ABSTRACT
Introduction: Pharmacological manipulation of signalling pathways is becoming an increasingly important avenue for the rational clinical management of disease but is hampered by a lack of technologies that allow the generation of comprehensive descriptions of cellular signalling.
Areas covered: Herein, the authors discuss the potential of peptide array-based kinome profiling for evaluating cellular signalling in the context of drug discovery.
Expert commentary: Genomic and proteomic approaches have been of significant value to our elucidation of the molecular mechanisms that govern physiology. However, an equally, if not more important goal, is to define those proteins that participate in signalling pathways that ultimately control cell fate, especially kinases. Traditional genetic and biochemical approaches can certainly provide answers here, but for technical and practical reasons, are typically pursued one gene or pathway at a time. A more comprehensive approach is one in which peptide arrays of kinase-specific substrates are incubated with cell lysates and 33P-ATP generating comprehensive descriptions, or where arrays are interrogated with phosphospecific antibodies. Both approaches allow analysis of cellular signalling without a priori assumptions to possibly influenced pathways.
1. Introduction
Pharmacological or biological inhibition of signaling pathways has delivered significant results with respect to devising rational therapy for disease [Citation1], including oncological [Citation2] and inflammatory diseases [Citation3]. As kinases are at the basis of most signaling pathways [Citation4] and are highly druggable [Citation5], this class of enzymes attracts most interest with respect to identifying novel avenues for therapy. Thus, the pursuit of technologies capable of robust characterization of kinase enzymatic activity in patient tissue samples or preclinical material is subject of intense investigation. Previously, in 2012 we reviewed the body of literature on this subject [Citation6]. However, since important progress has been made, with respect to analysis of data obtained, with respect to substrate design, and with respect to novel technological approaches. Especially on systems medicine, progress seems substantial. Thus in the present work we provide a comprehensive overview of the current state and outlook for the group of technologies involved and present our view on their disadvantages and advantages for systems medicine approaches to identifying kinase targets in disease.
2. Eukaryotic and prokaryotic kinases
Almost all members of the superfamily of kinase enzymes can be identified from their primary sequence by the presence of a distinctive catalytic eukaryotic protein kinase (ePK) domain that consists of approximately 250 amino acid residues, although a tiny fraction of protein kinases apparently do not contain this catalytic domain and hence are denominated atypical kinases [Citation7]. A comparison of the primary sequence of kinase domains of otological kinases between species and different kinases within species reveals substantial diversity and reflects the specificity of the reactions catalyzed. Further to specificity comes the non-catalytic protein domains that kinases harbor and that are apparently implicated in the regulation of kinase activity, the interactions with heteromerising protein partners, or their subcellular localization [Citation8,Citation9]. In conjunction, the variation in the catalytic and non-catalytic domains, these attributes can largely explain the variation in kinase reactions catalyzed. Nevertheless, it is possible to define a set of kinase reactions that is common to all eukaryotes and thus probably vital to eukaryotic life [Citation10]. In apparent agreement, in kinome profiling approaches similar peptide substrates have been used to profile highly different organisms, e.g. zebrafish [Citation11] and human leukemia cells [Citation12], suggesting that the variety in primary sequence of kinases is not reflected in substantial differences in the reactions catalyzed. This has not hampered, however, the advent of species-specific substrate platforms [Citation13,Citation14], thus apparently the meritocratic view is that the subtle differences that do exist, still require interrogation of the kinome by customized platform sets. It should prove interesting to observe how the field will develop in the coming years in this respect.
In this context it is interesting to note that an embryonic prokaryotic kinome profiling field is developing as well. Many prokaryotic organisms evidently harbor kinases in their genome, both belonging to the largely prokaryotic-specific family of histidine-aspartate kinases [Citation15] as well as seemingly eukaryote-like kinases [Citation16]. An analysis of the Physarum polycephalum genome demonstrated extensive use of the two-component-type prokaryotic kinases as well of metazoan-type tyrosine kinase-like genes [Citation17]. Approaches involving peptide arrays for characterizing the enzymatic activity towards substrates are now starting to be used for characterizing these elements [Citation18] and are used to study modulation of their activity during infection [Citation19]. As antibiotic resistance is emerging as serious health problem requiring radically novel approaches (e.g. acute pancreatitis is good example [Citation20]) targeting bacterial kinases could be useful. Indeed, the action of various novel anti-biotic antibiotics isolated from natural sources has been linked to their capacity to deregulate phosphorylation status [Citation21] for more than a decade. However, it has still not been formally proved that dysfunctional prokaryotic phosphorylation/dephosphorylation is responsible for the antibiotic properties of natural compounds. Circumstantial evidence is quite strong, though. The LysR-type transcriptional regulator responsible for virulence of Rhodococcus fascians, for instance, drives expression of a kinase [Citation22]. If proof that prokaryotic kinase inhibitors are useful especially for combating infection would emerge, prokaryotic kinome profiling could be exceedingly useful for the discovery of new antibiotics that work through specific interference of bacterial phosphorylation events. In that case prokaryotic kinome profiling, aimed at specifically measuring the enzymatic activity of different prokaryotic kinases would probably see very rapid development, maybe even to the level of shotgun proteomics of bacterial pathogens that has recently become important [Citation23].
