Abstract
Objective
The aim of the research was to evaluate the feasibility of measuring the bone conduction hearing threshold using self-administered mobile audiometry.
Design
A single-centre, closed, cross-over trial was carried out on patients from the ENT Department. A mobile-based, self-administered, audiologist-assisted assessment of the bone conduction hearing threshold was carried out by means of the open-access, freeware app Hearing Test using two types of bone conduction headphones: professional B71 bone transducer and commercially available AfterShokz Openmove open-ear headphones.
Study sample
Seventy-seven ears.
Results
A test-retest examination revealed the lowest standard deviation for open-ear headphones at 3.33 dB (95% CI 2.92–3.79). When compared with pure tone audiometry, the intraclass correlations of 0.95 (95% CI 0.94–0.96) and 0.90 (95% CI 0.88–0.92) were obtained for the bone transducer and for the open-ear headphones, indicating excellent and good reliability, respectively. However, the regression slope of 0.67 was found for the air-bone gap when using open ear headphones, which was significantly different from 1 (p < 0.001).
Conclusions
Open-ear headphones provide an alternative for estimating bone conduction once the air-bone gap has been adjusted by a factor of 1/0.6 7 ≅1.5. They demonstrate improved reproducibility over the bone transducer and are much easier to use with a mobile device. Trial Registration: Wroclaw Medical University, Science Support Centre, BW60/2020
Introduction
It has been estimated that about 1.6 billion people worldwide experienced hearing loss in 2019, which is more than 20% of the global population (Haile et al. Citation2021; Masalski and Morawski Citation2020; World Health Organization Citation2021). This number is projected to increase to 2.45 billion in 2050 (Haile et al. Citation2021). If left undiagnosed and untreated, it can result in a reduced quality of life, social exclusion, loneliness, and significant financial losses to healthcare systems (Tseng et al. Citation2018; Blazer Citation2020; Reed et al. Citation2019; World Health Organization Citation2021). Hearing loss is a risk factor of age-related dementia and cognitive impairment (Blazer Citation2020; Livingston et al. Citation2020). It is significantly more common in middle- and low-income countries (Stevens et al. Citation2013), where access to appropriate audiological diagnostics is limited. Hence, there is a need to develop easily accessible and inexpensive screening methods for hearing loss.
The COVID-19 pandemic demonstrated an increased need to develop reliable diagnostic methods for telemedicine (D'Onofrio & Zeng, Citation2021; Forde et al. Citation2022). To limit the spread of the pandemic, many medical appointments were conducted remotely and some scheduled hospital procedures were suspended. This situation increased the need for telemedicine solutions, including remote audiological solutions (Garcia et al. Citation2021).
There are a number of modalities for testing hearing outside of clinical settings. These include portable audiometers and mobile apps. Applications with gaming elements have been used in diagnosing hearing loss in children (Kung et al. Citation2021; Yeung et al. Citation2013). Recent reports also suggest the possibility of using data-driven modelling techniques, including machine learning and data mining (Wasmann et al. Citation2022; Wasmann et al. Citation2021; Masalski, Adamczyk, and Morawski Citation2021). Test results can be automatically evaluated or forwarded to audiologists for remote evaluation with properly validated methods.
Multiple studies have confirmed that it is possible to reliably test hearing using a smartphone (Irace et al. Citation2021; Aremu Citation2018; Barczik and Serpanos Citation2018; Swanepoel et al. Citation2014; Abu-Ghanem et al. Citation2016; Larrosa et al. Citation2015; Peer and Fagan Citation2015; Handzel et al. Citation2013; Masalski, Grysiński, and Kręcicki Citation2018; Chen et al. Citation2021; Van Tonder et al. Citation2017; Renda et al. Citation2016; Wasmann et al. Citation2022). The sensitivity and specificity of this method in detecting hearing loss with respect to threshold tonal audiometry are 89% and 93%, respectively, and the diagnostic accuracy is affected by patients’ age, type of headphones and use of a soundproof booth (Chen et al. Citation2021). However, evaluating air conduction hearing is not enough to provide full insight into the type of hearing loss. In order to differentiate between conductive and sensorineural hearing loss, it is necessary to investigate bone conduction hearing as well.
Assessment of the bone conduction threshold should be performed whenever the air conduction threshold is elevated, particularly if the low and mid-frequency range is affected. Currently, the bone conduction threshold is determined by pure tone audiometry, mainly in clinical settings. Adding the functionality of bone conduction testing to mobile audiometry may broaden the indications for its use. Mobile audiometry, especially when including bone conduction assessment, may prove useful, for instance in the following cases: as an alternative to pure tone audiometry in conditions of limited access to instrumentation, in the initial assessment before visiting an ENT specialist, in the diagnosis of sudden sensorineural hearing loss at a GP, or even in the remote fitting of hearing aids.
Pure tone audiometry evaluates bone conduction hearing by means of a bone transducer placed on the mastoid process or on the forehead. This transducer can also be driven by a mobile device (Dewyer et al. Citation2019; Thoidis et al. Citation2019) providing hearing thresholds consistent with pure tone audiometry. However, the use of a single transducer prevents masking of the non-tested ear, thus allowing only for the evaluation of the best-cochlea thresholds (Dewyer et al. Citation2019). In addition, the majority of individuals were found to require assistance in the proper placement of the bone transducer on the mastoid process (Dewyer et al. Citation2019).
