Abstract
Objective
To investigate the reliability of tone-burst auditory brainstem response (TB-ABR) latencies and thresholds in pre-term and full-term infants.
Design
TB-ABRs to 500 Hz and 4000 Hz tone-burst stimuli were recorded at two-week intervals over the first six weeks of life in a group of full-term infants (40–46 weeks gestational age [GA]) and over ten weeks (36–46 weeks gestation) in a group of preterm infants. Linear mixed model analyses evaluated within-subject changes and the effects of the subject group, age at assessment, and stimulus frequency on ABR latency and threshold.
Study sample
Twenty-four infants participated. Nine were full-term (GA: 39–41 weeks) and fifteen were healthy pre-term (GA: 28–34 weeks) at birth.
Results
TB-ABR wave V latencies at 70 dBnHL decreased throughout the study (p < 0.001) in pre-term babies for both test frequencies by approximately 0.5 ms. There were, however, no group or GA (at birth) effects indicating that response latency normalized in these children by the due date. Similarly, TB-ABR threshold levels in the premature group were elevated (p = 0.001) by approximately 5 dB in pre-term evaluation but were equivalent to those of full-term participants in the post-term assessment period.
Conclusions
In healthy, pre-term infants, tone-burst ABR testing is reliable from 36 weeks gestation.
Introduction
Early detection of infant hearing impairment has direct implications for long-term speech and language outcomes (Kennedy et al. Citation2006; Moeller Citation2000; Yoshinaga-Itano et al. Citation1998). Even “minimal” deficits including mild degree- and unilateral hearing loss have been associated with significant psychosocial abnormality, poorer health-related quality of life, and compromised academic performance (Bess et al. Citation1998; Borton et al. Citation2010; Porter et al. Citation2013). Identifying significant hearing loss early relies on an efficient newborn screening program and thorough diagnostic follow-up.
Measurement of evoked responses from the auditory pathway is a commonly used assessment approach for infant subjects. In particular, techniques based on auditory brainstem response (ABR) which is reliably recordable in newborns, have achieved widespread clinical application in the last 40 years. The ABR is a series of scalp-recorded electrical potentials occurring in 10–20 ms immediately following the presentation of an abrupt auditory stimulus such as an acoustic click or tone burst. Identification of the response within the electroencephalogram requires synchronous neural firing in the auditory pathway which is best generated by stimuli with rapid rise times. Acoustic clicks, by their near-instantaneous onset, generate the clearest ABR responses, but contain energy across a broad frequency range and only reflect hearing in the 1000–4000 Hz region (Stapells Citation1994). Similarly, broad-band “CHIRP” stimuli, which are designed to compensate for basilar-membrane-related time delays, may elicit large amplitude brainstem responses but represent the combined response from different frequency regions (Bargen Citation2015). As successful management (and intervention if warranted) requires test stimuli that can provide frequency-specific estimates of sound detection across the audiometric range, acoustic clicks, and broad-band CHIRPs have limited diagnostic value. Higher frequency specificity is offered by tone-burst stimuli (Stapells et al. Citation1990, Citation1995) which show good correspondence between ABR threshold, the lowest presentation level that can elicit a repeatable response, and behavioral hearing levels for a range of test frequencies in both normal and hearing-impaired subjects (Gorga et al. Citation1993; Johnson and Brown Citation2005; Purdy and Abbas Citation2002; Stapells et al. Citation1990, Citation1995).
Tone-burst-elicited ABRs can be recorded to low-level stimuli in healthy, full-term babies. Several studies have shown minimum response levels in normally hearing neonates that are comparable with those seen in older subjects, with mean TB-ABR thresholds typically around 5–15 dBnHL for frequencies across the audiometric range (500 Hz–4000 Hz) (Klein Citation1984; Rance et al. Citation2006; Sininger et al. Citation1997). In contrast, there is currently a lack of research describing TB-ABR result patterns for premature babies tested before their due date.
Hearing assessment in the pre-term period (Spong Citation2013) is desirable for several reasons. Firstly, babies are typically most accessible (i.e. hospital in-patients) during this period and may be discharged before their due date if healthy and stable. Secondly, medical interventions - such as the provision of antiviral medicines for hearing-impaired infants testing positive for cytomegalovirus may be most effective in minimizing hearing loss within this window (Rawlinson et al. Citation2017, Citation2018). Thirdly, parental anxiety about the potential (hearing) consequences of prematurity may be alleviated and finally, early intervention may be initiated if a hearing deficit is identified.
While hearing screening (using automated ABR techniques) is feasible from 34 weeks gestation (Coenraad et al. Citation2011; Jacobson et al. Citation1990), diagnostic assessment in the pre-term period is hampered by the lack of studies supporting feasibility, reliability, and impact of maturation on the response. As a result, hearing evaluation capable of forming a basis for intervention, may be delayed until the infant reaches term or is outside the neonatal period (Coenraad et al. Citation2011; Eggermont and Salamy Citation1988; Lary et al. 1985; Picton et al. Citation1994; Salamy Citation1984).
