Abstract
Poultry litter on agricultural lands could introduce nitrogen (N), phosphorus (P), heavy metals in soil and ground water. Native vegetations were identified to assess efficacy for phytoremediation of nutrients and metals from soil and water. Objective was to measure capability of multi-year native species to remove metals, nutrients, and prevent Nitrate-N leaching below the rooting zone. Treatments were distributed in four replicates with/without fertilization. Suction lysimeters were installed at 30, 60, and 90-cm depths in 3 of 4 replicates. Species were identified, recorded, five specified cuttings sampled. Plant, soil, water samples were prepared and analyzed by spectroscopy. Nitrate-N extraction, nitrates in water samples were determined using flow injection analysis. Fertilized plots (NVM) had 39% more biomass yield than unfertilized plots (NVN). In plants, nutrient and metal concentrations varied significantly with 14% increase in Zn, 36% and 26% in K and Mg over NVN for first and second year. Uneven between NVM and NVN, topsoil had higher values for most nutrients and metals. Largest P and (NO3−)−N in plant and water were observed from NVM. Cultivation of native vegetation appears to be an effective approach for remediation of excess nitrates-N, P, heavy metals from surface and sub-surface zones of the soil.
Graphical Abstract
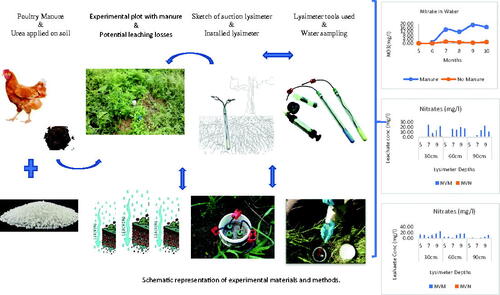
NOVELTY STATEMENT
Native vegetation has been used for soil fertility, specific reasons like the removal of pesticides or agrochemicals, and other chemical related exposures. Studies on the use of native vegetation for phytoremediation on agricultural lands are uncommon. This research looked at the capability of native vegetation of different species as a viable tool for the remo+val of excess nutrients and heavy metals from agricultural lands. Results indicated native vegetation can take up significant amounts of excess nutrients from soils, proportional to their biomass accumulation. Native Vegetation was therefore found to be a nutrient sink, capable of removing excess nutrients/metals from the soil.
Introduction
In agricultural production systems, different cropping practices are employed for various reasons, notably for agricultural and environmental sustainability. The bush-fallow has been categorized as an agronomic system in which soil fertility is maintained by allowing native vegetation to regrow following several years of cropping (Robertson and Harwood Citation2013). For decades, the fallow systems have been utilized universally for various purposes. Mediterranean agriculture (Karlen et al. Citation1994), regions in Asia and Africa (Ryan et al. Citation2008), the West, used fallow for refurbishing the soil for next planting season (Bado and Bationo Citation2018), improve yield (Hauchhum and Tripathi Citation2020), reduce pest infestations (Campbell et al. Citation2017) and improve N fixation (Kearney et al. Citation2019). Fallow systems have evolved over the years in different formats including intentional and unintentional fallow processes where specific vegetations are planted to achieve desired objectives. These include the use of cover crops (Schmidt et al. Citation2018; Runck et al. Citation2020), a two, three or multi- crop rotations (Browne et al. Citation2004) and even multifunctional cropping systems that incorporate different combinations of herbaceous crops, trees, and animals within a single farm unit (Lovell et al. Citation2018). These systems have the goal of improving soil fertility for crop productivity (Haque et al. Citation2019) as well as the added potential of increased soil carbon sequestration and reduced greenhouse emissions (Partey et al. Citation2017). Even a practice designated as summer fallow, though not generally tolerated in all regions has been shown to be beneficial for dryland agriculture in arid and semi-arid regions (Malhi et al. Citation2012; Stewart and Thapa Citation2016).
In many instances, land applications of manure and fertilizer are used to improve soil fertility, which could result in accumulations leading to off-site transport of phosphorus (P) and leaching of nitrogen (N). These applications could contaminate surface, sub-surface, and ground waters (DeLaune et al. Citation2004; Read Citation2012), causing eutrophication of waterways. There is therefore a need to circumvent this problem. While nutrient leaching depends on several factors including soil properties, fertilizer types and agronomic practices (Lawniczak et al. Citation2016), the types of crops and their fertilizer requirements could generate the release of N. The problems of P could be reduced by conservative agronomic practices that improve the health of the soil. The addition of other soil amendments such as alum, (Dao Citation1999; Codling et al. Citation2000) or fly ash and biochar (Dou et al. Citation2003; Codling and Jaja Citation2022) assist in restricting erosion and other off-site transport. Nitrogen, particularly soluble nitrate (NO3−) however dissolves easily in percolating waters and leaches to sub-surface horizons, causing water quality problems. Due to the general chemistry and possible interactions of nitrates within the soil, approaches that could remediate excess in situ soil NO3− levels are necessary to address environmental concerns related to water quality problems.
As concerns about the environment increase, the interest in the preservation and restoration of native plant communities increases as well. Native plants are valued for their economic, ecological, genetic, and esthetic benefits in addition to the growing societal belief in their intrinsic value as living species (Dorner Citation2002). Nielsen and Calderon (Citation2011) discussed a review of various methods and combinations of fallow and their effects on production parameters such as organic matter, soil structure, nutrient availability, and soil microbial activity among others. They concluded that the application of fallow on these factors generally produce positive effects on soil quality for crop production (Nielsen and Calderon Citation2011). Native plants are known for ecosystem restoration, providing numerous benefits. Several wild plants like Artemisia vulgaris L., Galium mollugo L. and Stellaria holostea L., as well as Lotus corniculatus L., Plantago lanceolata L., Medicago lupulina L., and other macrophytes have been used for remediation (Antoniadis et al. Citation2021; Matanzas et al. Citation2021). Additional benefits include promotion of associations of mycorrhizae with plant roots that may improve uptake of nutrients, presence of invertebrates with woody debris, and pollen sources for pollinators (Dorner Citation2002). In a study of seasonal biomass and nutrient uptake with cereal species/cultivars, maximum biomass accumulation rate was 204–232 kg ha−1 d−1, and maximum uptake rate of N, and P was 3.2–5.7 and 0.30–0.60 kg ha−1 d−1 (Malhi et al. Citation2006), suggesting sufficient supply of nutrients from soil and fertilizers. In a soybean study, each kilogram increase in yield, total dry matter accumulation, and total N uptake increased by 1.45 kg, and 0.054 kg, but all varied by environment at any specific yield level, whereas N removal did not (Gaspar et al. Citation2017). Removing heavy metals through harvestable biomass has been indicated to be an efficient technique for inorganic pollutants (Vamerali et al. Citation2010; Yan et al. Citation2020).
