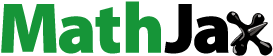
Abstract
The disposal of fly ash (FA) from coal power plants polluting the air, soil, and groundwater is a major environmental concern. Phytoremediation to rehabilitate fly ash dumpsites is a promising alternative but has practical concerns about the disposal of harvested biomass. This study investigated the effect of supplementing fly ash with fresh sewage sludge (FSS), aged sewage sludge, food waste, and compost (COM) to enhance the phytoremediation potential of Napier grass and its subsequent utilization for ethanol production. The highest removal of Mn (1196.12 g ha−1) and Ni (128.06 g ha−1) from FA could be obtained when Napier is grown in the presence of FSS and inorganic fertilizer (NPK). In addition, the highest bioethanol yield (19.31 g L−1) was obtained from Napier grown in fly ash with COM + NPK, thus providing additional economic benefits aside from the remediation process. Given the significant levels of heavy metals present in the pulp and bio-slurry after ethanol production, further research is required in this area to determine the best ways to utilize this waste such as converting it into biochar.
NOVELTY STATEMENT
Using energy crops as a phytoremediation agent for fly ash dumpsites has the potential to remediate heavy metal contamination and provide additional economic benefits. Napier grass was able to tolerate high concentrations of heavy metals and yield high biomass in fly ash in the presence of organic amendments. The harvested biomass was successfully converted into substrate for bioethanol production using heavy metal-tolerant yeast. This is the first report on the production of ethanol from the phytoremediation biomass of Napier grass.
Introduction
Fly ash (FA), a fine dust by-product of coal thermal power plants, is one of the major coal combustion wastes of environmental concern. The composition and the extent of its potential impact are dependent on the nature and properties of the combusted coal, the combustion process, and the recovery of the FA (Pandey et al. Citation2016). Fly ash contains considerable amount of macro-nutrients needed by plant such as calcium (Ca), magnesium (Mg), iron (Fe), manganese (Mn), boron (B), sodium (Na), and minimum amount of phosphorus (P) and potassium (K), while nitrogen (N) contained is not available for plant take, as it occurs in heterocyclic compounds (Antonkiewicz et al. Citation2022). On the other hand, fly ash also contains many hazardous heavy metals, including lead (Pb), nickel (Ni), chromium (Cr), Arsenic (As), and others (Antonkiewicz Citation2010). The number and concentration of heavy metals in fly ash are influenced by the mineralogy, particle size distribution, and combustion temperature of the source material being burned. The concentration of these minerals and elements found in FA is usually higher than its concentration in the parent coal (Baba et al. Citation2008). It also contains the particulates that float up with the flue gases after the combustion of coal (Dwivedi and Jain Citation2014; Van der Merwe et al. Citation2014). Worldwide, coal power plants currently produce roughly 1.2 billion tons of fly ash each year (Yadav et al. Citation2022). Of these billions of fly ash only about 64% is utilized in the production of cement, wallboard, concrete, and bricks (Zierold and Odoh Citation2020). In Botswana, about 672,000 tons of FA per annum are produced of which 70% is not reused due to a lack of technological advancements (Malumbela and Masuku Citation2017). A large amount of FA is disposed of in dumpsites, and in most cases, these dumps are ignored, left uncovered, and devoid of vegetation, resulting in air pollution from the dispersion of fine FA particles, contamination of ground water through leaching, polluting soils, and disruption of plant community structure (Zhai et al. Citation2009; Gajaje et al. Citation2021). Therefore, proper management of disposed FA is of urgent need, as it harms the environment and causes human health hazards. Fly ash dumpsite can be physically contained and stabilized with several biological interventions which include the use of organic amendments and the use of suitable plant species for phytoremediation. Sewage sludge is one of the biological amendments highly recommended to amend ash as it contains high amounts of phosphorus and sodium which are needed for plant growth. A study conducted by Antonkiewicz (Citation2010) indicated that sewage sludge applied at an application rate of 200 t ha−1 on a fly ash dumpsite improved the yield of legume grass by 130% compared to the control.
Desirable characteristics of plants suitable for phytostabilization of FA dumpsite include fast-growing plants, extensive root systems, high biomass-producing capability, ability to tolerate and accumulate high amounts of heavy metals (Couselo et al. Citation2012; Pandey et al. Citation2016). Pennisetum purpureum Schumach commonly known as Napier grass or Elephant grass, popularly used for forage or fodder is a potential plant species for phytoremediation (Osman et al. Citation2020). This is due to its fast-growing characteristics, high biomass yield, high effective photosynthetic rate (Cutts et al. Citation2011), and tolerance to high levels of pollutants (Ma C et al. Citation2016). Napier grass resistance to heavy metals typical of those found in fly ash is well documented (Juel et al. Citation2018; Wiangkham and Prapagdee Citation2018; Osman et al. Citation2020). However, some concerns about the use of Napier grass in phytoremediation of heavy metal degraded environments include its sensitivity to poor soil fertility and the presence of extremely high concentrations of heavy metals (Ko et al. Citation2017). Additionally, one potential issue of using Napier grass is the disposal or utilization of biomass containing high heavy metal concentrations. A possible option is to utilize the biomass of Napier grass grown in the heavy metal-contaminated environment as a substrate for bioethanol production. Several studies have shown that biomass containing high concentrations of heavy metals can be used as a substrate for bioethanol production using heavy metal-tolerant microorganisms such as Saccharomyces cerevisiae (Cagnin et al. Citation2021). Utilizing phytoremediation biomass for ethanol production is a sustainable fuel production strategy whose social, economic, and environmental benefits are extensively described (Madhumita et al. Citation2018; Broda et al. Citation2022).
Therefore, we investigated the potential of a heavy metal tolerant P. purpureum for rehabilitation of the Morupule FA dumpsite owned by Morupule Power Station in Botswana and its subsequent utilization as a substrate for bioethanol production. To enhance the growth and development of Napier grass in fly ash, we evaluated the effect of supplementation of nutrient-poor fly ash with fresh sewage sludge, aged sewage sludge, compost, and food waste. Subsequently, the biomass produced from fly ash was evaluated as lignocellulosic feedstocks for bioethanol. Heavy metal uptake by the Napier grass in different amendments was also investigated including the phytoextraction capacity of Napier grass for heavy metals. The data presented in this article give vital insight into how fly ash dumpsite can be converted into productive land that can be used to generate bioenergy.
Materials and methods
Collection of fly ash, soil, and organic amendments
Fly ash
Fly ash was collected from Morupule B dumpsite in Palapye, Central Botswana. All the samples were air-dried and sieved using a 2 mm diameter mesh before use and analysis and were stored in plastic containers.
Organic amendments
Organic amendments which are fresh and aged sewage sludge were collected from the Water Utilities treatment plant, in Palapye Botswana. Food waste was bought from the cafeteria at the Botswana International University of Science and Technology (BIUST) and air drying was done as above except that it was preceded by 60°C oven drying for 48 h. Compost manure (Culterra (PTY) LTD., South Africa) was bought from an Agri-Chem store in Palapye, Botswana. All the samples were prepared as mentioned in Section 2.1.1.
Soil
Palapye soil used as a reference was collected from the undisturbed soil in the BIUST forest area, similarly the samples were processed as mentioned in Section 2.1.1.