Changes in ABR thresholds with maturation in premature infants are not well understood. The response can be recorded in babies as early as 25 weeks gestation (Starr et al. Citation1977) and there have been numerous studies describing the course of ABR latency and amplitude development through the pre-term period (Jiang Citation2015; Martins-Ribeiro and Carvallo Citation2008; Pasman et al. Citation1992; Seethapathy et al. Citation2018, Citation2019; Stipdonk et al. Citation2016), but there is relatively little data describing response threshold changes in premature babies tested before their due date. Starr et al. (Citation1977) investigated click-ABR threshold levels for premature infants and found a decrease in minimum response level from around 45 dBnHL for babies tested at 27 weeks post-conceptual age to approximately 20 dBnHL for babies at term. Similarly, Lary et al. (Citation1985) in their cross-sectional sample of premature infants tested in the first week of life, found a progressive decrease in click-ABR thresholds obtained from 27 weeks GA to term with mean thresholds for infants assessed at 28–34 weeks gestation approximately 20 dB higher than those of full-term babies. Likewise, Ping and Jiang (Citation2013) found a linear decrease in click-ABR thresholds with increasing gestational age and a mean threshold approximately 10 dB higher for babies tested at 30 weeks compared to those assessed at term.
Findings for frequency-specific ABR thresholds in the pre-term period are particularly scarce. Martins-Ribeiro and Carvallo (Citation2008), did report that ABRs could be recorded at low presentation levels (20 dBnHL) to a range of tone-burst stimuli in babies tested at 28–35 weeks gestation, but these were not threshold data and potential developmental changes through the pre-term period were not considered.
In this longitudinal study, we sought to track tone-burst ABR latency and threshold changes in individual premature babies through the pre-term and immediate post-term periods. Our study hypotheses were as follows:
TB-ABR latencies in premature babies would be longer and more variable in the pre-term period and would be equivalent to those of the full-term participants by the due date.
TB-ABR thresholds in premature babies would be elevated and more variable in the pre-term period and would be equivalent to those of the full-term participants by the due date.
Materials and methods
Ethics
This study carried approval from the Ethics Committee of the Royal Victorian Eye and Ear Hospital (07/757H). All testing conformed to the tenets of the Declaration of Helsinki (2013) and informed consent was obtained from all participant families after an explanation of the nature, purpose, and expected outcomes of the study.
Participants
A total of 24 babies participated including nine full-term and fifteen pre-term infants. Children were recruited from the special care and well-baby nurseries of the Epworth Freemasons Hospital and Box Hill Hospital in Melbourne, Australia.
Participants were divided into two groups based on gestational age at birth. The full-term infants (four males) were born between 39 and 41 weeks GA (mean: 40 weeks). None of these required special care or had any risk factors for hearing loss. The pre-term participants were born at gestational ages ranging from 28 to 34 weeks (mean: 32 weeks). Each individual in this group (nine males) spent time in the special care nursery, where they received care for relatively mild neonatal health issues. The mean length of stay was 8 days. None suffered a critical illness and, as such, did not require treatment through a neonatal intensive care unit. Children were excluded from the study if they had any risk factors for hearing loss, including hyperbilirubinemia requiring blood transfusion, birth asphyxia requiring resuscitation, congenital abnormalities of the head or neck, infections requiring aminoglycoside administration, any neurological condition, or a family history of childhood hearing loss.
Apparatus and procedures
Infants were assessed at two-week intervals until they were six weeks post-due date. In the full-term group, the first of these tests was carried out 3–6 days after birth with subsequent tests every two weeks (42-, 44-, and 46 weeks gestation). Pre-term infants were also assessed at two-week intervals but over a longer period with assessments at 36-, 38-, 40-, 42, 44- and 46 weeks.
Initial assessment was performed at the hospital either in the special care nursery or in the private hospital room. Subsequent testing was undertaken at the family home. Background noise level recordings (from within the child’s ear canal) were taken at each test occasion to monitor potential environmental effects in the different settings. Measurements were made using a probe tube placed in the ear canal and attached to an external sound level meter as per Rance et al. Citation2006. The mean noise level for the hospital recordings was 51.8 dBSPL and the mean levels for the home assessments ranged from 45.4 to 49.1 dBSPL. Analysis of variance showed no significant difference between test settings.
Otoscopy was completed on each test occasion and normal middle-ear function was confirmed by the presence of transient evoked otoacoustic emission (TEOAE) responses to clicks at 80 dB SPL at each of the data collection points (Owens et al. 2008). An emission was considered present when a response signal-to-noise ratio of >6 dB and a waveform reproducibility of 50% was obtained for at least 3 octave bands.
All of the participants showed evidence of normal hearing at the 4000 Hz test frequency presenting with a repeatable ABR to a 4000 Hz tone-burst presented at ≤20 dBnHL at some point in the recording period. (Tharpe and Seewald Citation2016).
Tone-burst auditory brainstem response
Evoked response measurement was conducted while the infant was in natural sleep, either in a crib or being held by a parent. One ear was randomly selected at the initial assessment and the same ear was subsequently assessed on each occasion. In the full-term group, five babies had their left ear tested and in the pre-term group, nine had their left ear evaluated.
Responses were recorded using the GSI AUDERA evoked response system to 500 Hz and 4000 Hz tone-burst stimuli. Positive polarity Blackman (2:1:2 cycle) tones were presented via ER-3A insert earphones at a rate of 39.09 Hz. Differential recordings were made between electrodes placed on the high forehead (Fz) or vertex (Cz) and the ipsilateral mastoid. A third electrode, placed on either the low forehead or contralateral mastoid, served as a ground. The electroencephalogram in a post-stimulus window of 0–20 ms was recorded and bandpass filtered from 30 Hz to 1.5 kHz. Artifact rejection (set at 25 µV) was employed to avoid response contamination from high EEG levels and myogenic activity.
