ABSTRACT
This review gives an overview of current screening practices for cervical cancer. In the introduction, we will cover approaches of population screening focusing on high-risk Human Papilloma Virus (hrHPV) and the need for a better triage assay. We will further assess the impact of current vaccination programs on screening. Subsequently, the review will cover various technological aspects of nucleic acid- and protein-based biomarker assays. We will then detail different molecular markers in view of their use in triage assays, emphasizing epigenetic and protein markers. Finally, we will place this in the context of cost-effectiveness considerations in view of their implementation in high- as well as in low- to middle-income countries.
Introduction
Cervical cancer remains a significant healthcare problem, notably in low- to middle-income countries. While a negative test for hrHPV has a predictive value of more than 99.5%, its positive predictive value is less than 10% for CIN2+ stages. This makes the use of a so-called triage test indispensable for population-based screening to avoid referring women, that are ultimately at low risk of developing cervical cancer, to a gynecologist. This review will give an overview of tests that are based on epigenetic marker panels and protein markers.
Areas covered
There is a medical need for molecular markers with a better predictive value to discriminate hrHPV-positive women that are at risk of developing cervical cancer from those that are not. Areas covered are epigenetic and protein markers as well as health economic considerations in view of the fact that most cases of cervical cancer arise in low-to-middle-income countries.
Expert opinion
While there are biomarker assays based on changes at the nucleic acid (DNA methylation patterns, miRNAs) and at the protein level, they are not widely used in population screening. Combining nucleic acid-based and protein-based tests could improve the overall specificity for discriminating CIN2+ lesions that carry a low risk of progressing to cervical cancer within the screening interval from those that carry an elevated risk. The challenge is to reduce unnecessary referrals without an undesired increase in false-negative diagnoses resulting in cases of cervical cancer that could have been prevented. A further challenge is to develop tests for low-and middle-income countries, which is critical to reduce the worldwide burden of cervical cancer.
1. Introduction
Population screening has had a major impact on reducing the incidence of cervical cancer in high-income countries. However, screening is much less consistently done in low- to middle-income countries, where the incidence of cervical cancer is still high. Since testing for the presence of hrHPV has replaced the original cytological examination (PAP or LBC tests), there is a need for better triage to reduce the number of women that are referred to a gynecologist without being at risk for developing cervical cancer during the next screening interval. This review focuses on the different options currently available or under investigation for triage after a positive hrHPV test.
1.1. Population screening
Cervical cancer is the third-most-common cancer among women worldwide [Citation1], with most cases occurring in middle-and low-income countries (Table S1 and Figure S1). The original, primary diagnostic methods for detecting cervical cancer at an early stage are the cytomorphological PAP and liquid-based cytology (LBC) tests that evaluate the nuclear morphology of cervical epithelial cells. These tests have a high inter-observer variability (34–94% sensitivity) as their main limitation [Citation2]. More recently, these tests have been replaced by PCR-based assays for the presence of hrHPV in a number of high-income countries [Citation3]. While having an excellent negative predictive value, these assays lack specificity resulting in unnecessary referrals to a gynecologist and overtreatment ().
Figure 1. Flow chart of Dutch population-based screening for cervical cancer (numbers are from 2019). ASCUS (Atypical Squamous Cells of Undetermined Significance) [Citation4]
![Figure 1. Flow chart of Dutch population-based screening for cervical cancer (numbers are from 2019). ASCUS (Atypical Squamous Cells of Undetermined Significance) [Citation4]](/cms/asset/ac6bb0a5-aa30-45e8-87b1-a69f2e9feb71/ieru_a_1980387_f0001_oc.jpg)
To discover more specific biomarkers and develop and validate new tests, it is necessary to perform prospective studies and link test results to a relevant endpoint (e.g. CIN2+ with an elevated risk of progression). To this end, samples from population screening must be kept for many years in biobanks available for research and development. The Dutch RIVM has formulated guidelines for handling such samples, mainly based on cervical scrapings. Cytological specimens are stored for 15 years and made available for scientific research. The samples remain in the respective screening laboratories, but third parties may request them under strictly regulated conditions [Citation5]. For research purposes, patients have to give permission to collect and store pre-treatment and follow-up serum/plasma samples in an anonymous, password-protected database with unique patient codes according to specific guidelines (http://www.federa.org). Such biobanks are invaluable resources for improving current screening procedures and notably for evaluating novel biomarkers for triage after a positive hrHPV test [Citation6].
1.2. Pathology of cervical cancer
Infection with hrHPV is a necessary but not a sufficient condition for developing cervical cancer. Two major types of cervical cancer can be distinguished based on cell types: squamous cell carcinoma, which is determined in 80–90% of the cases, and adenocarcinoma [Citation2]. Squamous cell carcinoma appears in cells covering the lower part of the cervix (exocervix), whereas adenocarcinomas originate in the glandular cells of the upper part of the cervix (endocervix). Progression from normal cervical tissue via the precancerous stages of mild (CIN1) to moderate (CIN2) and severe neoplastic (CIN3) lesions, followed by (metastasized) squamous cell cervical cancer, is well-understood on a morphological level (). However, it is difficult to discriminate between CIN2+ lesions that carry an elevated risk of progressing toward cervical cancer (transforming lesions) during the next screening interval and those that produce viral particles but do not progress to cancer (productive lesions). Different types of molecular biomarkers, including DNA methylation patterns, miRNAs, and specific proteins, are currently being investigated in this respect to survey women with CIN2+ lesions [Citation7].
Figure 2. Progression from normal cervical tissue to CIN3 and further to invasive cervical cancer after an hrHPV infection. Women with a diagnosis of CIN1 are usually left untreated and invited for the next screening interval. The treatment of most women with a diagnosis of CIN2 is successful in preventing progression to the more severe stages, which has greatly diminished the incidence of cervical cancer and the associated mortality, mostly in high-income countries. Treatment success diminishes considerably upon further progression to cervical carcinoma. LBC: a method for preparing cervical samples in cytopathology; PAP: a screening test for the presence of HPV
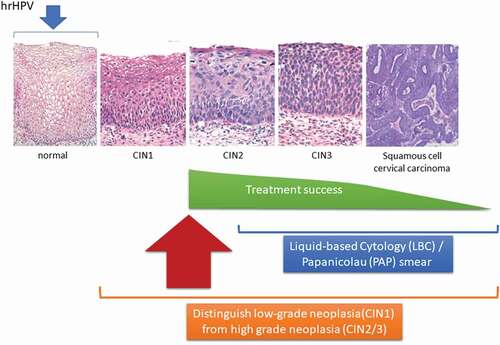
While hrHPV infection is a prerequisite for cervical cancer development, additional molecular changes are required for progression from infection to cervical cancer [Citation7,Citation8]. This process involves the integration of the viral oncogenes E6 and E7 into cellular DNA. Expression of the corresponding proteins inhibits tumor suppressors p53 and pRb, leading to a loss of cell cycle control, proliferation, and ultimately to malignant transformation [Citation9]. Co-expression of p16 and Ki-67, as detected by immunocytochemical methods, is increased in transforming CIN lesions and has been used clinically. Celewicz and coworkers [Citation1] assessed p16/Ki-67 positive cells and found that the sensitivity and specificity of detecting transforming, high-risk CIN2+ lesions increased compared with conventional PAP and LBC tests. A meta-analysis of the p16/Ki-67 dual stain by Chen and coworkers [Citation10] showed high sensitivity (91%) but moderate specificity (64%) for high-grade squamous intraepithelial (HSIL) lesions in patients with an abnormal cytology test.
1.3. Vaccination
Vaccination programs against hrHPV infections started around 2007 in 99 countries [Citation11]. First-generation vaccines, such as the bivalent vaccine, targeting hrHPV-types 16 and 18 and the quadrivalent vaccine, targeting hrHPV-types 6, 11, 16 and 18, effectively prevent infection with the highly oncogenic hrHPV types 16 and 18 found in roughly 70% of invasive cervical cancers [Citation12]. Currently, a nonavalent vaccine (a vaccine that induces an immune response against nine antigens) is used in an increasing number of countries. Approved vaccines contain L1 protein VLPs produced in different cell types [Citation13]. VLPs are morphologically and antigenically similar to native HPV virions providing protection against different types of viruses because of sequence similarity. HPV vaccines elicit immunity by producing high titers of neutralizing anti-HPV IgG antibodies, which block the virus from entering host cells [Citation14]. Current HPV vaccination programs aim to prevent cervical cancer, but they are not effective in curing preexisting HPV infections [Citation13]. In addition, they cover roughly 70% of HPV infections that may lead to cervical cancer, although this percentage is higher for the recently introduced nonavalent vaccine.
Matthijsse and coworkers [Citation15] used a mathematical microsimulation model to estimate that for the first cohorts who were offered HPV vaccination (uptake 50–60%) in the Netherlands the occurrence of cervical cancer and cancer-related deaths will be reduced by roughly 35% compared to primary hrHPV screening only (without vaccination). The NNV to gain one life year is 45 girls aged 12–29 years. Estimated relative reductions in the incidence of HPV-16 infections for the first four successive five-year birth cohorts (vaccinated and unvaccinated) that underwent the current girls-only vaccination program compared to no vaccination were calculated to range from 25% for cohort 1 (1993–1997) to 65% for cohort 4 (2008–2012) at the age of 15 years [Citation5].
Drolet et al. [Citation11] looked at the frequency of HPV-related endpoints (genital HPV infections, anogenital wart diagnosis, or histologically confirmed CIN2+) between the pre-vaccination and post-vaccination periods. Their review of data from 14 high-income countries (annual gross national income per capita of US$ 12,536 and more) shows a significant and substantial impact of HPV vaccination on these endpoints in the first nine years after the start of the vaccination programs. NNV and cost-effectiveness analyses in high-income countries suggest that vaccination of multiple cohorts of individuals up to 18 years is highly cost-effective while strongly depending on the price of the vaccine [Citation16,Citation17].
In 2018, 80% of new cervical cancer cases worldwide occurred in low- and middle-income countries. Less than 30% of these countries have introduced HPV vaccination compared to more than 85% of high-income countries. Only 20% of women in low- and middle-income countries have ever been screened compared to 60% in high-income countries. Girls-only vaccination (age 9 to 13 years) is predicted to reduce cervical cancer incidence from 19.8 to 2.1 cases per 100,000 women-years in low- and middle-income countries. Introducing twice lifetime screening at age 35 years and 45 years would further reduce the incidence to 0.7 cases per 100,000 women years and result in elimination of the disease within 11–13 years [Citation18]. Still, participation in national vaccination programs is an issue, with a coverage of 25% in France, up to 70% in The Netherlands, and more than 80% in the UK, Portugal, and Australia [Citation19].