An alternative to the bone transducer may be wireless, open-ear bone conduction headphones. They feature an open-ear design, allowing users to hear their surroundings. This is achieved by vibrating transducers placed bilaterally over the temporomandibular joint between the tragus and the mandibular condyle. Compared to a bone transducer, open-ear headphones are more comfortable to put on and wear, allow masking of the non-tested ear and are also less expensive and more widely available. However, open-ear headphones use a different conduction pathway (Sugawara, Otsuka, and Nakagawa Citation2021), which might not overlap adequately with bone conduction.
There is limited literature on the use of open-ear headphones in assessing the bone conduction hearing threshold. A study of the self-measured bone conduction hearing threshold using the AfterShokz Sportz 2 open-ear headphones identified the conductive component in 17 of 24 ears (71%) (Li et al. Citation2021). However, the study was performed without prior calibration and contains no direct comparison with pure tone audiometry thresholds. Hearing threshold analysis is essential in assessing the validity of open-ear headphones, given the different conduction pathway, as it may also depend on the frequency of the stimulus.
The purpose of this work was to compare the air conduction (AC) and bone conduction (BC) hearing threshold and the air-bone gap (ABG) determined by mobile audiometry with pure tone audiometry. Two different types of bone conduction headphones, bone transducer and open-ear headphones, were used in the evaluation of the bone conduction hearing threshold carried out on a mobile device.
Methods
It was a single-centre, closed, cross-over trial carried out on patients from the ENT Department. Consent to conduct the trial was given by the Bioethics Committee of Wroclaw Medical University (BW60/2020). Prior to the study, the participants were informed of the purpose and course of the study and signed the informed consent. Study participants were recruited offline using face-to-face prompting from among adult patients hospitalised in the ENT department for audiological or otological conditions. Patients with otorrhea, mental disability, or a lack of computer literacy were excluded from the study.
The study consisted of a mobile-based, self-administered, audiologist-assisted assessment of air and bone conduction hearing thresholds and a comparison of these thresholds with those determined by means of pure tone audiometry. In each ear, the hearing threshold was assessed five times, twice for air conduction (by means of pure tone and mobile audiometry) and three times for bone conduction (by pure tone audiometry and twice by mobile audiometry using two different types of bone headphones). The assessment order was randomised. Given the potential participant fatigue due to the number of measurements, the testing was divided into two sessions, separately for the right and the left ear. Additionally, retests were performed at the end of the session for randomly selected settings. Due to potential logistic limitations in conducting both sessions in each subject, the worse ear was tested first.
Air and bone conduction pure tone audiometry was performed using an Interacoustic AD629 clinical audiometer with TDH-39 headphones and a B71 bone transducer calibrated according to ISO 389-1:2017 and ISO 389-3:2017 standards, respectively. The measurements were performed using the conventional 10 dB down and 5 dB up bracketing method following the recommendations of the British Society of Audiology (Citation2018). Testing was performed at frequencies of 0.25, 0.5, 1, 2, 4, 6 and 8 kHz and at frequencies of 0.25, 0.5, 1, 2 and 4 kHz for air and bone conduction, respectively.
Mobile-based assessment of hearing threshold was performed using the open access, freeware Hearing Test app (Masalski, Citation2021) and a Samsung S10 smartphone. The app was developed for Android devices and includes functionality allowing for self-measurement of the hearing threshold. It generates frequency-specific stimuli of variable intensity, the level of which can be adjusted to the dB HL by providing calibration coefficients for each frequency. The app had previously been successfully validated for self-measurement of the air conduction hearing threshold (Renda et al. Citation2016; Masalski, Grysiński, and Kręcicki Citation2018). For the purpose of this work, modifications were made to the app in terms of stimulus masking. The air conduction hearing threshold was determined using the bundled earphones supplied by the manufacturer in a set with the smartphone. The bone conduction hearing threshold was determined twice, using a B71 bone transducer, the same as those used in pure tone audiometry and using AfterShokz Openmove open-ear headphones, which transmit vibrations in the temporomandibular joint region (). The bundled earphones were connected directly to a headphone jack, the bone transducer B71 was connected using a jack adapter and the open-ear headphones were connected wirelessly via Bluetooth. The masking noise was delivered via the contralateral earpiece of the bundled earphones and the open-ear headphones, whereas the bone transducer B71 required an additional earpiece connected via a jack adapter; for this purpose, the earpiece of the bundled headphones was used.
Figure 1. B71 bone transducer (left) and AfterShokz Openmove open-ear headphones (right) used to access the bone conduction hearing threshold.
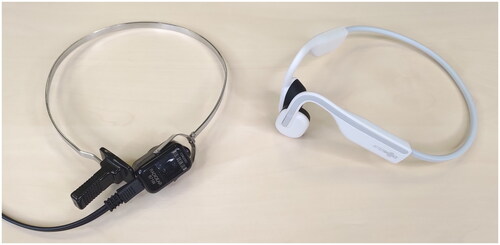
The masking noise was produced by digital filtering of the white noise with an infinite impulse response (IIR) filter. A 2nd-order Butterworth filter with a frequency bandwidth of 1/3 octave and a centre frequency equal to the stimulus frequency was used. The effective masking level (EML) was determined by evaluating the audibility of the stimulus presented together with the masking noise through a single earpiece of the bundled headphones.
