after the start of treatment, while other members of this family decreased [Citation28]. Cao, D et al. confirmed the alteration of Firmicutes and Bacteroidetes in the intestine during the treatment of TB [Citation55]. Compared to admission, Li et al. found that the community and the relative abundance of the bacteria in pediatric PTB patients presented no obvious changes in the gut microbiota after 1 month of treatment. This was in contrast to the findings of previous studies [Citation43]. The difference between this research and others may be mainly due to the age of individual recruited.
Table 1. Impact of antibiotic therapy on the gut-lung microbiota in TB patients.
Interestingly, the team of Cao observed that anti-TB treatment led to not only a change in gut bacteria but also fungi, which related to the progress of TB and might play a vital role in the balance of gut microbiota. After the anti-TB treatment, the fungal richness increased, especially Basidiomycota. Moreover, the ratios of Basidiomycota/Ascomycota of the TB2MT and TB6MT groups dramatically reduced compared with the HC and naive TB groups [Citation55]. This study observed that after 2 months of anti-TB treatment, the diversity of fungi decreased, and the transkingdom network of fungal-bacterial changed seriously.
Similarly, the lung microbiota vary between patients with tuberculosis and those following anti-tuberculosis treatment. In a study of Ugandan tuberculosis patients, Mycobacterium and Fusobacterium counts decreased and Ruminococcus and Peptococcus counts increased during the second month of anti-tuberculosis treatment compared with pre-treatment. In the fifth month, the number of characteristic Tanneria and Fusobacterium was decreased, while Neisseriaceae was increased [Citation58]. Xiao G et al. suggested that after anti-TB therapy, TB patients showed significantly higher α-diversity and decreased abundances of Staphylococcus aureus and Pasteurella multocida. This study also showed that the cured TB patients showed similar α and β diversity as healthy people [Citation48]. Hu et al. intended to find the different states of PTB patients’ microbial communities in the lung. It has been shown that comparing the microbiota with MTB-positive patients and their negative conversion, the study reported that MTB-positive had a lower α diversity, characterized by the increase of Rothia and reduction of Serratia, which implied that anti-TB treatment could contribute to the recovery of the microbiota in the lung [Citation56].
6. Other risk factors influence microbiota in TB
A balance between the virulence of an infected Mtb strain and the immune status of the infected host is essential to control TB susceptibility. So far, the parameters involved in this balance include host genetic background, gender, age, nutritional status, and the incidence of HIV co-infection or diabetes. Most studies involved the region, gender, and age of the individual, but few studies have focused on the micro-ecosystems of HIV with TB or diabetes with TB. Ueckermann, V. et al. showed that the lung microbiome in PTB with HIV co-infection was dominated by Proteobacteria, Firmicutes, Actinobacteria, and Bacteroidetes, with a connective loss of microbial diversity [Citation59]. People with type 2 diabetes have a threefold increased risk of developing TB. A study using a murine model suggested that compared to a non-diabetic animal, there was a significant dysbiosis of intestinal microbiota in TB with type 2 diabetes mice [Citation60]. Thus, researchers should pay attention to the factors that enhance TB susceptibility. To develop therapeutic agents for TB with type 2 diabetes or HIV and more relevant research and more experiments are needed to explore and verify.
7. Differences in technical methods for measuring the gut and lung microbiotas
16S rRNA amplicon sequencing is one of the NGS methods that directly sequence microbial DNA or RNA, for example in feces, BAL and/or tissue samples. The 16S rRNA gene is an ideal target because it is highly conserved and necessary in bacteria and contains nine hypervariable regions (V1-V9) that differ between bacterial species and genera [Citation61].