Chemical characteristics of fly ash, soil, and organic amendments
The chemical characteristics of fly ash, soil, and organic amendments were assessed through various laboratory techniques, such as pH measurement, Electrical Conductivity (EC) determination, and analysis of total nitrogen, total phosphorus, total potassium, as well as total and extractable metals. The methods employed for this analysis were established laboratory protocols (Ultra Citation2020; Majaule et al. Citation2022). The pH and electrical conductivity (EC) values of fly ash and organic amendments were evaluated after a 2-h shaking period of samples in a 1:5 proportion of sample to distilled water. In contrast, the soil samples were subjected to a 1:2 ratio, and the parameters were assessed using HANNA instruments, specifically the pH/EC/DO Multiparameter (HI98194), as documented by Ultra (Citation2020) and Majaule et al. (Citation2022) in their respective studies. The total nitrogen content of the fly ash, soil, and organic amendments was determined using the Kjeldahl method, as outlined by Bremner and Mulvaney in 1982. To determine the total amounts of phosphorus and potassium present in soil and fly ash, a quantity of 2 g of the respective samples underwent a digestion process. This entailed heating the samples for a duration of 40 min at a temperature of 180°C, in the presence of 10 mL of nitric acid (70%) and 1 mL of sulfuric acid (98%), utilizing a microwave digester (Milestone ETHOS UP). The procedure employed for this process was the comprehensive guidelines outlined by Van Reeuwijk (Citation2002). Upon reaching ambient temperature, the solution was diluted with distilled water to a volume of 25 mL and subsequently analyzed using Inductively Coupled Plasma-optical Emission Spectrometry (ICP-OES, Thermo Scientific ICAP 7000). Badran et al. (Citation2018) employed an acid digestion method to determine the total P and K content in sewage sludge, compost, and food waste. Specifically, 1.25 g of the sample was subjected to acid digestion in a mixture of nitric acid and hydrogen peroxide at a 4:1 v/v ratio using a microwave digester at 120°C for 40 min. The sample was then allowed to cool before analysis. The quantification of the total phosphorus and potassium in the organic amendments was performed as previously described.
By acid digestion of roughly 0.2 g the samples (soil, fly ash, sewage sludge, compost, and food waste) were treated with 7 mL of concentrated nitric acid (HNO3), 1 mL of concentrated hydrochloric acid (HCl), and 2 mL of concentrated hydrofluoric acid (HF), in a microwave digester (Milestone ETHOS UP) at 200°C for 1h, the total heavy metal (As, Cr, Cu, Mn, Ni, Pb, and Zn) contents were determined. The extractable fraction of these heavy metals in the samples was determined using a 1.0 M ammonium acetate (at pH 7.0) at a 1:5 ratio (sample: extractant), after shaking for one hour and quantified using ICP-OES. These methods were used in all samples that is before and after cultivation. All the samples including post-harvest and plants were analyzed in duplicates. When the standard deviation between the samples was greater than 10%, a reanalysis of the sample was performed. Additionally, an internal standard sample that was recovered had more than 95% values for all elements measured. Department of Earth and Environment Sciences’ Soil Science Laboratory at BIUST in Palapye, Botswana.
Pot preparation, treatment description, and experimental design
Under greenhouse conditions, this experiment employed 2 factors: (1) five types of organic amendments including the control, and (2) two levels of inorganic fertilizer nitrogen, phosphorus, and potassium (NPK) application (−NPK and + NPK). There were 10 treatment combinations and an additional two reference treatments using Palapye Soil. These 12 treatments were replicated 3 times and laid out following a completely randomized design (CRD). These treatments were (T1)fly ash only (FA − NPK) which was considered as the control, (T2) fly ash plus inorganic fertilizer (FA + NPK), (T3)fresh sewage sludge minus inorganic fertilizer (FA + FSS − NPK), (T4)fresh sewage sludge plus inorganic fertilizer (FA + FSS + NPK), (T5) aged sewage sludge minus inorganic fertilizer (ASS − NPK), (T6)aged sewage sludge plus inorganic fertilizer (FA + ASS + NPK), (T7) food waste minus inorganic fertilizer (FA + FW − NPK), (T8) food waste plus inorganic fertilizer (FA + FW + NPK), (T9) compost minus inorganic fertilizer (FA + CM − NPK), (T10)compost plus inorganic fertilizer (FA + CM + NPK), (T11) Palapye soil minus inorganic fertilizer (PS − NPK), and (T12) Palapye soil plus inorganic fertilizer (PS + NPK). For each treatment, three plastic pots (25 cm diameter × 26.5 cm height) were prepared by mixing 8 kg fly ash with different soil organic amendments at an application rate of 10% by weight. For those treatments with + NPK, an inorganic fertilizer was added at a rate of 380-84-110 kg NPK/ha using CULTERRA (2:3:4(21)) equivalent to 3 g pot−1. This mixture of fly ash and amendments were incubated under greenhouse condition for 2 weeks while waiting for the Napier grass seedling to fully germinate.
Plant establishment and maintenance
The P. purpureum was planted on each pot from pregerminated cuttings obtained from a mother plant grown in the BIUST experimental station, Palapye Botswana. For the germination process, the cuttings were immersed in tap water in a beaker and stored in a cool and humid place for 2 weeks until the roots and shoots were fully developed. The sprouted seedlings were planted on the previously prepared pots with amendments (Aection 2.3). After 1 week, those pots with NPK treatment were supplied with the prescribed amount of fertilizer basally. Within the growing period of 5 months (November 2020 to April 2021), the pots were rearranged once a week to reduce variation due to positional effects, irrigated regularly with tap water, and maintained inside the greenhouse.
Growth media sampling after harvest and chemical analysis
After harvest, growth media was collected from each pot, and prepared as mentioned in Section 2.1.1. The pH and electrical conductivity (EC), total heavy metal, and extractable heavy metals of these samples were determined using the methods prescribed in Section 2.2.
Plant sampling and chemical analysis
The number of leaves and plant height were measured at harvest, the shoots were excised, and the roots were carefully separated from the growing media, washed thoroughly with tap water, air-dried for 24 h at room temperature, and followed by oven drying for 46 h at 60°C before recording dry yields. Then the samples were grounded for chemical analysis. The heavy metal content on tissues was determined by digesting the samples with a nitric acid and hydrogen peroxide mixture (8:2) in microwave digestion (Milestone ETHOS UP) at 200°C for 1 h and ICP 0ES was used to quantify heavy metals in the samples. Heavy metal uptake by plants was computed using Equation (1)
Where is the heavy metal uptake by plants (g ha−1),
is the concentration of metal in shoots of the plant (mg kg−1), and
is the shoot dry matter of the plant.
The translocation factor (TF) factor for each metal was calculated using Equation (2)
Where is the translocation factor,
is the concentration of metal in the shoot of the plant (mg kg−1),
is the concentration of the metal in the root of the plant. The bioaccumulation factor was calculated using Equation (3)
where
is the bioaccumulation factor,
concentration of the metal in the shoot and roots of the plant (mg kg−1),
is the mass of the plant in grams,
is the concentration of the metal in the pot growth media.
Similarly, the heavy metal contents of the extracted juice before fermentation, the pulp, and the bio-slurry generated after bioethanol production was analyzed using the same method as the tissue analysis with some modifications. Specifically, 3 ml of the juice extract or the bio-slurry was digested with 24 ml concentrated nitric acid and 6 ml hydrogen peroxide (30%) using a microwave digestor as mentioned elsewhere. The pulp was oven-dried and homogenized before analysis. The heavy metal content was quantified using ICP OES.
