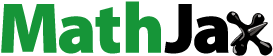
ABSTRACT
Wire rope used in cable logging, where a series of cables facilitate the extraction of timber on steep terrain, experiences high tensions that must be managed to ensure safety. Innovations change practices over time and double-hitch carriages, which allow trees to be extracted horizontally, are a recent example. This makes it feasible to harvest across terrain with limited deflection, increases the recovery of biomass, and potentially reduces high-dynamic load events associated with ground contact. In this study, a standard single-hitch carriage was compared against a new double-hitch carriage under controlled conditions. Tension was measured continuously and specific elements, such as tension when the carriage was at midspan, maximum tension at breakout and inhaul, and magnitude of cyclic tension during inhaul were identified, measured and compared against payload. While payload was similar in the treatments, the additional weight of the double-hitch carriage resulted in higher skyline tensions. In both treatments, payload and skyline tension when the carriage was at midspan were strongly correlated. Cyclic tension was reduced by the double-hitch carriage system. While a number of unique high-dynamic loads were identified that were not part of the normal extraction, they represented only 6% of the cycles and the maximum tension was similar to that experienced during break-out and inhaul. This study increased the understanding of skyline tension during logging operations and the effect of carriage type. It showed that, though tension often exceeds the safe working load of the cable, it does not exceed the endurance limit for a well-designed and operated system.
KEYWORDS:
Introduction
The need to balance cost-effective wood production with careful environmental protection and safety makes alpine forestry particularly complex (Aggestam et al. Citation2020). Continuous-cover forestry is popular as it mitigates hydro-geological risks, while still allowing for the extraction of revenue. However, continuous-cover forestry results in low harvest volumes that reduce operational profitability (Spinelli et al. Citation2015). Furthermore, the access constraints of a rugged mountain environment represent severe hurdles to mechanization, which is the main solution to controlling harvesting cost despite increasing fuel price and labor wages. While full mechanization may not be feasible, modernization of cable logging practices can still offer significant benefits (Bont and Heinimann Citation2012; Wassermann Citation2018).
Loggers in the European Alps have increasingly moved away from motor-manual delimbing and crosscutting at the stump site due to labor shortages and the need for improved work safety. Mechanized processing can reduce the total harvesting cost by 30%, so that stationing a processor at the yarder landing has become common practice (Spinelli et al. Citation2008). A number of yarder manufacturers offer mobile yarder models that integrate a boom and a processor so that the operation takes less space and becomes more economical to purchase and relocate compared with a standard two machine operation – i.e. yarder + stand-alone processor (Stampfer et al. Citation2006). Processing trees at the landing does not only offer the financial and safety benefits of mechanized work but also generates additional revenue in the form of forest biomass (Valente et al. Citation2011), which can be delivered to a well-developed biomass market with a growing number of energy conversion plants located in many alpine settlements.
The system of mechanized timber processing and forest biomass recovery from yarding sites is well established; trees are processed at the landing where tops and branches accumulate, ready for recovery as energy wood. However, tree–length material is cumbersome for extraction with cable yarders in selection cuts and its extraction is only viable at relatively short distances (300-500 m). Therefore, the benefits of mechanization and biomass production are currently restricted to forest areas with a well-developed forest road network, and conversely unavailable in alpine forests not served by a suitably dense road network (Mologni et al. Citation2016). Such forests are normally harvested with long-distance cableways, which can span over 1 or 2 km down to the nearest valley road. These systems are typically rigged in a shotgun configuration (gravity return) and best suited to the extraction of short logs processed in the forest, unless sufficient deflection can be guaranteed all along the line (Samset Citation1985).
A number of tower yarder manufacturers have recently started exploring long-distance extraction solutions, eventually developing new tower yarder models capable of spanning up to 1.5 km. These machines are configured for a three-cable installation because they are too large and cumbersome for moving uphill, or lack access roads to the ridges, to allow for the two-cable gravity return system. In turn, the three-cable configuration makes it possible to pull a load, even when full clearance is not achieved, thus solving the issues of tree-length harvesting. Increased extraction distance makes tree-length extraction critical again and is best offset by increasing carriage speed.
A tree-length load under a fast carriage may cause excessive strain of the cable set up and result in an accident if the load hits one of the standing trees at the sides of the yarding corridor. Hence, the idea of lifting trees horizontally under the carriage, suspended from two points has developed. This solution would make tree-length extraction viable at long distances regardless of yarder configuration and therefore a general technique for universal use. Double-hitch suspension requires a “double carriage,” composed of two separate elements working in tandem, each with its own lift line. Such carriages are already used in civil engineering for installing pipelines or other cumbersome structures in rugged terrain. However, the construction industry has different technical specifications compared with forestry and therefore the equipment used in that industry is typically too heavy and expensive for deployment in forestry. Double-hitch full-suspension technology has appeared only recently in forest operations, initially as a makeshift solution improvised by loggers in the field, and later as a commercial product.