At each test frequency, a 70 dBnHL stimulus was presented to obtain a clear reference waveform. Response latencies were based on this recording. The stimulus presentation level was then reduced in 20 dB steps until a response was no longer present (determined by the testing audiologist). The approximate threshold was then determined using step sizes of 5 dB around that provisional point. At each presentation level, two runs consisting of averaged responses from 2000 samples were obtained. Post-hoc analysis was carried out by two independent judges experienced in clinical infant diagnostic testing who determined the latency of ABR Wave V and the TB-ABR threshold level. The threshold was defined as the minimum stimulus presentation level required to elicit a repeatable waveform. If there was a discrepancy between the judge-determined thresholds, (in the present study this occurred in <3% of occasions), an average of the two estimates was used in the data analysis.
Statistical analyses
Linear mixed-model analyses of variance were used to analyze the relationship between ABR measures (response latency [Wave V at 70 dBnHL] and threshold) with a random effect for a participant and fixed effects for age at assessment (timepoint), subject group (pre-term versus full-term) and test stimulus frequency (500 Hz tone-burst versus 4000 Hz tone-burst). The model was also adjusted for gestational age at birth as a covariate.
ABR result variability was evaluated using the test for equal variances (Levene’s).
Statistical significance was defined as p < 0.05.
Results
Auditory brainstem response: Wave V latency
Repeatable ABRs were obtained from all participants to acoustic stimuli at 70 dBnHL at each of the data collection points. Averaged response waveforms for a typical participant from the premature cohort are shown in .
Figure 1. Auditory brainstem response waveforms were obtained over the 10-week data collection period for a female participant born at 32 weeks gestation. There are two tracings for every timepoint, each reflecting the averaged waveform from 2000 post-stimulus EEG samples. Waveform morphology and latencies are representative of those seen for the “pre-term” participant group showing improved response definition and decreased response latency throughout the study. Responses were obtained to 500 Hz tone bursts (panel A) and 4000 Hz tone bursts (panel B) presented to the test ear at 70 dBnHL. The arrow markers represent the Wave V peak in the response complex.
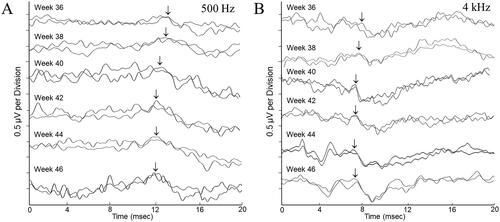
Wave V latencies decreased throughout the study. A mixed-effects analysis of variance revealed a significant effect of timepoint with earlier recordings showing longer latencies (F(5, 185) = 7.48, p < 0.001). Mean wave V latency values for each data collection point are shown in and listed in . There was also a stimulus frequency effect with ABRs elicited by 500 Hz tone bursts significantly longer than those elicited by 4000 Hz stimuli (F(1, 185) = 1822.35, p < 0.001). There were no main effects for subject group (pre-term versus full-term) (F(1, 185) = 0.5, p = 0.829) or gestational age at birth (F(1, 185) = 0.60, p = 0.448) and no interaction effects between timepoint and test frequency (F(5, 185) = 1.07, p = 0.380) or subject group and test frequency (F(1, 185) = 0.38, p = 0.537).
Figure 2. Mean ABR wave V latency values for pre-term and full-term groups show latency decreases over the recording period. Panel A shows findings for a 500 Hz tone-burst stimulus presented to the test ear at 70 dBnHL and panel B shows results for a 4000 Hz stimulus presented to the test ear at 70 dBnHL. “Timepoint” is GA in weeks. Error bars represent the mean ± 1 SD range.
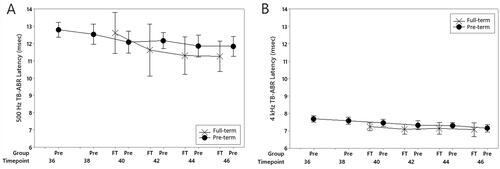
Table 1. Auditory brainstem response Wave V latency means, standard deviations, and standard deviation confidence intervals for each subject group at each data collection point.
The variability of Wave V latency was consistent throughout the study. Tests for equal variance showed equivalent values across study time points for both stimulus frequencies (500 Hz: p = 0.731; 4000 Hz: p = 0.916). Furthermore, there was no latency variance difference between subject groups across time points (p = 0.922) (). In contrast, there was a significant frequency difference with wave V latency estimates more variable for 500 Hz, than 4000 Hz stimuli (500 Hz SD: 0.95 ms; 4000 Hz SD: 0.37 ms, P < 0.001).
Auditory brainstem response threshold
Tone-burst ABR thresholds decreased throughout the study. Analysis of variance revealed a significant timepoint effect with earlier assessments showing higher threshold levels (F(5, 222) = 4.51, p = 0.001). Mean response threshold levels for each test frequency are represented in and listed in . There was also a stimulus frequency effect with ABR thresholds elicited by 500 Hz tone bursts significantly higher than those elicited by 4000 Hz stimuli (F(1, 222) = 637.92, p < 0.001). No main effects were observed for subject group (pre-term versus full-term) (F(1, 222) = 0.17, p = 0.683) or gestational age at birth (F(1, 222) = 0.01, p = 0.923) and no interaction effects between timepoint and test frequency (F(5, 222) = 1.36, p = 0.242) or subject group and test frequency (F(1, 222) = 1.51, p = 0.187).