While cultivated native vegetation as intentional fallow has been utilized on degraded or marginal lands for cleanup of trace metals due to mining, on industrial and military training lands (Busby et al. Citation2020; Salmani-Ghabeshi et al. Citation2021), there are limited instances where non-cultivated native vegetations are used on agricultural lands for uptake of heavy metals or excess nutrients. Research and experimental studies for fallow plants or native vegetation tested for the purposes of remediation of manure impacted soils or the removal of excess nutrients from sub-surface horizons are not readily available. Therefore, based on the benefit of native vegetation in areas of soil fertility build-up, increased crop production and probable nutrients/metal accumulation and uptake, the objective of this research is to evaluate the efficacy of unintentional native vegetation of various species for remediation of excess nutrients in both surface and sub-surface horizons. We hypothesize that native vegetation of various species would have the effectiveness to remediate excess nutrients and heavy metals in an agricultural land with poultry manure applications.
Materials and methods
Site description and preparation
The experiment was conducted at Randolph Farm of Virginia State University located in the Tri-Cities area of Central Virginia (37.1°N; 77.3°W) at an elevation of 45 m above sea level. The soil type at the site is a Bourne series fine sandy loam (mixed, semiactive, thermic Typic Fragiudults) (US-NRCS Citation2014). The research field is an area, conventionally prepared and divided into eight individual plots of 96 m2 (0.008 hectare). Each plot has a buffer stripe of about 3 m wide and a total of four replicates for the two treatments set on a completely randomized design (CRD).
The treatments which included native vegetation plus poultry litter (NVM) and native vegetation minus poultry litter (NVN) were randomly established within the field plots in the spring. Soil Solution Access Tube (SSAT) suction lysimeters that were constructed with a porous ceramic cup and connected to 30 cm, 60 cm, and 90 cm transparent tubes were installed at 30, 60, and 90 cm depths in 3 of 4 plots per treatment. A rubber hose extended from the plastic syringe (#DS-50CC) through the tube, into the round bottom porous ceramic cup (Irrometer, Company, Inc. CA).
A combination of poultry litter and urea (CO(NH2)2) (46.0.0) was split-applied annually to achieve a plant available N (PAN) rate of 200 kg·ha−1 (140 kg from litter and 60 kg from urea) to all the plots except the control plots. This provided an application of 65 kg of poultry litter and 1.04 kg of urea per plot for the first year, 47 kg and 1.04 kg of poultry litter and urea respectively for the second year. Prior to the determination of the plant available nitrogen (PAN), the poultry litter was analyzed, as well as the initial soil conditions, then used to calculate the nutrient need for the experimental layout (). Irrigation was provided as needed based on weather and visual inspection across the plots. The necessary agronomic attributes of the native vegetation including the relative amounts of the different species were monitored and recorded monthly through the study (). The Cumulative growing degree days (GDDs, °C) were calculated using the average temperature of each day from planting to end of study, using a base temperature of 8 °C. The positive values from planting to the end were added to obtain the cumulative GDDs (Yang et al. Citation1995; Zhou and Wang Citation2018).
Table 1. Initial soil and poultry litter nutrient and heavy metal properties.
Table 2. Recurrent dominant plant species on experimental plots over the first and second year.
Field sampling, preparation and analyses
Water
Water samples were collected monthly from the suction lysimeters for the entire season. The samples were stored in labeled polyethylene bottles, preserved in iced coolers, and transported to the laboratory. The samples were filtered with sterilized plastic syringes and membrane filters (0.45 µm) (Thermo Fisher Scientific, USA), employing one per sample, and acidified with sulfuric acid (H2SO4) as a preservative for the determination of nitrate-N and ammonia ion concentrations using the Lachat instrument (QuikChem 8000 Series by Lachat Instruments, USA). The pH and EC were determined with non-filtered and non-acidified samples.
Plants/biomass
Biomass harvests was completed five times for the year. The initial sample was collected by randomly releasing a 1 m quadrate to the plots and manually harvesting a 4–6-inch stubble. Afterwards, on a bimonthly basis, subsequent samples were harvested from this same spot. The fresh biomass weight was recorded; subsample was collected and oven-dried at 60 °C to constant weight for the determination of dry matter. The dry samples were ground in a cyclone mill (Retsch Cyclone Mill Twister 220–240 V, 50/60 Hz, USA, Germany) to pass a 1-mm-screen for tissue concentration analyses. Elemental concentrations in plant tissues were determined using the dry ashing method 3.014(a), whereby 2 g of each sample was heated at 480 °C for 16 h, then digested using concentrated nitric acid (HNO3) and 3 M hydrochloric acid (HCl). The digest was filtered through Whatman No. 40 filter paper, and the filtrate brought to a volume of 25 mL with 0.1 M HCl (AOAC Citation1984; Codling Citation2019). The concentration of Ca, Cu, Fe, K, Mg, Mn, P, Pb and Zn in each extract was analyzed using the Inductively Coupled Plasma Optical Emission Spectroscopy (ICP—PerkinElmer Avio 500 ICP OES, USA). Total N for plant samples was determined using the Perkin Elmer (Series 11 2400) CHNS/O Analyzer, USA.
The native vegetation plots were subjected to monthly specie identification to capture the different specie emergence and the growth habit of the native vegetations. The specie emergence though seasonal, were not necessarily time dependent because some species emerged faster than others.
