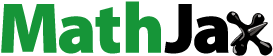
ABSTRACT
Forest fuel production from overgrowth brushwood is an option when regular clearing of forest roadsides or edges of arable land has been neglected for some reason. The basic economic advantages of recovery are that the cost of harvesting can be offset by the existing clearing costs and income from the harvested biomass. The objectives of this study were to: 1) produce a productivity model for a machine unit that can continuously cut and accumulate brushwood trees during linear crane movement; 2) determine the kind of cases when brushwood biomass should be recovered, when the harvesting is based on a one- or two-machine configuration. The cost analyses were performed as a spreadsheet-based simulated treatment, using existing or the productivity models produced herein, and cost parameters for brushwood clearing and harvesting. The Risupeto feller-buncher unit studied in this study was capable of cutting and accumulating trees with a continuous movement that enabled the efficient harvesting of roadside and field-edge brushwood for fuel. The economic result in the brushwood harvesting with the harwarder and Risupeto forwarder configuration was strongly affected by the average volume of the brushwood trees, as well as harvesting removal on the site. Correspondingly, the roadside price of whole trees had a great impact on the potential revenue of brushwood harvesting.
Introduction
Forest fuel production from overgrowth brushwood is an option when regular clearing of forest roadsides, the edges of arable land, power line corridors and other infrastructural objects has been neglected for some reason (Belbo Citation2011; Ebenhard et al. Citation2017; Fernandez-Lacruz Citation2019). Overgrowth brushwood, e.g. in the edge zone of arable land, shadows the field and reduces the harvest, and can even prevent the functioning of the ditching system if the woody vegetation is not cleared regularly. Such brushwood consists of saplings, bushes and young trees of a range of deciduous plant species mixed with conifers (Ebenhard et al. Citation2017). The basic economic advantages of brushwood recovery are that the cost of harvesting can be offset by the existing clearing costs and income from the harvested biomass (Iwarsson-Wide Citation2009a, Citation2009b, Citation2009c; Fernandez-Lacruz et al. Citation2020).
Normal forest road maintenance includes regular clearing of brushwood along roadsides, e.g. with rotor or chain mulchers, and thereby only involving cost but no revenue (Korpilahti Citation2008; Iwarsson-Wide Citation2011; Kiss et al. Citation2015; Kaakkurivaara Citation2018). Clearing cycles and management distances are derived from existing clearing technology, growing conditions, and species; faster growth on fertile soil requires more attention. If regular clearing of brushwood is neglected, overgrowth brushwood narrows the width of the functional roadway, prevents the proper drainage of the road surface, and can complicate use of the road e.g. timber transportation from forests to mills. In the worst case, overgrowth brushwood (diameter at 1.3-meter height over 5 cm) cannot be cleared by normal road maintenance machinery and instead must use harvesting technologies developed for small tree harvesting (Korpilahti Citation2008). In contrast to brushwood clearing, the productivity of mechanized small tree harvesting improves with increasing tree size and harvest intensity (Unrau et al. Citation2018; Laitila and Väätäinen Citation2020).
A multi-tree handling capability is a typical feature of current small tree harvesting heads. Many types of harvesting heads are currently available (e.g. Kärhä Citation2006; Bergström Citation2009; Belbo Citation2011; Laitila Citation2012; Petty Citation2014; Schweier et al. Citation2015; Erber et al. Citation2016; Spinelli et al. Citation2017). They can be classified by their cutting elements, which include disk saws, saw bars, and shear blades, the latter in either elliptical, guillotine, or scissor-like configurations (Erber and Kühmaier Citation2017). Harvesting heads developed for small tree harvesting are usually applied either in a one-machine (harwarder) or two-machine (harvester and forwarder) configuration (Belbo Citation2010; Laitila and Asikainen Citation2006; Kärhä Citation2006; Laitila Citation2008; Rottensteiner et al. Citation2008; Iwarsson-Wide Citation2009c), and can be mounted on a large range of other base machines, such as excavators or tractors. The advantages of excavators and tractors produced in high volumes include a purchase price lower than that of conventional forest machines such as harvesters and forwarders (Belbo Citation2011; Laitila and Väätäinen Citation2013; Malinen et al. Citation2016).
A harwarder is a dual-purpose machine for cutting and forwarding, and the harwarder head is capable of cutting, accumulating, and processing, as well as loading and unloading harvested trees (Belbo Citation2010; Laitila and Asikainen Citation2006; Kärhä Citation2006; Laitila Citation2008; Rottensteiner et al. Citation2008; Iwarsson-Wide Citation2009c). The competitiveness of the forwarder equipped for combined use is based on the large proportion of cutting work in relation to forwarding and the low relocation costs compared to operating with the conventional harvesting system using two machines (Kärhä Citation2006; Laitila Citation2008; Kärhä et al. Citation2018).
When harvesting small diameter trees in thinnings, costs often exceed the potential revenues (Oikari et al. Citation2010; Petty Citation2014). Tree volume governs the productivity in small tree harvesting and for each situation must identify the minimum tree volume that makes harvesting economical: Below such a size, productivity does not reach the required level, and the value of the harvest fails to exceed the machine’s operating cost (Oikari et al. Citation2010; Petty Citation2014). Belbo (Citation2011) lists strategies for reducing harvesting costs in small tree harvesting:
Improving the accumulation and packaging capacity of the accumulating harvesting head.
Employing a machine system with lower capital costs than dedicated forest machine systems.
Continuous felling and bunching of trees during crane movement
Although the harvesting technologies used in overgrowth brushwood harvesting can be like those used in conventional forestry in early thinnings, the harvesting productivity and costs can be expected to differ in integrated brushwood clearing and harvesting due to the different operating environment. In conventional thinning, where the aim is to optimize the future production of high-value roundwood, the operator selects the trees to be thinned and the permanence of the residual stand hinders machine movements, limiting the theoretical accumulation capacity of the harvester head (Belbo Citation2011; Petty Citation2014). Whereas in integrated roadside or field-edge brushwood clearing and harvesting, all the biomass from a specific area is harvested systematically, there is no remaining tree stand which will hamper harvesting, and the time spent in tree selection can be avoided.