There is no doubt that vaccination is effective in reducing the incidence of cervical cancer. However, as vaccination programs do not cover all relevant HPV types and not all women at risk for cervical cancer are vaccinated, screening will remain a medical need for at least a couple of decades. As vaccinated women entered the age of the screening population, it has become apparent that screening by cytology results in a higher percentage of false positives. These are women with mild lesions that are at low risk for developing cervical cancer. This argues in favor of further implementing hrHPV tests as the primary screen followed by a triage test. While it is expected that the incidence of cervical cancer will decrease with the age of vaccinated women and a higher degree of vaccination, the need for screening remains.
2. Molecular marker assays
2.1. Sample types
Before discussing the different options of molecular markers for triage and the respective assay technologies, it is important to outline the different types of samples that may be used. Conventional scrapes, which are performed at the outside of the cervix in search of cancerous cells (PAP test) in the transformation zone, are still in widespread use [Citation20]. LBC [Citation21] as well as the SP tests [Citation22], developed in the 1990s, and the TP-PAP test in combination with the ThinPrep2000 processor have advantages compared to the original PAP test due to the removal of contaminants like blood, mucus, and cellular debris [Citation21]. As samples are well protected against DNA, RNA, and protein degradation by suspending them immediately in a methanol-based fixation solution, they can be used for (i) PAP/LBC cytology, (ii) hrHPV testing, (iii) HPV16/18 genotyping, and (iv) p16/Ki-67 dual-staining [Citation23,Citation24].
In case a woman is referred to a gynecologist, a biopsy is taken from the abnormal areas outside or inside of the cervix for microscopic examination. Simultaneously, as a routine procedure in The Netherlands, blood is withdrawn at the following time points: during the first doctor’s visit, prior to an operation, at other treatment moments and during therapy follow-up. If examination indicates an advanced CIN stage, the concentration of SCCA in serum or plasma may be used to follow the development of the disease.
Detecting hrHPV in body fluids opens a wider range of possibilities for cervical cancer screening due to easier self-sampling [Citation25,Citation26]. Urine, CVF, and fractions of blood (serum or plasma) are more accessible compared to tissue or cytological samples. CVF is a body fluid that is used for routine screening (e.g. for hrHPV detection) [Citation27,Citation28] as well as for research purposes [Citation29,Citation30]. To find reliable molecular markers in these samples would be highly beneficial provided sufficient sensitivity and specificity can be reached. Translation of molecular markers originally discovered in tissue or cells to blood or other easily accessible body fluids for assay development is thus an attractive option, notably for developing PoC assays. However, concentrations of biomarkers are expected to be much lower in urine, CVF, or blood (plasma or serum) compared to tissue or cytology samples, which makes their detection more challenging, especially for proteins. This is less problematic for epigenetic and other nucleic acid-based markers, due to the possibility of PCR amplification.
2.2. Assays
Cervical cancer screening based on conventional PAP cytology or by LBC has significantly improved early detection and, as a consequence, the chances of preventing cervical cancer. However, their implementation requires trained laboratory personnel and experienced pathologists for data interpretation with considerable inter-observer variability. Recent developments in computer-guided image analysis using artificial intelligence may alleviate this problem with the goal to perform such assays reliably even when trained personnel is not available [Citation27,Citation28]. In the following, we will give an overview of techniques that are used for the detection of molecular markers starting from the already widely accepted PCR-based hrHPV assays and assays to assess changes in DNA methylation patterns to discovery, validation, and screening techniques for protein markers.
Box 1. Analytical techniques for molecular marker assays (PCR) based methods.
2.2.1. hrHPV DNA and mRNA assays
Detection of an hrHPV infection may be based on different viral targets (whole viral DNA, E6/E7 mRNA, E6/E7 DNA, etc.). A large number of tests has been developed (ca. 250 tests and more than 450 variants thereof) [Citation29], but only a limited number has been validated [Citation30] and approved for routine screening [Citation3]. These tests may be divided according to the target into (i) viral DNA- and (ii) E6/E7 mRNA-based tests [Citation31].
Testing for hrHPV DNA is based on hybrid capture assays, conventional and real-time PCR, reverse hybridization, PCR-microarrays, and assays based on in situ hybridization. These tests differ by the set of HPV types that are detected with i) tests targeting clinically relevant hrHPV types without distinguishing between them, ii) type- or group-specific tests that detect particular types and/or subsets of hrHPV, iii) hrHPV tests with partial genotyping that distinguish between the most dangerous hrHPV types (HPV16/18 and 45) and iv) full genotyping allowing to distinguish between all HPV types. Tests that are specific for the E6/E7 oncogenes comprise mRNA-based assays based on transcription-mediated amplification or nucleic-acid sequence-based amplification as well as protein-based lateral flow immunoassays [Citation32].
hrHPV tests differ in the level of automation, throughput [Citation33], the number of identified virus types, analytical sensitivity [Citation34], the requirement of equipment and skilled personnel and the cost per analysis. Despite some of these differences, all validated DNA-, as well as mRNA-based hrHPV tests provide significant cost benefits compared to PAP or LBC tests [Citation6]. While hrHPV tests are in most cases only qualitative or semi-quantitative, estimating the viral load is a parameter that might be related to the stage of the disease and its progression as well as to the persistence of viral infections [Citation35].
2.2.2. DNA methylation assays
DNA hypermethylation occurs when a methyl group is transferred to the 5-position of cytosine resulting in gene silencing. While there is ongoing research in DNA hypermethylation and hydroxymethylation to act as cervical cancer biomarkers, only a few tests have thus far been commercialized. The most widely used test (Qiasure) uses hypermethylation of the promoter region of a human tumor suppressor gene FAM19A4 measured by QSMP and a micro-RNA (mir124–2) [Citation23,Citation36]. The GynTect QSMP assay measures the level of methylation of six genes ASTN1, DLX1, ITGA4, RXFP3, SOX17, and ZNF671 [Citation37]. There are several other epigenetic marker tests under development, such as the PreCursor-M test targeting CADM1 and MAL (later supplemented with miR124-2), the Confidence Marker M test targeting POU4F3, or the Cervi-M test targeting PAX1. The further adoption of epigenetic markers requires proper selection of detection techniques, such as NGS, QSMP, methylation microarrays, or possibly LC-MS/MS [Citation38,Citation39] that provide high-throughput at a reduced cost per sample.
2.2.3. Protein assays
While there is a rationale to anticipate that there is a strong connection between viral infection, cell transformation and protein alterations, the contribution of proteomics to cervical cancer diagnostics and screening has been limited [Citation40]. The only proteins that have been used for triage are p16 and Ki-67, which are linked to the risk of progression of CIN2+ lesions and are measured by so-called dual-stain IHC. Although several other promising protein biomarkers are currently under investigation, none of them has reached the necessary sensitivity and specificity to add significant value to current screening programs. CEA, SCCA, and CA19-9 measured by ELISA in serum are frequently used as markers in oncology and particularly in cervical cancer research, but none of them is specific enough to detect the disease at an early stage, let alone to allow triage of hrHPV positive women [Citation36]. The wide applicability of proteomics techniques to biofluids (e.g. serum, plasma, urine) collected non-invasively as well as to samples from hrHPV and cytology screening together with the capability to detect post-translational modifications and degradation products, the possibility of multiplexing and the ability to develop PoC devices for rapid, cost-effective testing in low- to middle-income countries, emphasize the need to discover and validate better cervical cancer protein markers.
Protein bioanalytical techniques may be divided into three main categories: (i) immunoassays, (ii) mass-spectrometry or LC-MS assays, and (iii) assays based on (immuno)enrichment combined with MS or LC-MS detection (LBA-MS assays). All these techniques are undergoing rapid developments in terms of improved analytical sensitivity, proteome depth, automation, increased throughput, and reduction of cost per analysis to support biomarker discovery and validation studies and ultimately facilitate implementation in screening programs.
2.2.3.1. Immunoassays
Immunoassays are based on the interaction between an analyte (antigen) and an antibody or another affinity ligand. Many variants of immunoassays have been developed and used in protein research as well as routine screening, including but not limited to IHC, ELISA, LFIA, and immunoblots.
The most relevant clinical use of immunoassays in cervical cancer diagnostics is based on IHC, where antibodies are used to visualize specific proteins on cells or tissues followed by their microscopic analysis. IHC staining of p16, an inhibitor of cyclin-dependent kinases, and Ki-67, a cell proliferation marker, showed potential for improving diagnostic accuracy [Citation41,Citation42]. The p16/Ki-67 dual staining technology is more sensitive than cytology in detecting CIN2+ in hrHPV-positive women and can be used for triage after a positive hrHPV test to guide the decision on how to follow-up, for example, by colposcopy [Citation43,Citation44]. The mapping of other proteins related to cell cycle control or DNA repair, such as TOP2A and MCM2, has shown utility for detecting high-grade disease and confirmed the relationship between MCM2 overexpression and CIN stage [Citation45]. Despite the high level of automation that is possible, the technique requires an expert to evaluate the results in analogy to cytology. The advent of artificial intelligence systems and the growing database of images may overcome this limitation in the foreseeable future.
ELISA, a quantitative analytical technique that measures antigen-antibody interactions via a colorimetric, fluorescent, or chemiluminescent signal [Citation46], is often used for the quantification of protein markers in body fluids. The quantification of SCCA and CA-19-9 in serum are examples of protein biomarkers that are used in cervical cancer, albeit not for screening or triage. The advantage of ELISA is that data interpretation does not require skilled personnel, that automation of all steps is possible and that this technique is widely implemented in clinical chemistry laboratories around the world. However, the use of so-called autoanalyzers often requires a significant amount of sample. The single molecular array technique showed increased sensitivity in comparison with classical ELISA methods for SCCA detection and quantification in blood and can also be fully automated [Citation47]. Additionally, the spatial localization allows for the detection of a single immunocomplex on each bead, and coupling with different fluorophores allows for multiplexing of up to six analytes [Citation48]. The transition from laboratory research to the end-user resulted in the development of PoC tests. Many of them are based on optical or microscopic data and image evaluation [Citation49]. Molecular PoC tests are based on lateral immunochromatographic methods such as LFIA. The commercially available OncoE6 Test, designed to detect oncogenic HPV 16/18 types via the E6 oncoprotein [Citation32,Citation50], showed lower specificity and sensitivity in comparison with HPV PCR testing especially for the diagnosis of CIN2+ [Citation51]. However, similar tests could be useful as a triage method in screening programs, particularly in low- to middle-income countries. An additional benefit would come from the ability to differentiate HPV types in one test [Citation52,Citation53], which is currently under development [Citation54]. In view of a potential link between an increase in the level of oncoproteins and disease progression, a number of LFIA-based approaches that also provide quantitative results have been developed. A SERS-based analysis of two specific cervical cancer biomarkers comprising SCCA and CA125 in serum shows potential for multiplexed quantification [Citation55]. An LFIA with magnetic focusing for improving the sensitivity of detection was proposed to measure valosin-containing protein in fresh frozen tissue, but has not yet been tested on relevant sample cohorts [Citation56].