Soils
Soil samples were collected and composited from the 0–15 cm and 15–30 cm depths of individual treatment plots 90 days after the spring manure and fertilizer applications. The samples were air-dried and crushed to pass a 2-mm sieve. Soil pH was measured in a 1:2 (material: deionized water, v/v) suspension after equilibration using a glass electrode. Electrical conductivity (EC) was determined in a 1:2 (material: deionized water, v/v) suspension using an Orion conductivity meter (Rhoades Citation1996; Thomas Citation1996). Mehlich-3 extractable nutrients and metals (Ca, Cu, Fe, K, Mg, Mn, P, Pb and Zn) were determined as described by (Sparks Citation1996). After extractions, samples were analyzed using inductively coupled plasma-optical emission spectroscopy (ICP-OES).
All soil and plant samples analyzed were processed with blanks and respective reference materials for quality assurance purposes. Apple leaf standards from the National Institute of Standards and Technology (NIST), Gaithersburg, MD were used for the plant samples while in-house standard samples were used for the soil samples. The recovery percentage from the plant samples ranged from 100% to 77% for all the elements analyzed while our results for the soil samples were within the established percentage range of 80–100% for the in-house standard soil.
Data analyses
Analysis of variance (ANOVA) was used to determine statistically significant differences among treatments (SAS Institute Citation2021). Separation of means was performed using Duncan’s multiple range tests at p ≤ 0.05 (Steel and Torrie Citation1980).
Results and discussion
Precipitation and weather conditions
The rainfall data presented in shows the total monthly precipitation (mm) and mean temperature (°C) of the study area for the duration of the experiment and growing seasons. The monthly precipitation at the site was lower in the first year and increased slightly in the second year to a high of about 237 mm, while mean temperature was higher for the first year than the last year, with a range from −1. 87 °C to about 28 °C (). The rate of plant growth and development depends on the temperature around the plant, though each species has a specific temperature range (Hatfield and Prueger Citation2015) and might be affected differently. While the precipitation at the site could be insufficient for growth, it was supplemented with drip irrigation through the study.
Figure 1. Monthly precipitation (mm) and mean temperature (oC) records of the experimental region during the study and growing seasons (usclimatedata.com).
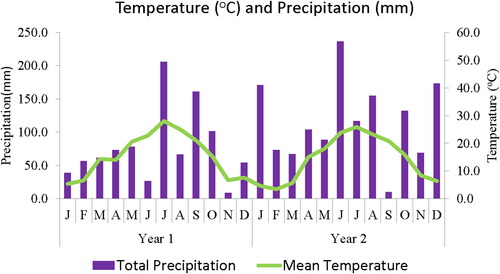
The cumulative growing degree days ((GDDs) °C) calculated for the experimental area (), showed the effect of temperature, and indicated that the first year of the study was warmer than the second year. This could contribute to a greater biomass yield irrespective of a greater precipitation in the second year.
Biomass yield, tissue nutrient and trace metal concentration
The fertilized treatments (NVM) had an average of 39% and 33% increase of biomass yield over the unfertilized (NVN) for the first and second year respectively (). While the biomass yield showed a significant difference for the 2 years between the fertilized and non-fertilized native vegetation, there were no significant differences for the nutrient and heavy metal concentrations of the plant tissues for all the elements analyzed for the first year apart from Zn which had a significant 14% increase over the unfertilized native vegetation. The second year however, showed a significant difference and increase of 36%, 26% for K and Mg for the fertilized plants as well as a decrease of 11% for Zn for the unfertilized plants.
Table 3. Means of biomass, and nutrient and metal uptake by Native Vegetation with (NVM) and without manure (NVN) for five cuttings.
The biomass from five different bimonthly harvests annually shown in () did indicate a consistent pattern among the five months of sampling between the NVM and NVN in that the first measurements were low, then increased and decreased again. While this seemed to be the pattern, it was not even, as the warm summer months produced more growth which often slowed toward the fall with subsequent harvests. Despite the general variations or lack of a pattern, NVM had more biomass than NVN in most cases with the second harvest from NVM having the most yield and was significantly higher than its first harvest by 70%, and to its last harvest by 88% but higher than the most yield from NVN by 41%. The harvests for the second year indicated that the fourth harvest was significantly higher for both treatments but with NVM being significantly greater than NVN by 36%. Regrowth was minimal for the last two harvests from the first year and the early two harvests from the second year for NVM and NVN but especially with NVN. The nutrient and heavy metal concentrations for the 2 years also did not show consistent differences between NVM and NVN. While the nutrient and metal concentrations did not appear to show any clear difference between NVM and NVN, there were also no clear relationships between the nutrient and metal concentrations, and biomass weights for the individual harvests (). Nutrient and heavy metal concentrations did significantly vary among the harvest dates, however. This might be due to individual plant species which have different physiological characteristics and could develop specific element composition and concentrations. The study also showed that total plant uptake for the major nutrient were 317.36 kg, 1235.89 kg, 118.25 kg and 156.55 kg for Ca, K, Mg and P respectively while both the essential and non-essential micronutrients (Cempel and Nikel Citation2006; Fasani et al. Citation2018) had plant uptake of 0.445 kg, 3.026 kg, 1.664 kg, 1.390 kg, 0.025 kg and 0.020 kg for Cu, Fe, Mn, Zn, Pb and Cd respectively (Supplementary Table).
Table 4. Biomass, and nutrient and metal uptake for five bi-monthly harvests.
Remarkably, the nutrients and heavy metal concentrations of the plant tissues were comparative to that of the poultry litter applied () except for the cadmium (Cd) concentrations. There was hardly any detectable Cd in the soil while that of the poultry litter was about a ratio of 1:4 the concentration in the plants in the first year and 1:3 in the second year. This might be a specific case of Cd which is more mobile and bioavailable than other metals, thus generally higher in plants than the soil. Other studies have found that the content of Cd in agricultural soil is known to be relatively low (Clemens and Ma Citation2016), as Cd has high mobility, and said to accumulate in leaves at high quantities (Yanqun et al. Citation2005; Oliveira et al. Citation2022). Leafy greens, particularly of the family Asteraceae like lettuce and endive have a relatively high potential for Cd uptake and translocation and are thus considered Cd accumulators (Baldantoni et al. Citation2016; Ismael et al. Citation2019). Several native vegetations identified in the study are from this Asteraceae family. Cd is also known to interfere with mineral nutrients in plants such as Zn, Fe, Ca, Mn, Mg, Cu, Si, and K (Jinadasa et al. Citation2016; Ismael et al. Citation2019) and prevent normal growth and yield.