A number of loggers have been using the new carriages successfully for some years in Austria, Germany, Italy and Switzerland. However, the definition of “successful” for a commercial logging company tends to focus on productivity, cost and reliability. The question remains about whether any of the predicted benefits of skyline tension and anchor stability have actually materialized. Loggers are not normally equipped with the precision instruments needed for measuring and monitoring those aspects, and to our knowledge no one equipped with these instruments has yet tackled the issue. Therefore, authors from many stakeholder groups gathered in a coordinated team and initiated a study with the general objective of determining the effect of double-hitch horizontal full-suspension yarding on skyline tension and high-dynamic loading. High-dynamic loading is a sudden peak in tension that is not part of the normal extraction cycle (Harrill Citation2014) and increases the risk of progressive failure (OR-OSHA Citation2010).
A controlled-study was carried out under the typical conditions of the forest in the Italian Alps with the specific goals of: 1) determining if the skyline tension, and high-dynamic loading events differed significantly when the same yarder set up was equipped with a double-hitch full-suspension carriage and a standard single-hitch carriage and 2) if compliance with all safety parameters differed significantly between the two techniques, for the same payload and conditions.
Materials and methods
Materials
The study was conducted in a mixed fir-spruce (Abies alba L. and Picea abies Karst.) stand in the Eastern Italian Alps, near Forni Avoltri in the province of Udine. The stand grew over a neutric cambisol soil on a south-west face and was divided in two separate belts: at the bottom of the slope and nearer to the forest road, the stand originated from the reforestation of an old pasture, carried out in the late 1950s, after farming was discontinued; further uphill and all the way to the top, the forest originated from natural regeneration and was about 100 years old. At the time of the study, the forest was being salvaged after the windthrow event in October 2018 that caused the loss of over 8 million m3 across much of North-eastern Italy (Motta et al. Citation2018).
Chainsaw operators separated windthrown trees from their root plates and crosscut the stems whenever needed for disentangling overlapping trees. Trees and tree sections were yarded downhill to the main forest road, where the yarder was installed. Once on the forest road, trees and tree sections were delimbed and cut to length using an excavator-based processor.
The yarder was a Valentini V600/M3/1000 trailer-mounted tower model, which is common with Alpine loggers in Austria, Germany and Italy with over 50 units sold. The machine had a maximum skyline capacity of 1000 m (22 mm cable) and was equipped with three hydraulically powered working drums, for the skyline, mainline and haulback line (22 mm, 11 mm and 11 mm, respectively). The mainline and haulback drums contained 1100 m and 2000 m of cable, respectively, and were fitted with a hydraulic interlock. Additional drums were available for the strawline and the guylines. The tower could telescope up to 12.5 m and, during the study, was fully extended. The machine was fitted with its own 175 kW diesel engine. All cables were wire rope core, swaged, ordinary lay. Skyline pre-tension was set between 100 kN and 130 kN depending on work conditions.
The tailhold was a large sound spruce tree, part of a solid clump of four healthy individuals. The rigging was a classic three-cable configuration, with a standing skyline, a mainline and a haulback line. For the purpose of the study, the yarder was run alternately with two separate carriage set-ups: conventional clamped single-hitch carriage (henceforth: single-hitch), set for partial suspension, and unclamped motorized double-hitch dropline carriage (henceforth: double-hitch), set for full load suspension by attaching the load at two points and keeping it horizontal.
The single carriage was a 3-t capacity Hochleitner BW4000, weighing 760 kg. The carriage was clamped at the loading site through a hydraulic clamp and the haulback line was used for slack-pulling. Loads were hooked to the mainline by one end and were carried semi-suspended or dangling from the carriage when contact with the slope profile was interrupted ().
The double carriage was the combination of a SEIK Skybull SFM 20/40 motorized dropline (37 kW) carriage and a dedicated SEIK NL20 extension. Both the carriage and the extension carried a 2-t capacity winch, powered by the diesel engine of the Skybull 20/40 through a hydraulic transmission. Loads could be attached at two points and lifted horizontally, achieving full suspension under all conditions, with a lower load oscillation during transport (). Total weight was 1000 kg, including fuel and dropline cables. During loading, the SEIK carriage combination was held in position by the mainline and the haulback line.
The study consisted of 74 and 75 complete cycles for the single-hitch and the double-hitch treatments, respectively. However, eight of the double-hitch cycles were excluded from the study because they were used for partial suspension only, thus violating the specifications set in the study protocol. Loads were extracted with the same setup, along the same corridor and at the same pre-defined stops for both carriages, in order to guarantee even test conditions. As a matter of fact, the only thing changed for the comparison was the carriage, with the two carriages being swapped at daily intervals. All extraction proceeded downhill. Total skyline length (tower tip to tailhold block) was 366 m. The horizontal distance to the tailhold was 328 m and the vertical distance was 140 m. An intermediate support was installed at a distance of 199 meters from the tower in order to guarantee sufficient ground clearance along the length of the corridor.
The harvesting system was manned by three operators: two at the loading site (choker setters) and one at the unloading site. The latter sat inside the cab of a processor that cut the incoming trees and tree sections into commercial assortments. The machine was a 21-t Liebherr 904 excavator fitted with a Konrad Woody 60 H harvesting head. The use of radio-controlled chokers allowed the processor operator to release the load without dismounting from the machine. Both the processor operator at the unloading site by the yarder and the choker setters at the loading site in the forest could operate the yarder using a remote control, and they did so when the carriage was in their respective work areas. The remote controls were mutually exclusive, so that one operator could not interfere with the carriage movements when the carriage was outside his own defined work zone. All operators were experienced and possessed the proper formal qualifications (under the regional certification scheme).