During testing, the masking noise level was set with respect to the stimulus level in 20 dB steps. For the bundled headphones and the open-air headphones, the masking noise level was set at 10, 30, 50 and 70 dB EML for the stimulus up to 20 dB HL, 25–40 dB HL, 45–60 dB HL and above 60 dB HL, respectively. For the bone transducer B71, higher masking levels were required due to lower interaural attenuation combined with possible ABG in the non-tested ear. The masking levels for the bone transducer were about 35 dB higher than those for the bundled and open-ear headphones and resulted from driving the earpiece of the bundled headphones with a signal calibrated for the bone transducer.
For each headphone, calibration was performed using a biological method (Masalski, Grysiński, and Kręcicki Citation2018; Masalski and Kręcicki, Citation2013; Masalski, Grysiński, and Kręcicki Citation2014; Masalski et al. Citation2016). Three normal-hearing employees with a hearing threshold verified in pure tone audiometry of less than 15 dB HL were tested using the bundled headphones, bone transducer and open-ear headphones. Calibration coefficients were determined as the average difference between pure tone audiometry and mobile audiometry thresholds. For each frequency and each transducer, the calibration coefficient was stored in the app and was used to calculate the sound level in dB HL scale. The tests performed for calibration were analogous to those performed by the study participants.
The mobile-based hearing test involved the self-determination of the quietest audible sound. The participant used the "I can hear" and "I can’t hear" buttons to change the sound intensity and confirmed the quietest audible sound with the "Barely audible" button. The tests were conducted in a soundproof booth under the supervision of an audiologist, who was responsible for familiarising the participant with the application and for ensuring that the test was performed correctly, in particular by checking that the headphones were properly placed, that the side was correct, that the measurements were performed at all frequencies and that the participant was responding to the stimuli and not to the masking noise. Analogous with pure tone audiometry, measurements were carried out at frequencies of 0.25, 0.5, 1, 2, 4, 6 and 8 kHz and at frequencies of 0.25, 0.5, 1, 2 and 4 kHz for air and bone conduction, respectively.
Statistical analysis
The sample size was determined based on the standard deviation of the difference in the ABG between pure tone audiometry and mobile audiometry estimated in preliminary measurements at the level of 11 dB. Assuming the statistical significance level of 0.05, the test power of 0.8 and the effect size of 5.0 dB, a sample size of 76 ears was targeted. The results were analysed using regression plots, Bland-Altman plots and intraclass correlation coefficients. Any hearing thresholds that exceeded the maximum output level of the equipment at a particular frequency were removed from the analysis.
Results
The study was conducted between July 22, 2021 and March 21, 2022 on 47 subjects, 27 males (57%) and 20 females (43%) aged 23 to 79 years, mean 46 (SD 17.4). A total of 77 ears were examined, revealing a normal hearing threshold in 32 (41.6%) ears, sensorineural hearing loss in 17 (22.1%), conductive hearing loss in 6 (7.8%) and mixed hearing loss in 22 (28.6%) ears. The baseline characteristics of the study group are shown in .
Table 1. Baseline characteristics of the study group.
For each investigated ear, the following tests were performed in a random order: AC pure tone audiometry, BC pure tone audiometry, AC mobile audiometry using bundled headphones, BC mobile audiometry using bone transducer, and BC mobile audiometry using open-ear headphones. Following all of the tests, retests were performed in a random order as well. Retesting was discontinued if the patient reported fatigue. This resulted in 385 tests (77 ears × 5 tests) and 226 retests (42, 43, 49, 46 and 46 for AC pure tone audiometry, BC pure tone audiometry, AC mobile audiometry, BC mobile audiometry using bone transducer and BC mobile audiometry using open-ear headphones, respectively). The duration of unilateral hearing threshold evaluation by means of a mobile device for a single headphone set measured by the app averaged 2 min 47 s (SD 58 s).
The ABG was calculated for pure tone audiometry and twice for mobile audiometry. The mobile audiometry ABG was determined once using the bone transducer B71 and the second using open-ear headphones in both cases assuming AC threshold measured using bundled headphones.
Test-retest
The test-retest summary results for all frequencies are presented in , whereas the results by frequency are presented in supplemental material (Supplementary Table A1). The lowest standard deviation of test-retest difference was obtained for open-ear headphones at the level of 3.33 dB (95% CI 2.92–3.79). The values for air conduction and bone conduction using bone transducer obtained by mobile were comparable to those obtained by pure tone audiometry. For the ABG, the lowest value was also revealed when using open-ear headphones.
Table 2. Test-retest differences in hearing threshold.
Comparison of hearing threshold
The air and bone conduction hearing thresholds obtained by mobile audiometry were compared with those of pure tone audiometry. A summary for all frequencies is presented in , and and by frequency in supplementary material (Table A2). An excellent intraclass correlation (Koo and Li Citation2016) was obtained for air conduction and for bone conduction when using a bone transducer at 0.95 (95% CI 0.94–0.96) and 0.90 (95% CI 0.88–0.92), respectively. The correlation for open-ear headphones was slightly lower at 0.82 (95% CI 0.78–0.85), indicating good reliability. The ABG measured using bone transducer had a higher correlation coefficient of 0.82 (95% CI 0.78–0.85) than using open-ear headphones at 0.59 (95% CI 0.52–0.65), corresponding to good and moderate reliability, respectively. The regression slope was found to be significantly different from 1 (p < 0.001) only for the ABG using open-ear headphones at 0.67 (95% CI 0.56–0.77).
