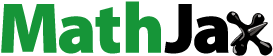
ABSTRACT
Black spruce trees lean to form “drunken” forest on degrading permafrost; however, the causes of tree leaning on continuous permafrost remain unclear. Leaning events are recorded by reaction wood formation in tree rings, and it remains unclear what soil conditions are required for reaction wood formation of drunken trees. Tree disk morphology and soil hummock properties were examined for fifty tree–mound combinations in Northwest Territories, Canada. Spruce trees growing on mound edges form reaction wood on the downslope sides of their trunks. Reaction wood formation in mature trees was greatest in stem tissues between ground level and 30 cm aboveground. Reaction wood formation occurred only in trees growing on mound edges. The extent of reaction wood formation was higher in trees growing in clayey soils than in trees on sandy soils. For trees growing on clayey mound edges, the extent of reaction wood formation decreased with increasing permafrost table depth. Black spruce tree rings formed between ground level and 30 cm aboveground could record movement of clayey soil hummocks over shallow, underlying permafrost tables. A combination of clayey soil texture and shallow permafrost table is likely required for development of hummocks and drunken forests on the continuous permafrost region studied.
Introduction
Circumpolar northern ecosystems located on permafrost may be greatly impacted by climate change, with knock-on effects that will potentially influence carbon cycles on a global scale (Schuur et al. Citation2015). Permafrost degradation caused by warming can be predicted at global and terrestrial ecosystem scales (Koven et al. Citation2011), but climate change impacts and potential feedbacks could vary among ecosystems (Camill Citation2005). In black spruce (Picea mariana L.) forest in a discontinuous permafrost zone, permafrost degradation (ground subsidence) causes tree leaning along with thaw, and trees will eventually die and topple (Euskirchen et al. Citation2014). This process leads to expansion of “drunken” forests and their replacement by bog (paludification). On the other hand, drunken forests formed before climate change started in forest–tundra ecosystems in continuous permafrost zones (Zoltai Citation1975). Predicting the effects of climate change on drunken forests on continuous permafrost will require a full understanding of the relationship between permafrost and the development of drunken forests.
Tree leaning could be caused by a permafrost-specific soil movement termed “cryoturbation” in continuous permafrost zones, as well as ground subsidence typically observed in discontinuous permafrost zones (Zoltai Citation1975). Typical soil surface microrelief is hummocky with mounds 1 to 3 m in diameter with depressions (or troughs) between them (Tarnocai and Zoltai Citation1978; Ping et al. Citation2015). Trees growing on hummocky microrelief tend to lean (Zoltai Citation1975). In addition to the effects of a hummocky soil microrelief, tree leaning can be caused by climatic factors (snow and wind) and disturbances (Kokelj, Burn, and Tarnocai Citation2007; Stoffel and Bollschweiler Citation2008). Some black spruce trees are able to remain vertical even in drunken forests in continuous permafrost zones. The underlying cause of drunken forest remains unresolved.
Hummocky microrelief is usually older than drunken trees, because surface polygons form through ice wedge formation and permafrost activities over thousands of years (Walker et al. Citation2008). On the other hand, soil hummocks may develop through the accumulation of organic matter (lichen and moss litters), debris, and ice aggregates through 200-year cycles of fire and forest regeneration (Kokelj, Burn, and Tarnocai Citation2007). The relationship between tree leaning and past or present mound formation is difficult to discern due to the complexity of geophysical and biological processes operating over multiple timescales.
Trees growing on mound slopes lean due to mound rising (Zoltai Citation1975). Leaning coniferous trees always try to return to the vertical posture by producing reaction wood on the downslope sides of their trunks (Stoffel and Bollschweiler Citation2008). Tree leaning events can be recorded as tree rings composed of lignin-rich round cells with thick cell walls, because gravitational imbalance of the tree triggers lignin production in wood (Yamashita, Yoshida, and Yamamoto Citation2009). Thus, formation of reaction wood in black spruce trees growing on soil mounds provides a record of leaning events in the annual rings, and this record allows the reconstruction of drunken forest development over time (Zoltai Citation1975; Kokelj, Burn, and Tarnocai Citation2007). The relationship between tree leaning and mound formation can be clarified by analyzing reaction wood and soil hummock morphology.