Western blots (immunoblots) that allow separating proteins electrophoretically prior to detection are irreplaceable in cervical cancer research but have low clinical utility [Citation57] due to difficulties with large-scale automation. Despite recent developments, where capillary electrophoresis is used to separate and chemiluminescence to detect and quantify proteins in an automated setup, it is still considered a research tool [Citation58].
2.2.3.2. Mass-spectrometry based assays
The fast development of liquid chromatography and mass-spectrometry instruments in parallel with software and data processing pipelines during recent years brings this technology closer to clinical applications. While in most cases, MS-based techniques are used during the discovery and early validation phases of biomarkers, there is a potential for technology evolution toward routine clinical applications and population screening. Since the technology is complex, costly and requires highly skilled personnel, it is unlikely to have a direct impact on the global incidence of cervical cancer, with most cases occurring in low- to middle-income countries. The way forward is likely that more specific and sensitive biomarkers may be discovered by MS-based approaches and then transferred to low-cost immunoassays for widespread implementation. It is in this perspective that we discuss several MS-based technologies.
The direct protein profiling of biofluids, cytological samples and tissue biopsies is the most straightforward MS-based approach. While technically suitable for measuring many samples in screening procedures, it suffers from limited analytical sensitivity and selectivity and is thus not suitable for the discovery of new biomarkers. The main drawback of direct MS detection without selective sample preparation strategies is its limitation to the detection of high- to medium-abundance proteins. For example, for body fluids like blood plasma sensitivity does not go below the µg/mL concentration range, which is insufficient to measure biomarkers that are mechanistically related to cancer development and notably to the risk of progression of a CIN2+ lesion. Combining MALDI-MS analysis with highly selective sample preparation strategies, such as enrichment of proteins with antibodies, overcomes some of these limitations but also obviates one of its major advantages, the detection of proteins without having to make a selection of which protein to follow beforehand. MALDI-MS has been used in cancer biomarker discovery [Citation59], the classification of cases versus controls by serum protein fingerprinting [Citation60], the visualization of spatial protein expression profiles in cytological samples by MS imaging [Citation61], and even for simultaneous genotyping of 14 hrHPV types. While conceptually interesting, MALDI-MS and the analogous SELDI-MS technique [Citation62] have been largely replaced by the more powerful LC-MS approach, which combines the separation of protein-derived peptides with online electrospray ionization mass spectrometry. This is doubtless the currently most widely used discovery approach in proteomics-based biomarker research. LC-MS is also suitable for biomarker validation when used in the targeted mode. New developments in the field of MALDI-MS imaging [Citation61] and its combination with protein digestion and LC-MS for protein identification [Citation63] or fast targeted protein isolation have shown potential for the analysis of tissue biopsies or cytology samples. However, current approaches are too slow for population screening not the least because they generate very large data sets per sample that need to be processed and analyzed. These approaches may, however, be useful in biomarker validation, as they provide spatially resolved images of proteins as well as of many other molecules. The reader is referred to a recent review for further details [Citation64].
Another approach that may become interesting in the future, not only for population screening but also for guiding surgery, is REIMS. REIMS was developed for the analysis of aerosols generated during electrosurgery and combined with tandem high-resolution MS was tested on histology samples showing excellent accuracy for discriminating normal, CIN and cancer tissues [Citation65]. A similar approach with LA-REIMS led to the development of a high-throughput screening procedure in cell pellets from LBC samples to discriminate hrHPV positive from negative samples and normal from CIN2+ [Citation66]. Discrimination was most likely based on differences in lipid patterns and analysis used a neural network-based approach. LDI-MS from a plasmonic chip was used for metabolite analysis of serum from patients with cervical cancer and healthy controls [Citation67], and exhaled breath analysis with proton transfer reaction mass spectrometry has also been used as well for this purpose [Citation68]. In both cases, the technology allows upscaling and fast analysis but has not been tested in the context of population screening and the triage of hrHPV positive women.
To overcome the limited depth of direct MS methods, efficient isolation, separation, and fractionation techniques are required in combination with advanced MS detection. UHPLC coupled with high-resolution qTOF and Orbitrap MS or medium-resolution triple quadrupole (QqQ) MS proved to be a powerful tool for protein and metabolite analysis in cervical cancer research [Citation69–72]. Electrophoretic separation techniques like 2D-GE [Citation73,Citation74], SDS-PAGE, or IPG-IEF are rarely used as primary separation techniques, as they are labor-intensive and difficult to automate. However, electrophoretic methods are often utilized as orthogonal methods to validate biomarkers that resulted from LC-MS-based discovery studies. While it goes beyond the scope of this review to enumerate the many different versions of LC-MS in protein analysis, approaches for protein biomarker discovery research comprise label-free quantification [Citation75,Citation76] as well as a variety of relative quantification strategies based on chemical stable isotope labeling, for example, based on iTRAQ [Citation76–78] or TMT [Citation79]. However, such approaches are costly in terms of time, the price of the reagents, as well as the rather complex (data) analysis, which requires considerable experience and highly skilled specialists. While being powerful discovery tools, these methods are not suited for screening.
In contrast to untargeted LC-MS for biomarker discovery, targeted quantification of defined proteins with SRM/MRM [Citation80] or parallel reaction monitoring PRM [Citation78] has reached a level of throughput and multiplexing [Citation46] that makes it competitive with antibody-based methods. Unfortunately, the wide dynamic concentration range of proteins in biological samples and notably in body fluids does not always allow to use targeted LC-MS without extensive sample preparation. Recent work on affinity enrichment coupled to mass spectrometry and more generic sample preparation strategies for biomarker analysis in routine clinical chemistry laboratories opens the way toward a more widespread use of LC-MS in these areas [Citation81–84].
3. Epigenetic and protein markers
3.1. Epigenetic markers
Epigenetics is the heritable change of gene expression without altering the DNA sequence [Citation85] (see Box 2).
Box 2. Epigenetics and cervical cancer [Citation86,Citation100].
Epigenetic regulation includes DNA methylation, histone modifications, and miRNA expression [Citation87]. Unlike genetic changes, epigenetic changes are reversible, making them an attractive candidate for early detection, diagnosis, and prognosis of cervical cancer [Citation88,Citation89]. Since several epigenetic changes occur prior to histopathological changes, they may serve as biomarkers for early diagnosis and risk assessment [Citation90]. Epigenetic alterations are part of the carcinogenic process [Citation89] and have been extensively studied in relation to cervical cancer [Citation91]. Early and advanced transforming CIN lesions can be distinguished based on epigenetic changes [Citation7]. Epigenetic changes in host cell DNA are needed for progression from CIN to cervical cancer, and part of these alterations are induced by the viral oncogenes E6 and E7, including the methylation and thus silencing of tumor suppressor genes [Citation92].
Infection of epithelial cells with hrHPV leads to several epigenetic modifications in regulatory regions of various host genes as well as to the integration of viral DNA into the host genome [Citation7]. A range of such modifications has been mapped and related to persistent infections with hrHPV strains. Persistent infections, which are those that are not resolved by the immune system of the host, can lead to CIN stages 1, 2, or 3. While stage 1 is generally considered benign, not requiring immediate action, CIN2 and CIN3 lesions (summarized as CIN2+) may progress to cervical cancer. This process takes many years with a number of critical events, as shown in .
Figure 3. Schematic representation of epigenetic processes following infection with hrHPV. While 80% of infections are cleared by the immune system, 20% persist leading to CIN1/2 lesions. Most of these persistent infections will be resolved by the immune system with clearance of the infection. Deregulation of E6 and E7 expression is a critical event that changes a productive CIN1/2 lesion (one that produces viral particles without progressing) into a transforming CIN2+ lesion. Transforming CIN2+ lesions may carry an elevated risk of progressing to cancer during the ensuing screening interval (high short-term risk) or not (low short-term risk). The risk of progression is correlated with changes in the methylation status of both host and viral DNA. Hallmarks of a high-risk lesion are a persistent infection of more than five years, elevated copy number alterations, low expression of E4, and DNA hypermethylation, while low-risk lesions show the opposite characteristics (adapted from Kremer et al., Bjog 2021, 128(3):504–514)
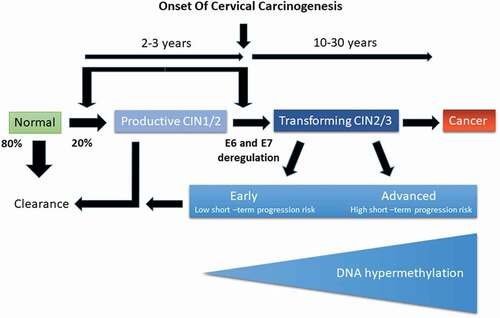
Combinations of epigenetic marker panels have been investigated for triage following a positive hrHPV test during population screening resulting in a number of commercialized assays. Some of these tests have been investigated in clinical studies (see for a selection and Table S2 for a broader overview) but have not yet entered routine use in population screening programs.
Table 1. Selected DNA methylation studies with high-grade CIN and cervical cancer as endpoints.Footnote1 All studies used QMSP as detection method
Current results indicate that the QIAsure Methylation test, targeting FAM19A4/miR124-2, outperforms cytology in assessing whether CIN2+ lesions might progress to cancer within the next screening interval (short-term risk) or not. The test has also been evaluated with respect to the risk of false-negative results (women that later developed CIN3+ or cervical cancer after a negative methylation marker test). While further work is needed, methylation marker assays show considerable promise for triaging women with a positive hrHPV test. Such tests may eventually replace cytology as triage and possibly even be used as primary tests in population screening. However, more extensive longitudinal studies are needed on larger study populations before definitive conclusions can be drawn [Citation7,Citation85,Citation100].
It is to be expected that methylation marker assays will complement current screening procedures and that commercially available assays will be further developed and automated. This will allow performing thousands of assays per week in a regular clinical diagnostic lab. The main challenge is ultimately to increase acceptance of the test results among the various stakeholders (e.g. medical specialists, health insurances, governments).