Many studies used V3-V4 16S rRNA gene sequencing analysis to help measure gut and lung microbiota. Fecal samples are used to reflect the intestinal microbiota roughly. However, the methods used to analyze the lung microbiota differed slightly from those used in the gut (). For example, some studies used real-time PCR, while others used bacterial 16S rDNA amplification and metagenome sequencing. The different regions of the 16S rRNA gene sequence were used for detection, such as V1-V2 regions [Citation47], V3-V4 regions [Citation56,Citation58], and V6-V7 regions [Citation49]. Furthermore, the samples used for lung micro-ecological analyses differ, including BAL samples [Citation18,Citation46,Citation48,Citation56], sputum [Citation47,Citation49,Citation58], and throat swab [Citation48], even nasopharyngeal swab samples [Citation50]. BAL is an ideal sample of the lower respiratory tract, superior to sputum, throat swabs, nasopharyngeal swab, greatly reducing contamination of the upper respiratory tract flora.
Table 2. Dysbiosis of gut and lung microbiota in TB.
8. Prospective
8.1. Host microbiota-directed therapies for TB
The study reports that prolonged use of anti-TB antibiotics can negatively affect the balance of gut microbiota, potentially increasing susceptibility to Mtb re-infection or TB recrudescence after successful treatment [Citation62]. Due to the side effects and drug resistance of antibiotics, whose usage enormously impacts human-associated microbial communities, for they perturb the ecological system by killing symbiotic bacterial communities, creating rare opportunities for a pathogenic population, the scientific community is trying to find novel TB strategies. Therefore, a new concept of ‘Host Microbiota Directed-Therapies (HMDT)’ was introduced to target the detrimental population of bacteria and cure microbiota dysbiosis, including probiotics and prebiotics showing anti-TB activity in vitro and in vivo [Citation63]. Some HMDTs have been well adopted in treating gastrointestinal disorders such as inflammatory bowel disease, colorectal cancer, antibiotic-induced diarrhea, and respiratory illnesses such as chronic obstructive pulmonary disease and Asthma [Citation64,Citation65]. As microbiota-based interventions, probiotics and prebiotics will benefit the host when administered in adequate amounts [Citation66], which might be potential adjunctive means to TB treatment by recovering the immune protective gut microbial populations eliminated by antibiotics, thus enhancing the efficiency and reducing the durability of antibiotics.
8.2. Probiotics therapy
Probiotics are part of the microbiota-targeted class of therapies, whose therapeutic properties are closely related to ecological principles. Probiotics refer to microorganisms that naturally colonize on the surfaces of our mucosal, including Bifidobacterium spp., Lactobacillus spp., Streptococcus salivarius, et al. Probiotics can promote and re-constitute the composition of microbiota in different parts of the human body such as the gut, skin, mouth, vagina, and upper respiratory tract. Using probiotics to supplement microbial flora can reduce adverse reactions, enhance the body’s immune function, and improve the ability to fight against Mtb infection. Additionally, some new technologies, such as nanotechnology and micro-capsules, can increase the permeability and viability of probiotics and enhance the therapeutic effect. Currently, researchers recommend developing animal models to explore whether combining anti-TB antibiotics treatment with probiotics will improve the treatment response and outcomes in TB patients [Citation62].
8.3. Prebiotics therapy
Instead of administrating live bacteria to patients, prebiotics is used for regulating the microbiome population or function and refer to a health-beneficial substrate selectively utilized by host microorganisms, including non-carbohydrate substances (fatty acids, phytochemicals phenols, and phytochemicals), topical applications other than gastrointestinal tract (GIT), and diverse categories other than food. The commonly used prebiotics are inulin, galactooligosaccharides (GOS), fructooligosaccharides (FOS), lactulose, and other fermentable oligosaccharides. Since more than 1000 microbial species are fixed in the human body, some researchers have recognized that supplementing certain prebiotic mixtures may be more likely to change the human microbiota than using specific probiotic strains [Citation67]. The microbiota-directed therapy aims to target harmful bacteria and shift them toward a mutually beneficial bacterial community. Therefore, strategies such as prebiotics could be an ideal asset to efficiently control TB infection [Citation63]. As an immune active ingredient, probiotics appear promising for clinical application, given their low risk of adverse effects, ease of administration, and strong potential to influence the composition and function of the gut and lung microbiota. However, further studies are required for an in-depth understanding and characterization of their effects.