Figure 2. Regression plots of air conduction (AC) and bone conduction (BC) hearing threshold for mobile audiometry versus pure tone audiometry with corresponding Bland-Altman plots. B71 bone transducer and AfterShokz Openmove open-ear headphones were used. The dashed lines indicate the 5th and 95th percentiles.
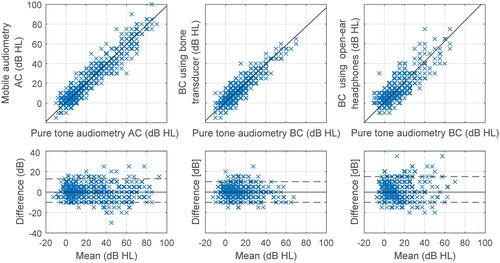
Figure 3. Regression plots of air-bone gap (ABG) for mobile audiometry versus pure tone audiometry with corresponding Bland-Altman plots. B71 bone transducer and AfterShokz Openmove open-ear headphones were used. The dashed lines indicate the 5th and 95th percentiles.
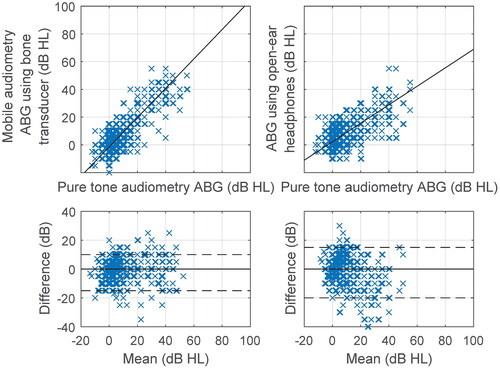
Table 3. Air conduction hearing threshold (AC), bone conduction hearing threshold (BC) and air-bone gap (ABG) comparison between pure tone audiometry and mobile audiometry.
Discussion
Principal results
The lowest test-retest standard deviation was obtained for open-ear headphones at 3.33 dB (95% CI 2.92–3.79) and it was significantly lower (p < 0.001) than both values obtained for the bone transducer at 4.75 dB (95% CI 4.21–5.49) and at 5.36 dB (95% CI 4.89–5.93). Despite the very high reproducibility of measurements using open-ear headphones, the intraclass correlation coefficient for the comparison with pure tone audiometry determined at the level of 0.82 (95% CI 0.78–0.85) was significantly worse (p < 0.001) than the coefficient of the bone transducer at 0.90 (95% CI 0.88–0.92). The underlying causes seem to be an underestimation of the ABG measured with open-ear headphones as confirmed by the regression slope of 0.67 (95% CI 0.56–0.77), which is significantly less than 1 (p < 0.001).
Limitations
Open-ear headphones and bone transducer differ in the location of the transducer. The transducers of the open-ear headphones are located between the tragus and the mandibular condyle while the bone transducer B71 is placed above the mastoid process. This implies a different stimulus conduction pathway. Generally, in addition to the air and bone conduction component, there is also an osseotympanic component (Sugawara, Otsuka, and Nakagawa Citation2021), which involves sound radiated into the ear canal primarily by outer ear cartilages. When the transducer is located in the preauricular region, the osseotympanic component seems to play a noticeable role besides the dominant bony component (Sugawara, Otsuka, and Nakagawa Citation2021). This explains the ABG underestimation using open-ear headphones, as the osseotympanic impairment in subjects with conductive hearing loss is compensated by higher intensities. In this work, the effect of the osseotympanic conduction was found primarily at 1 kHz and below. Sugawara et al. (Sugawara, Otsuka, and Nakagawa Citation2021) found it mainly at 1 and 2 kHz, which may be related to the different methodologies. In this study, the analysis was performed on subjects with conductive hearing loss and Sugawara et al. (Sugawara, Otsuka, and Nakagawa Citation2021) blocked the osseotympanic component with water injected into the ear canal in normal hearing subjects.
Transducers of the open-ear headphones, like the bone transducer B71, generate sound in addition to vibration, which can be audible at high intensities via air conduction even when not in contact with the body. The additional air conduction pathway, similar to the osseotympanic pathway, could lead to an underestimation of ABG. The effect associated with the additional air conduction pathway appears to be much weaker compared to the osseotympanic, since the sounds generated by open-ear headphones become louder when the ear canal is occluded. Nonetheless, these observations do not follow from the tests conducted and it seems reasonable to verify them in further studies.
The ABG analysis was performed without controlling the origin of conductive hearing loss. However, conductive hearing loss may be related to a number of causes, including earwax, perforation of the tympanic membrane, otitis media with effusion, cholesteatoma, or otosclerosis. Since the role of the osseotympanic component in the above conditions may vary, the relationship between the thresholds obtained using open-ear headphones and bone transducer thresholds may also be different. It seems reasonable to conduct similar studies in groups with a uniform cause of conductive hearing loss.
The lowest standard deviation of test-retest difference was found for open-ear headphones at the level of 3.33 dB (95% CI 2.92–3.79) indicating excellent reproducibility. However, the standard deviation of the difference between open-ear headphones and bone transducer was found to be considerably higher at 11.48 dB (95% CI 10.56–12.49) and stayed that way even when recalculated for normal-hearing subjects at 7.11 dB (95% CI 6.51–7.92). A possible explanation is an inter-personal difference in the anatomy of the preauricular region, e.g. a greater variation in the amount of adipose tissue (Pilsl, Anderhuber, and Rzany Citation2012) than that observed on the mastoid process.
