ABSTRACT
The arctic tern (Sterna paradisaea) is a ubiquitous migratory seabird of the High Arctic, currently thought to be in decline in most of the circumpolar world, but surprisingly little is known of its biology at high latitudes. We studied organ size and nutrient reserves of arctic terns breeding beside a High Arctic polynya in Nunavut, Canada, from their arrival at the colony into the chick-rearing period. Both males and females had a decrease in gizzard size through breeding, with gizzard mass during chick-rearing 39 percent lower than on arrival at the breeding grounds. Through the duration of the breeding season, heart, liver, and small intestine showed little change, but females had higher fat and protein stores than males. Terns from this colony likely have increasing fat levels and high body condition due to proximity to a highly productive polynya, where terns appear to gain more energy than they expend during foraging trips. This suggests that though terns at this colony may be near the northern limit of their range, local conditions have a strong impact on organ and nutrient reserve dynamics of these arctic seabirds.
Introduction
Extensive evidence shows that marine environments in many regions are changing rapidly due to anthropogenic activities (e.g., Watson and Tidd Citation2018), pollution (e.g., Bustnes et al. Citation2010; Clayden et al. Citation2015; Provencher et al. Citation2019), and broad-scale climatic shifts (e.g., Bryndum‐Buchholz et al. Citation2019). Seabirds are important indicators of marine ecosystem health because they feed at upper trophic levels; thus, their ecology and behavior are sensitive to changes in the marine environment (Furness and Camphuysen Citation1997; Parsons et al. Citation2008; Mallory, Karnovsky et al. Citation2010). For example, in the Arctic, poor seabird body condition and delayed breeding phenology can indicate low food availability, often due to late or extensive sea ice concentrations (Gaston, Gilchrist, and Mallory Citation2005; Moe et al. Citation2009). Understanding how breeding seabirds employ different energy use strategies to enable breeding at their range limit is critical to interpreting how changes in ecology and behavior may be linked to changing environments.
Changes in body condition in seabirds occur due to fluctuations in physiological or environmental factors such as molt or diet (Raveling Citation1979; Kitaysky, Wingfield, and Piatt Citation1999). In particular, the breeding season can be energetically demanding for different seabird species, because some fast for periods during incubation, and all experience the high energy demand of chick-rearing (Jacobs et al. Citation2011). Fluctuations in body mass, lipids, and proteins during the breeding season, along with the phenotypic flexibility of organ size, allow for the monitoring of changes in body condition over time to determine potential causes behind these changes. For example, digestive organs (e.g., gizzard) of some breeding birds may atrophy during fasting to reduce flight costs but then increase after chick-rearing for refueling before fall migration (Ankney Citation1977; Mallory, Robinson et al. Citation2010; Jacobs et al. Citation2011; Mathot et al. Citation2019). These changes in organ size and lipid reserves often result in similar changes in body mass.
Body mass loss during the breeding season has been recorded in many bird species (Moreno Citation1989). This decline in mass is thought to result from the higher energetic costs of breeding (compared to the nonbreeding season [reproductive stress hypothesis]; Wendeln and Becker Citation1996) or from an adaptation in anticipation for these higher energetic costs (programmed anorexia; Ankney Citation1977). However, the body components responsible for mass loss may differ between taxonomic groups, likely due to differences in life histories (Jacobs et al. Citation2011). Small seabirds, such as terns, have small energy reserves but energetically demanding foraging and chick-rearing strategies (Furness and Tasker Citation2000; Jodice et al. Citation2003). Thus, changes in food availability can result in subsequent changes in seabird body mass (Moreno Citation1989; Furness and Camphuysen Citation1997; Furness and Tasker Citation2000), but which organs or tissues contribute to these mass losses are not well understood.