3. Peptide arraying remains dominant for kinome profiling
The rise of new generation sequencing and RNAseq is currently eclipsing massive parallel analysis using array technology as the mainstay for the analysis of genomes and transcriptomes [Citation24]. Likewise, mass-spec technologies are becoming very powerful for generating a snapshot of cellular metabolism and protein content, especially for the analysis of clinical material [Citation25]. For now, however, array-like platforms remain the main mode of analysis of specific kinase activities in a mixture of different phosphorylating enzymes and high-impact papers employing this technology to address important clinical issues are regularly published. Important recent clinical advances dependent on such technology include the identification of PAK2 as a relevant target in tuberous sclerosis complex (TSC). TSC is caused by the germline transmission of an inactivating mutation in either TSC1 or TSC2 and upon somatic loss of the second copy, benign hyperplasia occurs, driven by uncontrolled mTORC1 activation. Especially in the brain, the resulting growth is problematic, causing mental defects especially autism, low intelligence, and epilepsy [Citation26]. Accordingly mTORC1 inhibition has revolutionized the clinical management of TSC [Citation27]. mTORC1 inhibition, however, fails to resolve disease completely, leading to the suspicion that other TSC-protein dependent pathways should exist. A recent paper in Scientific Reports [Citation28] showed that this pathway is most likely mediated by the kinase PAK1, a discovery only made possible by array-based kinase profiling. Likewise, in Crohn’s disease, a debilitating manifestation of inflammatory bowel disease apparently caused by deficient mucosal innate immunity and thus failure to control floral infiltration into the intestinal wall [Citation28], array-based kinome profiling appeared the way forward for designing improved drug-based therapy. In Crohn’s disease apparently healthy mucosa alternates with ulcerated intestinal lesions in so-called skip-lesions. In a recent paper in Science Translational Medicine, Parikh and colleagues showed that kinome profiles of uninflamed tissue from patients with Crohn’s disease revealed downregulation of p21Rac1-dependent signaling as compared with inflamed tissue from patients with IBD or even healthy individuals. It appeared that inhibition of Rac signaling constituted a powerful strategy to constrain disease [Citation29,Citation30]. Similarly, Voorneveld and Kodach were interested as to why SMAD4 frequently is lost from colorectal cancers and why this is associated with the development of metastases and poor prognosis. These authors hypothesized that non-canonical bone morphogenetic protein signaling mediates these effects (canonical bone morphogenetic protein signaling acts through SMAD4 [Citation31]). This theory was borne out when the investigators employed kinome profiling using peptide arrays to characterize non-canonical bone morphogenetic protein signaling. In a recent landmark study in the flagship journal Gastroenterology they showed that loss of SMAD4 alters BMP signaling to promote colorectal cancer cell metastasis through activation of Rho and ROCK [Citation32]. Further final high-profile clinical examples are the two related studies of Eveline de Bont cum suis, recently published in Leukemia and Proteomics, on pediatric acute lymphoblastic leukemia. She successfully employed kinome profiling to identify druggable targets in individual children afflicted by this disease [Citation33,Citation34]. Thus the dominance of peptide array formats for identifying clinically useful drugs, which now already lasts for more than a decade [Citation35–Citation38] seems not to be coming to an end.
This impression is reinforced by a likewise dominance of peptide array-based platforms in kinome profiling for veterinary medicine [Citation39]. As opposed to clinical medicine, custom-made array designs are much more common in this field, for instance peptide arrays containing bovine peptides have been successfully employed to identify novel drug targets to combat Jone’s disease, a granulomatous affliction of the intestine that affects cattle [Citation40–Citation42]. Likewise chicken-specific [Citation43], Syrian hamster-specific [Citation13], and even honey bee-specific [Citation44] platforms have been employed to generate specific insight into questions related to veterinary medicine. The results obtained in this field suggest that remarkably species-specific kinotypes appear to exist, supporting the use of custom species-specific peptide arrays [Citation45]. Our own results (), however, albeit unpublished, visually reveal remarkable similarity between kinome profiles B cells isolated from mice or humans, apparently at bay with the notion that species identity is an important factor driving specificity in kinome signatures, but that cellular identity is more important. Indeed investigation in which the different kinotypes of the radiations of the hematopoietic system were contrasted showed that differentiation along the hematopoietic lineage is associated with major remodeling of cellular kinase signature [Citation46]. A caveat here is the exact analysis method employed. Veterinary kinomes are usually analyzed through the Platform for Intelligent, Integrated Kinome Analysis (PIIKA [Citation47]), a quite advanced software tool customized for the analysis of data from kinome arrays (the software can be downloaded from Ref. [Citation47]). For the analysis of clinical data usually less advanced software platforms like the statistical spot reliability approach called PepMatrix [Citation48] are used. It is thus possible that specific kinome patterns recognizable by PIIKA are not recognized by other platforms and comparative studies on the same data set are needed to provide clarity with respect to this issue. In addition, it should be mentioned that arrays must be analyzed with ‘microarray’-style software (PIIKA can do this, but other types of analysis programs often cannot) for comparing spot intensities, subtracting background values, evaluating technical replicates, etc. The Eisen lab software, freely available and often used, can do this, but is designed for nucleotide analysis and not for peptide arrays. Clearly an unmet need exists here. With regard to the issue of species-specific kinotypes, kinome profiling in veterinary medicine remains solidly footed in the use of peptide array-based platforms.