Figure 3. Mean ABR threshold values for pre-term and full-term groups show minimum response level decreases across the recording period. Panel A shows findings for a 500 Hz tone-burst stimulus and panel B shows results for a 4000 Hz stimulus. “Timepoint” is GA in weeks. Error bars represent the mean ± 1 SD range.
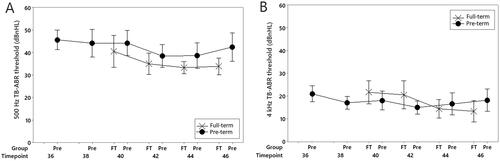
Table 2. Auditory brainstem response threshold means, standard deviations and standard deviation confidence intervals for each subject group at each data collection point.
Tone-burst ABR threshold variability was consistent throughout the study. Tests for equal variance showed equivalent values across study time points for each stimulus frequency (500 Hz: p = 0.731; 4000 Hz: p = 0.916). Furthermore, there was no threshold variance difference between pre-term and full-term subject groups across timepoints (p = 0.219) (). In contrast, response thresholds were more variable for 500 Hz, than for 4000 Hz stimuli (500 Hz SD: 8.8 dB; 4000 Hz SD: 6.9 dB, p = 0.002).
Discussion
The longitudinal results presented in this study indicate developmental changes affecting both ABR latency and threshold in pre-term assessment. Response variability, was, however, equivalent across the recording period suggesting that clinical testing for healthy premature babies will be as reliable in the late pre-term period (36–40 weeks gestational age) as evaluation undertaken post-due date.
Response latency
Auditory brainstem response latency showed a consistent frequency effect with ABRs elicited by the 500 Hz tone-burst showing significantly later Wave V peaks than those evoked by the 4000 Hz stimulus. This finding, which has been reported previously (Martins-Ribeiro & Carvallo Citation2008; Ponton et al. Citation1992; Sininger et al. Citation1997), is likely due (at least in part) to cochlear mechanics. The tonotopic frequency arrangement of the basilar membrane means that low-frequency acoustic inputs are processed more apically than high-frequency and, as such, are relatively delayed due to increased traveling wave times (von Békésy Citation1960).
Wave V latency decreases were observed for both low- and high-frequency stimuli throughout the study. This was particularly evident for recordings obtained from pre-term babies assessed before their due date where mean values were approximately 0.5 ms longer than those obtained at term (). Similar differences have been reported by other authors (Jiang Citation2015; Martins-Ribeiro & Carvallo Citation2008; Pasman et al. Citation1992; Seethapathy et al. Citation2018, Citation2019) who have demonstrated (primarily in cross-sectional data) increases in both absolute Wave V latency and Wave I–V interpeak latency with earlier assessment. Importantly, there was no group difference in our results and no effect of gestational age at birth indicating that peripheral auditory function and/or the efficiency of neural conduction in the auditory nerve/brainstem had normalized by the due date (40 weeks). This result is similar to those reported by Ponton et al. (Citation1992) who found no difference in neural conduction time (ABR I–V interpeak latency) for groups of premature and full-term babies tested at the same gestational age. Our findings are, however, inconsistent with those reported by Stipdonk et al. (Citation2016) whose meta-analysis of published click-ABR latencies suggested neural conduction delays, particularly between peaks III and V, in premature babies tested at term. Methodological differences make direct comparison difficult, but the conflicting results may reflect differences in the study populations. Some of the studies described in the meta-analysis, for example, included children born at 24–28 weeks gestation, where each of the participants in the current study was born at 28+ weeks. Furthermore, the meta-analysis included children who were intensive care unit graduates and found that “neonatal complications” were a significant factor in the rate of auditory neural development. In contrast, none of the infants in our group required treatment in the intensive-care unit.
Auditory brainstem response latency estimates to 500 Hz tone bursts were more variable than those evoked by 4000 Hz tones. This is likely related to the fact that the peaks in the response waveform were less well defined to low-frequency stimuli—see . This phenomenon, which suggests a lesser degree of neural synchrony in the auditory nerve/brainstem, may be stimulus-related. An acoustic click, which has a near-instantaneous onset, maximizes the consistency of neural activity across the stimulus series to produce an averaged ABR. Tonal stimuli, in contrast, require a rise period, which is typically related to the number of cycles in the signal. In this study, we used Blackman tone bursts with a two-cycle rise, so the onset time for the 500 Hz stimulus was 4 ms whereas for the 4000 Hz tone, it was only 0.5 ms. This onset difference results in relatively poorer temporal precision for the low-frequency burst as the point at which the neural activity is triggered is potentially more variable. Alternatively, the relative lack of synchrony to the 500 Hz burst could have been related to cochlear processing, as the traveling wave is slower in the apical turns than the basal which results in a greater temporal spread across the basilar membrane regions contributing to the low-frequency response (Johnston and Boyle Citation1967; von Békésy Citation1960).
In contrast to the frequency results, there were no timepoint, group, or age-at-birth effects on latency variability. This suggests that clinicians can be confident of where to look for a potential ABR waveform within the post-stimulus recording window, regardless of a baby’s degree of prematurity or gestational age—at least for testing carried out at 36 weeks GA or later.