The soils of the northern Mackenzie watershed region in Canada that was uncovered by the Laurentide Ice Sheet were frozen during the glacial period. Drunken forests have developed in the forest–tundra ecozone located on continuous permafrost (Ritchie Citation2004). Because the higher specific heat capacity and ice contents in the clayey soils compared to sandy soils could drive greater cryoturbation due to the greater amounts of thawed water and shallower permafrost table (Abu-Hamdeh and Randall Citation2000; Huisman Citation2002), the different surface geological substrates from glaciofluvial sands and a mixture of fluvial clayey sediments and glacial till provide the wide variation in permafrost table depths and hummock sizes. Comparing reaction woods between clayey and sandy sediments across a range of permafrost table depths enables an analysis of the effects of soil texture and permafrost table depth on hummock and drunken forest dynamics. We test the hypothesis that development of drunken forest and hummocks is significant on clayey soil with a shallow permafrost table.
Materials and methods
Experimental sites
We investigated sixteen black spruce forests sites in the Mackenzie upland area between Inuvik and Tsiigehtchic, which are located in the Northwest Territories, Canada (; Fujii, Matsuura, and Osawa Citation2019). The upland around the Mackenzie delta is located in a continuous permafrost zone, although the Mackenzie delta is located in a discontinuous zone (Brown et al. Citation1997). Inuvik has an arctic climate; the mean annual air temperature is −8.8°C. Annual precipitation is 248 mm year−1, of which snow accounts for 131 mm year−1. The soil surface is covered by mounds bearing lichens (Cladina mitis L., Cladina stellaris L.) and mosses (Pleurozium schreberii L., Hylocomium splendens L.) with depressions filled with Sphagnum spp.
Figure 1. Locations of sampling sites in Northwest Territories, Canada. Sites 1-6 and 7-9 (filled circle) are located on hummocky microrelief on clayey and sandy sediments, respectively. Sites 10-13 and 14-16 (clear circle) are located on non-hummocky (flat) microrelief on clayey and sandy sediments, respectively
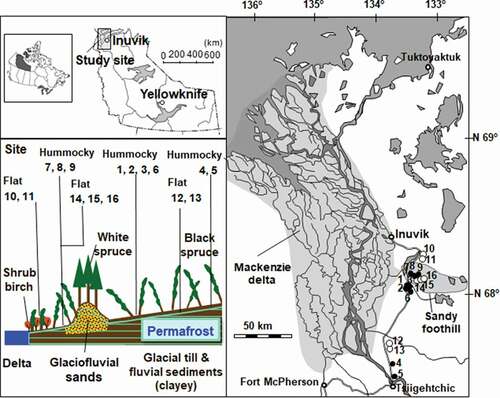
Soil microrelief survey
We investigated fifty mounds (1–3 m) in sixteen sites with soils of contrasting textures (thirty-eight mounds in ten clayey soil sites, twelve mounds in six sandy soils; ). To ensure independence of mounds, we studied hummocks located at least 20 m apart. Leaning trees typically grow on mound edges, not in depressions (Zoltai Citation1975). To analyze the relationship between hummocky microrelief and tree leaning, we collected disk samples from trees growing on mound edges and mound tops. Clayey soils are derived from a mixture of fine-grained glacial till (27–37 percent clay and 22–30 percent sand); sandy soils are derived from glaciofluvial sands (8–12 percent clay, 73–75 percent sand; ; Fujii et al. Citation2019). We measured depths from the thawed soil to the permafrost table in August (termed “active layer thickness”) by excavating two soil pits at higher and lower elevations surrounding the tree trunks along the mound slope direction (). If the tree trunks were located on mound edges, higher and lower elevations corresponded to mound top and depression, respectively. The differences in active layer thickness between higher and lower elevations were used as indicators of soil hummock formation ().