In general, it was reported that when the bioavailable Cd concentration becomes >0.001 mg kg−1, or the Cd concentration in plant tissue reaches 3–30 mg kg−1, most plants exhibit visible Cd toxicity symptoms (Solís-Domínguez et al. Citation2007; Dutta et al. Citation2020). While the results did not show any obvious Cd toxicity, there are indications that stunted plant growth under Cd stress may be due to several reasons, as it negatively affects water and nutrient uptake, photosynthesis, carbon and nitrogen assimilation, oxidative damage, disrupts plant metabolism, and inhibits plant morphology and physiology (Ismael et al. Citation2019; Sterckeman and Thomine Citation2020; Haider et al. Citation2021). However, from a phytoremediation perspective, the actual plant removal efficiency of any nutrient or heavy metal could be a result of the plant density and rate of elemental accumulations in the harvestable part of the plants (Jaja et al. Citation2022). Studies indicated that removing heavy metals through harvestable biomass is an efficient technique for inorganic pollutants (Vamerali et al. Citation2010; Yan et al. Citation2020).
The total N concentration presented in shows the difference between the NVM having a higher and significant level of N with a range of 3.30–1.83% than the NVN with a range of 3.26–1.10% particularly for the first year. The second year followed a similar trend but had lower levels of N than the first year. The annual mean is 2.18% and 1.90% for NVM as well as 2.07% and 1.25% for NVN for the first and second years respectively. The higher levels were evident for the fourth and fifth harvests for the first year and the first two harvests for the second year. These variations could be reminiscent of the varied plant species exhibiting different physiological attributes.
Table 5. Total Nitrogen (N) concentration in plants for Native Vegetation without Manure (NVN) and Native vegetation with Manure (NVM) for the first and second year.
Native vegetation includes range of plant species and relative frequency () signifying the abundance to moderate abundance, widespread or commonplace, average to below average occurrence and rarity of species observed (). These vegetations differ at physiological, biochemical, and genetic levels (Shtangeeva et al. Citation2011) and might result in contrasting quantitative distribution of different macro and trace metals in the plants for different harvest times. These different species were recurrent for the specific months and seasons, which presented different genotypes and possibly the demands for mineral elemental supply and uptake or transport and metabolism. There are differences in the mineral nutrition of plants (Epstein and Bloom Citation2005) suggesting that different plants growing in the same place could show different concentrations of trace and macro elements (Bourret et al. Citation2009) and this scenario might be irrespective of the elements’ presence in the soil.
Soil analyses, changes in nutrient and heavy metal concentrations
Soil analyses from 0–15 cm and 15–30 cm depths are shown in and . The pH of the soil samples ranged from 6.39 to 5.36 and 6.10 to 5.62 for the first and second year from the NVM and NVN respectively. The electrical conductivity (EC) measurements were 0.08–0.06 mS/cm and 0.085–0.046 mS/cm for the same plots for the 3 years considered. These measurements registered slight but significant differences between the NVM and NVN plots. The nutrients and heavy metals did not register any significant treatment difference for the two years except for Mg which was 27% higher with the NVM in the second year. The individual depths for the top and subsoils (), indicated no difference in pH and EC between the NVM and NVN except for pH in the second year which was slightly lower for NVN. The concentrations of the nutrients and heavy metals was also uneven but almost all the nutrients and metals had a higher value for the topsoil. Cadmium concentration, though substantial in the plant samples, did not reach the instrument detectable limits in the soil samples. Therefore, the elemental composition of the soil does not reflect that of the plant tissue samples. Individual plant species could develop specific element composition and concentrations rather than reflect the nutrients available in the soil (Markert Citation1989) especially with a collection of different plant species in one location. In occasions where the pH is low or rather high, indicating an acidic or alkaline soil, there could be an immobilization of heavy metals or a mineralization process where the metals are easily released into the soil for uptake by plants (Yan et al. Citation2020; Adamczyk-Szabela and Wolf Citation2022). The pH from this research was not considered within the range expected to have a major impact on the mobility of heavy metal.
Table 6. Soil pH, EC, and concentration of nutrients with (NVM) and without (NVN) poultry manure.
Table 7. Soil pH, EC, and Nutrients as a function of soil depth in soils treated with and without poultry manure.
Generally, the soil analyses showed a higher ammonium concentration than nitrate () by treatments and year. Though not significant, the nitrates and ammonium concentrations were slightly higher from the NVM plots than that of NVN. The higher NH4+ concentration over the NO3− could suggest sorption and the tendency of ammonium to be adsorbed due to cation exchange capacity and protected from leaching. This situation could be irrespective of the land use such as agricultural lands with manure and non- agricultural lands. Moreover, urea was applied as a fertilizer source which could inherently elevate the levels of ammonia in the soil in both treatments.
Table 8. Nitrate and Ammonium concentrations in the manure and no-manure treated soils.