Compared to small tree harvesting from early thinning (Kärhä Citation2006; Bergström Citation2009; Belbo Citation2011; Jylhä Citation2011; Laitila Citation2012; Röser Citation2012; Petty Citation2014; Windisch Citation2015), expertise in the cost-effective utilization and treatment of brushwood in forest roadside and field-edge sites is limited, and it is unclear in which cases brushwood biomass should be recovered (i.e. harvested for fuel), and in which it should be left to decay by clearing. The density and dimensions of brushwood, e.g. along the forest roadside and field edges, vary greatly, and the problem is that biomass-rich forest road sections are embedded in the less biomass-rich sections. In some sections of forest roadsides, there is plenty of tall brushwood, while others have virtually no brushwood that is suitable for harvesting but are excellent sections for clearing with normal maintenance machinery, such as rotor or chain mulchers (Iwarson-Wide Citation2011). In 2008 and 2009, Skogforsk conducted two case studies of brushwood harvesting along forest roadsides (Iwarsson-Wide Citation2009a, Citation2009b). In their conclusions, they highlight that there is currently no machine on the market that can fell and handle both larger dimension trees and bushes in a single operation (Iwarsson-Wide Citation2011).
The objectives of this study were to: 1) define the productivity and produce a time consumption model for the integrated clearing and harvesting of roadside and field-edge brushwood with a machine unit that can continuously cut and accumulate brushwood trees during the linear crane movement; 2) determine the cases in which brushwood biomass should be recovered when the integrated clearing and harvesting of brushwood is based on a one- or two-machine configuration at the roadside or in field-edge sites. The cost analyses were performed as a spreadsheet-based simulated treatment, using existing models or the productivity models produced herein, and cost parameters for the brushwood clearing and harvesting. The cost analyses were made from the harvesting entrepreneur’s perspective of whether the compensation for selling energy wood added to the compensation of the clearing costs was enough to cover the costs of integrated harvesting and clearing.
Materials and methods
Field study
Machinery studied
Risupeto (www.reformet.fi/risupeto/) is an accumulating harvesting head that is capable of continuous felling and bunching of brushwood trees during linear crane movement (). The prototype felling head cuts standing trees with two parallel disk sawblades and accumulates trees in an upright position to the collecting chamber using rotating collecting arms. The spring-loaded collecting arms are attached to the two vertical cylinders, which rotate at the same speed as the disk sawblades (). When the collecting chamber of the felling head is full, the accumulated tree bunch is moved to the pile and dropped out. The unloading of the tree bunch is done by tilting the felling head downward and rotating the disk saws and collecting arms in the opposite direction from that during cutting. The width of the hydraulically powered accumulating felling head is 1.0 m, and the maximum cutting diameter with one cut is 30 cm. The height of the accumulating felling head is 150 cm, and it weighs 750 kg.
Figure 1. The integrated harvesting and clearing of brushwood were done with the Risupeto disk saw feller-buncher unit in the study. The Risupeto accumulating felling head was attached to the boom tip of the medium-sized New Holland Kobelco E 200 SR crawler excavator
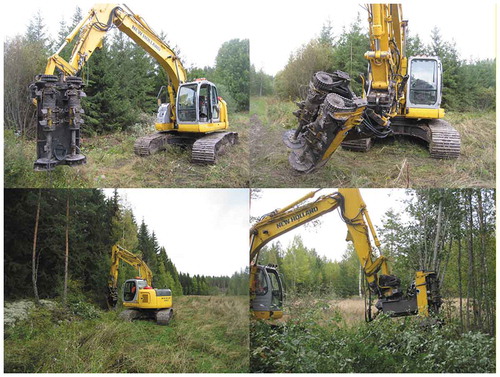
The studied Risupeto accumulating felling head was attached with a tilt rotator to the boom tip of the medium-sized New Holland Kobelco E 200 SR crawler excavator (20.4 tonnes, 92 kW), providing a total reach of 10 meters. The rotation speed of the disk sawblades, which were made of high-strength wear-resistant steel, was 990 rotations per minute. The base machine’s hydraulic oil flow and pressure were 300 l/min and 270 bar. The operator was skillful, with 20 years’ work experience of driving excavators and forest machines. In addition, he was the inventor and developer of the Risupeto accumulating harvester head, with two years’ harvesting work experience using the prototype device.
Time study plots and brushwood measurements
Time studies were carried out on an abandoned farming site in Iitti, Southeast Finland (61°02ʹN 26°12ʹE). The ditches alongside the road and field had been excavated for the last time in the late 1980s and clearing of field-edge and roadside brushwood had been done randomly or completely neglected. Alongside the forest road and field-edge 17 time study plots were established for the Risupeto disk saw feller-buncher unit. In establishing the time study plots, the aim was that the number and volume of trees should be varied between the plots. The length of the rectangular time study plots was 25 m, and the widths equaled the measured work path, which was an average of 3.0 m wide. The boundaries of the time study plots alongside the forest road and field edge were marked by marking paints and ribbons.
The initial data from the time study plots were based on stump diameter measurements by tree species, which were carried out after the harvesting and clearing of brushwood. For the time study plots, two rectangular (5 m long and 3 m wide) sample plots were established that were systematically located 5 m from the beginning and end of the time study plot. The coverage of the sample plot measurements was 40% of the total time study plot area. At the sample plots, the stump diameter was measured at a height of 10 cm from the ground to an accuracy of 1 mm, while the tree species was determined visually.
The stump diameter data by tree species were complemented by sample tree data, which consisted of measured information concerning tree height, DBH (diameter at a height of 1.3 m), and diameter at the stump height (10 cm from the ground). The accuracy of the tree height measurements was 10 cm, and the diameters were measured to an accuracy of 1 mm. The randomly selected sample trees were measured when establishing the time study plots before the beginning of the time studies. The aim was to get comprehensive measurement data for modeling brushwood tree height and DBH. There were a total of 88 sample trees from all the time study plots. The height of the measured sample trees varied between 2.5 and 13.4 m, and the DBH varied between 10 and 214 mm. The average height and DBH of the sample trees were 6.8 m, with a standard deviation (SD) of 3.0 m and 72 mm (SD 45). The stump diameter of the sample trees varied between 15 and 243 mm, and the average stump diameter of the sample trees was 92 mm (SD 52).
The measured sample tree data were combined as a data matrix, and common regression models were derived for brushwood tree height and DBH on that basis, in which the stump diameter was the independent variable. The derived regression models, with the biomass models of Repola et al. (Citation2007) for conifers and deciduous trees, were used to describe the brushwood properties and dimensions in the time study plots (17) before harvesting and clearing. The regression models for brushwood tree height and DBH, as well as the statistical characteristics of regression models, are detailed in . The estimated share of tree species from the total cutting removal was 9% for Scots pine (Pinus sylvestris L), 14% for Norway spruce (Picea abies L), 16% for Downy birch (Betula pubescens Ehrh.), and 61% for other broadleaved tree species such as aspen, alder, rowan, and willow.
Table 1. The statistical characteristics of regression models for brushwood tree height and DBH, in which the stump diameter (x1) is the independent variable
. The statistical characteristics of regression models for brushwood tree height and DBH, in which the stump diameter (x1) is the independent variable.