The test was conducted in September 2019, and lasted a total of 23 productive machine hours (PMH), or 26 scheduled machine hours (SMH). During the test, the yarder extracted 233 m3 of timber (over bark) or about 200 t of total biomass (timber + chips).
Methods
The study method aimed at determining, on a cycle basis: extraction distance, load size, time consumption, skyline tension, and dynamic loading. Two aspects of dynamic loading were measured, the scale of the normal dynamic oscillations as part of the inhaul phase, as well as any “high-dynamic load” to the system. High-dynamic loads represent an impact on the system that results in a sudden tension peak. This is especially harmful to cable integrity due to its magnitude and to its very sudden occurrence, which can generate torsion, internal friction in the cables and overheating of the component steel (Leech and OveringtonCitation1982).
The distance between the tower and the loading point (carriage stop on the skyline) was determined using a Bushnell Yardage Pro 500 laser range finder. The terrain profile under the line was determined from the Digital Terrain Model available for the area, with a resolution of 2 m. The locations of all the elements of the cable line were surveyed by a Garmin GPSmap 62 CSx hand-held GPS device, with an approximate accuracy of 4 m (Morgenroth and Visser Citation2013).
Load size was obtained by scaling every single log produced from each turn, using a caliper and a measuring tape. Diameter was taken at mid-length. The species of each log was identified and recorded. Two researchers were assigned to perform this job to avoid interference in the operation. Volume measurements were converted into weight measurements after determining the actual density of the two species. For this purpose, 10 logs per species were scaled and then weighed using a 9.8 kN capacity HKM HT series load cell, accurate to ± 9.8 N. The weight of the branch material was estimated by visually attributing a branch loading index to each tree or tree section as follows: a score between 0 and 4 was attributed based on the total length of the stem covered with branches (0 = no branches; 1 = branches observed on one quarter of the total length; 2 = branches observed on half of the total length, etc.). Then, an additional score between 0 and 4 was attributed based on the proportion of the total circumference covered with branches, according to the same principle. The factorial combinations of the two weights yielded the following possible scores: 0, 1, 2, 3, 4, 6, 8, 9, 12, 16. The results from all observations were analyzed and the mode was extracted, which was attributed to the baseline Biomass Expansion Factor (BEF) reported in a bibliography for windthrown spruce in the Eastern Italian Alps. This is equal to 110 kg of fresh biomass per m3 of commercial timber volume (Spinelli et al. Citation2006). This baseline value was then corrected by the ratio between the actual combination score for each tree or tree section and the baseline weight. The individual weights for the timber and the biomass components of each piece in a load were summed into the total load weight.
Time was recorded with the time-and-motion technique, separated by the following tasks: unloaded carriage trip (outhaul); lowering the dropline; connecting the chokers to the load; breaking out the load and dragging it under the skyline; lifting the load under the carriage; travel loaded (inhaul); unloading; downtime – split into mechanical, operational and personnel delays (Magagnotti et al. Citation2013). The time study was used to reconcile tension data with specific cycle and work element information, thus providing references for identification of outhaul, breakout and inhaul.
Tension was recorded at 100 Hz through a 200 kN-capacity Honigmann Cablebull tension meter. The tension meter was mounted on the skyline near the tailhold, in the upper segment of the cable corridor. Tension data were downloaded onto a laptop using the dedicated HCC-Easy software. A researcher was stationed by the laptop to check that data collection proceeded undisturbed. The tension meter was re-calibrated four times a day during short interruptions of the work routine (beginning of work, middle of the morning, lunch break and middle of the afternoon).
Monitoring provided a near continuous record (at 100 measures per second) of skyline tension as measured near the tailhold. The data were separated out into cycles and plotted on charts. Measurements (all in kN) of the following parameters were obtained from the chart for each cycle and used for further analysis:
SPT = skyline pre-tension, being the tension in the skyline before the unloaded carriage moves away from the yarder;
PTBR = peak tension during breakout; which is important as the overall skyline tension in nearly all cases is highest either during breaking out, or when the loaded carriage passes through midspan.
PTMS = peak tension midspan, being the highest tension recorded as the loaded carriage passed through midspan;
PT = peak tension, which is the greater of PTBR or PTMS;
TMS = tension midspan, being the average tension as the loaded carriage passes through midspan; This excludes the dynamic loading and is important; as in theory, it should relate most closely to any calculated tension.
MCLA = greatest peak to trough change in skyline tension during any part of the cycle, being the largest cyclic load experienced during the cycle;
AHDL = absolute peak value of the high-dynamic load, if any, was recorded;
MHDL = magnitude of the high-dynamic load, being the difference between the high-dynamic load tension and tension just prior to the event;
Tension increase (TI) and tension increase factor (TI Factor) were calculated as follows in EquationEquations 1(1)
(1) and Equation2
(2)
(2) (Pyles et al. Citation1994):
For the purpose of this study, a high-dynamic loading event was only recorded when it was clearly not associated with normal breaking out of the load or the inhaul phase of the cycle (where PT was measured). To qualify as a high-dynamic loading event, tension had to increase by at least 10 kN within a period of no longer than 2 seconds and the event had to be identifiable as unique in that it was followed by a drop in tension (Harrill Citation2014). Three examples of high-dynamic loading, as measured during the study, are shown in . Since no continuous video recording was taken, it is not possible to categorize the exact nature of each event. However, known examples of recorded high-dynamic loads include loads hung up on a stump or tree, or the release of the carriage clamp under a load (OR-OSHA Citation2010). MHDL was measured in the skyline at the tailhold, so it will be lower than the actual load in the cable in the immediate vicinity of the cause.