Response threshold
Auditory brainstem response thresholds to 500 Hz tones were significantly higher than those elicited by the 4000 Hz stimuli across the recording period. This consistently reported phenomenon (Hawes and Greenberg, Citation1981; Rance et al. Citation2006; Stapells et al. Citation1995) is also likely a reflection of the relative lack of temporal precision in the neural response to low-frequency sounds, which makes the resulting ABR waveform less distinct and harder to identify within the background EEG at levels close to the psychophysical hearing threshold.
Response thresholds, for both test stimuli, decreased over the recording period with mean thresholds obtained at the 36-week data point approximately 5 dB higher than those observed from the same babies at or post-due date (). There were, however, no group or gestational age-at-birth effects indicating that ABR threshold levels, even in the most premature participants, had normalized by the due date.
There are several possible causes underlying ABR sensitivity changes in the pre-term period. Differences in ear canal size and resonance are one possibility, although had the ear canals been smaller in the pre-term period (which is likely), the equivalent dBSPL levels at the tympanic membrane would have been higher and the resultant ABR thresholds relatively lower than later recordings (Rance et al., Citation2006; Sininger et al., Citation1997).
Cochlear immaturity in the pre-term period is another conceivable explanation. The human cochlea is anatomically mature at birth (Bredberg Citation1968; Lavigne-Rebillard and Pujol Citation1987) and studies of otoacoustic emission tuning curves (Abdala et al. Citation1996) and basilar membrane traveling wave times (Eggermont et al. Citation1991) have indicated maturity of the cochlear mechanical mechanisms at term. Less is known about cochlear physiology in the pre-term period, but Gkoritsa et al. (Citation2007) have shown normal transient OAE amplitudes in babies tested at 30–36 weeks post-conceptual age suggesting that a cochlear contribution to the elevated ABR thresholds in our pre-term data is improbable.
A more likely explanation for ABR threshold elevation in the pre-term period is the immaturity of the auditory neural system. Unlike the cochlea, the brainstem pathways are not mature at term. This can be most clearly seen in response latency. ABR wave I latencies are adult-like soon after birth in full-term infants (Eggermont and Salamy Citation1988) but absolute wave V and wave I–V interpeak latencies decrease over the first 2–3 years of life (Salamy Citation1984). Furthermore, as discussed previously, latency findings in the pre-term period suggest significant changes in the efficiency of neural conduction in the auditory nerve/brainstem.
Axonal and/or synaptic immaturity may explain both latency and threshold differences in the pre-term ABR findings. Myelination of auditory axons is present throughout the pathway by 29 weeks gestation, but the density of myelin sheath continues to increase throughout the 1st year of life (Moore et al., Citation1995). Myelin affects the speed of neural conduction and its developmental course is therefore likely to be a key factor in the maturation of ABR latencies. Similarly, post-natal synaptogenesis is thought to be a factor in the development of ABR peak latencies (Ponton et al. Citation1996). In addition to affecting the speed of neural conduction, both myelination and synaptic efficiency can impact the synchrony of neural firing in the auditory nerve/brainstem (for a review Rance and Starr Citation2015). In extreme (pathological) cases, this can mean that the ABR is unrecordable (i.e. auditory neuropathy presentation), but normal developmental changes could result in synchrony improvements over time which could, in turn, affect the precision of the ABR waveform and its detectability at low amplitudes when close to the EEG noise floor. Development of the accuracy of neural firing patterns in the pre-term ABR could therefore result in elevated threshold levels in pre-term testing.
The variability of ABR thresholds was consistent throughout the study. This finding is important for clinical assessment as the reliability of hearing level prediction from the ABR is determined by the consistency of the evoked response/hearing threshold relationship (Picton et al. Citation2005; Rance et al. Citation1995). That is, in clinical testing, the average ABR sensation level can be subtracted to determine the most likely hearing threshold, but how confident the clinician can be with that prediction is determined by the range of behavioral hearing levels that can correspond to a particular ABR threshold. In this study, standard deviations for our group of (presumed) normally hearing participants ranged from 5–10 dB (500 Hz stimulus) to 4–8 dB (4000 Hz) stimulus which is similar to those reported in previous studies involving newborns tested post-term (Rance et al. Citation2006).
Study limitations
A particular limitation of this study is that the participants were not followed up with a behavioral hearing assessment. All had normal TEOAE responses on each test occasion, so there was no evidence of middle ear abnormality, and normal 4000 Hz TB-ABR thresholds (≤20 dBnHL) on at least one test occasion, so it was presumed that they all had hearing within the normal range—but exact hearing levels were not established. As such, we do not know the precise ABR sensation levels in each case. Furthermore, while there was no evidence to suggest hearing level fluctuation, it was assumed that each child’s hearing was stable throughout the study and that any changes to ABR latency and threshold were the result of developmental processes.
Another limitation involves the study assessment range (36–46 weeks GA). Reliable ABR testing may be possible in premature babies before 36 weeks GA, but we were unable to access study participants before this date due to Special Care Unit policies at the participating hospitals.
A final possible limitation is the sample size. Our longitudinal study design limited the number of babies who could participate. Although each participant was evaluated on several occasions and the project included data from 126 separate test sessions, the fact that we had only 24 subjects may limit the generalization of the findings.