8.4. How TB patients can be better distinguished in the future
Based on the differences in gut and lung microbiota in healthy people, TB patients, and anti-TB patients, microbiome biomarkers have the potential to distinguish TB patients from healthy individuals and even assess the development status of TB [Citation28,Citation56,Citation68]. For instance, sputum microbiota could differentiate multidrug-resistant TB (genus Rothia is a potential biomarker for rifampicin-resistant TB patients, while Bordetella is a potential diagnostic marker for multidrug-resistant TB patients.) [Citation69,Citation70]. Microbiome biomarkers might also be used to predict the peripheral inflammatory state during anti-TB therapy (the higher richness of Clostridium IV and XIVa are associated with pro-inflammatory pathways) [Citation69]. Moreover, as Mtb can influence the epigenome in the host transcriptional profile, epigenetic modifications can be a diagnostic tool during TB diagnosis and development.
9. Conclusion
By summarizing the gut and lung microbiota characteristics and associated mechanisms in TB patients and anti-TB patients, it is known that the micro-ecosystem plays a key role in the pathogenesis of TB and that the use of anti-TB drugs has a definite impact on the micro-ecological environment. Dysbiosis of the microbiota not only inhibits the host immune control of TB but also affects the anti-TB treatment. In this review, we have learned about the recent relationship between TB patients and microbiota, including different microbiota compositions, mechanisms by which the microbiota influences TB susceptibility and methods to improve the efficiency of TB diagnosis and treatment. Ultimately, this may reduce patient suffering and adverse reactions during treatment. We hope that there will be relevant studies on the use of microecological agents in TB treatment.
10. Expert opinion
The relationship between the microbiome and tuberculosis (TB) is complex and not yet fully understood, hindering the development of improved TB treatments. Until now, we can only follow the basic treatment and endure painful side effects. Based on the above findings, it is now necessary to comprehensively unravel the mechanisms of dysbiosis during Mtb infection or anti-TB therapy and restore normal bacterial communities structurally and functionally. Studies on the gut and lung microbiota in TB provide insight into the diversity pattern of the microbial dysbiosis, which is essential for the development of microbiome-based therapeutics.
The concept of HMDT with probiotics and prebiotics supplementation has been well accepted in the treatment of several diseases. These therapeutic strategies resulted in good tolerability and better clinical outcomes, which could be clinically implemented in TB treatment. However, the currently available evidence on the effect of TB and anti-TB therapy on gut and lung dysbiosis is based on animal studies and observational epidemiological studies. In addition, there is no clinical research based on probiotics or prebiotics supplementation in TB. There is a need for well-designed clinical studies to provide solid evidence on the effects of HMDT on clinical outcomes in TB patients. Once this evidence is available, probiotics and prebiotics supplementation could be incorporated into the standard TB treatment regimen [Citation2].
New drugs for TB are urgently needed, and microbiome-based therapeutics may be a promising strategy. As members of an organism, the influence of the microbiome on disease is difficult to predict. If we can understand the relationship between the microbiota and tuberculosis, we can provide different treatments and even develop a personalized treatment plan. The tryptophan (Trp) analog indole propionic acid (IPA) is the first antitubercular metabolite produced by human gut bacteria. Research has shown that IPA has antimycobacterial activity that may be effective against a wide range of TB strains [Citation71]. Other microbe-derived metabolites and proteins may also work against Mtb. Apart from the metagenomics aspects, microbiota-based metabolomics and proteomics studies can help determine the missing link in the pathogenesis of TB. Moreover, most of the studies are based on 16S metagenomics, which provides an idea of microbial community diversity during TB. The functional diversity profile can be understood through shotgun metagenomics. However, the high sequencing cost of the latter method limits its use [Citation2].