Changes in body mass of common (Sterna hirundo) or arctic tern (S. paradisaea) have been previously examined in the North Sea (Langham Citation1968; Monaghan et al. Citation1989; Frank and Becker Citation1992; Wendeln and Becker Citation1996; Suddaby and Ratcliffe Citation1997). Terns lost body mass during incubation (Langham Citation1968; Wendeln and Becker Citation1996), even in food-rich colonies (Monaghan et al. Citation1989). However, research on arctic tern body condition is limited, especially in the Arctic where it is difficult to measure due to the remoteness and cost of working in this area (Mallory, Davis et al. Citation2018). Body condition may vary by geographic region due to differences in food availability, weather and climate, predation pressure, and more (Monaghan et al. Citation1989; Mallory et al. Citation2008; Jacobs et al. Citation2011); thus, it is unclear whether we can use information acquired from one region to model conditions in other regions.
The arctic tern is a small, surface-feeding seabird that generally nests on low ground close to shore in the Arctic (Hatch Citation2002). It has a circumpolar breeding range from 84° N in Greenland to 42° N in North America (Hatch Citation2002) and has the longest known migration of any organism, traveling over 80,000 km annually between breeding and wintering locations (Egevang et al. Citation2010). Despite their abundance, less is known about arctic terns in the Arctic portion of their range compared with other regions (Hatch Citation2002; Mallory et al. Citation2017). The Arctic is especially important to study because ice and snow conditions can limit food availability for seabirds, thus changing their reproductive schedule (Gaston, Gilchrist, and Mallory Citation2005). Additionally, long-distance migrants are thought to be more affected by environmental changes than short-distance migrants (Robinson et al. Citation2009); thus, as effects of climate change continue to manifest themselves on marine environments (Laxon, Peacock, and Smith Citation2003), it is important to have a benchmark of information on breeding arctic terns in this region to compare future years.
We investigated temporal changes in six different organs and nutrient reserves of arctic terns during the breeding season, from prelaying to chick-rearing, in the Canadian High Arctic. Body condition changes were examined in relation to sex and breeding stage. Based on the reproductive stress and programmed anorexia hypotheses, we predicted that body condition would decrease during the breeding season. We expected that gizzard mass would decline as shown in many other migrants (e.g., Mathot et al. Citation2019), but otherwise we had no a priori expectations of which organs would show significant changes and which would not during the breeding season.
Methods
Study site
Research was conducted in the Canadian High Arctic at Nasaruvaalik Island, a 3 × 1 km island in Penny Strait, Nunavut (75°49′ N, 96°18′ W, ; Maftei, Davis, and Mallory Citation2015) during the breeding season of 2007. Situated in a polynya, a usually productive area of open water surrounded by pack ice and maintained by current, tides, or upwellings (Stirling Citation1997; Hannah, Dupont, and Dunphy Citation2009), the island maintains one of the highest species diversities of seabirds in the North American Arctic (Maftei, Davis, and Mallory Citation2015). Supporting approximately 900 arctic terns, Nasaruvaalik Island may be the largest tern colony in the Canadian High Arctic (Maftei, Davis, and Mallory Citation2015). However, recent studies suggest that arctic tern populations in the circumpolar Arctic are in decline (BirdLife International Citation2018), and declines are evident around our study site (Maftei, Davis, and Mallory Citation2015; Mallory, Gilchrist et al. Citation2018).
Figure 1. Location of the study site at Nasaruvaalik Island in Penny Strait, Nunavut (75°49′ N, 96°18′ W), in the Canadian High Arctic (Mallory et al. Citation2017)
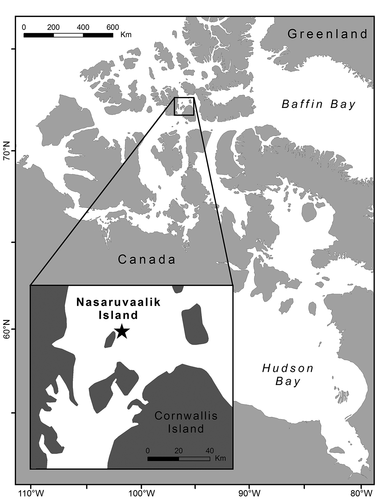
At our field site, first eggs are laid typically around 6 July (Mallory, Gilchrist et al. Citation2018), and terns have an egg formation duration of approximately seven days, with one to two days between eggs (Hatch Citation2002). We shot birds at nest sites using a 12 gauge shotgun and #6 steel shot, with collections occurring during prelaying (27–28 June; n = 11), early incubation (7 July; n = 10), late incubation (17–19 July; n = 10), and chick-rearing (4 August; n = 10). Carcasses were labeled with a unique identifier tag on the leg and then placed individually in small plastic bags and placed in a −20°C freezer within 2 hours of collection. These specimens were collected to answer a variety of research questions on wildlife health (e.g., diet, parasites, and contaminants; Provencher et al. Citation2014; Pratte et al. Citation2018; Mallory et al. Citation2019).