The maximum sound levels generated by bone transducer and open-ear headphones when connected to a mobile device resulted from frequency-specific calibration coefficients and the sound intensity produced at maximum output reduced by about 5 dB. The reduction was introduced to avoid potential non-linearities in the upper range and Android system beeps in open-ear headphones when reaching maximum output. The maximum sound level generated by the bone transducer ranged from 30 dB HL at 0.25 kHz to 65 dB HL at 2 kHz, while for open-ear headphones it ranged from 70 dB HL at 0.25 kHz to 80 dB HL at 2 kHz. Thus, theoretically, higher degrees of hearing loss can be identified with open-ear headphones than with the B71 bone transducer. However, above 60 dB HL, especially at low frequencies, the vibrations of the transducer can be detected by the sense of touch and not perceived as sound. Distinguishing whether a stimulus is felt or heard can be problematic for the subject.
The masking noise level was determined to be between a minimum level that effectively masks the non-tested ear and a maximum level that does not impair the perception of the stimulus in the tested ear, regardless of the hearing threshold. It was assumed that, in the non-tested ear, the masking level reduced by the possible ABG must be greater than the stimulus level reduced by the interaural attenuation and, in the tested ear, the stimulus level reduced by the possible ABG must be greater than the masking level reduced by the interaural attenuation. The minimum and maximum masking levels were calculated for interaural attenuation of 60 dB, 15 dB and 15 dB for bundled headphones, open-ear headphones and bone transducer, respectively and for a maximum ABG of 40 dB if the signal was delivered via air conduction (). For the bundled and open-ear headphones, the masking level was consistently between the maximum and minimum level. For the bone transducer, cross-hearing may have occurred if ABG in non-tested ear was 40 dB or more and the BC threshold of the tested ear was higher than of the non-tested ear at least by interaural attenuation. Such a combination occurred only 3 times, each time at a different frequency, and had no significant effect on the results of the study, as confirmed by the regression slope being no significantly different from 1 for the bone transducer and for ABG using the bone transducer ().
Table 4. Masking level (ML) applied during testing compared with theoretically determined minimum masking level (MLmin) and maximum masking level (MLmax).
Automatic masking significantly simplifies the test, especially for subjects without audiology training. For bundled and open-ear headphones, it is possible to set the masking level to fall between the minimum and maximum masking level by considering the stimulus level only. However, the solution used in this work, assuming a constant masking level within the ranges, makes it difficult to evaluate the audibility of the stimulus when the ranges change, since both the masking noise and the stimulus are altered. Additionally, the solution does not take into account central masking. Optimisation of the automatic masking algorithm requires further research.
The linearity of the output for the transducers connected to the mobile device has not been verified prior to the measurements. Nevertheless, the slopes of the regression lines calculated for the bundled headphones, bone transducer and open-ear headphones showed no statistically significant differences from 1 at p = 0.05 (), suggesting the linearity of their output. Additionally, the slope for open-ear headphones was recalculated after excluding subjects with air-bone gap. A value of 0.92(95% CI 0.82–1.01) was obtained, thus also indicating no statistically significant difference from 1 at the p = 0.05 level. These results are supported by the work of others (Larrosa et al. Citation2015; Hazan et al. Citation2022; Masalski, Grysiński, and Kręcicki Citation2018), who also reported linearity at the output when headphones are connected to a mobile device.
Comparison with prior work
It has already been proven that mobile audiometry demonstrates high compatibility with air conduction pure tone audiometry (Irace et al. Citation2021; Aremu Citation2018; Barczik and Serpanos Citation2018; Swanepoel et al. Citation2014; Abu-Ghanem et al. Citation2016; Larrosa et al. Citation2015; Peer and Fagan Citation2015; Handzel et al. Citation2013; Masalski, Grysiński, and Kręcicki Citation2018; Chen et al. Citation2021; Van Tonder et al. Citation2017; Renda et al. Citation2016; Wasmann et al. Citation2022). In this study, the mean difference in the air conduction hearing threshold between mobile audiometry and pure tone audiometry was found to be 0.23 dB (95% CI −0.42 to 0.89), SD 7.45 dB (95% CI 6.95–8.04). This finding is in agreement with a previous study using the same Hearing Test app in which the mean difference was obtained at 2.6 dB (95% CI 2.0–3.1), SD 8.3 dB (95% CI 7.9–8.7) (Masalski, Grysiński, and Kręcicki Citation2018). The values reported by other authors also remain at similar levels: 0.5 dB, SD 6.7 dB (Kam et al. Citation2012), −1.6 dB, SD 9.9 (Sandström et al. Citation2016) and −2.2 dB, SD 10.0 dB (Sandström et al. Citation2020).
While data on air conduction hearing threshold measured using a mobile device are well established, there are few studies on bone conduction. Thoidis et al. (Thoidis et al. Citation2019) developed a tablet-based diagnostic audiometer that used air-conduction TDH50P and bone-conduction B71 headphones driven directly by a mobile device. Audiologist-administered tests conducted in a soundproof booth according to the modified Hughson-Westlake method revealed extremely high agreement with a conventional audiometer. The ABG difference was found at −0.5 dB, SD 4.6 dB. This is significantly better than the ABG difference for the self-administered tests which was obtained in this work at the level of −0.85 dB (95% CI −1.79 to 0.00), SD 8.65 dB (95% CI 8.03–9.51). Self-administered bone conduction testing using B71 transducer driven directly by a mobile device was also described by Dewyer at al. (Dewyer et al. Citation2019). Here, 61% of thresholds were within 5 dB of pure tone audiometry thresholds and 79% were within 10 dB. These results are closer to this paper, where the above values were calculated at 74% and 95%, respectively.