3.2. Protein biomarkers
Proteins have an extensive history as biomarkers for disease diagnosis and prognosis. Many protein biomarkers are used experimentally in cervical cancer and related CIN stages, but none has reached the status of being implemented in routine population screening. This is likely due to the fact that most discovery studies do not address the most pressing need of cervical cancer screening, namely the discrimination of CIN2+ lesions carrying a low short-term risk of progression from those carrying a high short-term risk in view of developing a more specific triage test following hrHPV testing. To achieve this, protein biomarker discovery studies must go beyond measuring the level of proteins to assessing the state of their post-translational modifications, which is often more closely linked to proliferation and deregulation of the cell cycle. One can principally discriminate protein biomarkers for cell-based (tissue biopsy or cytological) assays and biomarkers that are measured in biofluids (e.g. blood, serum, plasma, CVF and urine). The most widely used and most promising protein biomarkers are those related to cellular proliferation and/or cell cycle control. These biomarkers are generally analyzed by IHC in biopsies or cytological specimens. We will discuss these biomarkers in greater detail below.
Protein biomarkers that are measured in biofluids like serum may be used to follow therapy and to assess whether there is complete remission or recurrence of the disease, for example, following surgery and/or radiotherapy. SCCA and CA125 are two examples of such markers. SCCA is primarily a tumor marker of the uterine. It is present in normal epithelial tissue and several benign lesions of squamous cells, including esophagus, head, neck, lung, or skin diseases [Citation73,Citation74]. These biomarkers are not specific for cervical cancer but may be useful in the context of therapy when the primary disease diagnosis has already been established without a doubt.
Comparison of published data on protein biomarkers is sometimes difficult, since the number of patients tested can vary widely, and the methodology of analysis may be different. As for epigenetic markers, there is a lack of studies with long-term follow-up to link outcome (e.g. development of CIN2+ lesions that progressed to cancer) to protein biomarker levels, with a few notable exceptions [Citation101].
Box 3. Protein markers.
3.2.1. Proteins related to cell division and DNA replication
As one of the major challenges in cervical cancer screening programs is to discriminate productive infections with hrHPV (those that produce viral particles but carry a low risk of transformation) from transforming infections (those that carry a high risk of transformation leading to cervical cancer), it is amenable that proteins that are implicated in regulating cell division and DNA replication may be candidates to make this discrimination. p16ink4a (referred to as p16 in this article) is a tumor suppressor and cell cycle regulatory protein that inhibits CDK 4 and 6, thereby suppressing cellular proliferation. Integration of viral genes coding for the oncoproteins E6 and E7 into the host genome leads to enhanced expression and cellular accumulation of p16 in epithelial cells of CIN2+ lesions undergoing transformation [Citation102]. Ki-67 is a marker of proliferating cells routinely used in diagnostic settings. It is present during all phases of the cell cycle and is often increased in cervical lesions [Citation103]. IHC staining for p16 and Ki-67 is used to discriminate lesions with a high short-term risk of transformation from those with a low risk [Citation101].
Güzel et al. [Citation78] showed that the combination of LCM and proteomics using high-resolution mass spectrometry in tissue from squamous cell cervical cancer patients allows to determine up-and down-regulated proteins in tumor tissue versus healthy epithelium and stroma. Particularly members of the MCM family showed strong upregulation confirming earlier observations that the MCM protein family is involved in progression. MCM proteins unwind duplex DNA and power fork progression during DNA replication. Regulation of MCM proteins and their phosphorylation is critical for tumor progression. MCM proteins and associated proteins may serve as targets for anti-cancer therapy and as molecular markers for diagnosis, notably to discriminate low-risk from high-risk CIN2+ lesions. Stevenson and coworkers [Citation104] showed that MCM and E4 are promising biomarkers to define the CIN stage in cervical tissue samples. Notably, MCM2, a member of this protein family, was shown to be elevated in lesions with increased transformative potential [Citation105]. MCM2 is a component of the pre-DNA-replication complex that is overexpressed in squamous cell carcinoma, and overexpression of MCM2 is characteristic of immature dysplastic cells in the cervix. Filho et al. [Citation105] found a linear correlation between HPV-infected cells and increased expression of MCM2. MCM3 and MCM7 appear to be better proliferative and diagnostic markers for various virus-induced neoplastic malignancies than the widely used Ki-67 [Citation106,Citation107]. TOP2A is another replication marker that has been reported to be overexpressed during the progression of CIN2+ lesions to cervical cancer [Citation108]. A combination of antibodies against MCM2 and TOP2A has been used to discriminate CIN lesions by IHC in cytological specimen [Citation109] (see for an overview of selected studies).
Table 2. Selected studies based on proteins related to cell division and DNA replication. All studies used IHC as detection method. The p16/Ki-67 studies were performed with the CINTec® PLUS assay (Roche)
Footnote10Footnote8In addition to measuring the level of different MCM proteins, it is also of interest to assess their phosphorylation state. Protein kinases and phosphatases play a critical role in the initiation and regulation of DNA replication [Citation112]. MCM3 is reported to be phosphorylated by DAPK at Ser-160, and SIK1-dependent MCM2 phosphorylation, mediated by Sid5, is required for MCM helicase activity. In HELA cells, a cell line originally derived from a cervical cancer patient, the EBV-PK phosphorylates at least two sites on MCM4 (Thr-19 and Thr-110), resulting in loss of enzymatic activity of the MCM4/6/7 sub-assembly, which leads to cell growth arrest. While most of the pThr-110 MCM4 detaches from chromatin, about half of the pThr-19 MCM4 remains bound [Citation113]. EBV-PK might also phosphorylate MCM6 and additional sites on MCM4 to block DNA replication in EBV-infected cells [Citation114]. Phosphorylation patterns of members of the MCM protein family at specific residues may thus serve as more specific biomarkers for transformation as well as markers to guide anti-proliferative therapy with kinase inhibitors. Targeted analysis of these phosphorylation sites by LC-MS/MS is a promising strategy to strengthen this hypothesis further.
3.2.2. Other protein markers
Next to these widely studied protein biomarkers, others have been advanced as being of potential interest. For example, Komdeur et al. showed that CD103 is a marker that may be used for the prognosis of cervical cancer based on the beneficial effect of CD8+ T-cell infiltration [Citation9]. The ratio of serum Angiopoietin-1 to Angiopoietin-2 has been proposed as a diagnostic and prognostic biomarker for cervical cancer [Citation115]. A panel of five proteins (MCM2, MCM4, MCM5, PCNA, RNASEH2A) [Citation116], as well as GPER, have been proposed as prognostic markers for cervical cancer [Citation117], and the same is true for TKT, FGA, and APOA1 [Citation118]. Most of these studies must be considered preliminary as they were conducted on small numbers of subjects with study designs that are not directly relevant for the current needs of cervical cancer screening. Detailing some of the biological functions of the proposed biomarkers reveals that many of them have no clear mechanistic link to cervical cancer. TKT is an enzyme in the nonoxidative arm of the pentose phosphate pathway, which is part of central carbon metabolism, which is essential for cell growth. FGA is associated with the pathogenesis of endometriosis as well as being involved in cell growth and many other functions, notably related to blood coagulation. APOA1 is a major component of HDL, and the ratio of HDL-C to APOA1 may be a risk factor for mortality most likely due to mechanisms that are not directly related to cervical cancer. APOA1 and mTOR have been advanced as potential prognostic serum biomarkers for cervical cancer, but further, more detailed studies are needed to substantiate these findings [Citation119]
It has been a longstanding goal of cervical cancer research to find blood-borne biomarkers with a good predictive value for the risk of developing cervical cancer, as blood is more easily obtained for screening and since blood-based assays may be more easily implemented in PoC devices. For example, preliminary data from serum proteomics have shown that a panel of six proteins (alpha-1-acid glycoprotein, alpha-1-antitrypsin, serotransferrin, haptoglobin, alpha-2-HS-glycoprotein, and vitamin D-binding protein) may serve to discriminate patients with CIN 2+ from healthy controls [Citation72,Citation77]. However, as these proteins are rather generic serum proteins often related to the so-called acute-phase response, there is doubt as to their specificity for CIN versus other underlying pathologies.
The cervicovaginal microbiome may be regarded as another source for biomarkers that reflects the host response to an hrHPV infection. The cervicovaginal microbiome is a dynamic network of microorganisms that can modulate the host immune response and affect susceptibility to viral infection, persistence, and the development of CIN [Citation120–122]. Neoplastic transformation of the cervix may be reflected in the CVF as revealed by label-free proteome profiling. Research by Starodubtseva et al. [Citation123] compared the CVF proteome at different stages of neoplastic transformation of the cervix (LSIL, HSIL, and cancer) and identified six potential biomarkers, ACTN4, VTN, ANXA1, CAP1, ANXA2, and MUC5B [Citation124.] Again, considerably more work is needed to substantiate these findings in view of turning them into useful starting points for developing an improved triage test in cervical cancer population screening [Citation125–127].
In another approach, Cardoso et al. [Citation128.] combined genetic and proteomic data to select putative biomarkers involved in metastasis, cell cycle regulation and tumor suppression. Van Baars et al. [Citation129.] studied a biomarker of HPV life-cycle completion (panHPVE40) and combined it with MCM cell-cycle markers and p16. Expression of E4 can be considered as a marker for the onset of the productive stage in the viral cell cycle and a low expression of E4 indicates an elevated risk of progression in a CIN2+ lesion. Griffin et al. [Citation130.] correlated the expression of E6 and E7 with p16 and MCM, showing that the combination of p16 and E4 might be used to divide CIN2 groups according to the extent of cell cycle deregulation and thus the risk of transformation.
Most of these studies must be considered preliminary at the moment excepting those investigating the combination of p16 and Ki-67. While protein biomarkers undoubtedly hold promise to improve cervical cancer screening due to the fact that progression to cancer is intimately linked to changes at the proteome level, it is currently too early to say whether protein markers will become a viable option for a better triage test. Widening the focus to include post-translational modifications and combining epigenetic with protein-based tests may be ways forward to arrive at better specificity without sacrificing sensitivity.
4. Cost-effectiveness analysis
No matter which biomarker and which type of assay platform is used, it is critical to consider cost-effectiveness prior to implementation. Assay formats and platforms may well be different depending on the cost structure of the health care system in a given country and the available infrastructure.
The primary measure of cost-effectiveness is the incremental cost-effectiveness ratio (ICER), which is the ratio of the additional costs to the additional health benefits of an intervention.
Box 4. ICER and QALY.
Taking the Netherlands as an example, the current screening program by hrHPV testing is estimated to prevent 361 cancer diagnoses, 74 cervical cancer deaths with 19,838 referrals to a gynecologist per 100,000 women [Citation131.] Similar calculations in the USA normalized to 100,000 women gave 94 cancer diagnoses, 28 deaths due to cervical cancer and 16,204 referrals [Citation6]. Most of the referrals were CIN0/1 indicating the rather low positive predictive value of hrHPV testing for CIN2 + . Screening strategies differ per country. presents the cost-effectiveness thresholds, the associated optimal strategies identified in cost-effectiveness analyses in terms of screening intervals and the ICER of those optimal HPV-based screening strategies (according to the methodology used in the specific studies). The table shows that HPV screening is cost-effective in these countries, albeit to different degrees.