Figure 2. Schematic of soil hummocks showing measurements made on the mounds, in the depressions, and on leaning (drunken) trees
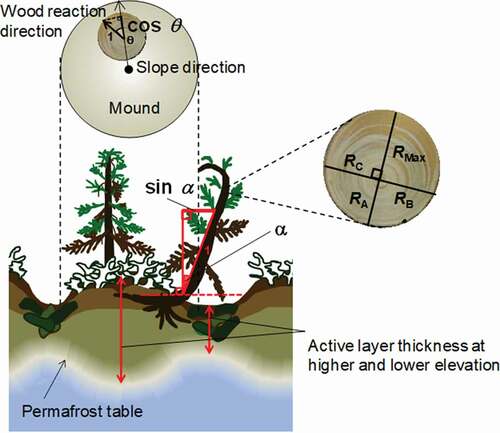
Table 1. Physicochemical properties of hummocky and non-hummocky soils on glaciofluvial sands and clayey sediments
Morphological analyses of wood disks
To determine the position of maximum reaction wood formation in tree trunks, we collected tree disk samples with different angles (α) of tree leaning relative to the vertical axis (). Tree trunks were cut at 10 cm below the ground (−10 cm), at ground level (0 cm), and at heights of 30, 130, 330, and 530 cm along the trunk (). To characterize the extent of tree leaning, a tree leaning index was defined as sin α (). In different angle (α) classes of tree leaning, the relationship between the extent of reaction wood formation and the distance along the tree trunk from the ground (0 cm) or tree leaning index (sin α) was assessed. In addition, to assess the relationship between the tree leaning direction and the mound slope, we measured the angle gap between the direction of wood reaction (the major axis, including the maximum wood radius) and the mound slope for the fifty disk samples, including the variation in extent of reaction wood (). The angle gap between reaction wood direction and mound slope direction (θ) was expressed as cosθ. ().
To evaluate the extent of reaction wood formation for fifty tree disks, the maximum wood radius was defined as RMax, and the radii in the other three directions, +180°, +90°, and −90° relative to the maximum disk radius orientation, were defined as RA, RB, and RC, respectively (). The extent of reaction wood formation was calculated from the ratio of the maximum wood radius (RMax) to the average radii in the other three directions (RA, RB, RC; ) as follows:
Dendrochronological analyses of wood disks
We used a stereomicroscope (MZ6, Leica, Solms, Germany) together with the Velmex “TA” system (Velmex Inc., Bloomfield, NY) for tree ring measurement to measure the annual widths of tree rings in two directions on the major axis, including the maximum wood radius and two perpendicular directions on the minor axis of the wood disk (a total of four radii). The tree ring data were visually cross-dated and statistically verified using COFECHA software (Holmes Citation1983).
Statistics
The extent of reaction wood formation in the clayey and sandy soil groups was expressed as mean ± standard error (SE). Two-way analysis of variance was used to evaluate statistical differences in mean soil carbon stocks by main effects (hummocky microrelief and soil types [clayey sediment vs. glaciofluvial sands]) and their interaction. The significance of differences in mean extents of reaction wood formation between the groups (tree sampling positions, mound edge vs. mound top, clayey sediment vs. glaciofluvial sands) was tested with analysis of variance or t tests (p < .05). The differences in linear regression slopes of reaction wood formation extents related to the tree leaning index (sin α) were tested using analysis of covariance between tree sampling positions. Pearson’s linear correlation was used to assess the relationships between extent of reaction wood formation and (a) tree leaning index (sin α), (b) permafrost table depth, or (c) difference in extent of reaction wood formation and active layer thickness between higher and lower elevations, respectively. The differences in linear regression slopes of extent of reaction wood formation related to time (year) were tested using analysis of covariance between trees growing on clayey mound edges, sandy mound edges, and mound tops. Statistical analyses were performed with Sigma Plot software v. 12.5 (SPSS Inc., Tokyo, Japan).
Results
Characteristics of soil microrelief
In clayey sediments, the active layer was composed of thick organic mats and shallow mineral soils; in sandy sediments, the active layer was dominated by mineral soil materials covered by a thin organic mat (). Two-way analysis of variance indicated that there was an interaction effect of hummocky microrelief and soil type (clayey vs. sandy) in soil carbon stocks in the mineral horizons (). Within clayey soils of similar clay contents, soil carbon stocks in the organic horizon were significantly (p < .05) greater in hummocky microrelief than in non-hummocky microrelief. There was no interaction effect for carbon stocks in the mineral soil horizons (). The soil carbon stock in the mineral soil horizons was influenced only by soil type, and the carbon stocks in clayey soils were significantly (p < .05) higher than those in sandy soils (). On well-developed clayey soils with hummocky microreliefs, trees grew on lichen-covered dry mounds with deep permafrost tables but not in the moss- and tussock-covered wet depressions with shallower permafrost tables. Among fifty mounds studied, we found twelve trees located on mound tops and thirty-eight on mound edges (). Consistent with the hypothesis, the mound size or differences in active layer thickness between higher elevation (mound tops for trees located at the mound edge) and lower elevation (depressions for trees located at the mound edge) were significantly greater (p < .05) in clayey soil mounds than in sandy mounds ().