Bioethanol production
A robust industrial bioethanol yeast, Ethanol Red (E. red) (Saccharomyces cerevisiae) (Fermentis, France) was used for fermentation of recovered phytoremediation biomass. Overnight cultures of the yeast cells were prepared by inoculating a loopful of yeasts into test tubes containing 5 mL Yeast Extract Peptone Dextrose (YPD) liquid medium (Yeast extract 10 g L−1, Peptone 20 g L−1, and glucose 20 g L−1 at a pH of 6.2). The cultures were incubated in a shaking incubator (Thermo Scientific, MaxQ 6000, Ohio, USA) at 30°C shaking at 180 rpm. The next day, the cells were centrifuged (ThermoFisher Scientific, SL 16 R, Germany) at 2000 × g for 2 min and washed with 5 mL of sterile distilled water. The optical density (OD) of cells was determined using a wavelength of 600 nm using a spectrophotometer (VWR UV-1600PC, 804 Pennsylvania, USA). The cells were inoculated into sterile 60 mL syringes (Healthease®, Neomedic, Durban, South Africa) containing 5 mL of the lignocellulosic extracts at a final OD600nm of 1. The syringes containing samples from different treatments were sealed using luer lock caps and then incubated at 30°C, shaking at 180 rpm. The movement of the syringe plunger (due to the production of gases associated with fermentation as described by Motlhanka et al. (Citation2022) and Semumu et al. (Citation2022) was recorded every 2 h until fermentation was complete for 48 h. After fermentation was complete, the suspension from the syringes was emptied into 15 mL centrifuge tubes and centrifuged at 4 000 g × for 5 min to separate the ethanol-containing supernatant from the yeast cells. The supernatant of about 1 mL was stored at −20°C and later used to determine the concentration of ethanol using an ethanol assay kit (BOEHRINGER MANNHEIM Enzymatic BioAnalysis/Food Analysis, 10176290035). The concentration of ethanol expressed in gL−1 was calculated using the following equation, as per kit manufacturer’s recommendations (4).
where
is the final volume (mL),
is the molecular weight ethanol (g mol−1),
is the extinction coefficient of NADH at 340 nm which is (6.3),
is the light path (cm) =1.00 cm,
is the sample volume (mL). All fermentation experiments and analyses were performed in triplicates.
Validation of the analytical method
The precision and accuracy of the analysis of all samples were secured using duplicate samples and the use of internal standard reference samples (soil and corn tissue) whose values were verified by multi-laboratory analysis in Botswana (). When the coefficient of variation between the duplicate samples was greater than 10%, the samples were reanalyzed. The recovery for each batch analysis of total heavy metal content and available fraction using 1.0 M ammonium acetate (at pH 7.0) in the internal standard reference samples is more than 95%.
Table 1. Percentage recovery of heavy metal concentrations of internal reference sample.
Statistical analysis
A two-factor ANOVA was used to analyze the data using SPSS v 12 software. Only treatments involving fly ash (T1 to T10) were included in the ANOVA. When significant differences were found, Waller-Duncan’s test was used to compare treatment means at a 5% significance level.
Results
Plant height and dry matter yield
In terms of plant height, a significant main effect (p = .000) of amendment was seen throughout the planting period (). However, the interaction of amendment and fertilizer application did not significantly (p = .017) affect plant height during the 20 weeks following planting. Regardless of NPK fertilizer application, plants did not grow in FA only, with the highest plant height (96.97 cm) being seen in fresh sewage sludge amendment (FSS) and the shortest plant height (40.43 cm) being observed in food waste (FW). There was no significant (p = .66) interaction between amendment and fertilizer treatment on the number of leaves. Throughout the planting period, regardless of fertilizer treatment, the significance of the major effect caused by organic amendments on the number of leaves was noted. Compost amendment (CM) contained the most leaves, whereas food waste (FW) contained the fewest leaves.
Table 2. Growth parameters of P. purpureum growing in fly ash as affected by different amendments and fertilizer application.1
The interaction of the amendment and fertilizer application, on the other hand, had a significant effect (p = .000) on the dry matter yield. In comparison to Palapye soil + NPK (reference treatment), fresh sewage sludge plus inorganic fertilizer (FSS + NPK) treatment yielded the maximum dry weight of shoots (100.58 g plant−1) while the root yield was obtained from compost plus inorganic fertilizer (CM + NPK) treatment as 52.68 g plant−1. On the other hand, food waste minus inorganic fertilizer (FW − NPK) yielded the lowest dry material, 3.08 g plant−1 for leaves, 5.52 g plant−1 for stem, and 2.88 g plant−1 for roots. As opposed to the reference treatment (Palapye soil + NPK), the shoot/root ratio was higher in the aged sewage sludge plus inorganic fertilizer (ASS + NPK) treatment. Shoot/root ratios were nearly the same for FSS -NPK, FW − NPK, and CM − NPK. When compared to other treatments, the FSS + NPK treatment significantly increased P. purpure’s dry matter yield.
The concentration of heavy metals in plants
The accumulation of Cr, Mn, Ni, and Pb in P. purpureum shoots was significantly (p = .01) influenced by the interaction between amendment and fertilizer application, but not Cu or Zn (). Neither the plant’s roots nor its shoots contained any arsenic. The aged sewage sludge plus inorganic fertilizer (ASS - NPK) treatment had the highest quantities of Mn (574.45 mg kg−1), whereas the CM − NPK treatment had the highest concentrations of Cr (78.87 mg kg−1), Ni (35.18 mg kg−1), and Pb (25.53 mg kg−1).
Table 3. Chemical properties and average exchangeable heavy metals extracted from fly ash with amendments 20 weeks after cultivation of P. purpureum.Table Footnote1
Table 4. Total heavy metal concentration in shoots of P. purpureum grown in fly ash with different amendments.Table Footnote1
On the other hand, the lowest content of Cr (42.39 mg kg−1) and Pb (10.15 mg kg−1) was found in ASS + NPK, whereas the lowest content of Mn (191.52 mg kg−1) in the plant was found in FSS + NPK, and the lowest Ni (23.52 mg kg−1) content in shoots was found in CM + NPK treatment. The main effect due to fertilizer was observed in Cu while the main effect due to amendment was found in Zn. Regardless of the amendments the average Cu content in shoots grown without NPK is 90.03 mg kg−1 which is significantly higher than Cu content in plants grown with NPK (83.71 mg kg−1). Among the amendment treatments, ASS had the highest average Zn (164.54 mg kg−1) content followed by fresh sewage sludge (67.52 mg kg−1), compost (56.65 mg kg−1), and food waste (46.59 mg kg−1).
For all the heavy metals accumulated in the roots, there was a significant interaction effect between amendment and fertilizer (). Depending on the type of metal and different fly ash amendments and fertilizer applications, could either increase or decrease the metal concentration. The highest content of Cr (126.61 mg kg−1), Cu (319.34 mg kg−1), Mn (497.57 mg kg−1), and Ni (33.33 mg kg−1) in roots was found in FSS – NPK treatment whereas the highest content of Pb (26.87 mg kg−1) in roots was found in CM – NPK treatment and the highest content of Zn (147.49 mg kg−1) was found in ASS – NPK treatment. On the other hand, the lowest content of Cr (36.42 mg kg−1) in roots was found in ASS – NPK treatment, while the lowest Cu (64.51 mg kg−1), Mn (263.60 mg kg−1), Ni (15.08 mg kg−1), and Pb (3.02 mg kg−1) content in the roots was found in FW + NPK treatment, and the lowest content of Zn (69.23 mg kg−1) was found in CM + NPK treatment.
Table 5. Heavy metal accumulated in roots of P. purpureum grown in fly ash with different amendmentsTable Footnote1.
Estimated removal of toxic metals by the plants
A significant interaction effect (p = .000) of amendments * fertilizer was observed on the estimated removal of toxic metals by P. purpureum (). The estimated results indicated that fresh sewage sludge plus inorganic fertilizer treatment (FSS + NPK) was able to remove Mn (1196.12 g ha−1) and Ni (128.06 g ha−1). Compost plus inorganic fertilizer treatment (CM + NPK) removed an estimated amount of 380.04 g ha−1 for Cu, 75.40 g ha−1 for Pb, and 446.82 g ha−1 for Zn. An estimated amount of 68.36 g ha−1 for Cr was removed by compost minus inorganic fertilizer treatment (CM – NPK).