Measuring body condition
We shipped frozen carcasses to the Long Point Waterfowl and Wetlands Research Fund Avian Energetics Laboratory, where they were thawed and standard morphometrics were taken (e.g., head and keel length, wing cord, tarsus length, culmen length, head width, all in millimeters; see also Provencher et al. Citation2014). Technicians then removed all feathers except the remiges, which were cut with scissors where they emerged from the skin, and the plucked bird was reweighed (plucked body mass, g). They removed the leg and breast muscle from the left side of the body (Alisauskas and Ankney Citation1985), as well as the heart, liver, gizzard, large and small intestines, and weighed (±0.01 g) or measured (± 0.1 mm) each component. Intestinal contents were removed and these organs were reweighed.
For nutrient analyses, liver, leg, and breast muscles were dried to constant mass at 80°C to 95°C (Kerr, Ankney, and Millar Citation1982). The intestine and the remainder of the carcass (excluding the half breast, one leg, liver, feathers, and gonads/oviduct) were weighed (g), homogenized in a food grinder, and oven-dried at 80°C to 95°C to constant dry mass. The dried liver, dried breast and leg muscles, and dried carcass homogenate were ground separately with a portable coffee grinder. Lipid (hereafter fat) was extracted from a 1 to 2 g subsample of each of the ground body parts, using petroleum ether in a modified Soxhlet apparatus for 8 hours (Dobush, Ankney, and Krementz Citation1985). We subtracted the mass of the resultant lean dry sample from the dry mass of the original sample to obtain the mass of fat in the sample. A subsample of the lean dry residue from each extracted homogenate was placed in a preweighed crucible and heated in a furnace at 550°C for 6 hours. The remaining ash was weighed and divided by the mass of the lean dry sample to obtain the fraction of ash in each sample. We determined total fat in each body part (carcass, liver, breast, leg) by multiplying the dried mass of the body part by the proportion of fat in the subsample of homogenate. Similarly, we determined total ash in each body part by multiplying the fraction of ash in the subsample by the lean dry mass of the body part. Ash-free lean dry mass (a measure of protein content) was calculated as the dry mass of the body part minus total fat and ash.
Statistical analyses
Analyses were conducted in RStudio 1.2.5 (RStudio Team Citation2019). To compare tern body mass between sex and breeding stage, we used size-corrected body mass to account for potential variation in structural size between male and female terns. Size-corrected body mass was calculated by using the plucked body mass (g)/head length (mm); head length is a reliable index of body size in many seabirds, including terns (Devlin, Diamond, and Saunders Citation2004). Note that both body mass and endogenous reserves (lipid, protein, ash) were not significantly correlated with any metrics of structural size (head, culmen, tarsus and wing cord, or principal components derived from these; Pearson correlations, all r35 < 0.25, all p > .05). Birds with longer wings had larger gizzards, although the correlation was not strong (r36 = 0.34, p < .05), but otherwise organ size was not significantly correlated with any measures of structural size (all p > .05). Size-corrected organ size (heart mass [g], gizzard mass [g], liver mass [g], and small intestine mass [g] and length [mm]) and size-corrected body mass (g/mm), body lipid, and body protein were compared between sex and breeding stage using two-way analyses of variance with a sex–breeding stage interaction term. We report results from size-corrected body components (mass, organs, reserves), but in all cases statistical significance of results was the same if we used uncorrected component size. Normality checks and Levene’s tests were carried out and assumptions were met. All significance levels were set at .05, and means are presented ± standard deviation.