Figure 1. Comparison of phosphorylation patterns on peptide array by lysates made from B cells from humans (left) and mice (right) One can observe that phosphorylation patterns are highly similar between the two species, thus phosphorylation of kinase substrates appears cell-type restricted and not species-restricted. Apparently the divergence in kinase primary sequence between the two species is not reflected in differences in substrate phosphorylation if a similar cell type is used. Another image from the same experiment showing the same phenomenon can also be found in [Citation6].
![Figure 1. Comparison of phosphorylation patterns on peptide array by lysates made from B cells from humans (left) and mice (right) One can observe that phosphorylation patterns are highly similar between the two species, thus phosphorylation of kinase substrates appears cell-type restricted and not species-restricted. Apparently the divergence in kinase primary sequence between the two species is not reflected in differences in substrate phosphorylation if a similar cell type is used. Another image from the same experiment showing the same phenomenon can also be found in [Citation6].](/cms/asset/729a3885-aadf-4ca4-9ae4-0c55a69bc290/ieru_a_1187564_f0001_b.gif)
The latter point is further emphasized by the experience with kinome profiling in plants. Such kinome profiling in plants was pioneered by Tita Ritsema again using a peptide array-based set-up [Citation49–Citation51]. It appears that multiple platforms used elsewhere are also suitable for profiling of enzymatic kinase activities, but all involve peptide arrays on a solid carrier [Citation52]. The kinome profiling in plant field was always hampered by poor annotation with respect to kinase enzymes in these organisms, but this situation has recently drastically improved [Citation53–Citation56] and it is to be expected that this field will take off vigorously now. In toto, there appears to be relatively little new development in the kinome profiling field, with the peptide array reigning supreme since its introduction early this century [Citation57,Citation58]. We shall argue, however, that the hegemony of the peptide array may soon be ending.
Spotted peptide microarrays have been the mainstay for kinome profiling since 2004. The principle of the technology is depicted in . In addition there is a successful fluidic technological variant for kinome profiling that is offered by the Pamgene company, which appears especially suitable for drug discovery. On this platform clinical samples are constantly pumped through a matrix, kinases in the mixture are thus allowed to phosphorylate substrate peptides. Using fluorescently labelled phosphospecific antibodies, peptide phosphorylation is assessed. The porous microarray platform from PamGene differs from other microarray formats in that its continuous flow allows for more reproducible kinase reaction (moreover the substrate has a 500 times greater surface area than conventional planar arrays), offers the possibility to assess the kinetics of the reaction (thus at least theoretically allows measurement of two kinases targeting the same substrate but with different affinity simultaneously) and does not require radioactivity. Phosphospecific antibodies as a measure of phosphorylation have already been validated for 15 years now [Citation59] and thus there is little doubt that the signals obtained through this platform are bona-fide representations of substate phosphorylation. In a landmark study, Sikkema et al. described global tyrosine kinase activity profiles in 30 pediatric brain tumors [Citation60]. Excitingly, substrate peptides containing phosphorylation consensus sequences for Src family kinases showed remarkably strong levels of phosphorylation compared with normal tissue types and the Src family kinase inhibitors PP1 and dasatinib induced substantial tumor cell death in nine cell culture models of pediatric brain tumors and thus identified dasatinib as a therapeutic option for this disease. Currently the main use of the Pamgene platform is inr product development for pharmaceutical industries, but also various academic studies using the platform have been published [Citation61–Citation67]. Arguably, the Pamgene platform is currently the most productive in the field with respect to identifying new drugs for clinical medicine, especially in the context of personalized treatment.
Figure 2. Principle of kinome profiling using peptide arrays. A peptide array is spotted with peptide consensus motifs for the different kinases present in the human genome. As an example, in this picture the consensus sequences of three alternative kinases have been shown including CamK1 (on the left). Upon incubation with a solution that contains active CamK1 kinase and radio-active ATP, the CamK1 kinase substrate sequence will be phosphorylated by the radio-active ATP without parallel phosphorylation of the other substrates. In this fashion, kinases can be assayed in parallel.
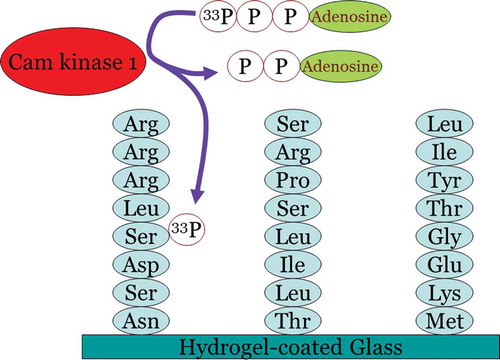
The relative success of a kinome platform that incorporates active fluidics has led to worldwide scientific and commercial recognition that fluidics-based solutions may be the way forward for devising new methods describing kinase signaling as a complex system, rather than as a collection of individual enzymes or pathways.