Heavy metal content of the extracted juice
The results indicated that the juice extracted from the phytoremediation biomass (leaves and stem) contained heavy metals (Tables S5 and S6). The heavy metal content ranged from 15.49 to 44. 86 mg L−1 for Cr, 13.28 to 23.50 mg L−1 for Cu, 34.17 to 106.00 mg L−1 for Mn, 4.18 to 22.18 mg L−1 for Ni, 1.74 to 9.29 mg L−1 for Pb, and 12.05 to 33.59 mg L−1 for Zn. Among these Mn (106.00 mg L−1) was the highest in the CM − NPK treatment while Pb (1.74 mg L−1) was the lowest in the CM + NPK treatment. The juice extracted from the stem contained heavy metal concentrations ranging from 0.19 to 1.40 mg L−1 for Cr, 11.83 to 17.46 mg L−1 for Cu, 15. 24 to 34.31 mg L−1 for Mn, 1.61 to 2.55 mg L−1 for Ni, 0.31 to 3.82 mg L−1 for Pb, and 8.21 to 27.02 mg L−1 for Zn. Among these metals, the highest content was Mn (34. 31 mg L−1) in the CM + NPK treatment, while Cr (0.19 mg L−1) was the lowest in the FW – NPK treatment.
Table 6. Estimated removal of heavy metal from fly ash by P. purpureum.Table Footnote1
Ethanol produced from the phytoremediation biomass
The fermentation results revealed that the compost + NPK (CM + NPK) treatment had the highest ethanol accumulation in the leaf extracts, reaching a concentration of 85.90 ± 4.50 g L−1 (). On the other hand, the FSS + NPK treatment exhibited the lowest ethanol accumulation in the leaf extracts (11.06 ± 0.563 g L−1). With the fermentation of Napier grass stems, the CM + NPK treatment accumulated the highest ethanol concentrations, reaching 42.15 ± 4.57 g L−1, whereas the ASS –NPK treatment accumulated the lowest ethanol concentrations at 27.27 ± 11.7 g L−1.
Figure 1. Ethanol accumulation after fermentation of juice extracts obtained from Napier grass leaves and stems using Saccharomyces cerevisiae (Ethanol red) yeast. SS: sewage sludge; Py: Palapye; FSS: fresh sewage sludge; COM: compost; ASS: aged sewage sludge; FW: food waste, +NPK: with inorganic NPK, and − NPK: without inorganic NPK. The experiment was conducted in triplicate, with the error bars indicating the standard deviation from the mean.
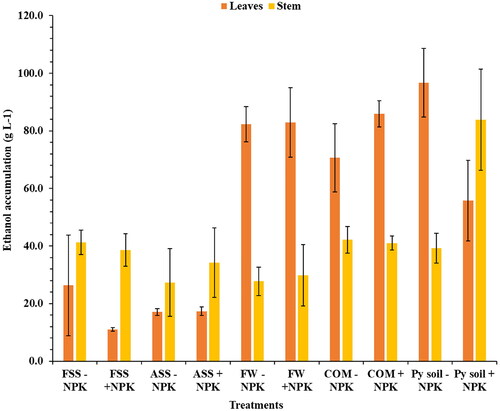
Further analysis of the fermentative capacity in terms of carbon dioxide (CO2) production rate provided additional insights. In the case of the leaves, the treatment combining food waste with NPK fertilizer (FW + NPK) had the fastest CO2 production rate at 0.4024 ± 0.104 mL h−1 (). This rate was comparable to the reference treatments involving Py soil with or without NPK (0.4024 ± 0.000 mL h−1). Conversely, the slowest CO2 production rate in the leaf samples was observed in the ASS – NPK treatment (0.0558 ± 0.000 mL h−1; ). In the stem samples, the ASS + NPK treatment exhibited the fastest CO2 production rate (0.2875 ± 0.111 mL h−1), surpassing all other treatments. In contrast, FW – NPK treatment had the slowest CO2 production rate at 0.1300 ± 0.0926 mL h−1.
Figure 2. CO2 production rate after fermentation of juice extracts obtained from Napier grass leaves and stems using Saccharomyces cerevisiae (Ethanol red) yeast. SS: sewage sludge; Py: Palapye; FSS: fresh sewage sludge; COM: compost; ASS: aged sewage sludge; FW: food waste; +NPK: with inorganic NPK; and − NPK: without inorganic NPK. The experiment was conducted in triplicate, with the error bars indicating the standard deviation from the mean.
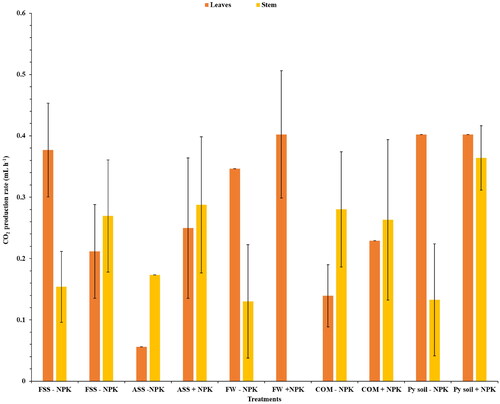
Heavy metal content of the pulp and the bio-slurry
The pulp waste after the extraction of juice indicated that it contained a substantial number of heavy metals (Table S7). The heavy metal content ranged from 0.01 to 0.09 mg kg−1 for Cr, 0.64 to 3.33 mg kg−1 for Cu, 0.73 to 7.31 mg kg−1 for Mn, 0.04 to 1.02 mg kg−1 for Ni, 0.04 to 0.88 mg kg−1 for Pb, and 2.39 to 13.75 mg kg−1 for Zn. Among these, Zn (13.75 mg kg−1) was the highest in the CM – NPK treatment while Cr (0.10 mg kg−1) was the lowest in the FSS + NPK treatment. In the pulp of the stem after juice extraction (Table S8), the heavy metals content ranged from 0.01 to 0.09 mg kg−1 for Cr, 0.64 to 3.33 mg kg−1 for Cu, 0.73 to 7.31 mg kg−1 for Mn, 0.04 to 1.02 mg kg−1 for Ni, 0.04 to 0.88 mg kg−1 for Pb, and 2.39 to 13.75 mg kg−1 for Zn. Among these, Zn (13.75 mg kg−1) was the highest in the CM – NPK treatment, while Cr (0.01 mg kg−1) was the lowest in the FW – NPK treatment.
The data obtained from the metal analysis of the bio-slurry after the fermentation indicated that it contained a substantial amount of heavy metals (Tables S9 and S10). The residual heavy metal content in the bio-slurry after fermentation of the leaves ranged from 0.26 to 11.74 mg L−1 for Cr, 3.65 to 9.70 mg L−1 for Cu, 26.92 to 89.83 mg L−1 for Mn, 4.07 to 7.76 mg L−1 for Ni, 0.76 to 3.08 mg L−1 for Pb, and 4.15 to 12.55 mg L−1 for Zn. Among these, Mn (89.83 0.06 mg L−1) was the highest content in the FW - NPK treatment while Cr (0.26 mg L−1) was the lowest in the FSS + NPK treatment. The heavy metals content in the bio-slurry after the fermentation of the stem-extracted juice ranged from 0.07 to 0.67 mg L−1 for Cr, 2.60 to 6.06 mg L−1 for Cu, 2.26 to 15.70 mg L−1 for Mn, 0.07 to 1.61 mg L−1 for Ni, 0.06 to 1.38 0.06 mg L−1 for Pb, and 7.46 to 16.29 mg L−1 for Zn. Among these, Zn (16.29 mg L−1) was the highest in the CM – NPK treatment, whereas Pb (0.06 mg L−1) was the lowest in the FSS – NPK treatment.
Discussion
The preliminary characteristics of fly ash indicated that it possessed a high level of alkalinity, with a pH of 8.50. Additionally, it was found to contain hazardous metals such as arsenic, chromium, copper, manganese, and lead, all of which exceeded the critical concentration, except for zinc. Furthermore, the fly ash lacked organic carbon and had a low total nitrogen content of 0.14 mg kg−1 (Table S1). As a result, the Napier grass could not survive in pure fly ash without the organic amendments indicating that the chemical properties of fly ash cannot support the growth of Napier. Combustion of coal removes nitrogen in fly ash making it the most constraining nutrient for the growth of Napier grass. Also, the higher pH, lack of organic matter content, poor nutrients, and high concentrations of heavy metals in fly ash made it difficult for Napier grass to survive in it.