Conclusions
The results of this study suggest that diagnostic hearing assessment based on tone-burst ABRs is feasible in healthy, premature babies from at least 36 weeks gestation. While response threshold levels were marginally higher in pre-term assessment, variability across participants was equivalent at each of the pre- and post-term data collection points meaning that hearing level estimation would be equally reliable at any point from 36- to 46 weeks gestational age. The range of observed ABR thresholds for the 4000 Hz stimulus was only 10–25 dB for each data point suggesting that normal hearing and mild hearing loss could be differentiated at this frequency. Results at 500 Hz were more variable, with threshold ranges of 15–35 dB, suggesting that ABR testing at this frequency would struggle to differentiate between normal hearing and mild degree loss, but this low-frequency shortcoming, which has been reported previously for full-term neonates (Rance et al. Citation2006; Sininger et al. Citation1997), was a consequence of test technique limitations rather than a reflection of participant immaturity. As such, the findings of this study suggest that frequency-specific quantification of hearing is possible in the pre-term period and that intervention options (amplification, etc.) may be considered with the same degree of confidence as for children tested beyond their due date.
Author contributions
GR: conception and design of the study; acquisition and analysis of data; drafting a significant portion of the manuscript/figures. DT: acquisition and analysis of data; drafting a significant portion of the manuscript/figures. KG: acquisition and analysis of data; drafting a significant portion of the manuscript/figures.
Acknowledgments
The authors would like to thank the families who participated and the staff from the maternity units at the Freemasons and Box Hill Hospitals, Melbourne, Australia.
Disclosure statement
No potential conflict of interest was reported by the authors.
Additional information
Funding
References
- Abdala, C., Y. S. Sininger, M. Ekelid, and F. G. Zeng. 1996. “Distortion Product Otoacoustic Emission Suppression Tuning Curves in Human Adults and Neonates.” Hearing Research 98 (1-2):38–53. https://doi.org/10.1016/0378-5955(96)00056-1.
- Bargen, G. A. 2015. “Chirp-evoked auditory brainstem response in children: a review.” American Journal of Audiology 24 (4):573–583. https://doi.org/10.1044/2015_AJA-15-0016.
- von Békésy. G. 1960. Experiments in hearing. Edited by Wever EG. New York: McGraw-Hill.
- Bess, F. H., J. Dodd-Murphy, and R. A. Parker. 1998. “Children with Minimal Sensorineural Hearing Loss: Prevalence, Educational Performance, and Functional Status.” Ear and Hearing 19 (5):339–354. https://doi.org/10.1097/00003446-199810000-00001.
- Borton, S. A., E. Mauze, and J. E. Lieu. 2010. “Quality of Life in Children with Unilateral Hearing Loss: A Pilot Study.” American Journal of Audiology 19 (1):61–72. https://doi.org/10.1044/1059-0889(2010/07-0043).
- Bredberg, G. 1968. “Cellular Pattern and Nerve Supply of the Human Organ of Corti.” Acta Otolaryngologica (Stockh) 236:1–135.
- Coenraad, S., A. Goedegebure, and L. J. Hoeve. 2011. “An Initial Overestimation of Sensorineural Hearing Loss in NICU Infants After Failure on Neonatal Hearing Screening.” International Journal of Pediatric Otorhinolaryngology 75 (2):159–162. https://doi.org/10.1016/j.ijporl.2010.10.026.
- Coenraad, S., M. S. Toll, H. L. Hoeve, and A. Goedegebure. 2011. “Auditory Brainstem Response Morphology and Analysis in Very Preterm Neonatal Intensive Care Unit Infants.” The Laryngoscope 121 (10):2245–2249. https://doi.org/10.1002/lary.22140.
- Eggermont, J. J., C. W. Ponton, S. G. Coupland, and R. Winkelaar. 1991. “Maturation of the Traveling‐Wave Delay in the Human Cochlea.” The Journal of the Acoustical Society of America 90 (1):288–298. https://doi.org/10.1121/1.401299.
- Eggermont, J. J., and A. Salamy. 1988. “Maturational Time Course for the ABR in Preterm and Full Term Infants.” Hearing Research 33 (1):35–47. https://doi.org/10.1016/0378-5955(88)90019-6.
- Gkoritsa, E., S. Korres, I. Psarommatis, M. Tsakanikos, N. Apostolopoulos, and E. Ferekidis. 2007. “Maturation of the Auditory System: 1. Transient Otoacoustic Emissions as an Index of Inner Ear Maturation: Maduración del sistema auditivo: 1. Emisiones otoacusticas evocadas por transitorios como índice de maduración del oído interno.” International Journal of Audiology 46 (6):271–276. https://doi.org/10.1080/14992020701261397.
- Gorga, M. P., J. R. Kaminski, K. L. Beauchaine, and B. M. Bergman. 1993. “A Comparison of Auditory Brain Stem Response Thresholds and Latencies Elicited by Air-and Bone-Conducted Stimuli.” Ear and Hearing 14 (2):85–94. https://doi.org/10.1097/00003446-199304000-00003.
- Hawes, M. D., and H. J. Greenberg. 1981. “Slow Brain Stem Responses (SN10) to Tone Pips in Normally Hearing Newborns and Adults.” Audiology 20 (2):113–122. https://doi.org/10.3109/00206098109072689.
- Jacobson, J. T., C. A. Jacobson, and R. C. Spahr. 1990. “Automated and Conventional ABR Screening Techniques in High-Risk Infants.” Journal of the American Academy of Audiology 1 (4):187–195.
- Jiang, Z. D. 2015. “A Longitudinal Study of Brainstem Auditory Response from Birth to Late Term in Late Preterm Babies and Abnormal Findings in High-Risk Babies.” Journal of Perinatal Medicine 43 (6):769–776. https://doi.org/10.1515/jpm-2014-0203.