Time study
The time study of the Risupeto disk saw feller-buncher unit was conducted between 17 and 18 September 2019, and the brushwood was harvested but not delimbed. The harvested brushwood was weighed during unloading by the Valmet 860.1 forwarder loader equipped with an Epec LoadOptimizer crane scale with an accuracy of 2 kg. The weighed biomass was converted to m3 (solid) based on the relative proportions of tree species on the time study plot. The relative proportion of tree species on the time study plot were based on the above-mentioned stumps diameter measurements, regression models for height and DBH () and the biomass models of Repola et al. (Citation2007). The number of forwarder loads weighed was the same as the number of time study plots. The weighed cutting removal from time study plots was converted to m3 with tree species distribution specific green density (kg m−3) values produced by the Finnish Forest Research Institute (Ministry of Agriculture and Forestry Citation2010; Lindblad et al. Citation2010). The average green density was 862 kg m−3 (SD 55) in the time study plots.
The respective values for the total number of brushwood trees harvested and volume were 1,179 and 21.9 m3 during the time study. The average volume of the harvested trees varied between 6 and 54 dm3, the harvesting intensity was 0.6 to 1.4 harvested trees per m2 (5,600–14,400 trees per hectare, ha), and the harvesting removal was 0.39–3.26 m3 in the time study plots (52–434 m3 per ha). The volume of brushwood trees harvested (dm3) in a time study plot was calculated by dividing the volume harvested (m3) by the number of trees harvested. The number of trees harvested was obtained from the time study, in which the number of trees in each felling head bunch during cutting was observed and recorded (). The average stump diameter was 41 mm (SD 21), and it varied between 21 and 97 mm in the time study plots. Based on the stump diameter measurements, the estimated average height of trees per time study plot ranged from 3.3 to 7.0 m.
Table 2. Work elements with detailed definitions of the integrated harvesting and clearing of brushwood with the Risupeto disk saw feller-buncher unit in the study
A single researcher was responsible for collecting all the time study material, using a Rufco-900 handheld field computer. The recording accuracy of the field computer was 0.6 seconds. The working time was recorded through the application of a continuous timing method wherein a clock ran continuously, and the times for different phases were separated from each other under distinct numeric codes (Harstela Citation1991; Magagnotti et al. Citation2013). During the recording, the cutting functions had the highest priority, followed by the moving and arrangement elements. The time for the highest prioritized work element was recorded if multiple work elements were performed simultaneously. Auxiliary time use (e.g. planning of work and preparations) was included in the work phases in which it was observed. Productive machine (PM) time, the working time excluding all delays (IUFRO Citation1995), was divided into the work phases listed in .
Data analysis
In the modeling of the integrated harvesting and clearing of brushwood with the Risupeto disk saw feller-buncher, the recorded plot-wise time study data and the measured harvested brushwood volumes were combined as a data matrix. The time consumption of working elements––positioning to cut, accumulating felling, and arranging the brushwood tree bunch into a pile––were combined for the felling-bunching time. The time consumption of the two main work elements (felling-bunching and moving) was formulated through the application of regression analysis, in which the harvesting conditions, volume of harvested brushwood trees (dm3), and harvesting intensity (harvested brushwood trees per ha) were independent variables. The unit for the calculation of productive machine time consumption was seconds per harvested brushwood tree, and productivity was expressed in solid cubic meters per productive machine hour time (m3 per PMh) or hectares per productive machine hour time (ha per PMh).
Statistical analyses were carried out with IBM SPSS Statistics 21.0 statistical software. Different transformations and curve types were tested to achieve symmetrical residuals for the derived regression models and ensure the statistical significance of the coefficients.
Cost analysis
Productivity and cost parameters
In the one-machine configuration, the productivity of harwarder logging was based on the study of Laitila and Väätäinen (Citation2020), which can be considered as a preliminary study for this study. In the two-machine configuration, the productivity of felling-bunching was based on the results of this study, and forwarding productivity was calculated by means of the model by Laitila et al. (Citation2007). The payload of the harwarder and the forwarder was 7.1 m3, and it was set in line with the work of Laitila and Väätäinen (Citation2020). The productive machine hour (PMh) productivities of harwarder logging, felling-bunching by Risupeto, and forwarding were converted to operating hour productivities––also known as scheduled machine hour (SMh) productivity––by the coefficients of 1.3, 1.25, and 1.2 respectively (Laitila Citation2008). The calculated operating hour costs for the harvesting machinery are presented in the .
Table 3. Hourly cost details of the harvesting machines without value added tax (VAT 0%)
The average roadside price of €20.70 m−3 for whole trees (SD 1.5), which was the value of harvested brushwood as an energy wood, was based on the official price statistics of delivery sales between 2018 and 2020 in Finland (Volumes and … Citation2020). In addition, it was assumed that nominal compensation was paid for the clearing work, and the compensation was allocated per cubic meter of brushwood cleared. The productivity of regular brushwood clearing (1.55 or 0.65 operating hour per cleared kilometer when the average stump diameter was 30 mm or 40 mm) was defined based on the study results of Laitila and Väätäinen (Citation2020), and the operating hour cost of €68.40 for clearing machinery (excluding value-added tax, VAT 0%) were obtained from the recent statistics of the TTS Institute (Palva Citation2019). Based on the parameters described above, the clearing cost for brushwood harvesting was €0.04 or €0.10 per harvesting work path meter. The width of the harvesting work path was 3 m, and the clearing compensation per volume of harvested brushwood (m3) thus depended on harvesting removal from the 1 m x 3 m area (=brushwood concentration, m3 per harvesting path m). In the cost analyses, the roadside price of whole trees or clearing compensation were compared to the harvesting cost of brushwood as a function of brushwood tree volume, forwarding distance, harvesting removal, and harvesting site size.
The operating hour cost calculations
The hourly costs (excluding value-added tax, VAT) of the harvesting machinery were calculated by the common machine cost calculation method (Ackerman et al. Citation2014), and costs were presented in euros per operating hour (). The costs included both time-dependent costs (e.g. capital depreciation, interest expenses, labor costs, insurance fees, and administration expenses) and variable operating expenses (e.g. fuel, repair, service, and machine relocations). In addition to the annual total cost, 5% was added to take the entrepreneurship risk and profit margin into account ().
The acquisition prices of the harvesting machinery were based on the prices given by machine vendors (VAT 0%). The calculation values for labor costs, relocation costs, fuel, insurance fees, repairs, and service expenses were obtained from forest machine entrepreneurs. The depreciation period for the base machines was 5.5 years, and for the equipment, 3.2 years. The salvage value was 25% of the purchase price of base machines and equipment. An interest rate of 3.0% was applied, and the annual operating hours for the harvesting machinery were 2,200 hours. The degree of machine utilization was 85% for the excavator equipped with Risupeto, 87.5% for the harwarder, and 90% for the forwarder. The relocation cost per one machine relocation was €174 per harvesting site. Wages were €16 h−1 for the harwarder and Risupeto operators, and €14 h−1 for the forwarder operator, with additional indirect wage costs of 57%. With the above figures factored in, the calculated operating hour cost for the harwarder was €98.40, €86 for the excavator equipped with Risupeto accumulating felling head, and €77.70 for the forwarder ().