The application of various organic amendments to FA resulted in an enhancement of the survival and development of Napier grass. The pH was significantly reduced by the addition of various organic amendments, bringing it down to a range of 7.20 to 8.15 (), which is a better pH for Napier grass growth (Negawo et al. Citation2017). Empirical research has demonstrated that P. purpureum is capable of surviving and growing within a pH range of 4.5 to 8.2, as evidenced by the findings of Kebede et al. (Citation2016) and Osman et al. (Citation2020). The introduction of fresh sewage sludge (FSS) and aged sewage sludge (ASS) had a notable impact on the pH levels of FA. This outcome can be attributed to the preexisting pH conditions of the FSS and ASS. The observed decline in pH levels of the treatments may potentially be attributed to the breakdown of organic substances present in the sludge. The utilization of compost as an amendment resulted in the acidification of the growth media to a minimum pH of 8.15. However, the decrease in pH levels due to the combination of CM and NPK was not found to be significantly distinct from the pH levels observed in the control group. The marginal decrease in pH may be ascribed to the compost’s relatively lower alkalinity in comparison to the FA. Despite having a higher C: N ratio compared to other amendments analyzed in this study, compost can be deemed as a more stable option that does not undergo significant active decomposition. Consequently, it releases a relatively lower quantity of organic acids. In contrast to compost, fresh food waste and sewage sludge are likely to contain a higher proportion of soluble constituents, such as soluble sugars and amino acids, as opposed to the more resistant and stable organic compounds, such as lignin and cellulose, found in compost. This difference in composition may result in a lower volume of organic acids being released into the soil, as noted by Taupedi and Ultra (Citation2022). The findings of this study indicate that the utilization of organic amendments, specifically fresh food waste, and sewage sludge at a concentration of 10% (W/W) in FA, can enhance the pH level to a state of near neutrality.
The FA utilized in the research exhibited substantial quantities of exchangeable and overall heavy metals (namely As, Cr, Cu, Mn, Pb, and Zn) before the introduction of amendments and the planting of P. purpureum (Table S1). However, the application of diverse organic amendments and NPK resulted in a noteworthy decrease in these hazardous metals. In comparison to the control group, it was observed that the ASS + NPK treatments resulted in a significant decrease in the exchangeable metals Cr and Cu by 41% and 62%, respectively. Similarly, the FSS + NPK treatment exhibited a reduction in exch-As by 24%, whereas the compost + NPK treatment showed a decrease in exch-Zn by 10%. The observed reduction in metal availability may be attributed to the humified organic matter present in sewage sludge, which generates negatively charged sites capable of adsorbing these metals. This phenomenon has been documented in previous studies (Ashworth and Alloway Citation2007; Gersztyn et al. Citation2013; Fang et al. Citation2016; Gusiatin et al. Citation2017;). According to Antonkiewicz et al. (Citation2022), fly ash are source of macronutrients for plants to phytoextraction heavy metals by dumping site as it contains Ca, Mg, Fe, Mn, B, Na, and minimum amount of P and K. The alterations in the chemical characteristics of FA led to its enhanced suitability for promoting plant growth, ultimately leading to the attainment of the maximum yield of Napier grass in the FSS–NPK intervention. The higher yield of Napier grass in FSS treatment could be attributed to the high total amount of nitrogen (5.26%) contained in this organic amendment, and this total N could have contained a higher amount of N available for plant uptake, as Wong et al. (Citation2001) study claims that during anaerobic digestion of sludge, most of the N is converted to NH4-N which is in an available form for plant uptake. A higher shoot/root ratio implies that there is sufficient nutrient uptake, by the plant, and that the plant can strive in a stressful environment while a lower ratio indicates a deficiency of essential nutrients especially nitrogen and phosphorus (Kim and Li Citation2016). The highest shoot/root ratio was found in ASS + NPK treatment while the lowest ratio was in CM + NPK (). The lower soot/root ratio in CM + NPK treatment is due to a higher mass of roots which is promoted by a deficiency of P in the compost amendment, this finding is consistent with that of Kim and Li. (2016) found out that plants grown with lower P allocate more biomass to the roots than to the shoots which leads to a lower shoot/root ratio. The higher amount of nitrogen in the sewage treatments contributed to the higher shoot/root ratio as compared to other treatments.
According to the study by Couselo et al. (Citation2012), P. purpureum facilitates the absorption of heavy metals from the polluted soil via its root system and then translocates them to various plant parts, resulting in their accumulation and elimination. The data presented in and show that P. purpureum shoots and roots demonstrated the ability to absorb heavy metals from the growth medium during the current investigation. Depending on the treatment, some metals had accumulated more in shoots than in roots while others were the opposite. Napier grass planted in the FA amended with compost – NPK had the highest accumulation of Cr (78.87 mg kg−1), Cu (115.37 mg kg−1), Mn (313.26 mg kg−1) and Ni (35.18 mg kg−1) in the shoots. Lead (26.87 mg kg−1) and Zinc (1471.49 mg kg−1) were highly accumulated in the roots of Napier grass in the compost – NPK and ASS – NPK amended fly ash, respectively. These concentrations exceeded the established limits of 1.30, 10.00, 200.00, 2.74, and 50.00 mg kg−1 for these elements, respectively, (as shown in and ). The availability and bioaccumulation of metals are influenced by a variety of environmental conditions, such as organic chelators, humic compounds, the presence of other metals, and salinity, as noted by Maiti and Pandey (Citation2020) and Osman et al. (Citation2020). Therefore, the results that were observed suggest that there are distinct mechanisms involved in the bioaccumulation and translocation of these metals. shows that the presence of Cr, Cu, Mn, and Ni in the root tissues of Napier grass grown with ASS–NPK could be due to the formation of metal-organic matter complexes, which makes it harder for metals to move to the top of the plant (Belhaj et al. Citation2016). The findings of the investigation suggest that P. purpureum cultivated in FA combined with organic amendments can serve as a cover crop to stabilize the fly ash dumpsite. The data shown in Tables S3 and S4 further demonstrate that the root matrix of this crop can retain the FA particles and potentially reduce the concentration of heavy metals in the FA dumpsite. The efficacy of P. purpureum in eliminating toxic metals from FA was observed to be augmented by the application of organic amendments, as evidenced by the significant quantities of toxic metals that were extracted from FA by the plants (as presented in and ). According to the estimated findings, the application of fresh sewage sludge in conjunction with inorganic fertilizer was found to effectively eliminate Mn (1196.12 g ha−1) and Ni (128.06 g ha−1). The application of nitrogen fertilizer to organic amendments leads to a decrease in the pH of the growth medium, thereby enhancing the absorption of manganese (Mn) and nickel (Ni).
Bioethanol is a highly appealing substitute for petroleum-based fuels because of its numerous benefits. It can be produced domestically from locally sourced biomass, thus decreasing dependence on imported fossil fuels, and giving countries greater control over their energy resources (Demirbas and Demirbas Citation2007). Furthermore, it increases energy security and reduces greenhouse gas emissions, contributing to a sustainable and environmentally friendly environment (Nwaefuna et al. Citation2021). Napier grass has garnered attention as a prospective feedstock for ethanol production owing to its high biomass yield (Pakwan et al., Citation2020, Mohammed et al., Citation2019), rapid growth facilitating quick biomass generation, and its composition comprising cellulose, hemicellulose, and lignin, which provides a potential source of fermentable sugars upon breakdown (Cutts et al. Citation2011, Delimanto, Citation2020).