Figure 2. Examples of high-dynamic loading events. These can occur when loads get hung up on a stump or tree during inhaul or during the release of the carriage clamp under a load. Time on the x-axis refers to the duration of the respective yarding cycle, with the origin equaling the beginning of the cycle
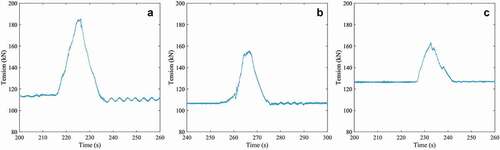
Figure 3. Example of observed tension during a complete yarding cycle, in which high-dynamic loading occurred, with selected work tasks (outhaul, breakout and inhaul) and waypoints (midspan, support) annotated. During phases in which the carriage was not moved (between outhaul and inhaul), e.g. lowering the dropline, connecting the chokers to the load, unloading or re-positioning of chokers after the high-dynamic load, tension varied only marginally. At the end of the cycle, tension dropped back to pre-tension level
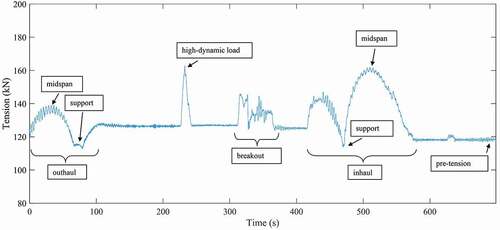
All values were matched against the safe working load (SWL), which was calculated to be 141 kN by using a factor of safety of 3 from the published breaking load for the skyline (i.e. 424 kN divided by 3).
Data were extracted from the tension records of each cycle using a specifically designed R-script (R Core Team Citation2018). Results were then checked visually on each single graph to make sure that no unexpected occurrences had tripped the automatic system in error (). If any inconsistencies were detected, the data and the respective time stamps were verified again to resolve any doubts. This further visual check allowed confirming which cycles had actually passed the midspan. These would be expected to show a typical parabolic tension graph as the loading increases, then decreases, as the carriage passed through midspan.
Once checked and adjusted when required, data were analyzed statistically using the Statview software (SAS Institute Inc Citation1999). Descriptive statistics were obtained separately for each treatment. The individual work cycle (turn) was selected as the observational unit. The significance of the differences between mean values for the two treatments was tested with non-parametric techniques, which are robust against violations of the statistical assumptions (normality, homoscedasticity, data imbalance, etc.). Multiple linear regression analysis allowed testing the relationship between selected dependent variables (e.g. TMS and MCLA) and potentially meaningful independent variables (e.g. load size and distance from the tower). The effect of treatment was introduced as an indicator (dummy) variable (Olsen et al. Citation1998). Differences in the frequency of occurrences (e.g. high-dynamic load events and MCLA peaks other than at midspan) were tested using Chi-square analysis. Compliance with the statistical assumptions was checked through the analysis of the residuals, which excluded serial correlation potentially deriving from gross measurement errors. In all analyses, the elected significance level was α < 0.05.
Results
The mean extraction distance did not differ significantly between treatments and was 183 m and 184 m for the double-hitch and the single-hitch treatments, respectively. However, the number of trips passing over the support and over midspan was significantly different between treatments, as confirmed by the Chi-square analysis (). For this reason, the TMS was calculated only on the cycles that passed midspan. Mean load size was 8% larger for the double-hitch treatment (1,328 kg vs. 1,226 kg), but this difference was not statistically significant. However, once the weight of the carriage was factored in, the mean total weight on the skyline increased to 2,294 kg and 1,986 kg for the double-hitch and the single-hitch treatments, respectively. As a result, the difference rose to 15% and became statistically significant. The maximum recorded payload was 3,073 kg and 2,820 kg for the double-hitch and the single-hitch treatment, respectively (or 4,073 kg and 3,580 kg including carriage weight).
Table 1. Results of the Chi-square analysis for the frequency of events
TMS was 150 kN and 129 kN for the double-hitch and the single-hitch treatments, respectively (). Therefore, the double-hitch treatment exceeded SWL by 6%, while the single-hitch was well within it. PTMS was not much higher than TMS, and the single-hitch treatment still remained within SWL, although barely. However, the maximum values for PTMS exceeded SWL by 29% and 16% for the double-hitch and the single-hitch treatments, respectively. MCLA at midspan was more than twice as large for the single-hitch treatment than for the double-hitch treatment. Even when recorded outside midspan, MCLA was larger for the single-hitch treatment, although not as much as when at midspan (58% larger). These values account for MCLA values recorded outside midspan that 1) occurred in those cycles that did pass through midspan and 2) were greater than the MCLA measured at midspan. They were calculated and reported because they were taken to represent sudden swings in the load possibly caused by contact with the terrain.