Results
Of the forty adult arctic terns collected, nineteen were male and twenty-one were female. Body mass of breeding terns ranged from 104 to 117 g. Male terns had a slightly larger head length (71.4 ± 1.8 mm) than females (69.6 ± 1.7 mm; t34 = 2.78, p = .009), but male culmen (32.0 ± 1.8 mm) and tarsus (15.4 ± 0.7 mm) lengths were similar to those of females (culmen 31.0 ± 2.3 mm, tarsus 15.8 ± 0.7 mm; both p ≥ .08). Terns did not differ in size-corrected body mass between sexes (analysis of variance; F1,31 = 2.819, p = .103), with females averaging 111 ± 4 g with feathers (89 ± 8 g plucked) compared to 111 ± 3 g (84 ± 5 g) for males (). Structural size (as indexed by head length) was similar across all stages of breeding (F1,37 = 1.366, p = .266). Body mass was also similar across breeding stages (p = .67; all comparisons had similar results when using body mass without size correction), although the pairwise direction of change was negative between prelaying and chick-rearing (). Females had significantly more fat (23 percent; F1,32 = 5.5, p = .02) and protein (5 percent; F1,32 = 14.5, p = .001) than males, but ash (largely mineral components) was similar between sexes and among sampling dates (all p > .07). In both sexes, protein was similar across breeding stages (all p = .78). Fat stores in males and females increased from prelaying to chick-rearing by 54 percent and 30 percent, respectively (, F3,32 = 4.0, p = .01). There was no significant interaction between sex and breeding stage on all factors (all F3,32 < 1.94, all p > .14).
Table 1. Mass (±SD) and percentage change in body tissues in male and female arctic terns during prelaying and chick-rearing
Figure 2. Changes in arctic tern (Sterna paradisaea) body mass (size-corrected dry mass) and associated organ sizes (lipid, protein, gizzard, liver, small intestine) through prelaying (P), early incubation (E), late incubation (L), and chick-rearing (C) on Nasaruvaalik Island, Nunavut, Canada, in 2007. Black circles are females (n = 5, 5, 5, 6) and gray circles are males (n = 6, 4, 4, 5). Circles represent mean values and vertical lines represent the 95 percent confidence interval
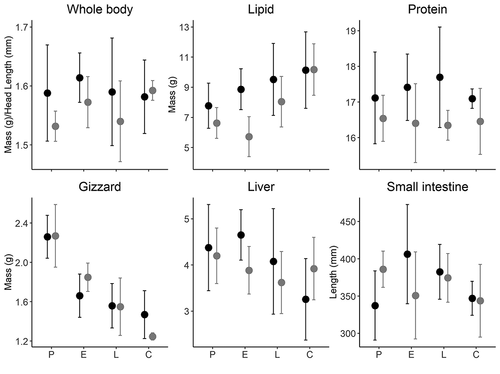
The majority of arctic tern organ length and mass did not change substantially throughout the breeding season (). Heart mass, small intestine length and mass, and liver mass were similar between males and females (all p > .46) and throughout the breeding season (all p > .16; ). Gizzard mass was similar between males and females (F1,32 = 0.51, p = .48) but was highest during prelaying and declined by 39 percent by chick-rearing (F3,32 = 20.5, p < .001; ).
Discussion
The average body mass of arctic terns that we collected on Nasaruvaalik Island in 2007 was 111 g, similar to masses reported for breeding arctic terns elsewhere (Hatch Citation2002). However, breeding arctic terns from the same colony averaged 107 (±8 g) over five breeding seasons (Mallory et al. Citation2017), suggesting that 2007 was a year where terns were in good body condition. It is thought that adults weighing less than 90 g are in poor condition (Monaghan et al. Citation1989; Hatch Citation2002) and thus may have lower reproductive success (Avery et al. Citation1992; Suddaby and Ratcliffe Citation1997). The lowest mass that has been measured for actively breeding terns at this site was 92 g (Mallory et al. Citation2017), above this hypothesized threshold. In years of poor breeding at this colony, mean tern body mass was ≥103 g, which may reflect that only terns in better condition even attempt to breed at this site in poor years.