In this study, the fermentative capacity of Napier grass leaves and stems was examined using Saccharomyces cerevisiae (Ethanol red) yeast to ferment the sugars present in the stem and leaf extracts. The fermentation results revealed that the compost + NPK (CM + NPK) treatment had the highest ethanol accumulation in the leaf extracts, reaching a concentration of 85.90 ± 4.50 g L−1 (). It was statistically significant from the fresh sewage sludge treatments (FSS + NPK and FSS – NPK), aged sewage sludge treatments (ASS – NPK and ASS + NPK), as well as the reference Palapye soil + NPK treatment. This outcome suggests that the combination of compost + NPK treatment was particularly effective in enhancing ethanol production from the leaf extracts. On the other hand, the FSS + NPK treatment exhibited the lowest ethanol accumulation in the leaf extracts (11.06 ± 0.563 g L−1), and it was statistically different when compared to all other treatments, except for ASS – NPK and ASS + NPK. This finding implies that certain treatments, such as those involving sewage sludge, might have limited efficiency in promoting ethanol production in Napier grass leaves. With the fermentation of Napier grass stems, the CM + NPK treatment accumulated the highest ethanol concentrations, reaching 42.15 ± 4.57 g L−1, whereas the ASS – NPK treatment accumulated the lowest ethanol concentrations at 27.27 ± 11.7 g L−1. Notably, there was no statistically significant difference in ethanol accumulation between CM – NPK and the other stem treatments, except for the Palapye soil + NPK treatment. Comparing the ethanol concentrations between Napier grass leaves and stems, the results indicate that the leaf extracts accumulated higher amounts of ethanol than the stems, indicating an inherent difference in the fermentative capacity or composition between these plant parts. The highest ethanol yield observed in compost treatment could be due to different factors like the higher accumulation of Cr in the shoots and lower accumulation of Zn. Ko et al. (Citation2017) claim that Cr contamination increases ethanol yield in Napier grass while Zn reduces ethanol yield as the study found out that the highest ethanol concentrations of 19.31 g L−1 and 12.68 g L−1 were obtained in Cr and Zn contaminated environment, respectively. Similar results were obtained in our study in terms of Cr content in ethanol, as for Zn we obtained contradicting results. There was a strong positive correlation (r = 0.73) between Cr content in the juice with ethanol concentration, while between Zn content and ethanol concentration, there was a weak positive correlation (r = 0.23). Furthermore, this higher yield could be attributed to lower dry yield matter in compost treatment, due to less nitrogen content in the treatment which resulted in higher sugar content in the biomass (Pakwan et al. Citation2020).
Further analysis of the fermentative capacity in terms of carbon dioxide (CO2) production rate provided additional insights. In the case of the leaves, the treatment combining food waste with NPK fertilizer (FW + NPK) had the fastest CO2 production rate at 0.4024 ± 0.104 mL h−1 (). This rate was comparable to the reference treatments involving Py soil with or without NPK (0.4024 ± 0.000 mL h−1), suggesting that the addition of food waste in conjunction with NPK supported a fermentation process similar to the standard soil conditions that were used. The FW + NPK treatment was statistically different from the FSS – NPK and ASS – NPK treatments. Conversely, the slowest CO2 production rate in the leaf samples was observed in the ASS – NPK treatment (0.0558 ± 0.000 mL h−1), which was statistically different from all the other treatments, including the references, except for CM – NPK (0.1395 ± 0.0507 mL h−1). The CO2 production rate of ASS – NPK was approximately 0.14 times slower than that of the reference treatments. In the stem samples, the ASS + NPK treatment exhibited the fastest CO2 production rate (0.2875 ± 0.111 mL h−1), surpassing all other treatments. In contrast, FW – NPK treatment had the slowest CO2 production rate at 0.1300 ± 0.0926 mL h−1. Statistically significant differences were observed when comparing FW – NPK to treatments FSS + NPK (0.2696 ± 0.0913 mL h−1), ASS + NPK (0.2875 ± 0.111 mL h−1), and CM – NPK (0.2632 ± 0.131 mL h−1). The ASS + NPK treatment exhibited a CO2 production rate that was approximately 2.20 times faster than that of the FW – NPK treatment. Notably, the FW + NPK treatment did not undergo fermentation at all, probably because the extract did not provide an adequate source of sugars to support yeast fermentation.
Most of the treatments with the NPK fertilizer exhibited faster CO2 production rates in both leaf and stem extracts. The role of NPK in providing essential nutrients to the Napier grass might have influenced microbial activity and fermentation rates (Pakwan et al., Citation2020). The CO2 production profiles indicate that Napier grass stem extracts exhibited a longer lag phase compared to the leaves ( and ). This likely occurred because the stem cell walls might possess more complex or resistant structures, requiring a longer duration for enzymatic breakdown and subsequent sugar release compared to the more readily accessible sugars present in leaf cell walls (Mohapatra et al., Citation2019). These variations observed among treatments suggest that the type and composition of applied treatments can distinctly impact ethanol production. Additionally, the inherent differences in fermentative capacity between leaves and stems highlight the need for a comprehensive understanding of the biochemical and metabolic processes in different plant parts to optimize ethanol production.
Figure 3. The CO2 production profile of fermented juice extracts obtained from Napier grass leaves after the phytoremediation of fly ash amended with different organic amendments. Saccharomyces cerevisiae (Ethanol red) yeast was used for the fermentation process SS: sewage sludge; Py: Palapye; FSS: fresh sewage sludge; COM: compost; ASS: aged sewage sludge; FW: food waste; +NPK: with inorganic NPK; and − NPK: without inorganic NPK. The experiment was conducted in triplicate, with the error bars indicating the standard deviation from the mean.
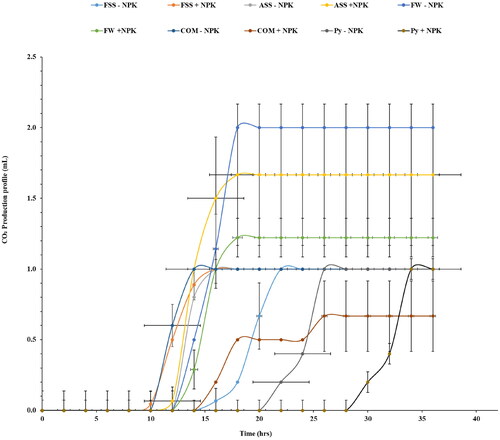
Figure 4. The CO2 production profile of fermented juice extracts obtained from Napier grass stems after the phytoremediation of fly ash amended with different organic amendments. Saccharomyces cerevisiae (Ethanol red) yeast was used for the fermentation process. SS: sewage sludge; Py: Palapye; FSS: fresh sewage sludge; COM: compost; ASS: aged sewage sludge; FW: food waste; +NPK: with inorganic NPK; and − NPK: without inorganic NPK. The experiment was conducted in triplicate, with the error bars indicating the standard deviation from the mean.
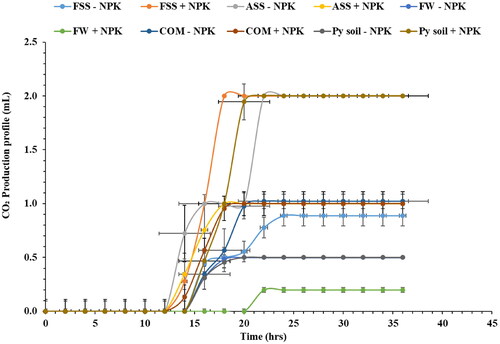
There were two forms of waste derived from biomass during the process of bioethanol production from the phytoremediation biomass. These consist of the pulp following juice extraction and the slurry following the synthesis of bioethanol. According to WHO (2011), it was noted that the concentrations of Cr, Cu, Ni, and Pb, among the heavy metals found in the juice extract, were over the critical values at 1.30 mg kg−1, 10 mg kg−1, 2.74 mg kg−1, and 2.0 mg kg−1, respectively. When comparing the pulp concentrations to the juice concentrations, these heavy metals were dramatically reduced by an average of 95.01%, 88.78%, 67.29%, and 82.92%, respectively. In the bio-slurry, the toxic metal decreased by 77.28%, 70.35%, 42.49%, and 55.72% compared to the concentration contained in the juice before fermentation. Except for Cr and Ni in the pulp and Cr in the bio-slurry, the metal concentrations in both the pulp and the bio-slurry were within permissible limits of WHO, 2011. When the yeast strain gained more tissues during fermentation, the concentration of harmful metals in the juice is lowered, which may account for the dilution of the toxic metal concentrations. Both substrates contained a substantial number of heavy metals (Tables S5 to S10) hence further research should be taken on how this should be valorized for another purpose. This could be done by converting the pulp to biochar and use of bio-slurry as soil amendments. This area needs further study for their future use toward a more efficient circular economy system.