Table 2. Skyline tension when carriage was at midspan
Regression analysis indicated that TMS increased linearly with SPT and payload size () and was 12.8 kN higher for the double-hitch treatment (). The estimated model could explain over 80% of the total variability in the dataset. A similar model was developed for PTMS, which used the same variables and was only slightly less accurate. Regression analysis also confirmed the relationship between MCLA (at midspan and outside midspan), load size, and carriage treatment, but in this case, the independent variable was negatively correlated with the double-hitch treatment. The explanatory power of the MCLA regressions was relatively low (30% to 47% of the total variability), but all terms were highly significant and the relationships seemed logical. Although the MCLA models may be weak predictors, they still offer a good description of a phenomenon that is also affected by other variables not included in the survey.
Table 3. Regression equations for predicting skyline tension when the carriage was at midspan and MCLA
Figure 4. Point scatter and regression graph for mean tension of the skyline when the carriage is at midspan (TMS). The graphs were calculated using the equation in , for the mean pre-tension of 109 kN for the double-hitch carriage and 103 kN for the single-hitch carriage
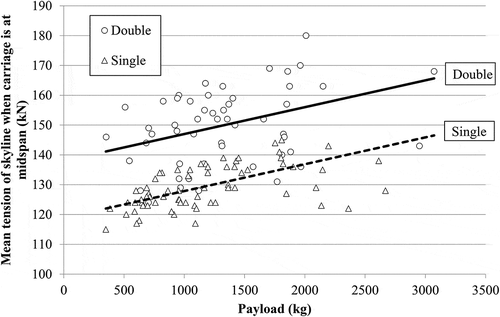
Chi-square analysis confirmed that high-dynamic load events were four times more frequent with the double-hitch treatment than with the single-hitch treatment, although they were very rare occurrences anyway (10% and 2.5% of the cycles, respectively). Although less frequent, high-dynamic loads under the single-hitch treatment showed an 8% higher PT and twice the amplitude than they did under the double-hitch treatment (). Furthermore, the highest dynamic loads exceeded SWL by 19% and by 30%, under the double-hitch and the single-hitch treatments, respectively.
Table 4. Pre-tension, high-dynamic load, and maximum tension during breakout
The tension figures recorded for the few high-dynamic loading events were very close to those recorded for PTBR, except that the latter occurred regularly in each cycle. In particular, PTBR was 4% higher for the double-hitch treatment (146 kN vs. 141 kN) in comparison to the single-hitch treatment. However, a 5% lower TI was incurred in the case of the former, given the higher SPT. The maximum values recorded for PTBR exceeded SWL by approximately 30%, with negligible differences between treatments.
As depicted by the examples A to C in , all high-dynamic loading events were quite similar in nature: they took about 3 to 4 seconds to build in a very linear manner, stayed at the highest loading level for a short time (approximately one second), and took a longer time to dissipate afterward. Since the loading level returned to the same value as observed before the occurrence, the skyline obviously did not slip. Therefore, the short stay at the highest loading level must be an artifact of the mainline first becoming overloaded and then being released.
Discussion
The study met its original goals of determining the differences between double-hitch horizontal yarding and conventional single-hitch yarding in terms of dynamic skyline stress and compliance with safety standards. In contrast, the study did not determine whether double-hitch yarding offers any specific advantages over long distances, given that the experimental setup covered a relatively short distance. However, that was necessary in order to limit the number of intermediate supports and facilitate tension monitoring, so that the primary objective of this study – determining skyline tension effects – could be best met.
As expected, the heavier double-hitch suspension carriage required a higher SPT to reach the same ground clearance. These two factors combined in a significant increase of the TMS compared with the conventional single-hitch carriage setup, even if payload size was not significantly larger. At the same time, reduced load swinging did result in a dramatic abatement of cyclic stress – also an expected outcome. MCLA at midspan was less than half as large for the double-hitch treatment compared with the single-hitch treatment, which also explained the apparent contradiction of a higher frequency of maximum amplitude events recorded at positions different from midspan for the double-hitch treatment. Minor tension spikes that would not have qualified for recording under the single-hitch treatment because they were below the amplitude measured at midspan, did so under the double-hitch treatment because the reference baseline recorded at midspan was much smaller. Though more frequent, non-midspan MCLA events recorded for the double-hitch treatment were still one third smaller than the fewer similar events recorded for the single-hitch treatment. In particular, most of these events occurred within approximately 50 m from the landing, and were likely related to a drop in the terrain profile where loads would suddenly swing from partial-suspension to full-suspension mode (Jorgensen et al. Citation1978). Ideally, that was not supposed to occur with the double-hitch treatment, where the load should have been fully suspended. However, even under this treatment, minor load components (tops or small trees) were occasionally left hanging from one end, even if the main load was fastened at two points. Therefore, it was possible that a minor component of the load did drag on the ground even under the double-hitch treatment and then would swing out when passing over a step in the terrain profile. In that case, the small weight of the swinging component and the general better stability of the tightly fastened main load would combine in restraining cyclic load, which is what was observed in the data.