Results
Time consumption of working elements
In the time study, moving between working locations represented 12% of the productive machine time () with the Risupeto disk saw feller-buncher unit. Positioning to cut and accumulating felling constituted 13% and 56% of the effective working time in the integrated harvesting and clearing of brushwood. Cross-cutting of standing trees at elevations higher than stump height took 1% of the effective working time, and arranging the brushwood tree bunches into piles, 17%.
Time consumption models for harvesting and clearing
The statistical characteristics of regression models for brushwood harvesting with the Risupeto disk saw feller-buncher unit are detailed in . Statistical criteria for accepting regression models and the variables of models was that residuals were linear and systematically distributed against predicted values and t-test p-values showed significance (p < 0.005) for each approved variable of the regression model.
Table 4. Regression model statistics for harvesting with the Risupeto disk saw feller-buncher unit (x1 = number of harvested brushwood trees per hectare, x2 = the average volume of the harvested brushwood trees)
Moving time (TMoving) depended on the number of harvested brushwood trees per hectare. The moving time per brushwood tree harvested decreased as the number of brushwood trees harvested per hectare increased; in such cases, it was possible to cut more trees from a single work location (). The time consumption of moving was formulated as (EquationEquation 1(1)
(1) ):
Figure 3. The time consumption of moving between working locations with the excavator-based Risupeto disk saw feller-buncher unit as a function of brushwood trees harvested per hectare
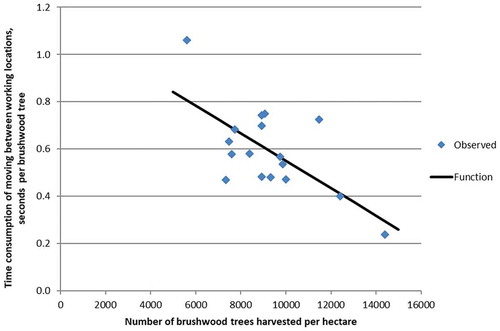
where
TMoving = time moving between working locations, expressed in seconds per harvested brushwood tree,
and x1 = number of harvested brushwood trees per hectare, ha.
The time consumption of multi-tree felling-bunching (TFelling-bunching), expressed in seconds per harvested brushwood tree, was modeled from the average volume of harvested brushwood tree of each study plot (). The time consumptions of felling-bunching increased when larger-sized brushwood trees were harvested. The time consumption of multi-tree felling-bunching was formulated as (EquationEquation 2(2)
(2) ):
Figure 4. The time consumption of multi-tree felling-bunching with the excavator-based Risupeto disk saw feller-buncher unit as a function of average volume of brushwood trees harvested (dm3)
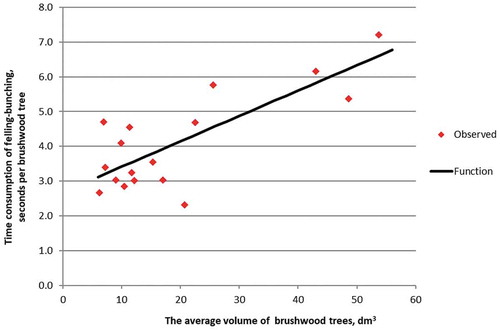
where
TFelling-bunching = time consumption of multi-tree felling-bunching, expressed in seconds per harvested brushwood tree,
and x2 = the average volume of the harvested brushwood trees, dm3
Total time consumption and productivity
The total time consumption of the integrated harvesting and clearing of roadside brushwood per harvested brushwood tree (TTotal) with the Risupeto disk saw feller-buncher unit was the sum of the two working elements (EquationEquation 3(3)
(3) ):
The number of harvested brushwood trees per PMh was calculated by dividing 3,600 seconds by the total time consumption of a harvested brushwood tree (TTotal). The PMh productivity, expressed as the number of brushwood trees harvested per PMh, was converted to m3 by multiplying the number of harvested trees by the average volume of the harvested brushwood trees (x2). The clearing productivity, expressed as hectares per PMh, was calculated based on tree-wise time consumption and the number of harvested brushwood trees per hectare.
Harvesting productivity was more sensitive to the average volume of harvested brushwood trees than to the number of harvested trees per hectare (). While the average volume of brushwood trees doubled, from 20 to 40 dm3, harvesting productivity increased by 55%, from 14.4 to 22.3 m3 PMh−1, with a harvesting intensity of 5,000 brushwood trees per hectare. The respective productivities were 15.3 and 23.4 m3 PMh−1, with a harvesting intensity of 10,000 harvested trees per hectare. Alternatively, when analyzing clearing productivity as in ha PMh−1, the effect of the average brushwood tree volume and number of harvested brushwood trees per hectare were the opposite of harvesting productivity: The larger the average brushwood tree volume and number of harvested trees per hectare were, the lower was the clearing productivity ().
Harvesting cost of brushwood
The volume of brushwood trees and harvesting removal had a major impact on harvesting costs with the one-machine and two-machine configurations. The two-machine configuration had the lowest harvesting costs when the cutting removal was 6,000 brushwood trees per hectare, the volume of harvested brushwood trees was 15–45 dm3, and the forwarding distance was 250 m (). The total harvesting costs at the roadside landing were in the range of €11.30–€18.80 per m3, and of this, the cost of felling-bunching constituted €4.66–€9.40 per m3 and forwarding €6.64–€9.30 per m3. With the harwarder (one-machine configuration), the harvesting costs at the roadside landing were €13.35–€20.10 per m3 respectively ().
Figure 7. The effect of brushwood tree volume (dm3) on the harvesting cost at the roadside landing with the one-machine and two-machine configurations by work phases when the cutting removal is 6,000 brushwood trees per hectare, and the forwarding distance is 250 m
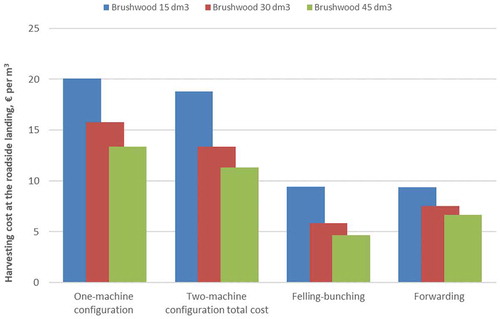
Harvesting costs of brushwood were generally below the average roadside price of whole trees () when the density of cutting removal was 6,000 or 12,000 brushwood trees per hectare, and the forwarding distance was 250 m. In such conditions, harvesting costs exceeded the potential revenues only with the smallest () harvesting removals of brushwood trees (brushwood tree volume <13 dm3). The cost competitiveness of brushwood harvesting changed somewhat when the cost analysis was done by the forwarding distance (). In the cost analysis, the density of cutting removal was 6,000 brushwood trees per hectare, and the volume of harvested brushwood trees was 15 dm3 or 30 dm3.