Table 3. Selected cost-effectiveness analyses that calculate the cost per QALY gained of primary HPV screening strategies in different countries
Jansen and coworkers [Citation132.] compared the cost-effectiveness of the original Dutch screening program using cytology with the current hrHPV screening program concluding that the hrHPV-based program was more cost-effective than the cytology program. Compared to no screening, the cytology program costs € 22,678 per QALY gained, and the hrHPV-based program costs € 12,225 per QALY gained. Per life-year gained the cytology program costs € 15,247, and the hrHPV- based program € 10,890.
As mentioned earlier, the number of unnecessary referrals to a gynecologist increased after implementing the hrHPV test in population screening. Kaljouw et al. [Citation133.] used the MISCAN-Cervix model to simulate an unvaccinated cohort of ten million 30-year-old Dutch women. Different triage strategies (cytology, HPV testing and/or genotyping) had varying effects on the number of unnecessary referrals, ranging from −72% to +35%. The largest reduction in unnecessary referrals without a substantial increase in mortality or incidence was achieved by adding genotyping for HPV16 (−45%) or HPV16/18 (−40%) to the screening algorithm. In this case, women with a lower risk HPV type (i.e. HPV types not covered by current vaccines) and a diagnosis of LSIL were not directly referred to a gynecologist. The time to repeat the test was set at 12 months. To implement novel biomarker tests into routine population screening, it is essential to calculate their cost-effectiveness based on a country’s healthcare system and economic performance.
5. Expert opinion
Cervical cancer screening has greatly reduced the incidence of the disease as well as mortality. The introduction of population screening using the PAP test can be considered one of the great successes of cancer prevention due to public health measures. As our understanding of the etiology of cervical cancer and its dependency on infection with hrHPV has expanded since the original discovery by Zur Hausen et al. [Citation134,Citation135], and due to the development of powerful molecular biology techniques, primary population screening has switched to hrHPV testing, which is more cost-effective while providing similar gains in QALYs. The high sensitivity but low specificity of hrHPV testing has, however, led to many unnecessary referrals to a gynecologist, resulting in anxiety and additional cost for the health care system. This emphasized the necessity for a triage test with higher specificity. Currently, triage is done by cytology (either PAP or LBC), although it still suffers from low specificity, notably with respect to discriminating CIN2+ lesions that carry a low risk of progression from those that carry an elevated risk. This has led to the development of a number of molecular tests, most of them based on assessing changes in DNA methylation patterns at critical regions of host and viral DNA. While some of these commercially available assays show promising results as triage tests, more extensive and notably long-term studies are needed to assess their value in population screening. In this respect, it is not only necessary to reduce the number of unnecessary referrals but also to avoid false negatives, meaning women that test negative but later develop CIN3+ lesions or invasive cervical cancer. The same is true for protein-based tests. While it is fair to say that protein-based tests lack behind epigenetic marker tests in terms of validation in population screening, some of them hold promise to improve triage. For example, proteins that are involved in cell cycle control or that are linked to cell proliferation have been successfully used on cytological samples analyzed by IHC. However, most of these studies were rather limited in number of subjects and did not have the necessary long-term follow-up. Protein-based assays might reach higher levels of specificity and sensitivity for triage if they were extended to cover critical post-translational modifications. This is a subject for future research that will hopefully lead to more specific and more sensitive triage tests.
Making such tests available to low- and middle-income countries is critical in order to reduce the global incidence of cervical cancer and to reduce mortality, which is still at about 250.000 deaths per year. Protein-based tests have historically been better adapted for this task, as they can be performed with technologies that do not require expensive equipment or highly skilled personnel. Unfortunately, there is currently no suitable protein biomarker that has the characteristics to be implemented in such a ‘field test.’ This should be motivation and inspiration for researchers and funding bodies to join hands to meet this challenge.
We hope to have given sufficient background information for more researchers to enter this field to further reduce the incidence of cervical cancer worldwide.
Article highlights
Epigenetic marker panels including miRNAs show promise in discriminating CIN2+ lesions that carry an elevated risk of progressing toward cervical cancer from those that do not. The availability of commercial kits facilitates their implementation in screening programs and clinical use.
There are a few promising protein-based tests that allow such a discrimination but they are less advanced compared to epigenetic markers in terms of validation in large population screens and notably in long-term studies with a defined endpoint.
Combined tests based on changes in epigenetic markers, miRNAs, and proteins might be the best choice to reduce the percentage of false positives (low-risk CIN2+) without increasing the percentage of false-negative tests (cervical cancer cases that remain undetected). Such integrated tests present technological challenges that must be met in order to allow their use in population-wide screening.
A real impact on mortality due to cervical cancer can only be achieved if tests are made available to low- and middle-income countries. This rules costly procedures requiring expensive equipment and/or highly skilled personnel out and argues in favor of simple and robust test systems based on lateral flow immunoassays or integrated microfluidic systems.
Health economic considerations, which are rather different for high- versus low- to middle-income countries, set the requirements for screening assays, notably in terms of cost.
Glossary
ASCUS | = | atypical squamous cells of undetermined significance |
ANKRD18CP | = | ankyrin repeat domain 18C |
APOA1 | = | apolipo protein A1 |
ASCL1 | = | achaete-scute homolog 1 |
ASTN | = | astratactin |
AUC | = | area under the curve |
5mC | = | methylation of cytosine at position 5 |
CA | = | cancer antigen |
CADM1 | = | cell adhesion molecule |
CAP1 | = | cyclase associated actin cytoskeleton regulatory protein 1 |
C13ORF18 | = | polyclonal antibody |
CC | = | cervical cancer |
CD | = | circular dichroism |
CDH | = | cutaneous delayed hypersensitivity |
CDH16 | = | cadherin |
CDK | = | cyclin-dependent kinase |
CEA | = | carcinoembryonic antigen |
Cervi M | = | molecular DNA-based diagnostic test |
CIN | = | cervical intraepithelial neoplasm |
CVF | = | cervico vaginal fluid |
2D-GE | = | two dimensional gel electrophoresis |
DAPK | = | death-associated protein kinase |
DLX | = | distal-less homeobox |
DNA | = | deoxyribonucleic acid |
E | = | early gene coding region |
EBV | = | Epstein-Barr Virus |
EBV-PK | = | Epstein-Barr virus-encoded protein kinase |
ELISA | = | enzyme-linked immunosorbent assay |
EPB41L3 | = | erythrocyte membrane protein band 4 |
FAM | = | family with sequence familiarity |
FKBP | = | prolyl isomerate |
FGA | = | fibrinogen alpha chain |
GATA4 | = | zinc-finger proteins, transcriptional activator of cyclin D2 and CDK4 |
GDP | = | gross domestic product |
GFRA | = | glial cell-line-derived factor receotor |
GHSR | = | growth hormone secretagogue receptor |
GP-EIA | = | GP5+/6 + PCR Enzyme ImmunoAssay |
GPER | = | G-protein-coupled estrogen receptor |
HDL | = | high-density lipoprotein |
HDLC | = | high level data; ink control |
HELA | = | human cervical cancer cells |
HPV | = | human pappiloma virus |
hrHPV | = | high risk human pappiloma virus |
HSIL | = | high grade squamous intraepithelial lesions |
ICER | = | incremental cost-effectiveness ratio |
IgG | = | immunoglobuline G |
IHC | = | immunohistochemistry |
IPG-IEF | = | immunobiline dry strip gels-isoelectric focusing |
ITGA | = | integrin subunit alpha |
iTRAQ | = | isobaric tags for relative and absolute quantitation |
JAM | = | junctional adhesion molecule |
KCNIP | = | potassium voltage-gated channel interacting protein |
Ki-67 | = | expression of proliferating cell association protein |
LA-REIMS | = | laser assistance rapid evaporative ionization mass spectrometry |
LBA-MS | = | ligand-binding mass spectrometry |
LBC | = | liquid-based cytology |
LCM | = | laser capture microdissection |
LC-MS | = | liquid chromatography-mass spectrometry |
LDI-MS | = | laser desorption mass spectrometry |
LFIA | = | lateral flow immunoassay assay |
LHX | = | LIM Homeobox |
LSIL | = | low grade squamous intraepithelial lesions |
LMCI | = | lower and middle-income countries |
LYG | = | life years gained |
MAL | = | myelin and lymphocyte protein |
MALDI-MS | = | matrix-assisted laser desorption/ionization mass spectrometry |
MCM | = | minichromosome maintenance protein |
miRNA | = | micro ribonucleic acid |
MISCAN | = | microsimulation screening analysis |
MUC5B | = | muscin 5B, oligomeric muscus/gel-forming |
MRM | = | multi-reaction monitoring |
MUS5B | = | mucin-5B protein |
NGS | = | next generation sequencing |
NNV | = | number needed to vaccinate |
NPV | = | negative predictive value |
panHPVE40 | = | antibody against the E4 protein of 15 HR-HPV types |
PAP | = | papanicolaou test |
P16 | = | cyclin-dependent kinase inhibitor |
PAX | = | paired box |
PCDHA | = | protocadherin alpha cluster complex locus |
PCR | = | polymerase chain reaction |
PoC | = | point of care (device) |
POU4F3 | = | POU domain class 4 eukaryotic transcription factor 3 |
PPV | = | positive predictive value |
pRb | = | retinoblastoma protein |
p 53 | = | cellular tumor suppressor protein 53 |
PRM | = | parallel reaction monitoring |
pThr | = | threonine peptide |
QALY | = | quality-adjusted life years |
QMSP | = | quantitative methylation-specific PCR |
QqQ | = | medium resolution triple quadrupole |
qTOF | = | quadrupole time of flight |
RAB3C | = | protein coding gene |
REIMS | = | rapid evaporative ionization mass spectrometry |
RIVM | = | Dutch Center for Population Studies |
RNASEH | = | ribonuclease |
RXFP | = | relaxin family peptide receptors |
GABRA2 | = | Gamma-aminobutyric acid receptor subunit alpha-2 |
SCCAg | = | squamous cell carcinoma antigen |
SDS-PAGE | = | sodium dodecyl sulfate polyacrylamide gel electrophoresis |
SELDI-MS | = | surface-enhanced laser desorption ionization |
SEPT9 | = | Septin 9 |
Ser | = | recombinant CTLA4 protein |
SERS | = | surface-enhanced Raman spectrometry |
SIK | = | salt-inducible kinase |
Sid5 | = | systemic RNA interference defective protein |
SIX | = | homeo domain containing DNA binding proteins |
SLC5A8 | = | solute carrier family 5 member 8 |
SLC6AS | = | creatine transporter |
SOX | = | SRY-box transcription factor |
SP | = | sure path |
SRM | = | selected reaction monitoring |
SST | = | somatostatin precursor |
ST6GALNAC5 | = | ST6-N acetylgalactosaminide alpha 2,6 sialyltransferase 2 |
TBX | = | T-box transcription factor |
TERT | = | telomerase reverse transcription |
Thr | = | thronine |
TKT | = | transketolase |
TMT | = | tandem mass tags |
mTOR | = | mechanistic target of rapamycin |
TOP2A | = | topoisomerase II alpha |
TP | = | thin preparation |
UHPLC | = | ultra high-performance liquid chromatography |
VLP | = | virus-like particles |
VTN | = | vitronectrin |
WHO | = | World Health Organization |
YLS | = | life years gained |
ZIC1 | = | zinc finger protein 1 |
ZNF | = | zinc finger protein |
ZNP | = | zinc oxide nanoparticle |
ZSCAN | = | zinc finger and SCAN domain-containing protein |
Reviewer disclosures
Peer reviewers on this manuscript have no relevant financial or other relationships to disclose.