Water analyses, nitrate and ammonium concentrations
The analyses of water samples from the lysimeter depths of 30 cm, 60 cm, and 90 cm are shown in . The concentration of nitrate and ammonium in water samples for NVM and NVN plots are shown in and . The 2-year experiment indicated a higher level of nitrates and ammonium in the fertilized plots particularly with the samples collected within the last four months of sampling in the first year. The first two months of sampling did not show any difference for the first year. Subsequently, the concentration for the lysimeter leachate from the fertilized plots was higher all through the months of sampling. This could be due to leaching losses of NO3- from the poultry manure applied as an N source of fertilizer. The U.S. Environmental Protection Agency (US-EPA Citation2022) standard for nitrate in drinking water is 10 mg/L of nitrate. The results indicate almost double the allowable limit in the water collected within the first 60 cm depth of the lysimeters and does not affect the ground water levels beyond the 90 cm depth. The high nitrate level could be due to leaching since heavy rainfall and irrigation might have contributed to N leaching. As a breakdown product of poultry manure, urea exhibits dynamics which correspond to the temperature and moisture conditions of the soil. In a normal scenario, with an adequate soil moisture in the presence of enzymes and microbes, NH3 which is the initial urea product from the breakdown will quickly convert to NH4+ and finally to NO3− for plant uptake. However, there are situations when NH4+ could be removed from the soil mainly via nitrification, volatilization, and leaching, which is harmful to the environment and has led to a series of agroecological issues (Ding et al. Citation2016). It has been indicated that the volatilization of NH3 is directly proportional to the concentration of ammonium (NH4+) in soil solution (Shaviv and Mikkelsen Citation1993), which is also associated with the formation of N2O and high nitrate (NO3−) concentrations in soil (Jenkinson Citation1990; Wang et al. Citation2021). Therefore, minimizing the accumulation of soil inorganic N (NH4+, NO3−, etc.) or manure which contributes to their accumulation in soils could reduce N2O emissions. This is with the understanding that concentrations of NO3− and NH4+ in soils are regulated by numerous factors, such as soil temperature, pH, soil microbiology, fertilizer form, and moisture (Schmidt Citation1982; Nieder et al. Citation2011) among others.
Figure 3. (a) Concentration of Nitrate in Water samples for fertilized and non-fertilized plots for Year 1. (b) Concentration of Ammonium in Water samples for fertilized and non-fertilized plots for Year 1.
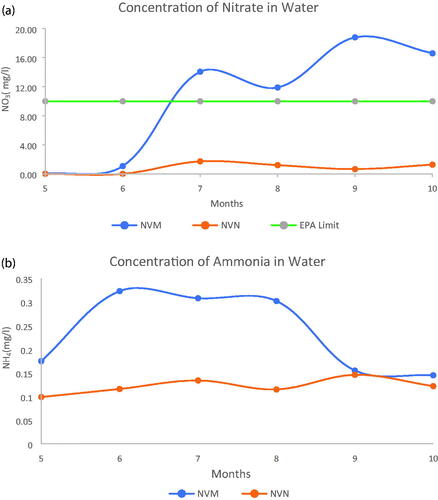
Figure 4. (a) Concentration of Nitrate in Water samples for fertilized and non-fertilized plots for Year 2. (b) Concentration of Ammonium in Water samples for fertilized and non-fertilized plots for Year 2.
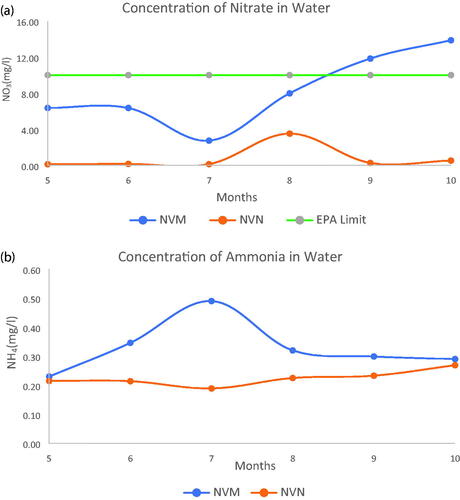
Figure 5. (a) Lysimeter samples for Monthly Nitrate Concentration for Year 1. (b) Lysimeter samples for Monthly Nitrate Concentration for Year 2.
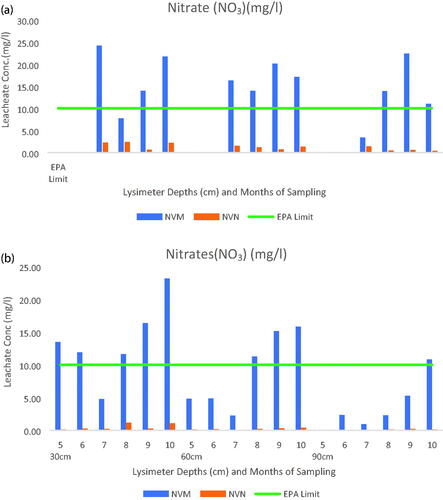
Only the leachates for nitrates from the NVM and NVN were further compared as shown in focusing on the depths and months of sampling. This confirmed the analysis in and that nitrate leaching was much higher in fertilized plots and within the 30 cm depth except for the first two months of the first year of experiment. The concentration of nitrates was in the order of 30 cm >60 cm >90 cm but in the second year, even the first months of sampling had higher concentrations of nitrates possibly due to carry-over or build up from the previous year of poultry litter and fertilizer applications as well as weather conditions. Comparatively, the nitrate concentrations reduced within the 90 cm depth as opposed to the 60 cm depth and the 30 cm depth.
Again, the concentrations of NO3− and NH4+ in soils are regulated by numerous factors, such as soil temperature, pH, soil microbiology, fertilizer form, and moisture (Schmidt Citation1982; Tong et al. Citation2018) that could have contributed to the results.
Conclusions
The 2-year experiment looked at the efficacy of recurrent native vegetation plant species in an agricultural setting to serve as a tool for remediation of excess nutrients and heavy metals from manure applications. There were significant differences in some elements between the soil depths and treatments but there were no obvious trends for the elemental concentrations of the nutrients and heavy metals in the soil. There was higher biomass yield and higher concentrations of several nutrients as well as heavy metals in the fertilized and manure amended plots. Since the elemental concentrations in the soil between NVM and NVN plots were not different, the native vegetations seem to hold onto nutrients. The water samples exhibited a higher concentration of nitrates in the fertilized plots over the course of the research and reduced concentration of nitrates in the sub-surface horizons, suggesting the possibility of the native vegetation as a tool for phytoremediation.