The study provided knowledge about the frequency and magnitude of high-dynamic loads, which is a well-known concern in cable logging but with almost no factual data published. The very high recording frequency (100 Hz) made sure that all events would be adequately captured, since high-dynamic loads in a skyline can occur within 0.2 s (Visser Citation1998; Harrill Citation2014). Older studies suggest that even a lower resolution of 0.5 s could be adequate for capturing high-dynamic loads (Jorgensen et al. Citation1978; Pyles et al. Citation1994), which might be correct for identifying a high-dynamic loading event, but not for capturing the magnitude of the actual maximum skyline tension of the high-dynamic loading event.
Under the conditions covered in this study, being a well-managed standing skyline setup, high-dynamic load events were relatively rare (≤10% of the cycles) and weak (max. 30% above SWL). They were weaker but more frequent under the double-hitch treatment, which can partially be explained by the smaller pulling power of the motorized carriage. Under the double-hitch treatment, the dropline was powered by a separate 37 kW engine, while under the single-hitch carriage treatment the pull was provided through the mainline and powered by the yarder 175 kW engine. Therefore, while the observed phenomenon was the same – i.e. a very rapid increase in tension followed by a sudden drop and a rest period – the mechanics were different. While in both instances, the root cause was the load getting stuck, under the double-hitch treatment, the sudden drop arrived earlier and depended on the dropline reaching its maximum pull without being able to break out the load and having to give up; in contrast, under the single-hitch treatment, it was the operator who decided to stop pulling when he realized that he would break the cable or tear down an anchor if he continued. The relatively long lull period after the tension spike derived from the operator changing the hitch or crosscutting the stem to free it from the hang-up. However, even under the more aggressive single-hitch treatment, high-dynamic loads were relatively small and always within the endurance limit (50% of minimum breaking strength: 220 kN in this specific case).
The same could be said for PT: It exceeded SWL by 30% in the worst case, which is still well within the endurance limit. PT was frequently recorded at breakout, similar to all previous studies on the subject (Hartsough Citation1993; Pyles et al. Citation1994; Harrill and Visser Citation2013; Spinelli et al. Citation2017). It is during breakout that stems must “break out” from other stems that may have been felled across them, the load may drag on the ground, and occasionally jams against rocks, stumps or other fixed terrain features. In such occurrences, the pulling force required to overcome the resistance can be higher than the stems own weight. Such pulling forces are transferred through the carriage and into the skyline to cause tension peaks. If a higher pulling force does not dislodge the stems being pulled, this is likely to cause a high-dynamic load event.
The study also produced a regression model for predicting TMS as a function of payload size. This model explained over 80% of the total variability in the data. As such, it was fit to produce a reliable estimate of TMS, where tension was highest. The study data indicated that PTMS values were 2% and 13% higher than TMS values (), for the double-hitch and single-hitch treatments, respectively. These were the largest differences recorded in the study, and PT exceeded TMS by smaller margins in general.
The results of this study are especially important because they indicate that the endurance limit of the skyline was not reached during the trial – even if SWL was often exceeded during lateral skidding and inhaul. That matches the findings of another study conducted a few years earlier in a similar three-cable setup also in the Italian Alps (Spinelli et al. Citation2017), and those of a larger observational study covering multiple installations and configurations also performed in the same region (Mologni et al. Citation2019). Taken together, these studies suggest that loggers in the Italian Alps (and possibly in the wider Alpine region) may operate within safe limits of wire rope capability, while occasionally exceeding legal requirements in terms of SWL. In turn, that supports the decision made by the European Standardization Agency (Technical Committee 144, Working Group 8) to decrease the skyline safety factor from 3 to 2.5 for those yarders equipped with a calibrated slip brake on the skyline drum, like the machines included in this and in the 2017 study. Of course, even if the level of overloading applied by the operators in these tests is likely representative of general practice, there will always be the occasional operator who may push the envelope (Marchi et al. Citation2019; Mologni et al. Citation2019). In that regard, it is worth recalling that the study was conducted during a salvage operation, where trees had not been felled systematically according to a well-defined plan but had been windthrown and were especially hard to disentangle. Under the conditions of a planned harvest, where trees are felled directionally with a view for facilitating extraction, it is likely that hang-ups would be less frequent and easier to resolve. Therefore, this study may represent a worst-case scenario. Even so, the results indicated that AHDL are not as extreme as to require oversize safety factors, provided that operators act responsibly. Of course, all the considerations made above are only valid for standing skyline setups, and cannot be extended to other configurations without proper validation.
Compared with the conventional single-hitch carriage, the double-hitch carriage used in this study offered the benefit of smaller dynamic loads, but that was due to its less powerful dropline engine and not to any specific characteristics of the double-hitch lifting configuration. Essentially, the weaker dropline gave up earlier and at a lower tension than the stronger mainline winch, and therefore AHDL was lower than it would be under the single-hitch carriage treatment. In fact, the double-hitch carriage operated one dropline at a time during breakout, and therefore high-dynamic loads were experienced when working in a single-hitch mode. Considering that high-dynamic loads and PT generally occur during breakout, a suitable measure to prevent excessive skyline tension could be to cap dropline (or mainline) pull. This could be a more efficient strategy than overdesigning the whole system. We now know that the problem arises during this one specific task and related to this one specific component, so it may be more economical to act on that one alone.