Supplemental Material
Download PDF (203.7 KB)Supplementary material
Supplemental data for this article can be accessed here.
Additional information
Funding
Notes
1. Adapted from Lorincz, A. T., Acta Cytol 2016, 60, 501–512 with additional studies published during 2017–2020.
2. C13ORF18, JAM3, EPB41L3, TERT, ZSCAN1, ST6GALNAC5, ANKRD18CP, CDH6, GFRA1, GATA4, KCNIP4, LHX8.
3. Threshold was the same for both sample types.
4. In need of treatment.
5. ASTN1, DLX1, ITGA4, RXFP3, SOX17, ZNF671 (GynTect).
6. QiaSure.
7. FAM19A4 methylation + 3 miRNAs.
8. In comparison: FAM19A4 methylation (AUC: 0.862).
9. GynTect.
10. hrHPV testing (women >30 years): sensitivity 93.3%; specificity 93.0%.
11. atypical squamous cells of undetermined significance.
12. ProEx C (author validated immunocytochemical assay).
References
- Sung H, Ferlay J, Siegel R. L., et al. Global Cancer Statistics 2020: GLOBOCAN Estimates of Incidence and Mortality Worldwide for 36 Cancers in 185 Countries. CA: A Cancer Journal for Clinicians. 2021(71):209–249.
- Couvreur K, Naert E, De Jaeghere E, et al. Neo-adjuvant treatment of adenocarcinoma and squamous cell carcinoma of the cervix results in significantly different pathological complete response rates. BMC Cancer. 2018 Nov 12;18(1):1101.
- Chrysostomou AC, Kostrikis LG. Methodologies of primary HPV testing currently applied for cervical cancer screening. Life (Basel). 2020 Nov 19;10(11):290.
- Screening for cervical cancer invitation: RIVM: Rijksinstituut voor Volksgezondheid en Milieu. 2019. [cited 21 Jun 2021]. Available from: https://www.rivm.nl
- [cited 2021 Jun 21]. Available from: https://www.rivm.nl/documenten/uitvoeringskader-bevolkingsonderzoek-baarmoederhalskanker
- Fontham ETH, Wolf AMD, Church TR, et al. Cervical cancer screening for individuals at average risk: 2020 guideline update from the American Cancer Society. CA Cancer J Clin. 2020 Sep;70(5):321–346.
- Steenbergen RD, Snijders PJ, Heideman DA, et al. Clinical implications of (epi)genetic changes in HPV-induced cervical precancerous lesions. Nat Rev Cancer. 2014 Jun;14(6):395–405.
- Woodman CB, Collins SI, Young LS. The natural history of cervical HPV infection: unresolved issues. Nat Rev Cancer. 2007 Jan;7(1):11–22.
- Komdeur FL, Prins TM, van de Wall S, et al. CD103+ tumor-infiltrating lymphocytes are tumor-reactive intraepithelial CD8+ T cells associated with prognostic benefit and therapy response in cervical cancer. Oncoimmunology. 2017;6(9):e1338230.
- Chen CC, Huang LW, Bai CH, et al. Predictive value of p16/Ki-67 immunocytochemistry for triage of women with abnormal Papanicolaou test in cervical cancer screening: a systematic review and meta-analysis. Ann Saudi Med. 2016 Jul-Aug;36(4):245–251.
- Drolet M, Benard E, Perez N, et al. Population-level impact and herd effects following the introduction of human papillomavirus vaccination programmes: updated systematic review and meta-analysis. Lancet. 2019 Aug 10;394(10197):497–509.
- Guan P, Howell-Jones R, Li N, et al. Human papillomavirus types in 115,789 HPV-positive women: a meta-analysis from cervical infection to cancer. Int J Cancer. 2012 Nov 15;131(10):2349–2359.
- Chabeda A, Yanez RJR, Lamprecht R, et al. Therapeutic vaccines for high-risk HPV-associated diseases. Papillomavirus Res. 2018 Jun;5:46–58.
- Li Z, Wang D, Gu Y, et al. Crystal structures of two immune complexes identify determinants for viral infectivity and type-specific neutralization of human papillomavirus. mBio. 2017;8(5):e00787–17.
- Matthijsse SM, Naber SK, Hontelez JAC, et al. The health impact of human papillomavirus vaccination in the situation of primary human papillomavirus screening: a mathematical modeling study. PLoS One. 2018;13(9):e0202924.
- Diaz M, de Sanjose S, Bosch FX, et al. Present challenges in cervical cancer prevention: answers from cost-effectiveness analyses. Rep Pract Oncol Radiother. 2018 Nov-Dec;23(6):484–494.
- Santhanes D, Wong CP, Yap YY, et al. Factors involved in human papillomavirus (HPV) vaccine hesitancy among women in the South-East Asian Region (SEAR) and Western Pacific Region (WPR): a scoping review. Hum Vaccin Immunother. 2018 Jan 2;14(1):124–133.
- Brisson M, Kim JJ, Canfell K, et al. Impact of HPV vaccination and cervical screening on cervical cancer elimination: a comparative modelling analysis in 78 low-income and lower-middle-income countries. Lancet. 2020 Feb 22;395(10224):575–590.
- Rousseau S, Gaillot-de Saintignon J, Barret AS. Challenges in the implementation of HPV vaccination for cancer prevention. Rev Prat. 2019 May;69(5):529–534.
- Papanicolaou GN. A new procedure for staining vaginal smears. Science. 1942 Apr 24;95(2469):438–439.
- Nance KV. Evolution of Pap testing at a community hospital: a ten year experience. Diagn Cytopathol. 2007 Mar;35(3):148–153.
- Naeem RC, Goldstein DY, Einstein MH, et al. SurePath specimens versus thinprep specimen types on the COBAS 4800 platform: high-risk HPV status and cytology correlation in an ethnically diverse bronx population. Lab Med. 2017 Aug 1;48(3):207–213.
- Luttmer R, De Strooper LM, Berkhof J, et al. Comparing the performance of FAM19A4 methylation analysis, cytology and HPV16/18 genotyping for the detection of cervical (pre)cancer in high-risk HPV-positive women of a gynecologic outpatient population (COMETH study). Int J Cancer. 2016 Feb 15;138(4):992–1002.
- Luttmer R, Dijkstra MG, Snijders PJ, et al. p16/Ki-67 dual-stained cytology for detecting cervical (pre)cancer in a HPV-positive gynecologic outpatient population. Mod Pathol. 2016 Aug;29(8):870–878.
- Sargent A, Fletcher S, Bray K, et al. Cross-sectional study of HPV testing in self-sampled urine and comparison with matched vaginal and cervical samples in women attending colposcopy for the management of abnormal cervical screening. BMJ Open. 2019 Apr 29;9(4):e025388.
- Hawkes D, Keung MHT, Huang Y, et al. Self-Collection for cervical screening programs: from research to reality. Cancers (Basel). 2020 Apr 24;12(4):1053.
- Xue P, Ng MTA, Qiao Y. The challenges of colposcopy for cervical cancer screening in LMICs and solutions by artificial intelligence. BMC Med. 2020 Jun 3;18(1):169.
- Chitra B, Kumar SS. Recent advancement in cervical cancer diagnosis for automated screening: a detailed review. J Ambient Intell Humaniz Comput 2021. DOI:https://doi.org/10.1007/s12652-021-02899-2.
- Poljak M, Ostrbenk Valencak A, Gimpelj Domjanic G, et al. Commercially available molecular tests for human papillomaviruses: a global overview. Clin Microbiol Infect. 2020 Sep;26(9):1144–1150.
- Arbyn M, Snijders PJ, Meijer CJ, et al. Which high-risk HPV assays fulfil criteria for use in primary cervical cancer screening? Clin Microbiol Infect. 2015 Sep;21(9):817–826.
- Kyrgiou M, Arbyn M, Bergeron C, et al. Cervical screening: ESGO-EFC position paper of the European Society of Gynaecologic Oncology (ESGO) and the European Federation of Colposcopy (EFC). Br J Cancer. 2020 Aug;123(4):510–517.
- Zhao FH, Jeronimo J, Qiao YL, et al. An evaluation of novel, lower-cost molecular screening tests for human papillomavirus in rural China. Cancer Prev Res (Phila). 2013 Sep;6(9):938–948.
- Loonen AJM, Huijsmans CJJ, WRR G-G, et al. Performance analysis of high-throughput HPV testing on three automated workflows. APMIS. 2020 Aug;128(8):497–505.
- Torres M, Fraile L, Echevarria J, et al. Human Papillomavirus (HPV) Genotyping: automation and application in routine laboratory testing. Open Virol J. 2012;6(1):144–150.
- Fu XL, Schiffman M, Ke Y, et al. Type-dependent association between risk of cervical intraepithelial neoplasia and viral load of oncogenic human papillomavirus types other than types 16 and 18. Int J Cancer. 2017 Apr 15;140(8):1747–1756.
- Floore A, Hesselink A, Ostrbenk A, et al. Intra- and inter-laboratory agreement of the FAM19A4/mir124-2 methylation test: results from an international study. J Clin Lab Anal. 2019 May;33(4):e22854.
- Dippmann C, Schmitz M, Wunsch K, et al. Triage of hrHPV-positive women: comparison of two commercial methylation-specific PCR assays. Clin Epigenetics. 2020 Nov 11;12(1):171.
- Kurdyukov S, Bullock M, Methylation analysis: choosing the right method. Biology (Basel). 2016 Jan 6;5(1):3.
- Rauluseviciute I, Drablos F, Rye MB. DNA methylation data by sequencing: experimental approaches and recommendations for tools and pipelines for data analysis. Clin Epigenetics. 2019 Dec 12;11(1):193.