Conclusion
Overall, our findings indicate that P. purpureum cannot survive in pure fly ash but it can thrive and produce high biomass when FA is combined with organic amendments at the rate of 10% by weight of fly ash. Organic amendments especially food waste reduced the pH, while compost and sewage sludge increased organic matter, increased nutrient pool, and reduced the heavy metal availability resulting in a more favorable environment for the growth of Napier grass, Napier grass can uptake heavy metals such as (Mn, Ni, Cr, and Cu) and periodic harvesting of shoots may result in the elimination of HMs from FA, ultimately lowering the level of HM contamination in FA. The Napier biomass harvested from fly ash with organic amendments can be used as a substrate for bioethanol production using the E. Red yeast strain. The maximum concentration attained was 19.31 g L−1 from Napier grass produced from FA amended with compost + NPK which shows that despite the presence of hazardous metals within the biomass, it is viable for the biochemical conversion of this biomass and produces ethanol, thus providing additional economic benefits aside from the remediation process. This confirms the potential of the biochemical conversion process for producing fermentable sugars and bioethanol from biomass obtained after phytoremediation. Given the significant levels of hazardous metals present, further research is required in this area to determine the best ways to dispose of or use the pulp that remains after juice extraction and the bio-slurry that is produced after bioethanol production. Possible uses for the pulp include converting it into biochar and utilizing the bio-slurry as soil amendments.
Ethical approval
This manuscript did not involve any human participants, human data, human tissues, or cloned animals hence ethical approval is not applicable.
Consent to participate
Not applicable
Consent to publish
All authors have full consent to publish the content of the manuscript.
Supplemental Material
Download MS Word (67.8 KB)Disclosure statement
The authors report there are no competing interests to declare.
Data availability statement
The data that support the findings of this study are available from the corresponding author upon reasonable request.
Additional information
Funding
References
- Antonkiewicz J. 2010. Effect of sewage sludge and furnace waste on the content of selected elements in the sward of legume-grass mixture. J Elem. 15(3):435–443. doi: 10.5601/jelem.2010.15.3.435-443.
- Antonkiewicz J, Kowalewska A, Mikołajczak S, Kołodziej B, Bryk M, Spychaj-Fabisiak E, Koliopoulos T, Babula J. 2022. Phytoextraction of heavy metals after application of bottom ash and municipal sewage sludge considering the risk of environmental pollution. J Environ Manage. 306:114517. doi: 10.1016/j.jenvman.2022.114517.
- Ashworth DJ, Alloway BJ. 2007. Complexation of copper by sewage sludge-derived dissolved organic matter: effects on soil sorption behavior and plant uptake. Water Air Soil Pollut. 182(1-4):187–196. doi: 10.1007/s11270-006-9331-7.
- Baba A, Gurdal G, Sengunalp F, Ozay O. 2008. Effects of leachant temperature and pH on leachability of metals from fly ash. A case study: can thermal power plant, province of Canakkale, Turkey. Environ Monit Assess. 139(1-3):287–298. doi: 10.1007/s10661-007-9834-8.
- Badran M, Morsy R, Elnimr T, Badran M, Soliman H. 2018. Assessment of wet acid digestion methods for ICP-MS determination of trace elements in biological samples by using Multivariate Statistical Analysis. J Elem. 23(1):179–189. doi: 10.5601/jelem.2016.21.3.1232.
- Belhaj D, Elloumi N, Jerbi B, Zouari M, Abdallah FB, Ayadi H, Kallel M. 2016. Effects of sewage sludge fertilizer on heavy metal accumulation and consequent responses of sunflower (Helianthus annuus). Environ Sci Pollut Res Int. 23(20):20168–20177. doi: 10.1007/s11356-016-7193-0.
- Broda M, Yelle DJ, Serwańska K. 2022. Bioethanol production from lignocellulosic biomass—challenges and solutions. Molecules. 27(24):8717. doi: 10.3390/molecules27248717.
- Cagnin L, Gronchi N, Basaglia M, Favaro L, Casella S. 2021. Selection of superior yeast strains for the fermentation of lignocellulosic steam-exploded residues. Front Microbiol. 12(2021):756032. doi: 10.3389/fmicb.2021.756032.
- Couselo JL, Corredoira E, Vieitez AM, Ballester A. 2012. Plant tissue culture of fast-growing trees for phytoremediation research. In: Loyola-Vargas V, Ochoa-Alejo N, editors. Plant cell culture protocols. Methods in molecular biology. Totowa, NJ. Springer. p. 247–263. doi: 10.1007/978-1-61779-818-4_19.
- Cutts G, Webster T, Grey T, Vencill W, Lee R, Tubbs R, Anderson W. 2011. Herbicide effect on Napiergrass (Pennisetum purpureum) control. Weed Sci. 59(2):255–262. doi: 10.1614/WS-D-10-00130.1.
- Delimanto WO. 2020. Production of Bioethanol from Napier grass: Comparison in Pretreatment and Fermentation Methods. InIOP Conference Series: Earth and Environmental Science. (Vol. 520, No. 1, p. 012005). IOP Publishing. doi: 10.1088/1755-1315/520/1/012005.
- Demirbas AH, Demirbas I. 2007. Importance of rural bioenergy for developing countries. Energy Convers Manag. 48(8):2386–2398. doi: 10.1016/j.enconman.2007.03.005.
- Dwivedi A, Jain M. 2014. Fly ash – waste management and overview: a Review Fly ash – waste management and overview: a review. Recent. Res. Sci. Technol. 6:30–35.
- Fang W, Wei Y, Liu J. 2016. Comparative characterization of sewage sludge compost and soil: heavy metal leaching characteristics. J Hazard Mater. 310:1–10. doi: 10.1016/j.jhazmat.2016.02.025.
- Gajaje K, Ultra VU, David PW, Rantong G. 2021. Rhizosphere properties and heavy metal accumulation of plants growing in the fly ash dumpsite, Morupule power plant, Botswana. Environ Sci Pollut Res Int. 28(16):20637–20649. doi: 10.1007/s11356-020-11905-7.
- Gersztyn L, Karczewska A, Gałka B. 2013. Influence of pH on the solubility of arsenic in heavily contaminated soils/Wpływ pH na rozpuszczalność arsenu w glebach silnie zanieczyszczonych. Environ. Nat. Resour J. 24(3):7–11. doi: 10.2478/oszn-2013-0031.
- Gusiatin ZM, Kulikowska D, Klik B. 2017. Suitability of humic substances recovered from sewage sludge to remedy soils from a former As mining area–a novel approach. J Hazard Mater. 338:160–166. doi: 10.1016/j.jhazmat.2017.05.019.
- Juel MAI, Dey TK, Akash MI, Das KK. 2018. Heavy metals phytoremidiation potential of Napier grass (Pennisetum purpureum) cultivated in tannery sludge. Proceedings of the 4th International Conference on Civil Engineering for Sustainability Development (ICCESD); 2018 Feb 9-11. Khulna, Bangladesh. p. 9–11.
- Kabir MS, Salam MA, Paul DN, Hossain MI, Rahman NM, Aziz A, Latif MA. 2016. Spatial variation of arsenic in soil, irrigation water, and plant parts: a microlevel study. The Scientific World Journal. 2016.2186069. doi: 10.1155/2016/2186069.