4. Non-peptide array-based kinome profiling employing artificial substrates: the future for drug discovery?
As stated above, over the years the methodology has been greatly improved, yet the basic design of the substrates used and the biochemical setup has not changed significantly. First and foremost, the set of substrates used on kinome profiling peptide microarrays is based on naturally occurring phosphorylation sites in proteins. Although these sites have been described in extenso in the literature and therefore thoroughly annotated [Citation68], it is very unlikely that they represent the most optimal substrate set for kinome profiling. They have neither been optimized for selectivity for specific kinases, nor have they been optimized for use tethered on a microarray surface. Indeed, analysis of substrate phosphorylation by the purified kinases c-Raf and c-Cot [Citation69] suggested that the optimal substrates for these kinases were not present on a popular and successful peptide array format [Citation70] and that optimal substrate peptides do not correspond with the actual phosphorylation sites as present in substrate proteins for c-Raf and c-Cot. A theoretical appealing approach for designing new substrates is the Predikin framework, which can conceivably be employed for improved substrate selection that detect and discriminate activity of different kinases better [Citation71,Citation72]. Predikin entails a theoretical analysis of all known substrates for the related kinases. Data presented at STW kinome profiling meeting in Rotterdam by Jetse Scholma showed that when a CDK1 and MAPK1 were analyzed using the Predikin framework, natural substrates performed relatively poorly with respect to specificity and sensitivity, and this was confirmed in mass spectrometry-based kinase assays. Two sets of six peptides were designed to detect and discriminate activity of CDK1 and MAPK1. One of these sets was optimized for sensitivity and outperformed the known, natural substrates for both kinases. Efforts using Predikin for better substrate design are currently being pursued by various parties, in particular by the Pepscope company that tries to develop a novel peptide substrate set and has attracted substantial funding for this endeavor. Optimized for kinase selectivity and interpretability and artificial substrates might constitute the next leap forward in protein kinase profiling for drug discovery.
Further improvements may come from non-isotopic mass spectrometry-based readouts. As mass spectrometry can easily distinguish different substrate peptides by size and distinguish phosphorylated form of peptides from unphosphorylated forms it is theoretically suitable for performing complex kinome measurements. It should, for instance, be possible to incubate different substrate peptides with clinical samples and upon subsequent extraction of the substrate peptides from the mixture (e.g. by coupling peptides to magnetic beads and subsequent magnetic separation, compare e.g [Citation73].) the unphoshorylated levels of these peptides can be compared with the phosphorylated levels of these peptides. A ratio then provides information on the relative capacity of the clinical sample to mediate phosphorylation of the peptide and thus provides information on kinase activity towards this substrate peptide in the clinical sample. Further revolution of the application of kinome profiling for personalized medicine and drug development is only a matter of time.
5. Expert commentary
Despite its power for generating comprehensive descriptions of cellular signaling, the use of kinome profiling remains for most areas of contemporary biomedical research somewhat of a niche application, with the exception of drug and target discovery and to a lesser extent also personalized medicine can be considered an exception. Most researchers, even when dealing with essentially biochemical phenomena, like signal transduction, prefer the use of transcriptomic approaches over proteomic and kinomic investigations, because of their better availability, the presence of core-facilities in institutions, the more-developed bio-informatical analysis tools, and also because of financial reasons. The pamgene platform, which is relatively accessible in these terms, is now making deeper market penetration, whereas Pepscope offers very attractive comprehensive kinome profiling platforms that substantially lower barriers of entry of researchers for using this technology. Pepscan is also becoming more active in this respect. Along with the continuous development of better analysis tools, it is to be expected that the reach of kinome profiling will break out of its pharmaceutical context and enter mainstream basal and translational research. Important factors in this context are also budding prokaryotic and plant-kinomic fields, which will serve as examples for others.
A very welcome development is the establishment of large biobanks based on large cohorts of patients and screening populations which contain kinome-profilable biomaterial coupled to very high quality clinical data. Examples are biobanks of patients with pancreatitis [Citation74], screening populations for colon cancers, or those containing resection material of esophageal atresia patients and multi-decade follow up [Citation75,Citation76]. Kinome profiling of these cohorts will help identifying the exact biochemical traits that determine disease progression and allow for rational implementation of targeted drugs. Likewise comparative epithelial organoid tissue banks are suitable for kinome profiling and can be linked to specific traits in different species and tissue types [Citation77] If kinome profiling is to fulfil this promise, however, the quality of the starting material and especially clinical description and follow-up needs to be of the highest quality. Fortunately biobanking has progressed to such an extent that that prerequisite is now often met.