NVM and NVN species had roughly the same elemental concentrations and levels of nutrient uptake but higher in NVM mostly because the NVM had larger biomass production. Therefore, it appears that native vegetation can remove and hold unto excess nutrients and metals from manure and fertilizer amended soil in agricultural lands. This could prove to be beneficial based on the economics of more expensive methods of remediation. While this study had more vegetation from the plant family of Asteraceae, Amaranthaceae, Brassicaceae, Plantaginaceae and Poaceae, the anticipation is that any regional native vegetation would yield an effective result. It also suggests the needed advantage of providing soil cover which prevents soil erosion naturally, and nitrates from leaching down sub-surface horizons. However, there is a need to properly calculate the PAN for each crop being considered to prevent an over-application of manure as well as the use of controlled release urea when applicable. The importance of understanding the accumulation of nitrates and ammonium under different fertilization treatments will also help to minimize nitrogen losses while maximizing its use efficiency.
Supplemental Material
Download MS Word (15.4 KB)Acknowledgments
The authors wish to thank Michael Brandt for assisting in field preparation and collection of experimental samples. The assistance of Mebrat Gesese analyzing the samples collected for this experiment is appreciated.
Disclosure statement
No potential conflict of interest was reported by the author(s).
Additional information
Funding
References
- [AOAC] Association of Official Analytical Chemists 1984. Official methods of analysis. 14th ed. Washington (DC): AOAC. p. 249–252.
- [SAS] Statistical Analysis System 2021. SAS 9.4 help and documentation. Cary (NC): SAS Institute Inc.
- [US-EPA] United States -Environmental Protection Agency. 2022. National primary drinking water regulations. [accessed 2022 Dec 9]. https://www.epa.gov/ground-water-and-drinking-water/national-primary-drinking-water-regulations
- [US-NRCS] United States-Natural Resources Conservation Service. 2014. Keys to soil taxonomy. Washington (DC): Government Printing Office.
- Adamczyk-Szabela D, Wolf WM. 2022. The impact of soil pH on heavy metals uptake and photosynthesis efficiency in Melissa officinalis, Taraxacum officinalis, Ocimum basilicum. Molecules. 27(15):4671–4671. doi:10.3390/molecules27154671.
- Antoniadis V, Shaheen SM, Stärk HJ, Wennrich R, Levizou E, Merbach I, Rinklebe J. 2021. Phytoremediation potential of twelve wild plant species for toxic elements in a contaminated soil. Environ Int. 146:106233. doi:10.1016/j.envint.2020.106233.
- Bado VB, Bationo A. 2018. Integrated management of soil fertility and land resources in Sub-Saharan Africa: involving local communities. Adv Agronomy. 150:1–33. doi:10.1016/bs.agron.2018.02.001.
- Baldantoni D, Morra L, Zaccardelli M, Alfani A. 2016. Cadmium accumulation in leaves of leafy vegetables. Ecotoxicol Environ Saf. 123:89–94. doi:10.1016/j.ecoenv.2015.05.019.
- Bourret MM, Brummer JE, Leininger WC. 2009. Establishment and growth of two willow species in a riparian zone impacted by mine tailings. J Environ Qual. 38(2):693–701. doi:10.2134/jeq2008.0153.
- Browne GT, Schneider SM, Trout TJ. 2004. Effects of pre-plant fallow and crop rotations on severity of Prunus replant disease. Phytopathology. 94:S11–S12.
- Busby RR, Douglas TA, LeMonte JJ, Ringelberg DB, Indest KJ. 2020. Metal accumulation capacity in indigenous Alaska vegetation growing on military training lands. Int J Phytoremediation. 22(3):259–266. doi:10.1080/15226514.2019.1658708.
- Campbell JW, Smithers C, Irvin A, Kimmel CB, Stanley-Stahr C, Daniels JC, Ellis JD. 2017. Trap nesting wasps and bees in agriculture: a comparison of sown wildflower and fallow plots in Florida. Insects. 8(4):107. doi:10.3390/insects8040107.
- Cempel M, Nikel G. 2006. Nickel: a review of its sources and environmental toxicology. Pol J Environ Stud. 15:375–382.
- Clemens S, Ma JF. 2016. Toxic heavy metal and metalloid accumulation in crop plants and foods. Annu Rev Plant Biol. 67:489–512. doi:10.1146/annurev-arplant-043015-112301.
- Codling EE. 2019. Effects of phosphorus amended low phosphorus soil on soybean (Glycine max L.) and wheat (Titicum aestivum L.) yield and phosphorus uptake. J Plant Nutr. 42(8):891–899. doi:10.1080/01904167.2019.1589506.
- Codling EE, Chaney RL, Mulchi CL. 2000. Use of aluminum‐and iron‐rich residues to immobilize phosphorus in poultry litter and litter‐amended soils. J Environ Qual. 29(6):1924–1931. doi:10.2134/jeq2000.00472425002900060027x.
- Codling EE, Jaja N. 2022. Effects of amendments on soil chemical properties, alfalfa yield and nutrient uptake. J Plant Nutr. 45(1):33–48. doi:10.1080/01904167.2021.1943678.
- Dao TH. 1999. Coamendments to modify phosphorus extractability and nitrogen/phosphorus ratio in feedlot manure and composted manure. J Environ Qual. 28(4):1114–1121. doi:10.2134/jeq1999.00472425002800040008x.
- DeLaune PB, Moore PA, Jr, Carman DK, Sharpley AN, Haggard BE, Daniel TC. 2004. Development of a phosphorus index for pastures fertilized with poultry litter—factors affecting phosphorus runoff. J Environ Qual. 33(6):2183–2191. doi:10.2134/jeq2004.2183.
- Ding J, Jiang X, Ma M, Zhou B, Guan D, Zhao B, Zhou J, Cao F, Li L, Li J. 2016. Effect of 35 years inorganic fertilizer and manure amendment on structure of bacterial and archaeal communities in black soil of northeast China. Appl. Soil Ecol. 105:187–195. doi:10.1016/j.apsoil.2016.04.010.
- Dorner J. 2002. An introduction to using native plants in restoration projects. Bureau of Land Management. [accessed 2022 Dec 5]. https://www.ser-rrc.org/resource/an-introduction-to-using-native/https://www.fs.fed.us/wildflowers/Native_Plant_Materials/documents/intronatplant.pdf
- Dou Z, Zhang GY, Stout WL, Toth JD, Ferguson JD. 2003. Efficacy of alum and coal combustion by-products in stabilizing manure phosphorus. J Environ Qual. 32(4):1490–1497. doi:10.2134/jeq2003.1490.