Throughout breeding, we found that there were no statistically significant body mass changes in arctic terns (although our sample sizes were small), contrary to Langham (Citation1968) and Monaghan et al. (Citation1989), both of whom found significant decreases in arctic tern body mass during the breeding season. Similar adult body mass at this colony may be due to the foraging range of arctic terns in this region. Arctic terns at this colony forage within 2 km of their breeding colony (many can be watched with the naked eye moving from nest site to feeding; Mallory pers. obs.), whereas at lower latitudes, they can forage up to 30 km away (Black and Diamond Citation2004; Perrow, Skeate, and Gilroy Citation2011). Thus, these terns may be able to better maintain energetic reserves because they expend less energy while foraging.
Despite similar body mass from prelaying through chick-rearing, male and female terns managed their reserves differently. Fat increased from the prelaying to chick-rearing period in both males and females, unlike other arctic terns (Johnston Citation1964) or northern fulmars (Fulmarus glacialis) and black-legged kittiwakes (Rissa tridactyla; Golet and Irons Citation1999; Jacobs et al. Citation2011). We note, however, that the average increase of 3.8 g of fat for males and 2.3 g for females over the breeding season () represents approximately 150 kJ and 91 kJ of energy, respectively (assuming 39.4 kJ/g for fat; Nagy Citation1983; Groscolas, Schreiber, and Morin Citation1991), and this represents 45 percent and 27 percent of daily field metabolic rate (Uttley, Tatner, and Monaghan Citation1994). Thus, fat accumulation provides a small but presumably important energetic buffer for these birds during a short breeding window (Mallory et al. Citation2017). Nonetheless, the increasing levels of fat reserves in terns from this colony may be because it is situated in a highly productive polynya, suggesting that terns gain more energy than they spend during foraging trips (Frank and Becker Citation1992; Wendeln and Becker Citation1996; Stirling Citation1997). At this site, terns rely heavily on zooplankton (e.g., Gammarus setosus, Calanus hyperboreus, Gamaracanthus spp.; ) that are typically energy and/or lipid rich (Percy and Fife Citation1981; Scott et al. Citation2000) and abundant in the polynya less than 1 km away (Clayden et al. Citation2015; Pratte et al. Citation2018; Mallory pers. obs.), which may have allowed terns to minimize change in body mass with relatively easy replenishment of stores during breeding compared to arctic terns breeding elsewhere. This suggests that though this colony may be on the geographic extreme for the species, with a very short breeding season and limited foraging locations due to extensive ice cover, the physical location near the polynya is what allows birds to breed in the region in high numbers. Additionally, terns may rely less on fat reserves than other seabirds to fuel incubation if local food supplies are abundant. For example, incubation shifts in arctic terns range from 1 minute to approximately 4 hours in length with an average 22-day incubation period (Hatch Citation2002), compared to a 4.6-day incubation shift (Mallory, Hatch, and Nettleship Citation2012) and 49-day incubation period (Mallory and Forbes Citation2013) in fulmars; the latter relies heavily on stored fat to provide energy for incubation.
Figure 3. Zooplankton from one regurgitation of an arctic tern captured during the breeding season at Nasaruvaalik Island. Photo by Mark Mallory
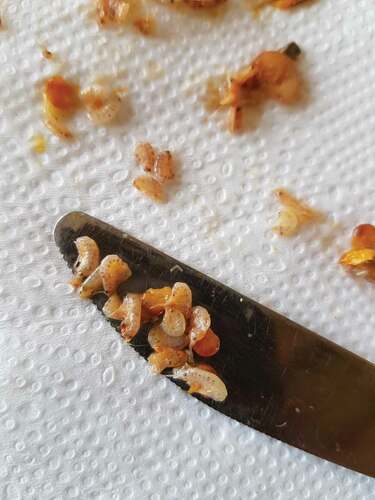
Females had more fat and protein than males throughout breeding. Given that males are often structurally slightly larger than females (Hatch Citation2002; this study), the lower levels of reserves in males suggest that they operate with a smaller energy buffer than females through the breeding season. Available evidence suggests that male arctic terns probably spend more time in energetically expensive activities during breeding than females (other than laying the egg) as follows: (1) during prelaying, males fly considerably more to forage for courtship prey to provision the female while she waits at the nest site; (2) females common terns and arctic terns spend more time on the nest than males during incubation; and (3) in both species, females spend more time brooding chicks than males (Hatch Citation2002; Nisbet et al. Citation2017; Mallory pers. obs.). For the latter two points, larger endogenous reserves would be adaptive for females who spend less time foraging (and thus having time to replenish energy supplies). Consequently, our observation of larger nutrient reserves in females than in males is consistent with behavior of the sexes during breeding.