Figure 8. The effect of brushwood tree volume (dm3) on the harvesting cost at the roadside landing with the one-machine and two-machine configurations when the density of cutting removal is 6,000 or 12,000 brushwood trees per hectare, and the forwarding distance is 250 m. The average market price of whole trees is presented for reference
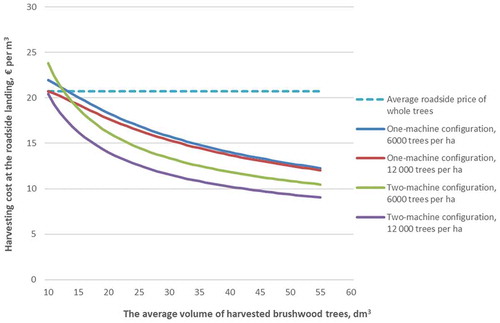
Figure 9. The effect of forwarding distance (m) on the harvesting cost at the roadside landing with the one-machine and two-machine configurations, when the density of cutting removal is 6,000 brushwood trees per hectare, and the volume of harvested brushwood trees is 15 dm3 or 30 dm3
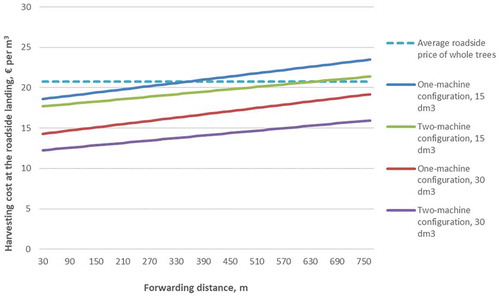
With the harwarder system, the harvesting costs exceeded the potential revenues when the forwarding distance was more than 350 m, and the brushwood tree volume was 15 dm3. With the two-machine configuration, the harvesting costs exceeded the potential revenues when the forwarding distance was more than 630 m (). A brushwood tree volume of 30 dm3 enabled cost-effective harvesting, which compensated for the extended forwarding distance and kept harvesting costs below the average market price of whole trees both with the one- and two-machine configurations when the forwarding distance was in the range of 30–750 m ().
At small harvesting sites, relocation costs play a major role in the total harvesting costs of brushwood (). In , , and , relocation costs are included in the hourly cost of the machinery (), and the harvesting cost at the roadside landing (€ per m3) is thus based on the annual relocation cost and average harvesting site size and structure. In , the effect of the relocation cost on the harvesting cost at the roadside landing is calculated as a function of brushwood tree volume and harvesting site size. The relocation cost of one machine per harvesting site was €174 (), and the size of the harvesting site was 25 m3, 50 m3, and 100 m3 for the one- and two-machine configurations (). Thus the machine relocation cost were in the range of €6.96–€1.74 per m3 for the one-machine configuration and €13.92–€6.96 per m3 for the two-machine configuration respectively. The density of cutting removal was 6,000 brushwood trees per hectare, and the forwarding distance was 250 m. The average volume of harvested brushwood trees was in the range of 10–55 dm3, which means that the length for the 3-m wide harvesting path was 1,389–253 m when the size of the harvesting site was 25 m3 and the brushwood concentration was in the range of 0.018–0.099 m3 per harvesting path m (). For the 50 m3 and 100 m3 harvesting sites, the length of the harvesting path was double or four times that of the smaller sites ().
Figure 10. The effect of harvesting site size (m3) and brushwood tree volume (dm3) on the harvesting cost at the roadside landing with the one-machine and two-machine configurations when the density of cutting removal was 6,000 brushwood trees per hectare, and the forwarding distance was 250 m. The relocation cost per harvesting site was €174
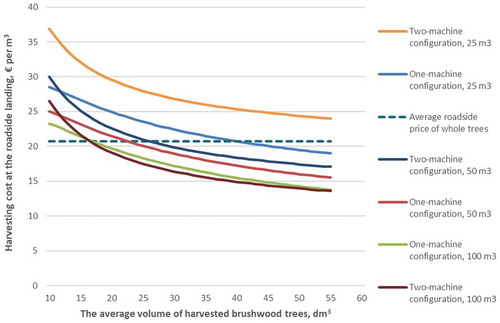
Figure 11. The effect of harvesting site size (m3) and brushwood tree volume (dm3) to the length of harvesting path (m) when the density of cutting removal was 6,000 brushwood trees per hectare
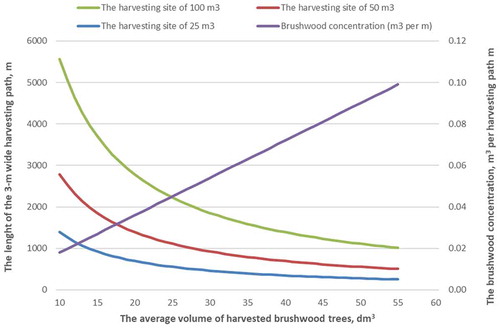
At the smallest harvesting site (25 m3), the harvesting costs always exceeded the potential revenues when harvesting was based on a two-machine configuration (). With a one-machine configuration, the harvesting costs went below the average roadside price of whole trees when the brushwood tree volume was 39 dm3. In such conditions, the length of the harvesting path was 356 m (). At the 50-m3 harvesting site, the harvesting costs went below the average roadside price of whole trees when the brushwood tree volume was 22 dm3 (one-machine configuration) or 25 dm3 (two-machine configuration). In such conditions, the length of the harvesting path was 1,263 m or 1,111 m (). At the 100-m3 harvesting site, the harvesting costs of one- and two-machine configurations were below the average roadside price of whole trees when the brushwood tree volume was 16 dm3. In such conditions, the length of the harvesting path was 3,472 m ().
presents the effect of clearing compensation on the revenue of brushwood harvesting as a function of harvesting removal. The clearing compensation, €0.04 or €0.10 per harvesting path m, was allocated per cubic meter of brushwood cleared, and it was added to the average roadside price of whole trees (). The clearing compensation was €5.57–€1.01 and €2.35–€0.43 per m3 when the volume of brushwood trees was in the range of 10–55 dm3, the density of cutting removal was 6,000 brushwood trees per hectare () and the brushwood concentration was in the range of 0.018–0.099 m3 per harvesting path m . Except for compensation for clearing work, the calculation parameters were otherwise the same as in .