If dynamic loading is small and the weaker dynamic loads experienced with the double-hitch treatment are not an inherent benefit of the double-hitch working mode, then what are the advantages of double-hitch carriages? This is summarized as better clearance. Assuming a piece length of 20 m (taller trees are generally crosscut before yarding), double-hitch yarding would offer a clearance gain of approximately 10 m, accounting for a crown radius of about 5 m. However, the heavier weight of the carriage would cause an increase in deflection, so some of this gain would be offset. In the case of the study setup, the midspan deflection for a mean payload of 1,300 kg, a span of 200 m, an SPT of 105 kN, an SWL of 141 kN and a cable weight of 2.35 kg m−1 can be calculated at 8.4 m and 9.3 m for the single-hitch and the double-hitch carriage, respectively (Worksafe BC Citation2006). Therefore, changing to double-hitch yarding would increase clearance by about 9 m. Whether this benefit is worth the cost depends on the specific setup and corridor; where clearance is not an issue, there is no point introducing a heavier and more expensive double-hitch carriage. Conversely, the advantage can be crucial for specific terrain profiles, and may allow shot-gunning loads downhill where that would not be feasible otherwise. For that reason, double-hitch carriages could represent an especially valuable addition to conventional sled-winch operations, which are still very popular in the Alpine area (Spinelli et al. Citation2013). Furthermore, double-hitch horizontal suspension would be crucial when extending cable yarding to flat terrain at sensitive sites (Erber and Spinelli Citation2020). In any case, it is worth noting that double-hitch carriages are designed by fitting a conventional motorized dropline carriage with a dedicated extension: the main investment remains that of the base carriage, which can easily swap configurations, thus adapting to highly variable terrain conditions.
Even where a three-cable configuration was set up and a full suspension would not be indispensable for technical operation, minimum ground contact would have the advantage of lower soil disturbance and reduced branch wood contamination – the latter being especially valuable in the case of biomass recovery (Spinelli et al. Citation2019). However, this study was not designed to explore these further potential advantages of full suspension, and therefore any remarks in that direction remain reasonable speculation that will need to be addressed in future studies. In fact, the operational aspects are being covered in a separate study that compares single-hitch and double-hitch suspensions in terms of productivity and cost (Spinelli et al. Citation2021).
Conclusions
Few skyline tension studies have been conducted under controlled experimental conditions, despite the growing interest for the safe design and operation of cable yarding equipment. Hence, the fundamental merit of this paper allows making at least two important conclusions: first, that high-dynamic loads in a well-managed standing skyline operation are less frequent and extreme than expected; second, that double-hitch horizontal suspension accrues some benefits in terms of reduced cyclic loading, but these benefits are not compelling, since cyclic loading is not extreme – even when extraction is conducted under the conventional single-hitch mode. On the other hand, double-hitch suspension offers a marked advantage in terms of increased clearance, which may be decisive when operating on broken terrain. In particular, the double-hitch option may be especially desirable for traditional sled-winch setups that can only operate in the shotgun configuration and depend on gravity for successful downhill yarding. A smart feature of all current double-hitch carriages is their capacity to quickly convert into single-hitch motorized dropline carriages, which allows maximum operational flexibility. Finally, the study suggests that high-dynamic load hazard could be minimized by capping dropline (or mainline) pulling power, since high-dynamic loads are generally experienced during breakout and originate from the excessive pulling of jammed loads. Therefore, limiting pull capacity might represent a more economical measure than overdesigning the whole setup.
Acknowledgements
The authors thank Mrs. Carolina Lombardinia, Dr. Giuliana Caliandro,a and Mr. Simon Maiere for their valuable assistance with data collection. Special thanks to Maurizio e Omar Del Fabbro for allowing access to their operation and offering full support to the experiment.
Disclosure statement
No potential conflict of interest was reported by the authors.
Additional information
Funding
References
- Aggestam F, Konczal A, Sotirov M, Wallin I, Paillet Y, Spinelli R, Lindner M, Derks J, Hanewinkel M, Winkel G. 2020. Can nature conservation and wood production be reconciled in managed forests? A review of driving factors for integrated forest management in Europe. J Environ Manage. 268:9. doi:https://doi.org/10.1016/j.jenvman.2020.110670.
- Bont L, Heinimann H. 2012. Optimum geometric layout of a single cable road. Eur J For Res. 131:1439–1448. doi:https://doi.org/10.1007/s10342-012-0612-y.
- Erber G, Spinelli R. 2020. Timber extraction by cable yarding on flat and wet terrain: a survey of cable yarder manufacturer’s experience. Silva Fenn. 54(2):19. doi:https://doi.org/10.14214/sf.10211.
- Harrill H. 2014. Improving cable logging operations for New Zealand’s steep terrain forest plantations [dissertation]. Christchurch (New Zealand): University of Canterbury.
- Harrill H, Visser R. 2013. Simulating skyline tensions of rigging configurations. Future Forests Research Ltd. (FFR): HTN05-12. 8 p.
- Hartsough B. 1993. Benefits of remote tension monitoring. Logging Industry Research Organization. LIRO Report 18-23. 13 p.
- Jorgensen J, Carson W, Chalupnik J, Garbini J. 1978. Skyline anchor dynamic tests. Technical Report FE-UW-7702. San Dimas (CA): USDA Forest Service, Equipment Development Center; p. 82.
- Leech C, Overington M. 1982. Dynamics of wire rope subjected to shock axial loads. In: Offshore Technology Conference; 3rd-6th May 1982; Houston, TX, U.S.A. 10 p.