- Johnson, T. A., and C. J. Brown. 2005. “Threshold Prediction Using the Auditory Steady-State Response and the Tone Burst Auditory Brain Stem Response: A Within-Subject Comparison.” Ear and Hearing 26 (6):559–576. https://doi.org/10.1097/01.aud.0000188105.75872.a3.
- Johnstone, B. M., and A. J. F. Boyle. 1967. “Basilar Membrane Vibration Examined with the Mössbauer Technique.” Science (New York, N.Y.)158 (3799):389–390. https://doi.org/10.1126/science.158.3799.389.
- Kennedy, C. R., D. C. McCann, M. J. Campbell, C. M. Law, M. Mullee, S. Petrou, P. Watkin, S. Worsfold, H. M. Yuen, and J. Stevenson. 2006. “Language Ability After Early Detection of Permanent Childhood Hearing Impairment.” The New England Journal of Medicine 354 (20):2131–2141. https://doi.org/10.1056/NEJMoa054915.
- Klein, A. J. 1984. “Frequency and Age-Dependent Auditory Evoked Potential Thresholds in Infants.” Hearing Research 16 (3):291–297. https://doi.org/10.1016/0378-5955(84)90118-7.
- Lary, S., G. Briassoulis, L. de Vries, L. M. Dubowitz, and V. Dubowitz. 1985. “Hearing Threshold in Preterm and Term Infants by Auditory Brainstem Response.” The Journal of Pediatrics 107 (4):593–599. https://doi.org/10.1016/s0022-3476(85)80030-5.
- Lavigne-Rebillard, M., and R. Pujol. 1987. “Surface Aspects of the Developing Human Organ of Corti.” Acta Oto-Laryngologica Supplementum 436 (sup436):43–50. https://doi.org/10.3109/00016488709124975.
- Martins Ribeiro, F., and R. Mamede Carvallo. 2008. “Tone-Evoked ABR in Full-Term and Preterm Neonates with Normal Hearing.” International Journal of Audiology 47 (1):21–29. https://doi.org/10.1080/14992020701643800.
- Moeller, M. P. 2000. “Early Intervention and Language Development in Children Who are Deaf and Hard Of Hearing.” Pediatrics 106 (3):e43-e43–e43. https://doi.org/10.1542/peds.106.3.e43.
- Moore, J. K., L. M. Perazzo, and A. Braun. 1995. “Time Course of Axonal Myelination in the Human Brainstem Auditory Pathway.” Hearing Research 87 (1-2):21–31. https://doi.org/10.1016/0378-5955(95)00073-d.
- Owens, J. J., M. J. McCoy, B. L. Lonsbury-Martin, and G. K. Martin. 1992. “Influence of Otitis Media on Evoked Otoacoustic Emissions in Children.” Seminars in Hearing 13 (01):53–65. https://doi.org/10.1055/s-0028-1085141.
- Pasman, J. W., J. J. Rotteveel, R. de Graaf, D. F. Stegeman, and Y. M. Visco. 1992. “The Effect Of Preterm Birth on Brainstem, Middle Latency and Cortical Auditory Evoked Responses (BMC AERs).” Early Human Development 31 (2):113–129. https://doi.org/10.1016/0378-3782(92)90039-j.
- Picton, T. W., A. Dimitrijevic, M. C. Perez-Abalo, and P. Van Roon. 2005. “Estimating Audiometric Thresholds Using Auditory Steady-State Responses.” Journal of the American Academy of Audiology 16 (3):140–156. https://doi.org/10.3766/jaaa.16.3.3.
- Picton, T. W., A. Durieux-Smith, and L. M. Moran. 1994. “Recording Auditory Brainstem Responses from Infants.” International Journal of Pediatric Otorhinolaryngology 28 (2-3):93–110. https://doi.org/10.1016/0165-5876(94)90001-9.
- Ping, L. L., and Z. D. Jiang. 2013. “Changes in Brainstem Auditory Response Threshold in Preterm Babies From Birth to Late Term.” Acta Oto-Laryngologica 133 (6):607–611. https://doi.org/10.3109/00016489.2012.762115.
- Ponton, C. W., J. J. Eggermont, S. G. Coupland, and R. Winkelaar. 1992. “Frequency‐Specific Maturation of the Eighth Nerve and Brain‐Stem Auditory Pathway: Evidence from Derived Auditory Brain‐Stem Responses (ABRs).” The Journal of the Acoustical Society of America 91 (3):1576–1586. https://doi.org/10.1121/1.402439.
- Ponton, C. W., J. K. Moore, and J. J. Eggermont. 1996. “Auditory Brain Stem Response Generation by Parallel Pathways: Differential Maturation of Axonal Conduction Time and Synaptic Transmission.” Ear and Hearing 17 (5):402–410. https://doi.org/10.1097/00003446-199610000-00006.
- Porter, H., D. P. Sladen, S. B. Ampah, A. Rothpletz, and F. H. Bess. 2013. “Developmental Outcomes in Early School-Age Children with Minimal Hearing Loss.” American Journal of Audiology 22 (2):263–270. https://doi.org/10.1044/1059-0889(2013/13-0013).
- Purdy, S. C., and P. J. Abbas. 2002. “ABR Thresholds to Tonebursts Gated with Blackman and Linear Windows in Adults with High-Frequency Sensorineural Hearing Loss.” Ear and Hearing 23 (4):358–368. https://doi.org/10.1097/00003446-200208000-00011.