In addition to the B71 transducer, bone conduction hearing threshold measurements were conducted with open-ear headphones, which are easier to use and, unlike bone transducer, do not require assistance for placement. A study by Li et al. (Li et al. Citation2021) was conducted using headphones of this type (Aftershokz Sportz 2) and reported sensitivity for detecting moderate hearing loss (mean bone-conduction hearing threshold at 0.5, 1, 2, 4 kHz greater than 40 dB HL) of 0.64 (95% CI 0.53–0.75) with a specificity of 0.71 (95% CI 0.62–0.78). However, the paper does not include more precise results and the study was conducted without prior calibration, which may be the reason for the limited sensitivity and specificity.
Generalisation
Open-ear headphones have improved reproducibility but underestimate the ABG when compared to the bone transducer B71. When determining the ABG using open-ear headphones, the results should be multiplied by a factor of 1/0.6 7 ≅1.5. With this solution, reproducibility of the ABG using open-ear headphones decreases to the level comparable with the bone transducer. The test-retest difference of ABG for open-ear headphones, multiplied by the factor of 1.5 equals 7.46 dB and stays within the 95% confidence interval for the bone transducer 6.08–7.50.
The study was designed primarily to compare hearing thresholds determined by different methods. Nevertheless, the observations of the supervising audiologist in terms of self-use of the equipment seem very relevant. Almost every subject required assistance to place the bone transducer B71 and hardly any to put on the open-ear headphones. The open-ear headphones connect wirelessly and do not require a jack adapter or additional air headphones for contralateral masking. The usability differences are definitely in favour of sport headphones, which is especially important for self-testing.
Conclusions
Bone conduction mobile audiometry using the bone transducer B71 demonstrated excellent compatibility with pure tone audiometry. Using open-ear headphones, even higher repeatability was obtained, but the air-bone gap proved to be underestimated and required multiplication by a factor of 1.5.
Open-ear headphones are much easier to use, especially with a mobile device, compared to bone transducer B71 and provides an alternative method for mobile audiometry to assess the bone conduction threshold. However, it is important to note that differences in the ABG can be observed due to the distinct conduction pathways. They may vary depending on the cause of the hearing loss and the anatomy of the preauricular region.
Supplemental Material
Download MS Word (18.6 KB)Supplemental Material
Download PDF (38.7 KB)Disclosure statement
The first author of this paper (MM) is the author and owner of the Hearing Test app, by means of which the measurements described in this paper were conducted. The authors have no commercial interest in AfterShokz products.
References
- Abu-Ghanem, S., O. Handzel, L. Ness, M. Ben-Artzi-Blima, K. Fait-Ghelbendorf, and M. Himmelfarb. 2016. “Smartphone-based audiometric test for screening hearing loss in the elderly.” European Archives of Oto-Rhino-Laryngology 273 (2):333–339. doi:10.1007/s00405-015-3533-9.
- Aremu, S. K. 2018. “Evaluation of the Hearing Test Pro Application as a Screening Tool for Hearing Loss Assessment.” Nigeria Medical Journal 59 (5):45–49.
- Barczik, J., and Y. C. Serpanos. 2018. “Accuracy of Smartphone Self-Hearing Test Applications Across Frequencies and Earphone Styles in Adults.” American Journal of Audiology 27 (4):570–580. doi:10.1044/2018_AJA-17-0070.
- Blazer, D. G. 2020. “Hearing loss and psychiatric disorders.” The Hearing Journal 73 (11):6. doi:10.1097/01.HJ.0000722492.41773.6c.
- British Society of Audiology 2018. Recommended procedure pure-tone air-conduction and bone-conduction threshold audiometry with and without masking. https://www.thebsa.org.uk/wp-content/uploads/2018/11/OD104-32-Recommended-Procedure-Pure-Tone-Audiometry-August-2018-FINAL.pdf
- Chen, C.-H., H.-Y H. Lin, M.-C. Wang, Y.-C. Chu, C.-Y. Chang, C.-Y. Huang, and Y.-F. Cheng. 2021. “Diagnostic accuracy of smartphone-based audiometry for hearing loss detection: Meta-analysis.” JMIR mHealth and uHealth 9 (9):e28378. doi:10.2196/28378.
- D'Onofrio, K. L., and F.-G. Zeng. 2021. “Tele-Audiology: Current State and Future Directions.” Frontiers in Digital Health 3:788103. doi:10.3389/fdgth.2021.788103.
- Dewyer, N. A., P. Jiradejvong, D. S. Lee, J. D. Kemmer, J. Henderson Sabes, and C. J. Limb. 2019. “Automated Smartphone Audiometry: A Preliminary Validation of a Bone-Conduction Threshold Test App.” Annals of Otology, Rhinology & Laryngology 128 (6):508–515. doi:10.1177/0003489419828770.