- Dutta A, Patra A, Jatav HS, Jatav SS, Singh SK, Sathyanarayana E, Verma S, Singh P. 2020. Toxicity of cadmium in soil-plant-human continuum and its bioremediation techniques. In: Larramendy ML, Soloneski S, editors. Soil contamination - threats and sustainable solutions. IntechOpen. p. 59–80. doi:10.5772/intechopen.94307. London (UK): IntechOpen Limited.
- Epstein E, Bloom AJ. 2005. Mineral nutrition of plants: principles and perspectives. 2nd ed. Sunderland (MA): Sinauer Associates.
- Fasani E, Manara A, Martini F, Furini A, DalCorso G. 2018. The potential of genetic engineering of plants for the remediation of soils contaminated with heavy metals. Plant Cell Environ. 41(5):1201–1232. doi:10.1111/pce.12963.
- Gaspar AP, Laboski CA, Naeve SL, Conley SP. 2017. Dry matter and nitrogen uptake, partitioning, and removal across a wide range of soybean seed yield levels. Crop Sci. 57(4):2170–2182. doi:10.2135/cropsci2016.05.0322.
- Haider FU, Liqun C, Coulter JA, Cheema SA, Wu J, Zhang R, Wenjun M, Farooq M. 2021. Cadmium toxicity in plants: impacts and remediation strategies. Ecotoxicol Environ Saf. 211:111887. doi:10.1016/j.ecoenv.2020.111887.
- Haque MM, Biswas JC, Islam MR, Islam A, Kabir MS. 2019. Effect of long-term chemical and organic fertilization on rice productivity, nutrient use-efficiency, and balance under a rice-fallow-rice system. J Plant Nutr. 42(20):2901–2914. doi:10.1080/01904167.2019.1659338.
- Hatfield JL, Prueger JH. 2015. Temperature extremes: effect on plant growth and development. Weather Clim Extremes. 10:4–10. doi:10.1016/j.wace.2015.08.001.
- Hauchhum R, Tripathi SK. 2020. Impact of rhizosphere microbes of three early colonizing annual plants on improving soil fertility during vegetation establishment under different fallow periods following shifting cultivation. Agric Res. 9(2):213–221. doi:10.1007/s40003-019-00422-w.
- Ismael MA, Elyamine AM, Moussa MG, Cai M, Zhao X, Hu C. 2019. Cadmium in plants: uptake, toxicity, and its interactions with selenium fertilizers. Metallomics. 11(2):255–277. doi:10.1039/c8mt00247a.
- Jaja N, Codling EE, Rutto LK, Timlin D, Reddy VR. 2022. Poultry litter and inorganic fertilization: effects on biomass yield, metal and nutrient concentration of three mixed-season perennial forages. Agronomy. 12(3):570. doi:10.3390/agronomy12030570.
- Jenkinson DS. 1990. An introduction to the global nitrogen cycle. Soil Use Manag. 6(2):56–61. doi:10.1111/j.1475-2743.990.tb00800.x.
- Jinadasa N, Collins D, Holford P, Milham PJ, Conroy JP. 2016. Reactions to cadmium stress in a cadmium-tolerant variety of cabbage (Brassica oleracea L.): is cadmium tolerance necessarily desirable in food crops? Environ Sci Pollut Res Int. 23(6):5296–5306. doi:10.1007/s11356-015-5779-6.
- Karlen DL, Varvel GE, Bullock DG, Cruse RH. 1994. Crop rotations for the 21st century. Adv Agron. 53:1–45.
- Kearney LJ, Dutilloy E, Rose TJ. 2019. Nitrogen fixation in summer-grown soybean crops and fate of fixed-N over a winter fallow in subtropical sugarcane systems. Soil Res. 57(8):845–850. doi:10.1071/SR19044.
- Lawniczak AE, Zbierska J, Nowak B, Achtenberg K, Grześkowiak A, Kanas K. 2016. Impact of agriculture and land use on nitrate contamination in groundwater and running waters in central-west Poland. Environ Monit Assess. 188(3):1–17. doi:10.1007/s10661-016-5167-9.
- Lovell ST, Dupraz C, Gold M, Jose S, Revord R, Stanek E, Wolz KJ. 2018. Temperate agroforestry research: considering multifunctional woody polycultures and the design of long-term field trials. Agroforest Syst. 92(5):1397–1415. doi:10.1007/s10457-017-0087-4.
- Malhi SS, Johnston AM, Schoenau JJ, Wang ZL, Vera CL. 2006. Seasonal biomas acumulación and nutrient uptake of wheat, barley, and oat on a Black Chernozem soil in Saskatchewan. Can J Plant Sci. 86(4):1005–1014. doi:10.4141/P05-116.
- Malhi SS, Sahota TS, Gill KS. 2012. Potential of management practices and amendments for preventing nutrient deficiencies in field crops under organic cropping systems. In: Bullar GS, Bhullar NK, editors. Agricultural sustainability: progress and prospects in crop research. Acadamic Press. p. 77. doi:10.1016/B978-0-12-404560-6.00005-8. Ontario (Canada): Canadian Science Publishing.
- Markert B. 1989. Multi-element analysis in ecosystems: basic conditions for representative sampling of plant materials. Z Anal Chem. 335(6):562–565. doi:10.1007/BF00474249.
- Matanzas N, Afif E, Día TE, Gallego JR. 2021. Phytoremediation potential of native herbaceous plant species growing on a paradigmatic brownfield site. Water Air Soil Pollut. 232:1–14. doi:10.1007/s11270-021-05234-9.
- Nieder R, Benbi DK, Scherer HW. 2011. Fixation and defixation of ammonium in soils: a review. Biol Fertil Soils. 47(1):1–14. doi:10.1007/s00374-010-0506-4.
- Nielsen DC, Calderón FJ. 2011. Fallow effects on soil. In: Hatfield JL, Sauer TJ, editors. Soil management: building a stable base for agriculture. Madison (WI): SSSA. p. 287–300. doi:10.2136/2011.soilmanagement.c19.