6. Five-year view
Kinome profiling remains an important technique for drug discovery, especially in the context of personalized medicine. It is clear that the field of tailored medicine is evolving fast, especially in oncological medicine. A case in the point is pancreatic ductal adenocarcinoma, an usually lethal disease, resistant against therapy, but a subpopulation of PDACs shows strong activation of the mTOR signaling cassette and reacts well to pharmacological intervention with inhibitors of this pathway [Citation78]. However, other subpopulations of pancreatic cancer react to completely different types of drugs [Citation79]. Identifying those subpopulations likely to react to one drug or another is now typically pursued one pathway or molecule at the time and is for most clinical practice considered too cumbersome for use in routine medicine [Citation80]. However, better selection of patients can and will improve patient outcome in oncological disease, also in the 5 years to come. Kinome profiling offers a way forward here: with its unrivaled potential to characterize pathways active in clinical samples without a prioiri assumptions as to the signaling present in a sample, potentially useful drugs can easily be selected. Many drawbacks still exist, most related to the logistic difficulties of timely transport of analyzable samples to a diagnostic laboratory and the difficulties of introducing the technology into such laboratories. Spotted arrays require radio-active detection, an absolute no-go in most routine settings, whereas the non-radioactive pamgene format is mainly useful for tyrosine kinase profiling, which delivers too little price to value for most routine applications. Pamchip 4 currently provides phosphotyrosine or phosphoserine/threonine fluorescent reactions, but its usefulness is for now limited, as it does measure protein kinase B activity quite well, but many other kinase not so well. Thus if kinome profiling is to make its breakthrough in routine medicine for drug discovery, either non-radioactive formats also suitable serine/threonine kinase profiling should be introduced or the price for the existing non-radioactive formats should be substantially reduced. Encouragingly, with respect to both aspects progress seems to be made. If such development indeed materializes one can expect that kinome profiling can also contribute to more and better personalized medicine in other areas of disease, e.g. cystic fibrosis [Citation81].
With respect to analysis of the signals obtained by kinome profiling still substantial work to be done. Various analysis platforms have been developed around the globe but there is an urgent need for standardization, also to better compare results obtained at different locations. Perhaps the field should organize a consensus meeting to jointly discuss this. Furthermore, especially for spotted arrays there is an urgent need to improve software. Especially intraarray and interarray normalization of peptide array phosphorylation remain problematic, due to the absence of ‘household’ kinases and the obvious fallacy of the assumption that different experimental conditions should exhibit equal amounts of kinase activity. Efforts on the way leading to development of analysis tools that reliably quantify phosphorylation of peptide arrays and that allow normalization of the signals obtained, as is for instance now pursued at the Technical University of Twente should thus prove instrumental in moving the field forward.
Finally, the development of revolutionary new mass spectrometry-based assay systems has substantial potential to deliver significant results. Again, preliminary work looks promising, but nothing has been published in peer-reviewed journals. Nevertheless, in the light of these developments it looks highly unlikely if the advent of kinome profiling for drug discovery will come to a halt soon.
Key issues
Kinome profiling is unusual in that provides comprehensive overview of cellular signalling based on kinase enzymatic activities rather as the steady state determinations
Kinome profiling is becoming the leading technique for drug selection in experimental personalized medicine
Specialized veterinary medicine kinome profiling, prokaryotic kinome profiling and kinome profiling in plant biology fields are emerging
The issue whether species-customised arrays are necessary is not resolved
Arrays of immobilised peptides continue to dominate the field
Artificial substrates allowing greater sensitivity and specificity towards individual kinases are emerging and changing the field
Liquid phase phosphorylation of soluble peptides represent a dramatic rupture with past practices but may bring kinome profiling into routine medicine
Declaration of interest
The authors have no relevant affiliations or financial involvement with any organization or entity with a financial interest in or financial conflict with the subject matter or materials discussed in the manuscript. This includes employment, consultancies, honoraria, stock ownership or options, expert testimony, grants or patents received or pending, or royalties.
References
- Kosako H, Nagano K. Quantitative phosphoproteomics strategies for understanding protein kinase-mediated signal transduction pathways. Expert Rev Proteomics. 2011;8:81–94.
- van Veelen W, Korsse SE, van de Laar L, et al. The long and winding road to rational treatment of cancer associated with LKB1/AMPK/TSC/mTORC1 signaling. Oncogene. 2011;30:2289–2303.
- den Hollander WJ, Kuipers EJ. Current pharmacotherapy options for gastritis. Expert Opin Pharmacother. 2012;13:2625–2636.
- Mok J, Zhu X, Snyder M. Dissecting phosphorylation networks: lessons learned from yeast. Expert Rev Proteomics. 2011;8:775–786.
- Volkamer A, Eid S, Turk S, et al. Pocketome of human kinases: prioritizing the ATP binding sites of (yet) untapped protein kinases for drug discovery. J Chem Inf Model. 2015;55:538–549.
- Peppelenbosch MP. Kinome profiling. Scientifica (Cairo). 2012; 2012:306798.
- Johnson SA, Hunter T. Kinomics: methods for deciphering the kinome. Nat Methods. 2005;2(1):17–25.