- Pouliquen DL, Boissard A, Coqueret O, et al. Biomarkers of tumor invasiveness in proteomics (Review). Int J Oncol. 2020 Aug;57(2):409–432.
- Vasiljevic N, Carter PD, Reuter C, et al. Role of quantitative p16 INK4A mRNA assay and digital reading of p16 INK4A immunostained sections in diagnosis of cervical intraepithelial neoplasia. Int J Cancer. 2017 Aug 15;141(4):829–836.
- Han C, Zhao F, Wan C, et al. Associations between the expression of SCCA, MTA1, P16, Ki-67 and the infection of high-risk HPV in cervical lesions. Oncol Lett. 2020 Jul;20(1):884–892.
- Wentzensen N, Fetterman B, Castle PE, et al. p16/Ki-67 dual stain cytology for detection of cervical precancer in HPV-Positive women. J Natl Cancer Inst. 2015 Dec;107(12):djv257.
- Wright TC Jr., Behrens CM, Ranger-Moore J, et al. Triaging HPV-positive women with p16/Ki-67 dual-stained cytology: results from a sub-study nested into the ATHENA trial. Gynecol Oncol. 2017 Jan;144(1):51–56.
- Dixon EP, King LM, Nelson R, et al. Characterization and clinical validation of MCM2 and TOP2A monoclonal antibodies in the BD ProEx C assay: an immunoassay which detects aberrant S-phase induction in cervical tissue. J Immunol Methods. 2017 Mar;442:35–41.
- Van Gool A, Corrales F, Colovic M, et al. Analytical techniques for multiplex analysis of protein biomarkers. Expert Rev Proteomics. 2020 Apr;17(4):257–273.
- Ye S, Sun X, Kang B, et al. The kinetic profile and clinical implication of SCC-Ag in squamous cervical cancer patients undergoing radical hysterectomy using the Simoa assay: a prospective observational study. BMC Cancer. 2020 Feb 21;20(1):138.
- Rivnak AJ, Rissin DM, Kan CW, et al. A fully-automated, six-plex single molecule immunoassay for measuring cytokines in blood. J Immunol Methods. 2015 Sep;424:20–27.
- Kundrod KA, Smith CA, Hunt B, et al. Advances in technologies for cervical cancer detection in low-resource settings. Expert Rev Mol Diagn. 2019 Aug;19(8):695–714.
- Krings A, Duckelmann AM, Moser L, et al. Performance of OncoE6 cervical test with collection methods enabling self-sampling. BMC Womens Health. 2018 May 21;18(1):68.
- Ndizeye Z, Menon S, Van Geertruyden JP, et al. Performance of OncoE6(TM) Cervical Test in detecting cervical precancer lesions in HIV-positive women attending an HIV clinic in Bujumbura, Burundi: a cross-sectional study. BMJ Open. 2019 Sep 6;9(9):e029088.
- Ferrera A, Valladares W, Cabrera Y, et al. Performance of an HPV 16/18 E6 oncoprotein test for detection of cervical precancer and cancer. Int J Cancer. 2019 Oct 15;145(8):2042–2050.
- Kelly H, Mayaud P, Segondy M, et al. A systematic review and meta-analysis of studies evaluating the performance of point-of-care tests for human papillomavirus screening. Sex Transm Infect. 2017 Dec;93(S4):S36–S45.
- Rezhake R, Hu SY, Zhao S, et al. Eight-type human papillomavirus E6/E7 oncoprotein detection as a novel and promising triage strategy for managing HPV-positive women. Int J Cancer. 2019 Jan 1;144(1):34–42.
- Panikar, S. S.; Banu, N.; Haramati, J.; et al. Anti-fouling SERS-based immunosensor for point-of-care detection of the B7-H6 tumor biomarker in cervical cancer patient serum. Anal Chim Acta 2020;1138:110–122.
- Ren W, Mohammed SI, Wereley S, et al. Magnetic focus lateral flow sensor for detection of cervical cancer biomarkers. Anal Chem. 2019 Feb 19;91(4):2876–2884.
- Villa LL, Denny L. CHAPTER 7 Methods for detection of HPV infection and its clinical utility. Int J Gynaecol Obstet. 2006 Nov;94(Suppl 1):S71–S80.
- Keeratichamroen S, Subhasitanont P, Chokchaichamnankit D, et al. Identification of potential cervical cancer serum biomarkers in Thai patients. Oncol Lett. 2020 Jun;19(6):3815–3826.
- Hellman K, Alaiya AA, Becker S, et al. Differential tissue-specific protein markers of vaginal carcinoma. Br J Cancer. 2009 Apr 21;100(8):1303–1314.
- Liu C, Pan C, Shen J, et al. Discrimination analysis of mass spectrometry proteomics for cervical cancer detection. Med Oncol. 2011 Dec;28(Suppl 1):S553–9.
- Kriegsmann J, Kriegsmann M, Kriegsmann K, et al. MALDI imaging for proteomic painting of heterogeneous tissue structures. Proteomics Clin Appl. 2019 Jan;13(1):e1800045.
- Van Gorp T, Cadron I, Daemen A, et al. Proteomic biomarkers predicting lymph node involvement in serum of cervical cancer patients. Limitations of SELDI-TOF MS. Proteome Sci. 2012 Jun 13;10(1):41.
- Longuespee R, Ly A, Casadonte R, et al. Identification of MALDI imaging proteolytic peptides using LC-MS/MS-Based biomarker discovery data: a proof of concept. Proteomics Clin Appl. 2019 Jan;13(1):e1800158.
- Han J, Permentier H, Bischoff R, et al. Imaging of protein distribution in tissues using mass spectrometry: an interdisciplinary challenge TrAC - Trends in Analytical Chemistry. 2019;112:13–28.
- Tzafetas M, Mitra A, Paraskevaidi M, et al. The intelligent knife (iKnife) and its intraoperative diagnostic advantage for the treatment of cervical disease. Proc Natl Acad Sci U S A. 2020 Mar 31 117(13):7338–7346.
- Paraskevaidi M, Cameron SJS, Whelan E, et al. Laser-assisted rapid evaporative ionisation mass spectrometry (LA-REIMS) as a metabolomics platform in cervical cancer screening. EBioMedicine. 2020 Oct;60:103017.
- Shu W, Wang Y, Liu C, et al. Construction of a plasmonic chip for metabolic analysis in cervical cancer screening and evaluation small methods. Small Methods. 2020;4:4.
- Zhou W, Huang C, Zou X, et al. Exhaled breath online measurement for cervical cancer patients and healthy subjects by proton transfer reaction mass spectrometry. Anal Bioanal Chem. 2017 Sep;409(23):5603–5612.
- Khan I, Nam M, Kwon M, et al. LC/MS-Based polar metabolite profiling identified unique biomarker signatures for cervical cancer and cervical intraepithelial neoplasia using global and targeted metabolomics. Cancers (Basel). 2019 Apr 10;11(4):511.
- Govorukhina NI, Reijmers TH, Nyangoma SO, et al. Analysis of human serum by liquid chromatography-mass spectrometry: improved sample preparation and data analysis. J Chromatogr A. 2006 Jul 7;1120(1–2):142–150.
- Hao Y, Ye M, Chen X, et al. Discovery and validation of FBLN1 and ANT3 as potential biomarkers for early detection of cervical cancer. Cancer Cell Int. 2021 Feb 18;21(1):125.
- Kontostathi G, Zoidakis J, Anagnou NP, et al. Proteomics approaches in cervical cancer: focus on the discovery of biomarkers for diagnosis and drug treatment monitoring. Expert Rev Proteomics. 2016 Aug;13(8):731–745.
- Guo X, Hao Y, Kamilijiang M, et al. Potential predictive plasma biomarkers for cervical cancer by 2D-DIGE proteomics and ingenuity pathway analysis. Tumour Biol. 2015 Mar;36(3):1711–1720.
- Bae SM, Lee CH, Cho YL, et al. Two-dimensional gel analysis of protein expression profile in squamous cervical cancer patients. Gynecol Oncol. 2005 Oct;99(1):26–35.
- Govorukhina N, Horvatovich P, Bischoff R. Label-free proteomics of serum. Methods Mol Biol. 2008;484:67–77.
- Chokchaichamnankit D, Watcharatanyatip K, Subhasitanont P, et al. Urinary biomarkers for the diagnosis of cervical cancer by quantitative label-free mass spectrometry analysis. Oncol Lett. 2019 Jun;17(6):5453–5468.
- Boichenko AP, Govorukhina N, Klip HG, et al. A panel of regulated proteins in serum from patients with cervical intraepithelial neoplasia and cervical cancer. J Proteome Res. 2014 Nov 7;13(11):4995–5007.
- Güzel C, Govorukhina NI, Wisman GBA, et al. Proteomic alterations in early stage cervical cancer. Oncotarget. 2018;9(26):18128–18147.
- Filippova M, Filippov V, Williams VM, et al. Cellular levels of oxidative stress affect the response of cervical cancer cells to chemotherapeutic agents. Biomed Res Int. 2014;2014:574659.
- Guzel C, Govorukhina NI, Stingl C, et al. Comparison of targeted mass spectrometry techniques with an immunoassay: a case study for HSP90alpha. Proteomics Clin Appl. 2018;12:1700107.
- Stevens KG, Pukala TL. Conjugating immunoassays to mass spectrometry: solutions to contemporary challenges in clinical diagnostics. Trends Analyt Chem. 2020 Nov;132:116064.
- Pratt MS, van Faassen M, Remmelts N, et al. An antibody-free LC-MS/MS method for the quantification of intact insulin-like growth factors 1 and 2 in human plasma. Anal Bioanal Chem. 2021 Mar;413(8):2035–2044.
- Klont F, Pouwels SD, Hermans J, et al. A fully validated liquid chromatography-mass spectrometry method for the quantification of the soluble receptor of advanced glycation end-products (sRAGE) in serum using immunopurification in a 96-well plate format. Talanta. 2018 May 15;182:414–421.
- Klont F, Hadderingh M, Horvatovich P, et al. Affimers as an alternative to antibodies in an affinity LC-MS assay for quantification of the Soluble Receptor of Advanced Glycation End-Products (sRAGE) in human serum. J Proteome Res. 2018 Aug 3;17(8):2892–2899.
- Szalmas A, Konya J. Epigenetic alterations in cervical carcinogenesis. Semin Cancer Biol. 2009 Jun;19(3):144–152.
- Kremer WW, Steenbergen R, Heideman D, et al. The use of host cell DNA methylation analysis in the detection and management of women with advanced cervical intraepithelial neoplasia: a review. BJOG. 2021 Feb;128(3):504–514.