- Oliveira BRM, De Almeida AAF, de Almeida Santos N, Pirovani CP. 2022. Tolerance strategies and factors that influence the cadmium uptake by cacao tree. Sci Hortic. 293:110733. doi:10.1016/j.scienta.2021.110733.
- Partey ST, Zougmoré RB, Ouédraogo M, Thevathasan NV. 2017. Why promote improved fallows as a climate-smart agroforestry technology in sub-Saharan Africa? Sustainability. 9(11):1887. doi:10.3390/su9111887.
- Read JJ. 2012. Spring nitrogen fertilization of ryegrass–bermudagrass for phytoremediation of phosphorus‐enriched soils. Agron J. 104(4):908–916. doi:10.2134/agronj2011.0385.
- Rhoades JD. 1996. Salinity: electrical conductivity and total dissolved solids. In: Sparks DL, editor. Methods of soil analysis. Madison (WI): SSSA. p. 417–435.
- Robertson GP, Harwood RR. 2013. Agriculture, sustainable. In: S. A. Levin, editor. Encyclopedia of biodiversity. 2nd ed. Academic Press. p. 111–118. ISBN 9780123847201. doi:10.1016/B978-0-12-384719-5.00287-2. ScienceDirect. Elsevier B.V.
- Runck BC, Khoury CK, Ewing PM, Kantar M. 2020. The hidden land use cost of upscaling cover crops. Commun Biol. 3(1):1–4. doi:10.1038/s42003-020-1022-1.
- Ryan J, Singh M, Pala M. 2008. Long-term cereal-based rotation trials in the Mediterranean Region: implications for cropping sustainability. Adv Agron. 97:273–319. doi:10.1016/S0065-2113(07)00007-7.
- Salmani-Ghabeshi S, Fadic-Ruiz X, Miró-Rodríguez C, Pinilla-Gil E, Cereceda-Balic F. 2021. Trace element levels in native plant species around the industrial site of Puchuncaví-Ventanas (Central Chile): evaluation of the phytoremediation potential. Appl Sci. 11(2):713. doi:10.3390/app11020713.
- Schmidt EL. 1982. Nitrification in soil. Nitr Agric Soils. 22:253–288. doi:10.2134/agronmonogr22.c7.
- Schmidt R, Gravuer K, Bossange AV, Mitchell J, Scow K. 2018. Long-term use of cover crops and no-till shift soil microbial community life strategies in agricultural soil. PloS ONE. 13(2):e0192953. doi:10.1371/journal.pone.0192953.
- Shaviv A, Mikkelsen RL. 1993. Controlled-release fertilizers to increase efficiency of nutrient use and minimize environmental degradation—a review. Nutr Cycl Agroecosyst. 35(1–2):1–12. doi:10.1007/BF00750215.
- Shtangeeva I, Steinnes E, Lierhagen S. 2011. Macronutrients and trace elements in rye and wheat: similarities and differences in uptake and relationships between elements. Environ Exp Bot. 70(2–3):259–265. doi:10.1016/j.envexpbot.2010.09.013.
- Solís-Domínguez FA, González-Chávez MC, Carrillo-González R, Rodríguez-Vázquez R. 2007. Accumulation and localization of cadmium in Echinochloa polystachya grown within a hydroponic system. J Hazard Mater. 141(3):630–636. doi:10.1016/j.jhazmat.2006.07.014.
- Sparks DL. 1996. Mehlich III method. In: Sparks DL, editor. Methods of soil analysis. Madison (WI): SSSA. p. 739–768.
- Steel RGD, Torrie JH. 1980. Duncan’s new multiple range test. Principles and procedures of statistic. New York: McGraw-Hill. p. 187–88.
- Sterckeman T, Thomine S. 2020. Mechanisms of cadmium accumulation in plants. Crit Rev Plant Sci. 39(4):322–359. doi:10.1080/07352689.2020.1792179.
- Stewart BA, Thapa S. 2016. Dryland farming: concept, origin and brief history. In: Farooq M, Siddique K, editors. Innovations in dryland agriculture. Springer, Cham. p. 3–29. doi:10.1007/978-3-319-47928-6.
- Thomas GW. 1996. Soil pH and soil acidity. In: Sparks DL, editor. Methods of soil analysis. Madison (WI): SSSA. p. 475–490.
- Tong X, He X, Duan H, Han L, Huang G. 2018. Evaluation of controlled release urea on the dynamics of nitrate, ammonium, and its nitrogen release in black soils of Northeast China. IJERPH. 15(1):119. doi:10.3390/ijerph15010119.
- Vamerali T, Bandiera M, Mosca G. 2010. Field crops for phytoremediation of metal-contaminated land. A review. Environ Chem Lett. 8(1):1–17. doi:10.1007/s10311-009-0268-0.
- Wang C, Amon B, Schulz K, Mehdi B. 2021. Factors that influence nitrous oxide emissions from agricultural soils as well as their representation in simulation models: a review. Agronomy. 11(4):770. doi:10.3390/agronomy11040770.
- Yan A, Wang Y, Tan SN, Mohd Yusof ML, Ghosh S, Chen Z. 2020. Phytoremediation: a promising approach for revegetation of heavy metal-polluted land. Front Plant Sci. 11:359. doi:10.3389/fpls.2020.00359.
- Yang S, Logan J, Coffey DL. 1995. Mathematical formulae for calculating the base temperature for growing degree days. Agric for Meteorol. 74(1–2):61–74. doi:10.1016/0168-1923(94)02185-M.
- Yanqun Z, Yuan L, Jianjun C, Haiyan C, Li Q, Schvartz C. 2005. Hyper accumulation of Pb, Zn and Cd in herbaceous grown on lead-zinc mining area in Yunnan, China. Environ Int. 31(5):755–762. doi:10.1016/j.envint.2005.02.004.
- Zhou G, Wang Q. 2018. A new nonlinear method for calculating growing degree days. Sci Rep. 8(1):10149. doi:10.1038/s41598-018-28392-z.