Table 2. Morphological properties of soil hummocks and woods
Morphology of reaction woods
Tree stem profile analyses showed that the extent of reaction wood formation at ground level and 30 cm aboveground was significantly (p < .05) greater than that at higher positions (). Root growth direction and reaction wood formation below ground level (−10 cm) was affected by the presence of the permafrost table (). There were positive correlations between reaction wood formation and tree leaning index (sin α) at tree positions at ground level to 130 cm aboveground (). The regression slopes or wood reaction in response to tree leaning was significantly higher at 30 cm aboveground than at other positions ().
Figure 3. Relationship between reaction wood formation and (a) distance along the tree trunk and (b) tree leaning angle (a) relative to the vertical axis at different tree positions from the ground (0 cm). The different letters (A–D, a–d) indicate that the values are significantly (p < .05) different between positions along the tree trunk in each class of tree leaning angles (a). Asterisks indicate that regression slopes are significantly (P < 0.05) different between positions along tree trunk
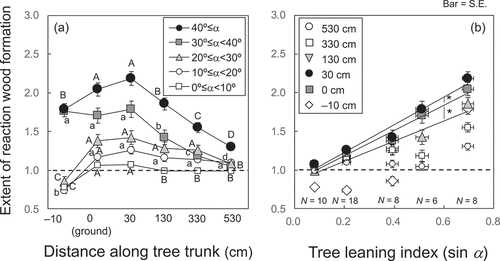
The extent of reaction wood formation was consistently low on sandy mounds (1.2 ± 0.1; ) but varied widely on clayey mounds (1.0–2.6; ). The extent of reaction wood formation on clayey mound edges (1.7 ± 0.1) was significantly (p < .05) greater than those on sandy mound edges (). The wide variation in the extent of reaction wood formation on clayey soils was related to the mound positions on which trees were located (). For tree disk samples collected from the clayey soil sites, the extent of reaction wood formation on mound edges (1.5 ± 0.1) were significantly (p < .05) greater than those on mound tops (1.2 ± 0.0; ). Trees standing vertically on mound tops had regular circular tree rings, but trees growing on mound edges formed reaction wood on the downslope sides of their trunks (). The maximum axis radii of trees with regular growth had diverse reaction directions relative to the mound slope direction (). The greatest extent of reaction wood formation was limited to the condition in which cosθ. was near 1.0 (), and there was a positive correlation between wood reaction direction (cosθ) and extent of reaction wood formation for trees on clayey mound edges (). Thus, the direction of reaction wood formation matched the direction of the mound slope in most cases.
The extent of reaction wood formation at sites with deep permafrost tables was consistently low regardless of mound position (). The extent of reaction wood formation varied widely in clayey soil sites with shallow permafrost tables (). Reaction wood formation extents greater than 1.5 were limited to trees growing on edges of clayey mounds and the extent of reaction wood formation was negatively correlated with permafrost table depth for trees growing on clayey mound edges (). For the data from clayey soils, the extent of reaction wood formation increased with increasing soil hummock size and was positively correlated with permafrost table depth gaps between higher and lower elevations ().