Further exploration of the relationship between changes in the lung and gut microbiome and TB is important for clinicians and researchers, as they may be risk factors for the development of TB and treatment failure. A lot of significant evidence have supported the idea that alteration in the diversity and composition of the microbiota may have direct links between the immune response of TB [Citation7,Citation23]. However, further studies are required to assess in detail the evidence of microbiota composition changes in relation to the mechanisms of TB development. If we can correlate the pattern of microbiota dysbiosis with latent TB pathogenesis, then new therapeutics that balance the microbiota in the gut and lungs can be used to manage TB or distinguish TB, thus culminating the burden of TB disease in the future. In addition, the research on HIV/diabetes with TB can also be explored to pave the way for the future development of relevant microbial agents for the benefit of humanity.
Article highlights
TB or anti-TB contributed to decreased microbial diversity in the gut and lungs.
Microbiota affects susceptibility to TB by regulating inflammation and immunity.
Gut -lung axis microbiome variability may affect individual responses to vaccines.
Maintenance of gut microbiota homeostasis may be beneficial for host recovery and prevention of recurrent Mtb infection.
Microbiota-based interventions such as probiotics and prebiotics could serve as a potential advantageous supplementary approach to TB therapy.
Declaration of interest
The authors have no relevant affiliations or financial involvement with any organization or entity with a financial interest in or financial conflict with the subject matter or materials discussed in the manuscript. This includes employment, consultancies, honoraria, stock ownership or options, expert testimony, grants or patents received or pending, or royalties.
Reviewer disclosures
Peer reviewers on this manuscript have no relevant financial or other relationships to disclose.
Ethics statement
This study was approved by the ethics committee of the Baoan People’s Hospital of Shenzhen (approval no. BYL20220611).
Acknowledgments
We thank the reviewers for their valuable suggestions. The figures were created with BioRender.com.
Data availability statement
The datasets generated or analyzed during this study are available in PubMed.
Additional information
Funding
References
- Organization WH. Global tuberculosis report 2022 Available from: https://www.who.int/publications/i/item/9789240061729
- Baral T, Kurian SJ, Thomas L, et al. Impact of tuberculosis disease on human gut microbiota: a systematic review. Expert Rev Anti Infect Ther. 2023;21(2):175–188. doi: 10.1080/14787210.2023.2162879
- Wu S, Wang M, Zhang M, et al. Metabolomics and microbiomes for discovering biomarkers of antituberculosis drugs-induced hepatotoxicity. Arch Biochem Biophys. 2022;716:109118. doi: 10.1016/j.abb.2022.109118
- Noor S, Ismail M, Khan F. Drug safety in hospitalized patients with tuberculosis: drug interactions and adverse drug effects. Clin Respir J. 2021;15(1). doi: 10.1111/crj.13276
- Malesza IJ, Malesza M, Walkowiak J, et al. High-fat, Western-style Diet, systemic inflammation, and gut microbiota: a narrative review. Cells. 2021 Nov 14;10(11). doi: 10.3390/cells10113164