- Laengsri V, Kerdpin U, Plabplueng C, et al. Cervical Cancer Markers: epigenetics and microRNAs. Lab Med. 2018 Mar 21;49(2):97–111.
- Bhat S, Kabekkodu SP, Noronha A, et al. Biological implications and therapeutic significance of DNA methylation regulated genes in cervical cancer. Biochimie. 2016 Feb;121:298–311.
- Lorincz AT. Cancer diagnostic classifiers based on quantitative DNA methylation. Expert Rev Mol Diagn. 2014 Apr;14(3):293–305.
- Verma M. The role of epigenomics in the study of cancer biomarkers and in the development of diagnostic tools. Adv Exp Med Biol. 2015;867:59–80.
- Fang J, Zhang H, Jin S. Epigenetics and cervical cancer: from pathogenesis to therapy. Tumour Biol. 2014 Jun;35(6):5083–5093.
- Wilting SM, Steenbergen RDM. Molecular events leading to HPV-induced high grade neoplasia. Papillomavirus Res. 2016 Dec;2:85–88.
- Boers A, Wang R, van Leeuwen RW, et al. Discovery of new methylation markers to improve screening for cervical intraepithelial neoplasia grade 2/3. Clin Epigenetics. 2016;8(1):29.
- De Strooper LMA, Verhoef VMJ, Berkhof J, et al. Validation of the FAM19A4/mir124-2 DNA methylation test for both lavage- and brush-based self-samples to detect cervical (pre)cancer in HPV-positive women. Gynecol Oncol. 2016 May;141(2):341–347.
- Luttmer R, De Strooper LM, Dijkstra MG, et al. FAM19A4 methylation analysis in self-samples compared with cervical scrapes for detecting cervical (pre)cancer in HPV-positive women. Br J Cancer. 2016 Aug 23;115(5):579–587.
- Lorincz AT, Brentnall AR, Scibior-Bentkowska D, et al. Validation of a DNA methylation HPV triage classifier in a screening sample. Int J Cancer. 2016 Jun 1;138(11):2745–2751.
- Dick S, Verhoef L, De Strooper LM, et al. Evaluation of six methylation markers derived from genome-wide screens for detection of cervical precancer and cancer. Epigenomics. 2020 Sep;12(18):1569–1578.
- Babion I, De Strooper LMA, Luttmer R, et al. Complementarity between miRNA expression analysis and DNA methylation analysis in hrHPV-positive cervical scrapes for the detection of cervical disease. Epigenetics. 2019 Jun;14(6):558–567.
- Schmitz M, Eichelkraut K, Schmidt D, et al. Performance of a DNA methylation marker panel using liquid-based cervical scrapes to detect cervical cancer and its precancerous stages. BMC Cancer. 2018 Dec 3;18(1):1197.
- Lorincz AT. Virtues and weaknesses of DNA methylation as a test for cervical cancer prevention. Acta Cytol. 2016;60(6):501–512.
- Ikenberg H, Bergeron C, Schmidt D, et al. Screening for cervical cancer precursors with p16/Ki-67 dual-stained cytology: results of the PALMS study. J Natl Cancer Inst. 2013 Oct 16;105(20):1550–1557.
- Sarwath H, Bansal D, Husain NE, et al. Introduction of p16(INK4a) as a surrogate biomarker for HPV in women with invasive cervical cancer in Sudan. Infect Agent Cancer. 2017;12(1):50.
- von Knebel Doeberitz M, Reuschenbach M, Schmidt D, et al. Biomarkers for cervical cancer screening: the role of p16(INK4a) to highlight transforming HPV infections. Expert Rev Proteomics. 2012 Apr;9(2):149–163.
- Stevenson A, Kavanagh K, Pan J, et al. Risk stratification of cervical disease using detection of human papillomavirus (HPV) E4 protein and cellular MCM protein in clinical liquid based cytology samples. J Clin Virol. 2018 Nov;108:19–25.
- Amaro Filho SM, Nuovo GJ, Cunha CB, et al. Correlation of MCM2 detection with stage and virology of cervical cancer. Int J Biol Markers. 2014 Dec 9;29(4):e363–71.
- Valverde LF, de Freitas RD, Pereira TA, et al. MCM3: a novel proliferation marker in oral squamous cell carcinoma. Appl Immunohistochem Mol Morphol. 2018 Feb;26(2):120–125.
- Aporowicz M, Czopnik P, Kubicka E, et al. Minichromosome maintenance proteins MCM-3, MCM-5, MCM-7, and Ki-67 as proliferative markers in adrenocortical tumors. Anticancer Res. 2019 Mar;39(3):1151–1159.
- Branca M, Giorgi C, Ciotti M, et al. Over-expression of topoisomerase IIalpha is related to the grade of cervical intraepithelial neoplasia (CIN) and high-risk human papillomavirus (HPV), but does not predict prognosis in cervical cancer or HPV clearance after cone treatment. Int J Gynecol Pathol. 2006 Oct;25(4):383–392.
- Jenson EG, Baker M, Paydarfar JA, et al. MCM2/TOP2A (ProExC) immunohistochemistry as a predictive marker in head and neck mucosal biopsies. Pathol Res Pract. 2014 Jun;210(6):346–350.
- Ebisch RM, van der Horst J, Hermsen M, et al. Evaluation of p16/Ki-67 dual-stained cytology as triage test for high-risk human papillomavirus-positive women. Mod Pathol. 2017 Jul;30(7):1021–1031.
- Kelly D, Kincaid E, Fansler Z, et al. Detection of cervical high-grade squamous intraepithelial lesions from cytologic samples using a novel immunocytochemical assay (ProEx C). Cancer. 2006 Dec 25;108(6):494–500.
- Moiseeva TN, Bakkenist CJ. Regulation of the initiation of DNA replication in human cells. DNA Repair (Amst). 2018 Dec;72:99–106.
- Bialik S, Berissi H, Kimchi A. A high throughput proteomics screen identifies novel substrates of death-associated protein kinase. Mol Cell Proteomics. 2008 Jun;7(6):1089–1098.
- Fei L, Xu H. Role of MCM2-7 protein phosphorylation in human cancer cells. Cell Biosci. 2018;8(1):43.
- Yang P, Chen N, Yang D, et al. The ratio of serum Angiopoietin-1 to Angiopoietin-2 in patients with cervical cancer is a valuable diagnostic and prognostic biomarker. PeerJ. 2017;5:e3387.
- Li X, Tian R, Gao H, et al. Identification of significant gene signatures and prognostic biomarkers for patients with cervical cancer by integrated bioinformatic methods. Technol Cancer Res Treat. 2018 Jan 1;17:1533033818767455.
- Friese K, Kost B, Vattai A, et al. The G protein-coupled estrogen receptor (GPER/GPR30) may serve as a prognostic marker in early-stage cervical cancer. J Cancer Res Clin Oncol. 2018 Jan;144(1):13–19.
- Chen Y, Xiong X, Wang Y, et al. Proteomic screening for serum biomarkers for cervical cancer and their clinical significance. Med Sci Monit. 2019 Jan;9(25):288–297.
- Guo X, Abliz G, Reyimu H, et al. The association of a distinct plasma proteomic profile with the cervical high-grade squamous intraepithelial lesion of Uyghur women: a 2D liquid-phase chromatography/mass spectrometry study. Biomarkers. 2012 Jun;17(4):352–361.
- Curty G, de Carvalho PS, Soares MA. The role of the cervicovaginal microbiome on the genesis and as a biomarker of premalignant cervical intraepithelial neoplasia and invasive cervical cancer. Int J Mol Sci. 2019 Dec 28;21(1)222.
- Castanheira CP, Sallas ML, Nunes RAL, et al. Microbiome and cervical cancer. Pathobiology. 2021;88(2):187–197.
- Champer M, Wong AM, Champer J, et al. The role of the vaginal microbiome in gynaecological cancer. BJOG. 2018 Feb;125(3):309–315.
- Starodubtseva NL, Brzhozovskiy AG, Bugrova AE, et al. Label-free cervicovaginal fluid proteome profiling reflects the cervix neoplastic transformation. J Mass Spectrom. 2019 Aug;54(8):693–703.
- Liu C, Pan C, Shen J, et al. Discrimination analysis of mass spectrometry proteomics for cervical cancer detection. Med Oncol. 2011 Dec;28(Suppl 1):S553–9.
- Panicker G, Ye Y, Wang D, et al. Characterization of the human cervical mucous proteome. Clin Proteomics. 2010 6;Jun(1–2):18–28.
- Van Ostade X, Dom M, Tjalma W, et al. Candidate biomarkers in the cervical vaginal fluid for the (self-)diagnosis of cervical precancer. Arch Gynecol Obstet. 2018 Feb;297(2):295–311.
- Gutierrez AL, Lindberg JH, Shevchenko G, et al. Identification of candidate protein biomarkers for CIN2+ lesions from self-sampled, dried cervico-vaginal fluid using LC-MS/MS. Cancers (Basel). 2021 May 25;13(11):2592.
- Cardoso MFS, Castelletti CHM, Lima-Filho JL, et al. Putative biomarkers for cervical cancer: SNVs, methylation and expression profiles. Mutat Res. 2017 Jul;773:161–173.
- van Baars R, Griffin H, Wu Z, et al. Investigating diagnostic problems of CIN1 and CIN2 associated with high-risk HPV by combining the novel molecular biomarker PanHPVE4 with P16INK4a. Am J Surg Pathol. 2015 Nov;39(11):1518–1528.
- Griffin H, Soneji Y, Van Baars R, et al. Stratification of HPV-induced cervical pathology using the virally encoded molecular marker E4 in combination with p16 or MCM. Mod Pathol. 2015 Jul;28(7):977–993.
- de Kok I, Korfage IJ, van den Hout WB, et al. Quality of life assumptions determine which cervical cancer screening strategies are cost-effective. Int J Cancer. 2018 Jun 1;142(11):2383–2393.
- Jansen E, Naber SK, Aitken CA, et al. Cost-effectiveness of HPV-based cervical screening based on first year results in the Netherlands: a modelling study. BJOG. 2021 Feb;128(3):573–582.
- Kaljouw S, Jansen EEL, Aitken CA, et al. Reducing unnecessary referrals for colposcopy in hrHPV-positive women within the Dutch cervical cancer screening programme: a modelling study. Gynecol Oncol. 2021 Mar;160(3):713–720.
- Durst M, Gissmann L, Ikenberg H, et al. A papillomavirus DNA from a cervical carcinoma and its prevalence in cancer biopsy samples from different geographic regions. Proc Natl Acad Sci U S A. 1983 Jun;80(12):3812–3815.
- zur Hausen H. Papillomaviruses and cancer: from basic studies to clinical application. Nat Rev Cancer. 2002 May 2;2(5):342–350.