- Forde, C. T., L. Dimitrov, S. Doal, J. Patel, D. Clare, M. Burslem, N. Mehta, and J. G. Manjaly. 2022. “Delivery of remote otology care: a UK pilot feasibility study.” BMJ Open Quality 11 (1):e001444. doi:10.1136/bmjoq-2021-001444.
- Garcia, A., D. A. Chari, K. M. Stankovic, D. J. Lee, and E. D. Kozin. 2021. “Implementation of Mobile Audiometry During the COVID-19 Pandemic.” Otolaryngology - Head and Neck Surgery 167 (3): 465–468.
- Haile, L. M., K. Kamenov, P. S. Briant, A. U. Orji, J. D. Steinmetz, A. Abdoli, M. Abdollahi, E. Abu-Gharbieh, A. Afshin, H. Ahmed, et al. 2021. “Hearing loss prevalence and years lived with disability, 1990-2019: Findings from the Global Burden of Disease Study 2019.” The Lancet 397 (10278):996–1009. doi:10.1016/S0140-6736(21)00516-X.
- Handzel, O., O. Ben-Ari, D. Damian, M. M. Priel, J. Cohen, and M. Himmelfarb. 2013. “Smartphone-based hearing test as an aid in the initial evaluation of unilateral sudden sensorineural hearing loss.” “p.” Audiology and Neurotology 18 (4):201–207. doi:10.1159/000349913.
- Hazan, A., J. Luberadzka, J. Rivilla, A. Snik, B. Albers, N. Méndez, N. Wack, O. Paytuvi, A. Zarowski, E. Offeciers, et al. 2022. “Home-Based Audiometry With a Smartphone App: Reliable Results?” American Journal of Audiology 31 (3S):914–922. doi:10.1044/2022_AJA-21-00191.
- Irace, A. L., R. K. Sharma, N. S. Reed, and J. S. Golub. 2021. “Smartphone-Based Applications to Detect Hearing Loss: A Review of Current Technology.” Journal of the American Geriatrics Society 69 (2):307–316. doi:10.1111/jgs.16985.
- Kam, A. C. S., J. K. K. Sung, T. Lee, T. K. C. Wong, and A. van Hasselt. 2012. “Clinical evaluation of a computerized self-administered hearing test.” International Journal of Audiology 51 (8):606–610. doi:10.3109/14992027.2012.688144.
- Koo, T. K., and M. Y. Li. 2016. “A Guideline of Selecting and Reporting Intraclass Correlation Coefficients for Reliability Research.” Journal of Chiropractic Medicine 15 (2):155–163. doi:10.1016/j.jcm.2016.02.012.
- Kung, B., L. Kunda, S. Groff, E. Miele, M. Loyd, and D. M. Carpenter. 2021. “Validation Study of Kids Hearing Game: A Self-Administered Pediatric Audiology Application.” The Permanente Journal 25 (2):1–1. doi:10.7812/TPP/20.157.
- Larrosa, F., J. Rama-Lopez, J. Benitez, J. M. Morales, A. Martinez, M. A. Alañon, D. Arancibia-Tagle, A. Batuecas-Caletrio, M. Martinez-Lopez, N. Perez-Fernandez, et al. 2015. “Development and evaluation of an audiology app for iPhone/iPad mobile devices.” Acta Oto-Laryngologica 135 (11):1119–1127. doi:10.3109/00016489.2015.1063786.
- Li, L. Y. J., S. Y. Wang, J. M. Yang, C. J. Chen, C. Y. Tsai, et al. 2021. “Validation of a personalized hearing screening mobile health application for persons with moderate hearing impairment.” Journal of Personalized Medicine 11 (10): 1035. doi:10.3390/jpm11101035
- Livingston, G., J. Huntley, A. Sommerlad, D. Ames, C. Ballard, S. Banerjee, C. Brayne, A. Burns, J. Cohen-Mansfield, C. Cooper, et al. 2020. “Dementia prevention, intervention, and care: 2020 report of the Lancet Commission.” Lancet (London, England) 396 (10248):413–446. doi:10.1016/S0140-6736(20)30367-6.
- Masalski, M., M. Adamczyk, and K. Morawski. 2021. “Optimization of the Speech Test Material in a Group of Hearing Impaired Subjects: A Feasibility Study for Multilingual Digit Triplet Test Development.” Audiology Research 11 (3):342–356. https://www.mdpi.com/2039-4349/11/3/32 doi:10.3390/audiolres11030032.
- Masalski, M., T. Grysiński, and T. Kręcicki. 2018. “Hearing tests based on biologically calibrated mobile devices: Comparison with pure-tone audiometry.” JMIR mHealth and uHealth 6 (1):e10. doi:10.2196/mhealth.7800.
- Masalski, M., T. Grysiński, and T. Kręcicki. 2014. “Biological calibration for web-based hearing tests: Evaluation of the methods.” Journal of Medical Internet Research 16 (1):e11. doi:10.2196/jmir.2798.
- Masalski, M., L. Kipiński, T. Grysiński, and T. Kręcicki. 2016. “Hearing tests on mobile devices: Evaluation of the reference sound level by means of biological calibration.” Journal of Medical Internet Research 18 (5):e130. doi:10.2196/jmir.4987.
- Masalski, M., and T. Kręcicki. 2013. “Self-test web-based pure-tone audiometry: Validity evaluation and measurement error analysis.” Journal of Medical Internet Research 15 (4):e71. doi:10.2196/jmir.2222.