- Yang JJ, Wang JT, Cheng A, et al. Impact of broad-spectrum antimicrobial treatment on the ecology of intestinal flora. J Microbiol Immunol Infect. 2018 Oct;51(5):681–687. doi: 10.1016/j.jmii.2016.12.009
- Hong B-Y, Maulén NP, Adami AJ, et al. Microbiome changes during tuberculosis and antituberculous therapy. Clin Microbiol Rev. 2016;29(4):915–926. doi: 10.1128/CMR.00096-15
- Dumas A, Corral D, Colom A, et al. The host microbiota Contributes to Early protection against lung colonization by Mycobacterium tuberculosis. Front Immunol. 2018;9:2656. doi: 10.3389/fimmu.2018.02656
- Kim S-H, Jang Y-S. Recent insights into cellular crosstalk in respiratory and gastrointestinal mucosal immune systems. Immune Netw. 2020;20(6):e44. doi: 10.4110/in.2020.20.e44
- Shah T, Shah Z, Baloch Z, et al. The role of microbiota in respiratory health and diseases, particularly in tuberculosis. Biomed Pharmacother. 2021;143:112108. doi: 10.1016/j.biopha.2021.112108
- Budden KF, Gellatly SL, Wood DLA, et al. Emerging pathogenic links between microbiota and the gut-lung axis. Nat Rev Microbiol. 2017;15(1):55–63. doi: 10.1038/nrmicro.2016.142
- Comberiati P, Di Cicco M, Paravati F, et al. The role of gut and lung microbiota in susceptibility to tuberculosis. Int J Environ Res Public Health. 2021;18(22): doi: 10.3390/ijerph182212220
- Cai Y, Chen L, Zhang S, et al. The role of gut microbiota in infectious diseases. WIREs mechanisms of disease. 2022 Jul;14(4):e1551. doi: 10.1002/wsbm.1551
- Whitehead GS, Thomas SY, Cook DN. Modulation of distinct asthmatic phenotypes in mice by dose-dependent inhalation of microbial products. Environ Health Perspect. 2014;122(1):34–42. doi: 10.1289/ehp.1307280
- Lu J, Xiong L, Zhang X, et al. The role of lower airway dysbiosis in Asthma: dysbiosis and Asthma. Mediators Inflamm. 2017;2017:3890601. doi: 10.1155/2017/3890601
- Song P, Yang D, Wang H, et al. Relationship between intestinal flora structure and metabolite analysis and immunotherapy efficacy in Chinese NSCLC patients. Thorac Cancer. 2020;11(6):1621–1632. doi: 10.1111/1759-7714.13442
- Pizzo F, Maroccia Z, Hammarberg Ferri I, et al. Role of the microbiota in lung cancer: insights on prevention and treatment. Int J Mol Sci. 2022;23(11): doi: 10.3390/ijms23116138
- Lee Y, Seo H, Kim S, et al. Activity of lactobacillus crispatus isolated from vaginal microbiota against Mycobacterium tuberculosis. J Microbiol. 2021;59(11):1019–1030. doi: 10.1007/s12275-021-1332-0
- He C, Wang H, Yu C, et al. Alterations of gut microbiota in patients with intestinal tuberculosis that different from Crohn’s disease. Front Bioeng Biotechnol. 2021;9:673691. doi: 10.3389/fbioe.2021.673691
- Enjeti A, Sathkumara HD, Kupz A. Impact of the gut-lung axis on tuberculosis susceptibility and progression. Front Microbiol. 2023;14:1209932. doi: 10.3389/fmicb.2023.1209932
- Wood MR, Yu EA, Mehta S. The human microbiome in the fight against tuberculosis. Am J Trop Med Hyg. 2017;96(6):1274–1284. doi: 10.4269/ajtmh.16-0581
- Negi S, Pahari S, Bashir H, et al. Gut Microbiota Regulates Mincle Mediated Activation of Lung Dendritic Cells to Protect Against Mycobacterium tuberculosis. Front Immunol. 2019;10:1142. doi: 10.3389/fimmu.2019.01142