- Rance, G., F. W. Rickards, L. T. Cohen, S. De Vidi, and G. M. Clark. 1995. “The Automated Prediction of Hearing Thresholds in Sleeping Subjects Using Auditory Steady-State Evoked Potentials.” Ear and Hearing 16 (5):499–507. https://doi.org/10.1097/00003446-199510000-00006.
- Rance, G., and A. Starr. 2015. “Pathophysiological Mechanisms and Functional Hearing Consequences of Auditory Neuropathy.” Brain: a Journal of Neurology 138 (Pt 11):3141–3158. https://doi.org/10.1093/brain/awv270.
- Rance, G., D. Tomlin, and F. W. Rickards. 2006. “Comparison of Auditory Steady-State Responses and Tone-Burst Auditory Brainstem Responses in Normal Babies.” Ear and Hearing 27 (6):751–762. https://doi.org/10.1097/01.aud.0000240491.68218.ed.
- Rawlinson, W. D., S. B. Boppana, K. B. Fowler, D. W. Kimberlin, T. Lazzarotto, S. Alain, K. Daly, S. Doutré, L. Gibson, M. L. Giles, et al. 2017. “Congenital Cytomegalovirus Infection in Pregnancy and the Neonate: Consensus Recommendations for Prevention, Diagnosis, and Therapy.” The Lancet. Infectious Diseases 17 (6):e177–e188. https://doi.org/10.1016/S1473-3099(17)30143-3.
- Rawlinson, W. D., P. Palasanthiran, B. Hall, L. Al Yazidi, M. J. Cannon, C. Cottier, W. J. van Zuylen, and M. Wilkinson. 2018. “Neonates with Congenital Cytomegalovirus and Hearing Loss Identified Via the Universal Newborn Hearing Screening Program.” Journal of Clinical Virology 102:110–115. https://doi.org/10.1016/j.jcv.2018.03.006.
- Salamy, A. 1984. “Maturation of the Auditory Brainstem Response From Birth Through Early Childhood.” Journal of Clinical Neurophysiology 1 (3):293–329. https://doi.org/10.1097/00004691-198407000-00003.
- Seethapathy, J., P. Boominathan, A. K. Uppunda, and B. Ninan. 2018. “Auditory Brainstem Response in Very Preterm, Moderately Preterm and Late Preterm Infants.” International Journal of Pediatric Otorhinolaryngology 111:119–127. https://doi.org/10.1016/j.ijporl.2018.06.006.
- Seethapathy, J., P. Boominathan, A. K. Uppunda, and B. Ninan. 2019. “Changes in Auditory Brainstem Response in Very Preterm and Late Preterm Infants.” International Journal of Pediatric Otorhinolaryngology 121:88–94. https://doi.org/10.1016/j.ijporl.2019.03.008.
- Sininger, Y. S., C. Abdala, and B. Cone-Wesson. 1997. “Auditory Threshold Sensitivity of the Human Neonate as Measured by the Auditory Brainstem Response.” Hearing Research 104 (1-2):27–38. https://doi.org/10.1016/s0378-5955(96)00178-5.
- Spong, C. Y. 2013. “Defining “Term” Pregnancy: Recommendations from the Defining “Term” Pregnancy Workgroup.” JAMA 309 (23):2445–2446. https://doi.org/10.1001/jama.2013.6235.
- Stapells, D. R. 1994. “Low-Frequency Hearing and the Auditory Brainstem Response.” American Journal of Audiology 3 (2):11–13. https://doi.org/10.1044/1059-0889.0302.11.
- Stapells, D. R., J. S. Gravel, and B. A. Martin. 1995. “Thresholds for Auditory Brain Stem Responses to Tones in Notched Noise from Infants and Young Children with Normal Hearing or Sensorineural Hearing Loss.” Ear and Hearing 16 (4):361–371. https://doi.org/10.1097/00003446-199508000-00003.
- Stapells, D. R., T. W. Picton, A. Durieux-Smith, C. G. Edwards, and L. M. Moran. 1990. “Thresholds for Short-Latency Auditory-Evoked Potentials to Tones in Notched Noise in Normal-Hearing and Hearing-Impaired Subjects.” Audiology 29 (5):262–274. https://doi.org/10.3109/00206099009072857.
- Stipdonk, L. W., N. Weisglas‐Kuperus, M. C. J. Franken, K. Nasserinejad, J. Dudink, and A. Goedegebure. 2016. “Auditory Brainstem Maturation In Normal‐Hearing Infants Born Preterm: A Meta‐Analysis.” Developmental Medicine and Child Neurology 58 (10):1009–1015. https://doi.org/10.1111/dmcn.13151.
- Starr, A., R. N. Amlie, W. H. Martin, & S. Sanders. 1977. Development of Auditory Function in Newborn Infants Revealed by Auditory Brainstem Potentials. Pediatrics 60 (6):831–839.
- Tharpe, A. M., & Seewald, R. (Eds.) 2016. Comprehensive Handbook of Pediatric Audiology. Plural Publishing.
- Yoshinaga-Itano, C., A. L. Sedey, D. K. Coulter, and A. L. Mehl. 1998. “Language of Early-and Later-Identified Children with Hearing Loss.” Pediatrics 102 (5):1161–1171. https://doi.org/10.1542/peds.102.5.1161.