Figure 12. The effect of clearing compensation on the revenue of brushwood harvesting as a function of harvesting removal. The harvesting cost at the roadside landing was calculated as a function of harvesting site size (m3) and brushwood tree volume (dm3). The density of cutting removal was 6,000 brushwood trees per hectare, the forwarding distance was 250 m, and the relocation cost was €174
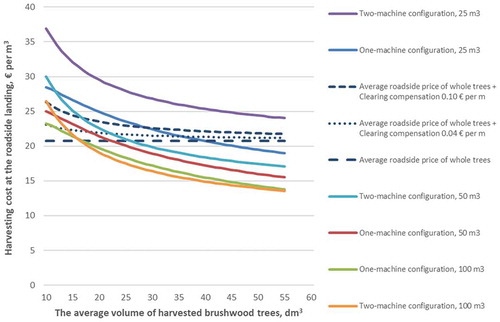
With a two-machine configuration, the harvesting costs exceeded the potential revenues even though clearing compensation was considered when the size of the harvesting site was 25 m3 (). With a one-machine configuration, the break-even point shifted from a brushwood tree volume of 39 dm3 to 36 dm3 when the clearing compensation was €0.04 per m, and to 29 dm3 when the clearing compensation was €0.10 per m (). At the 50-m3 harvesting site, the break-even point shifted from 25 dm3 to 23 dm3 and 17 dm3 when the harvesting was based on a two-machine configuration, and the clearing compensation was €0.04 or €0.10 per m. With a one-machine configuration, the break-even point shifted from 22 dm3 to 19 dm3 and below 10 dm3 respectively (). At the 100-m3 harvesting site, the break-even point of two-machine configuration shifted from 16 dm3 to 14 dm3 and 11 dm3 when the clearing compensation was considered. With a one-machine configuration, the break-even point shifted from a brushwood tree volume of 16 dm3 to 12 dm3 when the clearing compensation was €0.04 per m, and below 10 dm3 when the clearing compensation was €0.10 per m ().
Discussion
The trial was carried out using the first prototype of the Risupeto accumulating felling head, which was attached to the boom tip of the secondhand crawler excavator in the study. The disk saw feller-buncher unit operated without any unnecessary breakdown delays, and the quality of work was good. Brushwood was cleared properly and contaminants, e.g. roots and soil in the harvested brushwood, were excluded. The results of our study are based on relatively limited time study data, which is natural when new devices or methods are being evaluated and tested. The productivity observed during the field study was based on the output of one machine operator and one site and does not therefore represent the full productivity range. Nevertheless, the reported results can be used as a basis for cost calculations and simulations for the integrated clearing and harvesting of roadside and field-edge brushwood.
In this study, a static spreadsheet-based calculation approach was applied, which meant that the normal fluctuation of interactions in felling-bunching and forwarding were not considered. There may be an imbalance between the productivities of feller-bunchers and forwarders in brushwood harvesting, and this may result in increased harvesting costs due to long waiting times for the forwarder. However, a feasible and economic way of operation with such a machine combination would be to cut and finish the working site before the forwarder is relocated to the site. The statistical model does not take random impacts into consideration and therefore yields more optimistic results than a dynamic simulation model. However, systems such as the two-machine configuration, whose individual operations are independent of each other, are more predictable. Overgrown and dilapidated forest roads understandably have little traffic, which reduces the proportion of random interruptions accordingly.
Felling-bunching productivity figures were relatively high compared to the current Nordic harvesting technology in short-rotation forestry (Jylhä and Bergström Citation2016) or harvesting power line corridors for energy (Fernandez-Lacruz et al. Citation2013). In a naturally afforested dense downy birch-dominated stand (Jylhä and Bergström Citation2016), the productivity of whole tree cutting with an average tree volume of approximately 6–56 dm3 was 12–23 m3 per PMh. In the study by Fernandez-Lacruz et al. (2013), the average whole tree volume ranged between 1.4 and 5.3 dm3, and the productivity was 2.6–6.0 m3 per PMh. In both studies, clear cutting was done with a medium-sized harvester, equipped with an accumulating felling head fitted with a circular saw disk. The width of the work path was an average of 20 or 10 m, considerably wider than in this study.
In 2009, a study of brushwood harvesting along forest roadsides (Iwarsson-Wide Citation2009b) was conducted in which the felling-bunching was done with similar machinery as in the studies of Fernandez-Lacruz et al. (2013) and Jylhä and Bergström (Citation2016). At the study site, the width of the work path was 4.5 m, the average height and DBH of trees were 5.5 m and 4 cm, and the cutting removal was 39 tonnes dry matter (oven-dry tonne of forest biomass) and 7,000 trees per hectare. The average felling-bunching productivity was 2.6 tonnes dry matter per PMh or 5.2 m3 per PMh (assuming a basic density of 497 kg per m3), which is lower than the productivity figures in our study.
In the study of Fernandez-Lacruz et al. (Citation2020), the felling-bunching productivity of roadside brushwood was 3.4–10.5 m3 per PMh (assuming a basic density of 497 kg per m3), and it was in line with the results of Iwarsson-Wide (Citation2009b), despite the different cutting technology. The productivity data of Fernandez-Lacruz et al. (Citation2020) were originally from 2008, and the time study was done at a site where the width of the work path was 2.5 m, and the average height of trees was in the range of 3.9–9.5 m in the time study plots. The studied accumulating felling head was equipped with guillotine cutting, and it was attached to a standard harvester during the time studies (Fernandez-Lacruz et al. Citation2020). Compared with conventional small-diameter tree harvesting in early thinnings (Kärhä Citation2006, Citation2011; Laitila and Väätäinen Citation2013; Laitila et al. Citation2016), the observed felling-bunching efficiency in our study was also remarkably higher.
Disk sawblades made of wear-resistant steel seem an appropriate choice for work in tough conditions on forest roads or at field edges with lots of stones or other objects in the soil and vegetation. In addition, the semi-sharp disk sawblades shatter the cutting surface of the stump, which may prevent brushwood coppicing and regrowth. Continuous felling and bunching improved cutting productivity with the Risupeto feller-buncher unit, and because the brushwood trees were relatively short, the cross-cutting of trees into forwarding lengths took place only occasionally in the study. It should be kept in mind that short brushwood lengths may also decrease the forwarding payload size.
Harvested brushwood trees were not delimbed, which increased harvesting removal and reduced the amount of logging slash within the harvesting site. Brushwood harvesting has a negative effect on soil fertility, especially in poor sites, and when leaves are also removed from the site, coppicing and regrowth may be reduced (Unrau et al. Citation2018). The disadvantages of whole tree harvesting are somewhat higher forwarding, chipping, and transportation costs than systems based on delimbed or compacted wood material (Laitila and Väätäinen Citation2012).