- Nakhaee M, Rezaee A, Basiri R, et al. Relation between lower respiratory tract microbiota and type of immune response against tuberculosis. Microb Pathog. 2018;120:161–165. doi: 10.1016/j.micpath.2018.04.054
- Huang S-F, Yang Y-Y, Chou K-T, et al. Systemic proinflammation after Mycobacterium tuberculosis infection was correlated to the gut microbiome in HIV-uninfected humans. Eur J Clin Invest. 2019;49(5):e13068. doi: 10.1111/eci.13068
- Yang F, Yang Y, Chen Y, et al. MiR-21 is remotely governed by the commensal bacteria and impairs anti-TB immunity by down-regulating IFN-γ. Front Microbiol. 2020;11:512581. doi: 10.3389/fmicb.2020.512581
- Mathieu E, Escribano-Vazquez U, Descamps D, et al. Paradigms of lung microbiota functions in health and disease, particularly, in Asthma. Front Physiol. 2018;9:1168. doi: 10.3389/fphys.2018.01168
- Eshetie S, van Soolingen D. The respiratory microbiota: new insights into pulmonary tuberculosis. BMC Infect Dis. 2019;19(1):92. doi: 10.1186/s12879-019-3712-1
- Hu Y, Yang Q, Liu B, et al. Gut microbiota associated with pulmonary tuberculosis and dysbiosis caused by anti-tuberculosis drugs. J Infect. 2019;78(4):317–322. doi: 10.1016/j.jinf.2018.08.006
- Wypych TP, Wickramasinghe LC, Marsland BJ. The influence of the microbiome on respiratory health. Nat Immunol. 2019;20(10):1279–1290. doi: 10.1038/s41590-019-0451-9
- Hufnagl K, Pali-Schöll I, Roth-Walter F, et al. Dysbiosis of the gut and lung microbiome has a role in asthma. Semin Immunopathol. 2020;42(1):75–93. doi: 10.1007/s00281-019-00775-y
- Nadeem S, Maurya SK, Das DK, et al. Gut dysbiosis thwarts the efficacy of vaccine against Mycobacterium tuberculosis. Front Immunol. 2020;11:726. doi: 10.3389/fimmu.2020.00726
- Jeyanathan M, Vaseghi-Shanjani M, Afkhami S, et al. Parenteral BCG vaccine induces lung-resident memory macrophages and trained immunity via the gut-lung axis. Nat Immunol. 2022 Dec;23(12):1687–1702. doi: 10.1038/s41590-022-01354-4
- Valdez-Palomares F, Muñoz Torrico M, Palacios-González B, et al. Altered microbial composition of drug-sensitive and drug-resistant TB patients compared with healthy volunteers. Microorganisms. 2021;9(8): doi: 10.3390/microorganisms9081762
- Khaliq A, Ravindran R, Afzal S, et al. Gut microbiome dysbiosis and correlation with blood biomarkers in active-tuberculosis in endemic setting. PLoS One. 2021;16(1):e0245534. doi: 10.1371/journal.pone.0245534
- Ding X, Zhou J, Chai Y, et al. A metagenomic study of the gut microbiome in PTB’S disease. Microbes Infect. 2022;24(2):104893. doi: 10.1016/j.micinf.2021.104893
- Cadena AM, Ma Y, Ding T, et al. Profiling the airway in the macaque model of tuberculosis reveals variable microbial dysbiosis and alteration of community structure. Microbiome. 2018;6(1):180. doi: 10.1186/s40168-018-0560-y
- Huang H-L, Luo Y-C, Lu P-L, et al. Gut microbiota composition can reflect immune responses of latent tuberculosis infection in patients with poorly controlled diabetes. Respir Res. 2023;24(1):11. doi: 10.1186/s12931-023-02312-w
- Zafar H, Saier MH. Gut Bacteroides species in health and disease. Gut Microbes. 2021;13(1). doi: 10.1080/19490976.2020.1848158
- Wang Y, Deng Y, Liu N, et al. Alterations in the gut microbiome of individuals with tuberculosis of different disease states. Front Cell Infect Microbiol. 2022;12:836987. doi: 10.3389/fcimb.2022.836987