- Kebede G, Feyissa F, Assefa G, Mengistu A, Minta M, Tsadik T. 2016. Agronomic performance and nutritive values of Napier grass (Pennisetum Purpureum (L.) Schumach) accessions in the Central Highland of Ethiopia. Int J Dev Res. 6:8717–8726.
- Kim H, Li X. 2016. Effects of phosphorus on shoot and root growth, partitioning, and phosphorus utilization efficiency in Lantana. Horts. 51(8):1001–1009. doi: 10.21273/HORTSCI.51.8.1001.
- Ko CH, Yu FC, Chang FC, Yang BY, Chen WH, Hwang WS, Tu TC. 2017. Bioethanol production from recovered Napier grass with heavy metals. J Environ Manage. 203(Pt 3):1005–1010. doi: 10.1016/j.jenvman.2017.04.049.
- Kumar O, Singh SK, Latare AM, Yadav SN. 2018. Foliar fertilization of nickel affects growth, yield component and micronutrient status of barley (Hordeum vulgare L.) grown on low nickel soil. Archives of Agronomy and Soil Science. 64(10):1407-18. doi: 10.1080/03650340.2018.1438600.
- Ma C, Ming H, Lin C, Naidu R, Bolan N. 2016. Phytoextraction of heavy metal from tailing waste using Napier grass. Catena. 136:74–83. doi: 10.1016/j.catena.2015.08.001.
- Madhumita ROY, Roychowdhury R, Mukherjee P. 2018. Remediation of fly ash dumpsites through bioenergy crop plantation and generation: A review. Pedosphere. 28(4):561–580. doi: 10.1016/S1002-0160(18)60033-5.
- Maiti D, Pandey VC. 2020. Metal remediation potential of naturally occurring plants growing on barren fly ash dumps. Environ Geochem Health. 43(4):1415–1426. doi: 10.1007/s10653-020-00679-z.
- Majaule U, Dikinya O, Glaser B. 2022. Biochar and sewage sludge phosphorus fertilizer affects on phosphorus bioavailability and spinach (Spinacia oleracea L.) yields under no-till system in semi-arid soils. Int J Recyc Organ Waste Agric. 11(4):527–539. doi: 10.30486/ijrowa.2022.1927076.1231.
- Malumbela G, Masuku EU. 2017. Resources and strategies towards the development of a sustainable construction materials industry in Botswana. Int J Arch Civil Construct Sci. 10.0(2):108–114 doi: 10.5281/zenodo.1339934.
- Mohammed IY, Abakr YA, Mokaya R. 2019. Biofuel and valuable products recovery from Napier grass pre-processing: Process design and economic analysis. Journal of Environmental Chemical Engineering. 7(2):102962.doi: 10.1016/j.jece.2019.102962.
- Mohapatra S, Ray RC, Ramachandran S. 2019. Bioethanol from biorenewable feedstocks: technology, economics, and challenges. InBioethanol production from food crops. pp. 3-27. Academic Press. doi: 10.1016/B978-0-12-813766-6.00001-1.
- Motlhanka K, Lebani K, Garcia-Aloy M, Zhou N. 2022. Functional Characterization of Khadi yeasts isolates for selection of starter cultures. J Microbiol Biotechnol. 32(3):307–316. doi: 10.4014/jmb.2109.09003.
- Negawo AT, Teshome A, Kumar A, Hanson J, Jones CS. 2017. Opportunities for Napier grass (Pennisetum purpureum) improvement using molecular genetics. Agronomy. 7(2):28. doi: 10.3390/agronomy7020028.
- Nwaefuna AE, Rumbold K, Boekhout T, Zhou N. 2021. Bioethanolic yeasts from dung beetles: tapping the potential of extremophilic yeasts for improvement of lignocellulolytic feedstock fermentation. Biotechnol Biofuels. 14(1):86. doi: 10.1186/s13068-021-01940-y.
- Ogundele DT, Adio AA, Oludele OE. 2015. Heavy metal concentrations in plants and soil along heavy traffic roads in North Central Nigeria. Journal of Environmental & Analytical Toxicology. 5(6):1. doi: 10.4172/2161-0525.1000334.
- Osman NA, Roslan A, Ibrahim M, Hassan M. 2020. Potential use of Pennisetum purpureum for phytoremediation and bioenergy production: a mini review. APJMBB. 28(1):14–26. doi: 10.35118/apjmbb.2020.028.1.02.
- Pakwan C, Jampeetong A, Brix H. 2020. Interactive effects of N form and P concentration on growth and tissue composition of hybrid Napier grass (Pennisetum purpureum × Pennisetum americanum). Plants (Basel). 9(8):1003. doi: 10.3390/plants9081003.
- Pandey VC, Bajpai O, Singh N. 2016. Plant regeneration potential in fly ash ecosystem. Urban Forest Urban Green. 15:40–44. doi: 10.1016/j.ufug.2015.11.007.
- Semumu T, Gamero A, Boekhout T, Zhou N. 2022. Evolutionary engineering to improve Wickerhamomyces subpelliculosus and Kazachstania gamospora for baking. World J Microbiol Biotechnol. 38(3):48. doi: 10.1007/s11274-021-03226-9.
- Shah A, Niaz A, Ullah N, Rehman A, Akhlaq M, Zakir M, Suleman Khan M. 2013. Comparative study of heavy metals in soil and selected medicinal plants. Journal of Chemistry. 2013. 621265. doi: 10.1155/2013/621265.
- Taupedi S, Ultra VU. 2022. Morupule fly ash as amendments in agricultural soil in Central Botswana. Environ Technol Innov. 28:102695. doi: 10.1016/j.eti.2022.102695.
- Ultra VU. 2020. Fly ash and compost amendments and mycorrhizal inoculation enhanced the survival and growth of Delonix regia in Cu-Ni mine tailings. Philipp J Sci. 149(3):479–489. http://repository.biust.ac.bw/handle/123456789/381. doi: 10.56899/149.03.01.
- Van der Merwe EM, Prinsloo LC, Mathebula CL, Swart HC, Coetsee E, Doucet FJ. 2014. Surface and bulk characterization of an ultrafine South African coal fly ash with reference to polymer applications. Appl Surf Sci. 317:73–83. doi: 10.1016/j.apsusc.2014.08.080.
- Van Reeuwijk LP. 2002. Procedures for soil analysis. (6th edition). Technical Paper No. 9. FAO/ISRIC. Wageningen, the Netherlands. p. 120. pp.
- Wiangkham N, Prapagdee B. 2018. Potential of Napier grass with cadmium-resistant bacterial inoculation on cadmium phytoremediation and its possibility to use as biomass fuel. Chemosphere. 201:511–518. doi: 10.1016/j.chemosphere.2018.03.039.
- Wong JW, Li K, Fang M, Su DC. 2001. Toxicity evaluation of sewage sludges in Hong Kong. Environ Int. 27(5):373–380. doi: 10.1016/S0160-4120(01)00088-5.
- Yadav VK, Gacem A, Choudhary N, Rai A, Kumar P, Yadav KK, Abbas M, Khedher NB, Awwad NS, Barik D, et al. 2022. Status of coal-based thermal power plants, coal fly ash production, utilization in India and their emerging applications. Minerals. 12(12):1503. doi: 10.3390/min12121503.
- Zhai M, Totolo O, Modisi MP, Finkelman RB, Kelesitse SM, Menyatso M. 2009. Heavy metal distribution in soils near Palapye, Botswana: an evaluation of the environmental impact of coal mining and combustion on soils in a semi-arid region. Environ Geochem Health. 31(6):759–777. doi: 10.1007/s10653-009-9260-7.
- Zierold K, Odoh C. 2020. A review on fly ash from coal-fired power plants: chemical composition, regulations, and health evidence. Rev Environ Health. 35(4):401–418. doi: 10.1515/reveh-2019-0039.