- Creixell P, Palmeri A, Miller CJ, et al. Unmasking determinants of specificity in the human kinome. Cell. 2015;163:187–201.
- Creixell P, Schoof EM, Simpson CD, et al. Kinome-wide decoding of network-attacking mutations rewiring cancer signaling. Cell. 2015;163:202–217.
- Diks SH, Parikh K, van der Sijde M, et al. Peppelenbosch MP. Evidence for a minimal eukaryotic phosphoproteome? PLoS One. 2007;2(8):e777.
- Lemeer S, Ruijtenbeek R, Pinkse MW, et al. Endogenous phosphotyrosine signaling in zebrafish embryos. Mol Cell Proteomics. 2007;6:2088–2099.
- Sikkema AH, den Dunnen WF, Diks SH, et al. Optimizing targeted cancer therapy: towards clinical application of systems biology approaches. Crit Rev Oncol Hematol. 2012;82:171–186.
- Falcinelli S, Gowen BB, Trost B, et al. Characterization of the host response to pichinde virus infection in the Syrian golden hamster by species-specific kinome analysis. Mol Cell Proteomics. 2015;14:646–657.
- Trost B, Kindrachuk J, Scruten E, et al. Kinotypes: stable species- and individual-specific profiles of cellular kinase activity. BMC Genomics. 2013;14:854.
- Attwood PV. Histidine kinases from bacteria to humans. Biochem Soc Trans. 2013;41:1023–1028.
- Pereira SF, Goss L, Dworkin J. Eukaryote-like serine/threonine kinases and phosphatases in bacteria. Microbiol Mol Biol Rev. 2011;75(1):192–212.
- Schaap P, Barrantes I, Minx P, et al. The Physarum polycephalum genome reveals extensive use of prokaryotic two-component and metazoan-type tyrosine kinase signaling. Genome Biol Evol. 2015;8:109–125.
- Miller M, Dreisbach A, Otto A, et al. Mapping of interactions between human macrophages and Staphylococcus aureus reveals an involvement of MAP kinase signaling in the host defense. J Proteome Res. 2011;10:4018–4032.
- Miller M, Donat S, Rakette S, et al. Staphylococcal PknB as the first prokaryotic representative of the proline-directed kinases. PLoS One. 2010;5(2):e9057.
- Bakker OJ, Issa Y, van Santvoort HC, et al. Treatment options for acute pancreatitis. Nat Rev Gastroenterol Hepatol. 2014;11:462–469.
- Ferreira CV, Justo GZ, Souza AC, et al. Natural compounds as a source of protein tyrosine phosphatase inhibitors: application to the rational design of small-molecule derivatives. Biochimie. 2006;88:1859–1873.
- Maes T, Vereecke D, Ritsema T, et al. The att locus of Rhodococcus fascians strain D188 is essential for full virulence through the production of an autoregulatory compound. Mol Microbiol. 2001;42:13–28.
- Semanjski M, Macek B. Shotgun proteomics of bacterial pathogens: advances, challenges and clinical implications. Expert Rev Proteomics. 2016;13:139–156.
- Mantione KJ, Kream RM, Kuzelova H, et al. Comparing bioinformatic gene expression profiling methods: microarray and RNA-Seq. Med Sci Monit Basic Res. 2014;20:138–142.
- Subbannayya Y, Pinto SM, Gowda H, et al. Proteogenomics for understanding oncology: recent advances and future prospects. Expert Rev Proteomics. 2016;25:1–12.
- Korsse SE, Peppelenbosch MP, van Veelen W. Targeting LKB1 signaling in cancer. Biochim Biophys Acta. 2013;1835:194–210.
- Franz DN, Belousova E, Sparagana S, et al. Everolimus for subependymal giant cell astrocytoma in patients with tuberous sclerosis complex: 2-year open-label extension of the randomised EXIST-1 study. Lancet Oncol. 2014;15:1513–1520.
- Alves MM, Fuhler GM, Queiroz KC, et al. PAK2 is an effector of TSC1/2 signaling independent of mTOR and a potential therapeutic target for tuberous sclerosis complex. Sci Rep. 2015;5:14534.
- Comalada M, Peppelenbosch MP. Impaired innate immunity in Crohn’s disease. Trends Mol Med. 2006;12:397–399.
- Parikh K, Zhou L, Somasundaram R, et al. Suppression of p21Rac signaling and increased innate immunity mediate remission in Crohn’s disease. Sci Transl Med. 2014;6:233ra53.
- Hardwick JC, Kodach LL, Offerhaus GJ. van den Brink GR. Bone morphogenetic protein signalling in colorectal cancer. Nat Rev Cancer. 2008;8:806–812.
- Voorneveld PW, Kodach LL, Jacobs RJ, et al. Loss of SMAD4 alters BMP signaling to promote colorectal cancer cell metastasis via activation of Rho and ROCK. Gastroenterology. 2014;147:196–208.
- van der Sligte NE, Scherpen FJ, Meeuwsen-de Boer TG, et al. Kinase activity profiling reveals active signal transduction pathways in pediatric acute lymphoblastic leukemia: a new approach for target discovery. Proteomics. 2015;15:1245–1254.