- Magagnotti N, Kanzian C, Schulmeyer F, Spinelli R. 2013. A new guide for work studies in forestry. Int J For Eng. 24:249–253.
- Marchi L, Mologni O, Trutalli D, Scotta R, Cavalli R, Montecchio L, Grigolato S. 2019. Safety assessment of trees used as anchors in cable-supported tree harvesting based on experimental observations. Biosyst Eng. 186:71–82. doi:https://doi.org/10.1016/j.biosystemseng.2019.06.022.
- Mologni O, Grigolato S, Cavalli R. 2016. Harvesting systems for steep terrain in the Italian Alps: state of the art and future prospects. Contemporary Engineering Sciences. 9:1229–1242. doi:https://doi.org/10.12988/ces.2016.68137.
- Mologni O, Lyons CK, Zambon G, Proto AR, Zimbalatti G, Cavalli R, Grigolato S. 2019. Skyline tensile force monitoring of mobile tower yarders operating in the Italian Alps. Eur J For Res. 138(5):847–862. doi:https://doi.org/10.1007/s10342-019-01207-0.
- Morgenroth J, Visser R. 2013. Uptake and barriers to the use of geospatial technologies in forest management. N Z J Forestry Sci. 43:9.
- Motta R, Ascoli D, Corona P, Marchetti M, Vacchiano G. 2018. Silviculture and wind damages. The storm “Vaia”. Forest@ - Rivista di Selvicoltura ed Ecologia Forestale. 15(1):94–98. doi:https://doi.org/10.3832/efor2990-015.
- Olsen E, Hossain M, Miller M. 1998. Statistical comparison of methods used in harvesting work studies. Corvallis (OR): Oregon State University, Forest Research Laboratory; p. 31. Research Contribution 23.
- OR-OSHA. 2010. Yarding and loading handbook. Salem (Oregon): Oregon Occupational Safety and Health Division; p. 184.
- Pyles M, Womack K, Laursen H. 1994. Dynamic characteristics of a small skyline logging system with a guyed tailspar. J For Eng. 6:35–49.
- R Core Team. 2018. R: a language and environment for statistical computing. Vienna: R Foundation for Statistical Computing. [ accessed 2020 Jun 03]. https://www.R-project.org/
- Samset I. 1985. Winch and cable systems (Forestry Sciences). Dordrecht (The Netherlands): Martinus Nijhoff/Dr. W. Junk Publishing; p. 415.
- SAS Institute Inc. 1999. StatView reference. Cary (NC): SAS Publishing; p. 84–93.
- Spinelli R, Magagnotti N, Cosola G, Labelle E, Visser R, Erber G. Forthcoming 2021. The effect of yarding technique on yarding productivity and cost: conventional single-hitch suspension vs. horizontal double-hitch suspension. Croat J For Eng
- Spinelli R, Magagnotti N, Dellagiacoma F. 2008. Meccanizzazione nelle fustaie alpine: due diversi sistemi di lavoro. Sherwood– Foreste e Alberi Oggi. 147: 45–49.
- Spinelli R, Magagnotti N, Facchinetti D. 2013. A survey of logging enterprises in the Italian Alps: firm size and type, annual production, total workforce and machine fleet. Int J For Eng. 24:109–120.
- Spinelli R, Marchi E, Visser R, Harrill H, Gallo R, Cambi M, Neri F, Lombardini C, Magagnotti N. 2017. The effect of carriage type on yarding productivity and cost. Int J For Eng. 28:34–41.
- Spinelli R, Nati C, Magagnotti N. 2006. Recupero di biomassa. Alcune utilizzazioni in boschi alpini (Biomass recovery: case studies in Alpine forests). Sherwood– Foreste e Alberi Oggi. 119: 21–27.
- Spinelli R, Visser R, Björheden R, Röser D. 2019. Recovering energy biomass in conventional forest operations: a review of integrated harvesting systems. Curr For Rep. 5:90–100.
- Spinelli R, Visser R, Thees O, Sauter H, Krajnc N, Riond C, Magagnotti N. 2015. Cable logging contract rates in the Alps: the effect of regional variability and technical constraints. Croat J For Eng. 36:195–203.
- Stampfer K, Visser R, Kanzian C. 2006. Cable corridor installation times for European yarders. Int J For Eng. 17:71–77.
- Valente C, Spinelli R, Hillring BG. 2011. LCA of environmental and socio-economic impacts related to wood energy production in alpine conditions: Valle di Fiemme (Italy). J Cleaner Prod. 19(17–18):1931–1938. doi:https://doi.org/10.1016/j.jclepro.2011.06.026.
- Visser R. 1998. Tension monitoring of forestry cable systems [dissertation]. Vienna (Austria): University of Natural Resources and Life Sciences, Vienna.
- Wassermann C. 2018. Mastseilgeräte für die Holzernte: Eine Analyse des europäischen Herstellerangebotes (Tower yarders for wood extraction: an analysis of the European market offer) [master’s thesis]. Vienna (Austria): University of Natural Resources and Life Sciences, Vienna.
- WorkSafeBC. 2006. Cable yarding systems handbook. Vancouver (British Columbia): Workers’ Compensation Board of British Columbia; p. 204.