Due to the narrow working path, the harvesting removal per meter is rather low, which increases driving distances during loading, as well as forwarding distances when loaded and empty. Forwarding distances can be shortened by increasing the number of roadside storages. However, many small storages along the road increase the covering cost of piles, as well as the costs for relocating machinery during chipping and transportation next to each pile, which can reduce the wood-paying capability of the energy wood procurement company and decrease the roadside price of whole trees.
The size of harvesting site greatly affects the harvesting costs of brushwood, which thus encourages the harvesting of more energy wood from neighboring early thinnings to the same storages at the same visit when harvesting roadside and field-edge brushwood for fuel. The harvesting potential of roadside and field-edge brushwood is relatively low and scattered compared with conventional forestry, and it would therefore be interesting to determine the harvesting productivity and quality with the Risupeto disk saw feller-buncher unit in early thinnings as well. Due to high cutting costs, the production of fuel chips from small-diameter trees originating from early thinnings greatly depends on financial incentives in Finland (Oikari et al. Citation2010; Petty Citation2014), and the only way to decrease the operational costs is to organize the work in a new way or introduce novel machinery. The entrepreneur has made thinnings with the Risupeto disk saw feller-buncher unit in young stands, and practical experiences have been encouraging.
Conclusions
The Risupeto disk saw feller-buncher unit in this study was capable of cutting and accumulating trees with a continuous movement that enabled the efficient harvesting of roadside and field-edge brushwood for fuel. From the harvesting entrepreneur’s perspective, the economic result in the brushwood harvesting with one- and two-machine configurations was strongly affected by the average volume of the brushwood trees, as well as on-site harvesting removal. Correspondingly, the roadside price of whole trees had a great impact on potential brushwood-harvesting revenue. The clearing compensation per volume of harvested brushwood (m3) was rather nominal in this case study, and it affected the economic result mainly in the smaller brushwood tree volume categories, where the harvesting removal was low. From the forest road owner’s or farmer’s perspective, it is crucial that the overgrowth brushwood trees have value as a fuel instead of waste that may just be cleared from edge zones. Based on the clearing productivities presented in , it can be stated that the clearing cost of overgrowth brushwood trees can be remarkable, and significantly higher than an operating model in which edge-zone brushwood is cleared regularly as part of normal field or forest road maintenance.
Acknowledgements
The authors gratefully acknowledge financial support from the research consortium project FORBIO (decision no. 293380) funded by the Strategic Research Council of the Academy of Finland.
Disclosure statement
No potential conflict of interest was reported by the authors.
References
- Ackerman P, Belbo H, Eliasson L, de Jong A, Lazdins A, Lyons J. 2014. The COST model for calculation of forest operations costs. Int J For Eng. 25:75–81. doi:10.1080/14942119.2014.903711
- Belbo H. 2010. Comparison of two working methods for small tree harvesting with a multi tree felling head mounted on farm tractor. Silva Fenn. 44(3):453–464. doi:10.14214/sf.142.
- Belbo H. 2011. Efficiency of accumulating felling heads and harvesting heads in mechanized thinning of small diameter trees. Linnaeus University Dissertations No. 66/2011. 42 p.
- Bergström D. 2009 2009. Techniques and systems for boom-corridor thinning in young dense forests. Acta Universitatis Agriculturae Sueciae. 87:53.
- Ebenhard T, Forsberg M, Lind T, Nilsson D, Andersson R, Emanuelsson U, Eriksson L, Hultåker O, Iwarsson Wide M, Ståhl G. 2017. Environmental effects of brushwood harvesting for bioenergy. For Ecol Manage. 383:85–98. doi:10.1016/j.foreco.2016.05.022.
- Erber G, Holzleitner F, Kastner M, Stampfer K. 2016. Effect of multi-tree handling and tree-size on harvester performance in small-diameter hardwood thinnings. Silva Fenn. 50(1):1–17. doi:10.14214/sf.1428.
- Erber G, Kühmaier M. 2017. Research trends in European forest fuel supply chains: a review of the last ten years (2007–2017) – part one: harvesting and storage. Croatian J For Eng. 38(2):269–278.
- Fernandez-Lacruz R, Di Fulvio F, Bergström D. 2013. Productivity and profitability of harvesting power line corridors for bioenergy. Silva Fennica 47(1) article id 904). 23 p
- Fernandez-Lacruz R 2019. Improving supply chains for logging residues and small-diameter trees in Sweden [Ph.D. Thesis]. Umeå (Sweden): Department of Forest Biomaterials and Technology, Swedish University of Agricultural Sciences. Doctoral thesis no. 2019:44. https://pub.epsilon.slu.se/16161/
- Fernandez-Lacruz R, Edlund M, Bergström D, Lindroos O. 2020. Productivity and profitability of harvesting overgrown roadside verges – a Swedish case study. Int J For Eng. doi:10.1080/14942119.2020.1822664
- Harstela P. 1991. Work studies in forestry. Silva Carelica. 18: 41 p. University of Joensuu.
- IUFRO WP 3.04.02. 1995. Forest work study nomenclature. Test edition valid 1995–2000. Department of Operational Efficiency, Swedish University of Agricultural Sciences. 16.Garpenberg, Sweden.
- Iwarsson-Wide M 2009a. Teknik och metod Ponsse EH25. Trädbränsleuttag med Ponsse EH25 i kraftledningsgata [Technique and method Ponsse EH25.Wood fuel recovery with Ponsse EH25 in power line corridor]. Arbetsrapport från Skogforsk nr 681–2009. 14 p. In Swedish.
- Iwarsson-Wide M 2009b. Skogsbränsleuttag i vägkanter. Prestationsstudie – uttag av skogsbränsle i vägkant med Bracke C16 [Recovery of forest fuel along forest road. Productivity study – recovery of forest fuel along forest road with Bracke C16]. Arbetsrapport från Skogforsk nr 695–2009. 15 p. In Swedish.
- Iwarsson-Wide M 2009c. Skogsbränsleuttag i vägkanter – prestationsstudie. Uttag av skogsbränsle i vägkant med Ponsse Dual med EH25 [Recovery of forest fuel along forest road. Productivity study – recovery of forest fuel along forest road with Ponsse Dual EH25]. Arbetsrapport från Skogforsk nr 696–2009. 16 p. In Swedish.
- Iwarsson-Wide M. 2011. Forest fuel harvest from roadsides. In publication. Thorsén Å, Björheden R, Eliasson L, editors. Efficient forest fuel supply systems. Composite report from a four-year R&D programme 2007-–2010.Uppsala, Skogforsk. ISBN: 978-91977649-4-0 65–67.