- Masalski, M., and K. Morawski. 2020. “Worldwide prevalence of hearing loss among smartphone users: Cross-sectional study using a mobile-based app.” Journal of Medical Internet Research 22 (7):e17238. doi:10.2196/17238.
- Masalski, M. 2021. Hearing Test, app for Android. https://play.google.com/store/apps/details?id=mobile.eaudiologia
- Peer, S., and J. J. Fagan. 2015. “Hearing loss in the developing world: Evaluating the iPhone mobile device as a screening tool.” South African Medical Journal = Suid-Afrikaanse Tydskrif Vir Geneeskunde 105 (1):35–39. doi:10.7196/samj.8338.
- Pilsl, U., F. Anderhuber, and B. Rzany. 2012. “Anatomy of the cheek: Implications for soft tissue augmentation.” Dermatologic Surgery 38 (7 Pt 2):1254–1262. doi:10.1111/j.1524-4725.2012.02382.x.
- Reed, N. S., A. Altan, J. A. Deal, C. Yeh, A. D. Kravetz, M. Wallhagen, and F. R. Lin. 2019. “Trends in Health Care Costs and Utilization Associated with Untreated Hearing Loss over 10 Years.” JAMA Otolaryngology- Head & Neck Surgery 145 (1):27–34. doi:10.1001/jamaoto.2018.2875.
- Renda, L., Ö. T. Selçuk, H. Eyigör, Ü. Osma, and M. D. Yılmaz. 2016. “Smartphone based audiometric test for confirming the level of hearing; is it useable in underserved areas?” The Journal of International Advanced Otology 12 (1):61–66. doi:10.5152/iao.2016.1421.
- Sandström, J., D. W. Swanepoel, H. Carel Myburgh, and C. Laurent. 2016. “Smartphone threshold audiometry in underserved primary health-care contexts.” International Journal of Audiology 55 (4):232–238. doi:10.3109/14992027.2015.1124294.
- Sandström, J., D. W. Swanepoel, C. Laurent, G. Umefjord, and T. Lundberg. 2020. “Accuracy and Reliability of Smartphone Self-Test Audiometry in Community Clinics in Low Income Settings: A Comparative Study.” The Annals of Otology, Rhinology, and Laryngology 129 (6):578–584. doi:10.1177/0003489420902162.
- Stevens, G., S. Flaxman, E. Brunskill, M. Mascarenhas, C. D. Mathers, and M. Finucane, Global Burden of Disease Hearing Loss Expert Group 2013. “Global and regional hearing impairment prevalence: An analysis of 42 studies in 29 countries.” European Journal of Public Health 23 (1):146–152. doi:10.1093/eurpub/ckr176.
- Sugawara, Y., S. Otsuka, and S. Nakagawa. 2021. “Estimation of relationships between transducer placements and peripheral propagation in cartilage conduction.” Annual International Conference of the IEEE Engineering in Medicine and Biology Society. IEEE Engineering in Medicine and Biology Society. Annual International Conference 2021:6755–6758. doi:10.1109/EMBC46164.2021.9629860.
- Swanepoel, D. W., H. C. Myburgh, D. M. Howe, F. Mahomed, and R. H. Eikelboom. 2014. “Smartphone hearing screening with integrated quality control and data management.” International Journal of Audiology 53 (12):841–849. doi:10.3109/14992027.2014.920965.
- Thoidis, I., L. Vrysis, K. Markou, and G. Papanikolaou. 2019. “Development and evaluation of a tablet-based diagnostic audiometer.” International Journal of Audiology 58 (8):476–483. Available at: doi:10.1080/14992027.2019.1600204.
- Tseng, Y. C., S. H. Y. Liu, M. F. Lou, and G. S. Huang. 2018. “Quality of life in older adults with sensory impairments: a systematic review.” Quality of Life Research: An International Journal of Quality of Life Aspects of Treatment, Care and Rehabilitation 27 (8):1957–1971. doi:10.1007/s11136-018-1799-2.
- Van Tonder, J., D. W. Swanepoel, F. Mahomed-Asmail, H. Myburgh, and R. H. Eikelboom. 2017. “Automated smartphone threshold audiometry: Validity and time efficiency.” Journal of the American Academy of Audiology 28 (3):200–208. doi:10.3766/jaaa.16002.
- Wasmann, J.-W A., C. P. Lanting, W. J. Huinck, E. A. Mylanus, J. W. M. van der Laak, P. J. Govaerts, D. W. Swanepoel, D. R. Moore, and D. L. Barbour. 2021. “Computational audiology: new approaches to advance hearing health care in the digital age.” Ear & Hearing 42 (6):1499–1507. doi:10.1097/AUD.0000000000001041.
- Wasmann, J.-W., L. Pragt, R. Eikelboom, and D. W. Swanepoel. 2022. “Digital Approaches to Automated and Machine Learning Assessments of Hearing: Scoping Review.” Journal of Medical Internet Research 24 (2):e32581. doi:10.2196/32581.
- World Health Organization 2021. World report on hearing. Geneva: WHO. https://www.who.int/publications/i/item/world-report-on-hearing
- Yeung, J., H. Javidnia, S. Heley, Y. Beauregard, S. Champagne, and M. Bromwich. 2013. “The new age of play audiometry: Prospective validation testing of an iPad-based play audiometer.” Journal of Otolaryngology - Head & Neck Surgery 42 (1):1. doi:10.1186/1916-0216-42-21.