Figure 5. Relationships between the extent of reaction wood formation and permafrost table depth (active layer thickness) at lower elevation of mound edges and tops in clayey and sandy soils
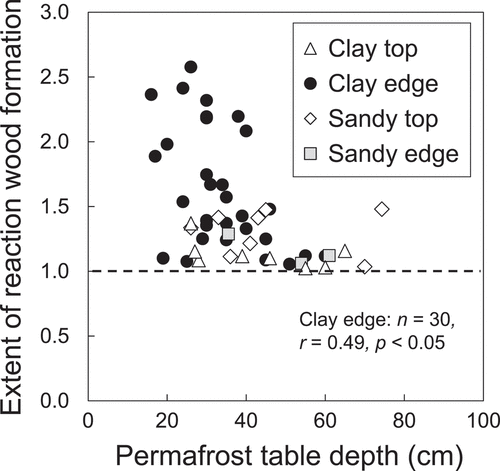
Figure 6. Relationships between the extent of reaction wood formation and the difference in active layer thickness between higher and lower elevations of mounds [Depth gap (A–B)]. Data sources are listed in
![Figure 6. Relationships between the extent of reaction wood formation and the difference in active layer thickness between higher and lower elevations of mounds [Depth gap (A–B)]. Data sources are listed in Table 2](/cms/asset/0fa4b8b6-a8e5-4c16-8461-8d0900de3362/uaar_a_1712858_f0006_b.gif)
When maximum and average tree ring widths were studied for normal and reaction woods based on dendrochronology, disturbance events were identifiable during the lives of the specimens examined (; Stoffel and Bollschweiler Citation2008). Both maximum and average tree ring widths decreased with tree age in the normal wood (), but periodic spikes in maximum tree ring widths (relative to average tree ring widths) were found in the reaction wood for trees growing on mound edges ().
Figure 7. Tree ring widths in wood disks of trees growing on clayey mound edges (Nos. 1–33; clear), growing on sandy mound edges (Nos. 39–44; yellow-colored), and exhibiting normal growth on non-hummocky microrelief (Nos. 17, 22, 46; shaded)
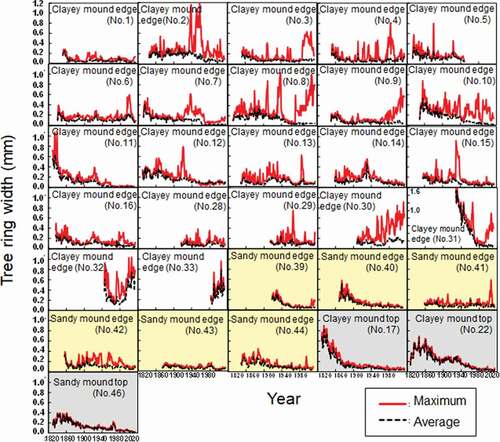
Tree leaning and hummock formation events can be reconstructed using tree ring records (). The mean extent of reaction wood formation increased with age, but the regression slopes were significantly (p < .05) different between trees growing on clayey mound edges, trees growing on sandy mound edges, and trees growing on non-hummocky microrelief (). The rates of reaction wood formation were significantly (p < .05) higher in trees growing on mound edges than in trees growing on non-hummocky microrelief (). Consistent with the hypothesis, the rates of reaction wood formation were significantly higher in trees growing on clayey mounds than in trees growing on sandy mounds ().
Figure 8. Reaction wood formation of trees growing on clayey mound edge (n = 22), trees growing on sandy mound edge (n = 6), and trees growing on non-hummocky soil (n = 3). The pink, blue, and gray bars indicate standard errors. The symbols (*) indicate that regression slopes are significantly (P < 0.05) different between positions along tree trunk
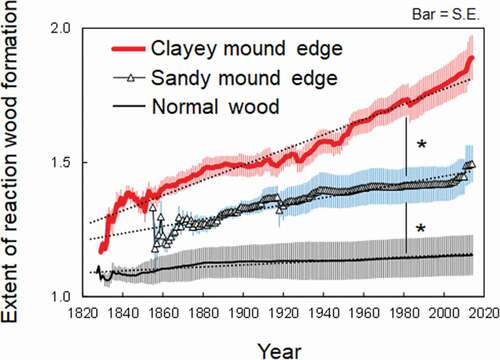
Discussion
Environmental factors regulating reaction wood formation
We found that the extent of reaction wood formation was variable, ranging from near zero in straight-growing trees to high values in drunken forests (). This variability was related to soil conditions, such as permafrost table depth, soil texture, and tree position on mound slopes (). The extent of reaction wood formation at the sites with deep permafrost tables was consistently small, but the extent of reaction wood formation could vary widely at sites with shallow permafrost tables (). This is consistent with the existing finding that drunken forests are associated with a shallow permafrost table in the continuous permafrost zone around the Mackenzie delta upland (Zoltai Citation1975; Kokelj and Burn Citation2003). Both limited root expansion on permafrost and hummocky soil microrelief can contribute to the development of drunken forests (Zoltai Citation1975; ). Because they have no distinct taproot, black spruce trees also expand their main root system toward deeper and warmer soil, escaping from cold and poorly drained soil on the shallow permafrost table (Noguchi et al. Citation2016). Therefore, a shallow permafrost table appears to be a fundamental requirement for the development of drunken forests (). This is consistent with the model of hummock formation proposed by Kokelj, Burn, and Tarnocai (Citation2007) in which soil hummocks develop along with the development of shallow permafrost following a fire. Our study indicates that a clayey soil texture promotes hummock formation.