- Jylhä P. 2011. Harvesting undelimbed Scots pine (Pinus sylvestris L.) from first thinnings for integrated production of kraft pulp and energy. Dissertationes Forestales. 133:73. doi:10.14214/df.133
- Jylhä P, Bergström D. 2016. Productivity of harvesting dense birch stands for bioenergy. Biomass Bioenergy. 88:142–151. doi:10.1016/j.biombioe.2016.03.016.
- Kaakkurivaara T. 2018. Innovative methods for measuring and improving the bearing capacity of forest roads. Dissertationes Forestales. 251:57. doi:10.14214/df.251
- Kärhä K. 2006. Whole-tree harvesting in young stands in Finland. For Stud. 45:118–134.
- Kärhä K. 2011. Integrated harvesting of energy wood and pulpwood in first thinnings using the two-pile cutting method. Biomass Bioenergy. 35(8):3397–3403. doi:10.1016/j.biombioe.2010.10.029.
- Kärhä K, Poikela A, Palander T. 2018. Productivity and costs of harwarder systems in industrial roundwood thinnings. Croatian J For Eng. 39(1):23–33.
- Kiss K, Malinen J, Tokola T. 2015. Forest road quality control using ALS data. Can J For Res. 45(11):1636–1642. doi:10.1139/cjfr-2015-0067.
- Korpilahti A 2008. Metsäteiden kuntoinventoinnin ja kuntotiedon hyödyntämisen toimintamalli [Condition inventory of forest roads and operating model for the utilization of collected information]. Metsätehon raportti 202, 2.12. 2008. 60 p.
- Laitila J. 2008. Harvesting technology and the cost of fuel chips from early thinnings. Silva Fenn. 42(2):267–283. doi:10.14214/sf.256.
- Laitila J. 2012. Methodology for choice of harvesting system for energy wood from early thinning. Dissertationes Forestales. 143:103 p. doi:10.14214/df.143
- Laitila J, Asikainen A. 2006. Energy wood logging from early thinnings by harwarder method. Balt For. 12(1):94–102.
- Laitila J, Asikainen A, Nuutinen Y. 2007. Forwarding of whole trees after manual and mechanized felling bunching in pre-commercial thinnings. Int J For Eng. 18(2):29–39. doi:10.1080/14942119.2007.10702548.
- Laitila J, Niemistö P, Väätäinen K. 2016. Productivity of multi-tree cutting in thinnings and clear cuttings of young downy birch (Betula pubescens) dominated stands in the integrated harvesting of pulpwood and energy wood. Balt For. 22(1):116–131.
- Laitila J, Väätäinen K. 2012. Truck transportation and chipping productivity of whole trees and delimbed energy wood in Finland. Croatian J For Eng. 33(2):199–210.
- Laitila J, Väätäinen K. 2013. The cutting productivity of the excavator-based harvester in integrated harvesting of pulpwood and energy wood. Balt For. 19(2):289–300.
- Laitila J, Väätäinen K. 2020. Productivity of harvesting and clearing of brushwood alongside forest roads. Silva Fenn. 54(5):21. article id 10379. doi:10.14214/sf.10379.
- Lindblad J, Äijälä O, Koistinen A. 2010. Energiapuun mittaus (27.9.2010) [Measurement of energy wood]. p. 31. In Finnish
- Magagnotti N, Kanzian C, Schulmeyer F, Spinelli R. 2013. A new guide for work studies in forestry. Int J For Eng. 24(3):249–253. doi:10.1080/14942119.2013.856613.
- Malinen J, Laitila J, Väätäinen K, Viitamäki K. 2016. Variation in age, annual usage and resale price of cut-to-length machinery in different regions of Europe. Int J For Eng. 27(2):95–102. doi:10.1080/14942119.2016.1171964.
- Ministry of Agriculture and Forestry. 2010. Maa- ja metsätalousministeriön asetus kuormainvaa’an käytöstä puutavaran mittauksessa ja erien erillään pidosta annetun maa- ja metsätalousministeriön asetuksen muuttamisesta [Statute of the Ministry of Agriculture and Forestry for the use of loader-mounted scales in the weighing of timber lots]. (Dnro 666/14/2010, Nro 8/10). 8 p.
- Oikari M, Kärhä K, Palander T, Pajuoja H, Ovaskainen H. 2010. Analyzing the views of wood harvesting professionals related to the approaches for increasing the cost-efficiency of wood harvesting from young stands. Silva Fenn. 44(3):481–495. doi:10.14214/sf.144.
- Palva R. 2019. Konetyön kustannukset ja tilastolliset urakointihinnat. [The cost of machine work and the statistics of contractor prices]. Rajamäki. Vol. 447, TTS:n julkaisuja. p. 16.
- Petty A. 2014. Opportunities for cost mitigation and efficiency improvements through rationalization of small-diameter energy wood supply chains. Dissertationes Forestales. 175:47. doi:10.14214/df.175
- Repola J, Ojansuu R, Kukkola M. 2007. Biomass function for Scots pine, Norway spruce and birch in Finland. Working Papers of the Finnish Forest Research Institute 53. 28 p
- Röser D. 2012. Operational efficiency of forest energy supply chains in different operational environments. Dissertationes Forestales. 146:83. doi:10.14214/df.146
- Rottensteiner C, Affenzeller G, Stampfer K. 2008. Evaluation of the feller-buncher Moipu 400E for energy wood harvesting. Croatian J For Eng. 29(2):117–128.
- Schweier J, Spinelli R, Magagnotti N, Becker G. 2015. Mechanized coppice harvesting with new smallscale feller-bunchers: results from harvesting trials with newly manufactured felling heads in Italy. Biomass Bioenergy. 72(2015):85–94. doi:10.1016/j.biombioe.2014.11.013.
- Spinelli R, Magagnotti N, Schweier J. 2017. Trends and perspectives in coppice harvesting. Croatian J For Eng. 38(2):219–230.
- Unrau A, Becker G, Spinelli R, Lazdina D, Magagnotti N, Nicolescu VN, Buckley P, Bartlett D, Kofman PD (Eds.) 2018. Coppice forests in Europe. Freiburg i. Br. (Germany): Albert Ludwig University of Freiburg. 392 p. https://www.eurocoppice.uni-freiburg.de/intern/coppiceineurope-volume/coppice-forests-in-europe-2018-09-10-final-small.pdf
- Volumes and prices in energywood trade. 2020. Natural Resources Institute Finland. Helsinki. https://stat.luke.fi/en/volumes-and-prices-energy-wood-trade
- Windisch J. 2015. Process redesign in development of forest biomass supply for energy. Dissertationes Forestales. 189:55. doi:10.14214/df.189