- Kampen KR, Ter Elst A, Mahmud H. Insights in dynamic kinome reprogramming as a consequence of MEK inhibition in MLL-rearranged AML. Leukemia. 2014;28:589–599.
- Azijli K, Yuvaraj S, Peppelenbosch MP, et al. Kinome profiling of non-canonical TRAIL signaling reveals RIP1-Src-STAT3-dependent invasion in resistant non-small cell lung cancer cells. J Cell Sci. 2012;125:4651–4661.
- Bralten LB, Kloosterhof NK, Balvers R, et al. IDH1 R132H decreases proliferation of glioma cell lines in vitro and in vivo. Ann Neurol. 2011;69:455–463.
- Löwenberg M, Tuynman J, Scheffer M, et al. Kinome analysis reveals nongenomic glucocorticoid receptor-dependent inhibition of insulin signaling. Endocrinology. 2006;147:3555–3562.
- van Baal JW, Diks SH, Wanders RJ, et al. Comparison of kinome profiles of Barrett’s esophagus with normal squamous esophagus and normal gastric cardia. Cancer Res. 2006;66:11605–11612.
- Li Y, Arsenault RJ, Trost B, et al. A systematic approach for analysis of peptide array kinome data. Sci Signal. 2012;5(220):pl2.
- Arsenault RJ, Li Y, Bell K, et al. Mycobacterium avium subsp. paratuberculosis inhibits gamma interferon-induced signaling in bovine monocytes: insights into the cellular mechanisms of Johne’s disease. Infect Immun. 2012;80:3039–3048.
- Arsenault RJ, Li Y, Maattanen P, et al. Altered Toll-like receptor 9 signaling in mycobacterium avium subsp. paratuberculosis-infected bovine monocytes reveals potential therapeutic targets. Infect Immun. 2013;81(1):226–237.
- Määttänen P, Trost B, Scruten E, et al. Divergent immune responses to mycobacterium avium subsp. paratuberculosis infection correlate with kinome responses at the site of intestinal infection. Infect Immun. 2013;81(8):2861–2872.
- Napper S, Dadgar S, Arsenault RJ, et al. Induction of tissue- and stressor-specific kinomic responses in chickens exposed to hot and cold stresses. Poult Sci. 2015;94:1333–1345.
- Robertson AJ, Trost B, Scruten E. Identification of developmentally-specific kinotypes and mechanisms of Varroa mite resistance through whole-organism, kinome analysis of honeybee. Front Genet. 2014;5:139.
- Trost B, Arsenault R, Griebel P, et al. Dapple: a pipeline for the homology-based prediction of phosphorylation sites. Bioinformatics. 2013;29(13):1693–1695.
- Hazen AL, Diks SH, Wahle JA, et al. Major remodelling of the murine stem cell kinome following differentiation in the hematopoietic compartment. J Proteome Res. 2011;10:3542–3550.
- Trost B, Kindrachuk J, Määttänen P, et al. PIIKA 2: an expanded, web-based platform for analysis of kinome microarray data. PLoS One. 2013;8(11):e80837.
- Milani R, Ferreira CV, Granjeiro JM, et al. Phosphoproteome reveals an atlas of protein signaling networks during osteoblast adhesion. J Cell Biochem. 2010;109:957–966.
- Ritsema T, Joore J, van Workum W, et al. Kinome profiling of Arabidopsis using arrays of kinase consensus substrates. Plant Methods. 2007;3:3.
- Ritsema T, Brodmann D, Diks SH, et al. Are small GTPases signal hubs in sugar-mediated induction of fructan biosynthesis? PLoS One. 2009;4(8):e6605.
- Ritsema T, van Zanten M, Leon-Reyes A. Kinome profiling reveals an interaction between jasmonate, salicylate and light control of hyponastic petiole growth in Arabidopsis thaliana. PLoS One. 2010;5(12):e14255.
- Ritsema T, Peppelenbosch MP. Kinome profiling of sugar signaling in plants using multiple platforms. Plant Signal Behav. 2009;4:1169–1173.
- Liu J, Chen N, Grant JN, et al. Soybean kinome: functional classification and gene expression patterns. J Exp Bot. 2015;66:1919–1934.
- Hindle MM, Martin SF, Noordally ZB, et al. The reduced kinome of Ostreococcus tauri: core eukaryotic signalling components in a tractable model species. BMC Genomics. 2014;15:640.
- Zulawski M, Schulze G, Braginets R, et al. The Arabidopsis Kinome: phylogeny and evolutionary insights into functional diversification. BMC Genomics. 2014;15:548.
- Singh DK, Calviño M, Brauer EK, et al. The tomato kinome and the tomato kinase library ORFeome: novel resources for the study of kinases and signal transduction in tomato and solanaceae species. Mol Plant Microbe Interact. 2014;27:7–17.
- Houseman BT, Huh JH, Kron SJ, et al. Peptide chips for the quantitative evaluation of protein kinase activity. Nat Biotechnol. 2002;20:270–274.