In addition to the clay mound hypothesis tested, reaction wood formation occurred in black spruce trees growing on the edges of clayey mounds (). Soil texture influences hummocky microrelief through its effects on physical stability, water-holding capacity, and heat conductivity (Walker et al. Citation2008). Clayey soils have a higher water-holding capacity and a lower heat conductivity than sandy soils (Farouki Citation1981; Abu-Hamdeh and Randall Citation2000). The high ice content of clayey soils can generate strong upward pressures during mound formation (Kokelj and Burn Citation2003). The reduced heat conductivity of the thick organic mat on clayey soils may prevent deep thawing of permafrost in the depressions during summer and generate a large gap in active layer thickness between higher and lower elevations (). Clayey soil texture favors the development of hummocky microreliefs and drunken forests on shallow permafrost tables (), although permafrost table depths may also vary spatially and temporally as a result of variability in landscape, slope aspect, and fire history (Kokelj, Burn, and Tarnocai Citation2007).
Requirements for the development of drunken forests and soil hummocks
Tree leaning is not only caused by soil condition but is also influenced by factors such as snow, wind, and fire, which can affect the development of drunken forests. The effect of wind on tree leaning would likely be expressed as a function of the strength of winds during winter; however, we found that most tree leaning followed the direction of the mound slope (). The seasonal effect of snow on tree leaning is not recorded in the tree rings (Zoltai Citation1975). Because fires can destabilize soil foundations and collapse soil mounds, they can induce tree leaning toward directions other than the slope of the mound. We cannot rule out factors other than soil hummock formation as agents that induce tree leaning, but in general the coincidence between mound slope direction and reaction wood formation () supports the link between reaction wood formation and mound formation. Exceptions were found in dead trees and those growing on partially collapsed mounds, which were not included in our study. The tree rings of black spruce between ground level and 30 cm aboveground could reflect the sizes and slopes of clayey soil mounds with shallow permafrost tables (, , and ).
We found that drunken forest development is positively correlated with a hummocky microrelief in the studied areas ( and ). This differs from the mechanism of drunken forest development in discontinuous permafrost zones where permafrost degradation loosens the soil foundation that trees stand on (Schuur and Abbott Citation2011). When mounds have formed due simply to polygon formation over geological timescales, tree leaning events should occur continuously. Judging from the temporal spikes of reaction wood formation (), we predicted that periodic hummock growth should precede the respective peaks of tree leaning events. 14C dating suggests that earth hummock formation takes 3,000 to 6,000 years (Tarnocai and Zoltai Citation1978), but tree ring records suggest that soil hummock formation may contribute to the development of drunken forests over about 200 years (). The relatively fast hummock formation is associated with accumulation of organic matter (lichen and moss litters), debris, and ice aggregation (Kokelj, Burn, and Tarnocai Citation2007; Walker et al. Citation2008). In continuous permafrost zones, the development of drunken forests and well-developed hummocks can be promoted by shallow permafrost tables and clayey soil textures ( and ).
The lower amount of reaction wood formation on soils with a deeper active layer () suggests that drunken forests should diminish when future warming increases the active layer thickness. This prediction is concordant with a scenario in which warming induces hummock collapse by permafrost degradation, causing a vegetation shift toward white spruce dominance on well-drained soils or, on poorly drained soils, to shrub and tussock tundra (Schuur et al. 2007; Turetsky et al. Citation2007). Such a fate of vegetation covers depends on overall temperature and nutrient availability, as well as water drainage (Van Cleve and Yarie Citation1986). The extensive effects of climate warming on permafrost degradation and vegetation shift have been proposed by several studies (Lantz, Gergel, and Kokelj Citation2010; Pries, Schuur, and Crummer Citation2012), but our data suggest that the potential impacts of permafrost degradation on hummocky microrelief and drunken forest development could be more sensitive in clayey sediments than in sandy sediments ().