- Majlessi L, Sayes F, Bureau JF, et al. Colonization with Helicobacter is concomitant with modified gut microbiota and drastic failure of the immune control of Mycobacterium tuberculosis. Mucosal Immunol. 2017;10(5):1178–1189. doi: 10.1038/mi.2016.140
- Wang S, Yang L, Hu H, et al. Characteristic gut microbiota and metabolic changes in patients with pulmonary tuberculosis. Microbiol Biotechnol. 2022;15(1):262–275. doi: 10.1111/1751-7915.13761
- Qin N, Zheng B, Yao J, et al. Influence of H7N9 virus infection and associated treatment on human gut microbiota. Sci Rep. 2015;5:14771. doi: 10.1038/srep14771
- Li W, Zhu Y, Liao Q, et al. Characterization of gut microbiota in children with pulmonary tuberculosis. BMC Pediatr. 2019;19(1):445. doi: 10.1186/s12887-019-1782-2
- Luo M, Liu Y, Wu P, et al. Alternation of gut microbiota in patients with pulmonary tuberculosis. Front Physiol. 2017;8:822. doi: 10.3389/fphys.2017.00822
- Ding L, Liu Y, Wu X, et al. Pathogen metagenomics reveals distinct lung microbiota signatures between bacteriologically confirmed and negative tuberculosis patients. Front Cell Infect Microbiol. 2021;11:708827. doi: 10.3389/fcimb.2021.708827
- Vázquez-Pérez JA, Carrillo CO, Iñiguez-García MA, et al. Alveolar microbiota profile in patients with human pulmonary tuberculosis and interstitial pneumonia. Microb Pathog. 2020;139:103851. doi: 10.1016/j.micpath.2019.103851
- Sala C, Benjak A, Goletti D, et al. Multicenter analysis of sputum microbiota in tuberculosis patients. PLoS One. 2020;15(10):e0240250. doi: 10.1371/journal.pone.0240250
- Xiao G, Cai Z, Guo Q, et al. Insights into the unique lung microbiota profile of pulmonary tuberculosis patients using metagenomic next-generation sequencing. Microbiol Spectr. 2022;10(1):e0190121. doi: 10.1128/spectrum.01901-21
- Krishna P, Jain A, Bisen PS. Microbiome diversity in the sputum of patients with pulmonary tuberculosis. Eur J Clin Microbiol Infect Dis. 2016;35(7):1205–1210. doi: 10.1007/s10096-016-2654-4
- Huang Y, Tang J-H, Cai Z, et al. Alterations in the nasopharyngeal microbiota associated with active and latent tuberculosis. Tuberculosis (Edinb). 2022;136:102231. doi: 10.1016/j.tube.2022.102231
- Yang F, Yang Y, Chen L, et al. The gut microbiota mediates protective immunity against tuberculosis via modulation of lncRNA. Gut Microbes. 2022;14(1):2029997. doi: 10.1080/19490976.2022.2029997
- Naidoo CC, Nyawo GR, Sulaiman I, et al. Anaerobe-enriched gut microbiota predicts pro-inflammatory responses in pulmonary tuberculosis. EBioMedicine. 2021;67:103374. doi: 10.1016/j.ebiom.2021.103374
- Karo-Atar D, Khan N, Divangahi M, et al. Helminth-mediated disease tolerance in TB: a role for microbiota? PLOS Pathog. 2021;17(7):e1009690. doi: 10.1371/journal.ppat.1009690
- Perry S, de Jong BC, Solnick JV, et al. Infection with Helicobacter pylori is associated with protection against tuberculosis. PLoS One. 2010;5(1):e8804. doi: 10.1371/journal.pone.0008804
- Cao D, Liu W, Lyu N, et al. Gut mycobiota dysbiosis in pulmonary tuberculosis patients undergoing anti-tuberculosis treatment. Microbiol Spectr. 2021;9(3):e0061521. doi: 10.1128/spectrum.00615-21