- Diks SH, Kok K, O’Toole T, et al. Kinome profiling for studying lipopolysaccharide signal transduction in human peripheral blood mononuclear cells. J Biol Chem. 2004;279:49206–49213.
- Versteeg HH, Nijhuis E, van den Brink GR, et al. A new phosphospecific cell-based ELISA for p42/p44 mitogen-activated protein kinase (MAPK), p38 MAPK, protein kinase B and cAMP-response-element-binding protein. Biochem J. 2000;350:717–722.
- Sikkema AH, Diks SH, den Dunnen WF, et al. Kinome profiling in pediatric brain tumors as a new approach for target discovery. Cancer Res. 2009;69:5987–5995.
- Rosenberger AF, Morrema TH, Gerritsen WH, et al. Increased occurrence of protein kinase CK2 in astrocytes in Alzheimer’s disease pathology. J Neuroinflammation. 2016;13:4.
- Rosenberger AF, Hilhorst R, Coart E, et al. Protein kinase activity decreases with higher braak stages of Alzheimer’s disease pathology. J Alzheimers Dis. 2015;49:927–943.
- Anderson JC, Willey CD, Mehta A, et al. High throughput kinomic profiling of human clear cell renal cell carcinoma identifies kinase activity dependent molecular subtypes. PLoS One. 2015;10(9):e0139267.
- Sanz Sanz A, Niranjan Y, Hammarén H, et al. The JH2 domain and SH2-JH2 linker regulate JAK2 activity: A detailed kinetic analysis of wild type and V617F mutant kinase domains. Biochim Biophys Acta. 2014;1844:1835–1841.
- Nwachukwu JC, Srinivasan S, Bruno NE, et al. Resveratrol modulates the inflammatory response via an estrogen receptor-signal integration network. Elife. 2014;3:e02057.
- Kawada I, Hasina R, Arif Q. Dramatic antitumor effects of the dual MET/RON small-molecule inhibitor LY2801653 in non-small cell lung cancer. Cancer Res. 2014;74:884–895.
- Eriksson A, Kalushkova A, Jarvius M, et al. AKN-028 induces cell cycle arrest, downregulation of Myc associated genes and dose dependent reduction of tyrosine kinase activity in acute myeloid leukemia. Biochem Pharmacol. 2014;87:284–291.
- MacDonald JA, Mackey AJ, Pearson WR, et al. A strategy for the rapid identification of phosphorylation sites in the phosphoproteome. Mol Cell Proteomics. 2002;1:314–322.
- Parikh K, Diks SH, Tuynman JH, et al. Comparison of peptide array substrate phosphorylation of c-Raf and mitogen activated protein kinase kinase kinase 8. PLoS One. 2009;4(7):e6440.
- Tuynman JB, Vermeulen L, Boon EM, et al. Cyclooxygenase-2 inhibition inhibits c-Met kinase activity and Wnt activity in colon cancer. Cancer Res. 2008;68(4):1213–1220.
- Ellis JJ, Kobe B. Predicting protein kinase specificity: predikin update and performance in the DREAM4 challenge. PLoS One. 2011;6(7):e21169.
- Saunders NF, Brinkworth RI, Huber T, et al. A computational framework for the prediction of protein kinase peptide specificity and an associated database of phosphorylation sites. BMC Bioinformatics. 2008;9:245.
- O’Brien LM, Stewart LD, Strain SA, et al. Novel monoclonal antibody and peptide binders for mycobacterium avium subsp. paratuberculosis and their application for magnetic separation. PLoS One. 2016;11:e0147870.
- Issa Y, Bruno MJ, Bakker OJ, et al. Treatment options for chronic pancreatitis. Nat Rev Gastroenterol Hepatol. 2014;11:556–564.
- Schreuders EH, Ruco A, Rabeneck L, et al. Colorectal cancer screening: a global overview of existing programmes. Gut. 2015;64:1637–1649.
- Vergouwe FW, IJsselstijn H, Wijnen RM, et al. Screening and surveillance in esophageal atresia patients: current knowledge and future perspectives. Eur J Pediatr Surg. 2015;25:345–352.
- Schwarz JS, De Jonge HR, Forrest JN Jr. Value of organoids from comparative epithelia models. Yale J Biol Med. 2015;88(4):367–374.
- Utomo WK, Narayanan V, Biermann K, et al. mTOR is a promising therapeutical target in a subpopulation of pancreatic adenocarcinoma. Cancer Lett. 2014;346:309–317.
- Sideras K, Braat H, Kwekkeboom J, et al. Role of the immune system in pancreatic cancer progression and immune modulating treatment strategies. Cancer Treat Rev. 2014;40:513–522.
- Braat H, Bruno M, Kuipers EJ, et al. Pancreatic cancer: promise for personalised medicine? Cancer Lett. 2012;1(318):1–8.
- Ikpa PT, Bijvelds MJ, de Jonge HR. Cystic fibrosis: toward personalized therapies. Int J Biochem Cell Biol. 2014;52:192–200.