Conclusions
Reaction wood formation was greatest in trees growing on the edges of clayey mounds with shallow permafrost tables. The extent of reaction wood formation between ground level and 30 cm aboveground in mature black spruce may be a proxy for soil hummock development over a tree’s life span (ca. 200 years) that could be used for the reconstruction of soil hummocks. The occurrence of drunken forests is not a phenomenon that responds to geological polygonal microrelief; it changes with soil hummock formation over biological timescales (tree ages). Future warming may decrease the extent of drunken forests on hummocky microrelief when the active layer thickness exceeds 50 cm due to permafrost degradation ().
Acknowledgments
We thank the government of the Northwest Territories for issuing a license for our research (No. 16174). We are grateful to Dr. Darwin Anderson at the University of Saskatchewan for providing valuable advice and to Soichiro Fujii for assistance with the field survey.
Disclosure statement
No potential conflict of interest was reported by the authors.
Additional information
Funding
References
- Abu-Hamdeh, N. H., and C. R. Randall. 2000. Soil thermal conductivity effects on density, moisture, salt concentration, and organic matter. Soil Science Society of America Journal 64:1285–90. doi:https://doi.org/10.2136/sssaj2000.6441285x.
- Brown, J., O. J. Ferrians Jr., J. A. Heginbottom, and E. S. Melnikov. 1997. Circum-Arctic map of permafrost and ground-ice conditions, 45. Reston, VA: US Geological Survey.
- Camill, P. 2005. Permafrost thaw accelerates in boreal peatlands during late-20th century climate warming. Climatic Change 68 (1–2):135–52. doi:https://doi.org/10.1007/s10584-005-4785-y.
- Euskirchen, E. S., C. W. Edgar, M. R. Turetsky, M. P. Waldrop, and J. W. Harden. 2014. Differential response of carbon fluxes to climate in three peatland ecosystems that vary in the presence and stability of permafrost. Journal of Geophysical Research: Biogeosciences 119:1576–95.
- Farouki, O. T. 1981. Thermal properties of soils (No. CRREL-MONO-81-1). Hannover, NH: Cold Regions Research and Engineering Laboratory.
- Fujii, K., Y. Matsuura, and A. Osawa. 2019. Effects of hummocky microrelief on organic carbon stocks of permafrost-affected soils in the forest-tundra of northwest Canada. Pedologist 63:12–25.
- Holmes, R. 1983. Program COFECHA user’s manual. Tucson: University of Arizona, Laboratory of Tree-Ring Research.
- Huisman, L. M. 2002. Development of compression wood in trees of the “drunken forest”, Central Yukon Territory. Doctoral dissertation, Carleton University. Available https://curve.carleton.ca/f9b1ce5c-f0d8-4896-ab65-fb586324fb58
- Kokelj, S. V., and C. R. Burn 2003. “Drunken forest” and near surface ground ice in Mackenzie Delta, Northwest Territories, Canada. In Proceedings of the Eighth International Conference on Permafrost, L. U. Arenson, M. Phillips, and S. M. Springman, eds. Vol. 1. Boca Raton: CRC Press. 567–72.
- Kokelj, S. V., C. R. Burn, and C. Tarnocai. 2007. The structure and dynamics of earth hummocks in the subarctic forest near Inuvik, Northwest Territories, Canada. Arctic Antarctic, and Alpine Research 39:99–109. doi:https://doi.org/10.1657/1523-0430(2007)39[99:TSADOE]2.0.CO;2.
- Koven, C. D., B. Ringeval, P. Friedlingstein, P. Ciais, P. Cadule, D. Khvorostyanov, G. Krinner, and C. Tarnocai. 2011. Permafrost carbon-climate feedbacks accelerate global warming. Proceedings of the National Academy of Sciences of the United States of America 108:14,769–14,774. doi:https://doi.org/10.1073/pnas.1103910108.