- Hu Y, Kang Y, Liu X, et al. Distinct lung microbial community states in patients with pulmonary tuberculosis. Sci China Life Sci. 2020;63(10):1522–1533. doi: 10.1007/s11427-019-1614-0
- Namasivayam S, Maiga M, Yuan W, et al. Longitudinal profiling reveals a persistent intestinal dysbiosis triggered by conventional anti-tuberculosis therapy. Microbiome. 2017;5(1):71. doi: 10.1186/s40168-017-0286-2
- Kateete DP, Mbabazi MM, Nakazzi F, et al. Sputum microbiota profiles of treatment-naïve TB patients in Uganda before and during first-line therapy. Sci Rep. 2021;11(1):24486. doi: 10.1038/s41598-021-04271-y
- Ueckermann V, Lebre P, Geldenhuys J, et al. The lung microbiome in HIV-positive patients with active pulmonary tuberculosis. Sci Rep. 2022;12(1):8975. doi: 10.1038/s41598-022-12970-3
- Sathkumara HD, Eaton JL, Field MA, et al. A murine model of tuberculosis/type 2 diabetes comorbidity for investigating the microbiome, metabolome and associated immune parameters. Animal Model Exp Med. 2021;4(2):181–188. doi: 10.1002/ame2.12159
- Wensel CR, Pluznick JL, Salzberg SL, et al. Next-generation sequencing: insights to advance clinical investigations of the microbiome. J Clin Invest. 2022;132(7): doi: 10.1172/JCI154944
- Eribo OA, du Plessis N, Ozturk M, et al. The gut microbiome in tuberculosis susceptibility and treatment response: guilty or not guilty? Cell Mol Life Sci. 2020;77(8):1497–1509. doi: 10.1007/s00018-019-03370-4
- Diallo D, Somboro AM, Diabate S, et al. Antituberculosis therapy and gut microbiota: review of potential host microbiota directed-therapies. Front Cell Infect Microbiol. 2021;11:673100. doi: 10.3389/fcimb.2021.673100
- Chung KF. Potential role of the lung microbiome in shaping Asthma phenotypes. Ann Am Thorac Soc. 2017;14(Supplement_5):S326–S331. doi: 10.1513/AnnalsATS.201702-138AW
- Vaughan A, Frazer ZA, Hansbro PM, et al. COPD and the gut-lung axis: the therapeutic potential of fibre. J Thorac Dis. 2019;11(Suppl 17):S2173–S2180. doi: 10.21037/jtd.2019.10.40
- Liu Y, Wang J, Wu C. Microbiota and Tuberculosis: A Potential Role of Probiotics, and Postbiotics. Front Nutr. 2021;8:626254. doi: 10.3389/fnut.2021.626254
- Miqdady M, Al Mistarihi J, Azaz A, et al. Prebiotics in the infant microbiome: the past, present, and future. Pediatr Gastroenterol Hepatol Nutr. 2020;23(1): doi: 10.5223/pghn.2020.23.1.1
- Eribo OA, du Plessis N, Chegou NN, et al. The Intestinal Commensal, Bacteroides fragilis, Modulates Host Responses to Viral Infection and Therapy: Lessons for Exploration during Mycobacterium tuberculosis Infection. Infect Immun. 2022;90(1):e0032121. doi: 10.1128/IAI.00321-21
- Lin D, Wang X, Li Y, et al. Sputum microbiota as a potential diagnostic marker for multidrug-resistant tuberculosis. Int J Med Sci. 2021;18(9):1935–1945. doi: 10.7150/ijms.53492
- Wiqoyah N, Mertaniasih NM, Artama WT, et al. Microbiome in sputum as a potential biomarker of chronicity in pulmonary resistant to rifampicin-tuberculosis and multidrug-resistant-tuberculosis patients. Int J Mycobacteriol. 2021;10(3):260–267. doi: 10.4103/ijmy.ijmy_132_21
- Negatu DA, Yamada Y, Xi Y, et al. Gut Microbiota Metabolite Indole Propionic Acid Targets Tryptophan Biosynthesis in Mycobacterium tuberculosis. MBio. 2019 Mar 26;10(2). doi: 10.1128/mBio.02781-18