- Lantz, T. C., S. E. Gergel, and S. V. Kokelj. 2010. Spatial heterogeneity in the shrub tundra ecotone in the Mackenzie Delta region, Northwest Territories: Implications for Arctic environmental change. Ecosystems 13:194–204. doi:https://doi.org/10.1007/s10021-009-9310-0.
- Noguchi, K., Y. Matsuura, S. D. Sparrow, and L. D. Hinzman. 2016. Fine root biomass in two black spruce stands in interior Alaska: Effects of different permafrost conditions. Trees 30:441–49. doi:https://doi.org/10.1007/s00468-015-1226-z.
- Ping, C. L., J. D. Jastrow, M. T. Jorgenson, G. J. Michaelson, and Y. L. Shur. 2015. Permafrost soils and carbon cycling. Soil 1:147–71. doi:https://doi.org/10.5194/soil-1-147-2015.
- Pries, C. E. H., E. A. Schuur, and K. G. Crummer. 2012. Holocene carbon stocks and carbon accumulation rates altered in soils undergoing permafrost thaw. Ecosystems 15:162–73. doi:https://doi.org/10.1007/s10021-011-9500-4.
- Ritchie, J. C. 2004. Post-glacial vegetation of Canada. Cambridge: Cambridge University Press.
- Schuur, E. A. G., A. D. McGuire, C. Schädel, G. Grosse, J. W. Harden, D. J. Hayes, G. Hugelius, C. D. Koven, P. Kuhry, D. M. Lawrence, et al. 2015. Climate change and the permafrost carbon feedback. Nature 520:171–79. doi:https://doi.org/10.1038/nature14338.
- Schuur, E. A. G., and B. Abbott. 2011. Climate change: High risk of permafrost thaw. Nature 480 (7375):32. doi:https://doi.org/10.1038/480032a.
- Schuur, E. A. G., K. G. Crummer, J. G. Vogel, and M. C. Mack. 2007. Plant species composition and productivity following permafrost thaw and thermokarst in Alaskan tundra. Ecosystems 10:280–92. doi:https://doi.org/10.1007/s10021-007-9024-0.
- Stoffel, M., and M. Bollschweiler. 2008. Tree-ring analysis in natural hazards research – An overview. Natural Hazards Earth System Science 8:187–202. doi:https://doi.org/10.5194/nhess-8-187-2008.
- Tarnocai, C., C. A. S. Smith, and C. A. Fox. 1993. International tour of permafrost affected soils: The Yukon and Northwest Territories of Canada, 197. Ottawa: Centre for Land and Biological Resources Research, Research Branch, Agriculture Canada.
- Tarnocai, C., and S. C. Zoltai. 1978. Earth hummocks of the Canadian Arctic and Subarctic. Arctic and Alpine Research 10:581–94. doi:https://doi.org/10.2307/1550681.
- Turetsky, M. R., R. K. Wieder, D. H. Vitt, R. J. Evans, and K. D. Scott. 2007. The disappearance of relict permafrost in boreal North America: Effects on peatland carbon storage and fluxes. Global Change Biology 13:1922–34. doi:https://doi.org/10.1111/gcb.2007.13.issue-9.
- Van Cleve, K., and J. Yarie. 1986. Interaction of temperature, moisture, and soil chemistry in controlling nutrient cycling and ecosystem development in the taiga of Alaska. In Forest ecosystems in the Alaskan taiga, ed. K. Van Cleve, 160–89. New York, NY: Springer.
- Walker, D. A., H. E. Epstein, V. Romanovsky, C. L. Ping, G. J. Michaelson, R. Daanen, Y. Shur, R. A. Peterson, W. B. Krantz, M. K. Raynolds, et al. 2008. Arctic patterned-ground ecosystems: A synthesis of field studies and models along a North American Arctic Transect. Journal of Geophysical Research 113:G03S01. doi:https://doi.org/10.1029/2007JG000504.
- Yamashita, S., M. Yoshida, and H. Yamamoto. 2009. Relationship between development of compression wood and gene expression. Plant Science 176:729–35. doi:https://doi.org/10.1016/j.plantsci.2009.02.017.
- Zoltai, S. C. 1975. Tree ring record of soil movements on permafrost. Arctic and Alpine Research 7:331–40. doi:https://doi.org/10.2307/1550177.