In both sexes, gizzard size significantly decreased from incubation to chick-rearing, as found in fulmars (Mallory, Karnovsky et al. Citation2010; Jacobs et al. Citation2011), geese (Ankney Citation1977; Raveling Citation1979), and many shorebirds (e.g., Van Gils et al. Citation2005). Raveling (Citation1979) suggested that gizzard mass decline in female geese was largely due to egg laying. Gizzard mass in female terns declined by 26 percent from prelaying to early incubation (compared to an 18 percent decline in males). In females, we suspect that some of these endogenous protein resources were used for egg formation (see also Hatch Citation2002), although the majority of resources would have come from local exogenous foods because terns are income breeders (relying on resources from the local environment rather than stored internally; Hobson, Sirois, and Gloutney Citation2000; Pratte et al. Citation2018). The main driver of the decrease in gizzard size might be the relative disuse of this organ during egg laying (females) and incubation (both) compared to heavy use during the energetically expensive, northward migration (Raveling Citation1979; Jacobs et al. Citation2011; Mathot et al. Citation2019); this could also be affected by a shift in diet during these periods (Pratte et al. Citation2018; Mallory et al. Citation2019). Unlike observed declines in gizzard size, male and female terns showed no consistent change in the size of other organs, suggesting that these other organs are not as plastic through the breeding season.
Plasticity in seabird organs and tissue sizes is a result of both life history and external environmental conditions (Jacobs et al. Citation2011). In this study, we only examined temporal changes in tissues and organs during the breeding season. There are other confounding variables, such as food availability, that directly influence seabird body condition (Cairns Citation1988; Chastel, Weimerskirch, and Jouventin Citation1995). For example, Wendeln and Becker (Citation1996) found that reduced availability of energy-rich food in common tern colonies causes a decline in female body mass, particularly during incubation. In Alaska, kittiwakes in food-poor colonies had lower body condition and higher stress hormone levels than kittiwakes in food-rich colonies (Kitaysky, Wingfield, and Piatt Citation1999). Low body mass can limit reproduction (Suddaby and Ratcliffe Citation1997) and thus food availability should be considered in body condition research. In 2007 (and many other years), we observed terns flying short distances to the open water in the polynya, diving repeatedly, and returning, typically with large loads of zooplankton (), and these years were usually associated with good breeding success (Mallory et al. Citation2017). However, in other years, despite apparent abundant food supplies, predation pressure (from gulls, jaegers, polar bears Ursus maritimus, etc.) was much higher and may have precluded terns from foraging or incubating effectively, leading to reduced body condition and reproductive success (Mallory et al. Citation2017). Furthermore, increasingly warm, wet springs with extensive snow and sea ice cover (Boisvert and Stroeve Citation2015) may reduce nesting and foraging options for terns (i.e., physically covering nesting or foraging sites) and increase thermoregulatory costs, all of which may reduce body condition and reproductive success (Gabrielsen, Mehlum, and Karlsen Citation1988). Unfortunately, in these years where snow and sea ice remain relatively later than normal, terns typically arrive in the open water vicinity of the colony but do not come in to nest sites or nest, meaning that we cannot capture or kill birds to examine body mass or organs.
Our data on body condition of arctic terns in the Canadian High Arctic suggest that male and female terns maintain similar body masses and increase lipid reserves through breeding, but females typically carry larger endogenous stores. This highlights the need for resources directly around the colony for females to be in good enough condition to breed, but we caution that this may not reflect other colonies in the Arctic region. Species that cover a wide geographic range may vary in physiological patterns due to differences in local environmental drivers such as resource availability or weather (Mallory et al. Citation2008; Alisauskas and Devink Citation2015). For example, despite the mean arctic tern body mass of approximately 111 g we found in 2007, almost all of the more than 500 breeding arctic terns we have measured over nine years at Nasaruvaalik Island were less than 110 g, whereas at least half of the terns weighed in the Bay of Fundy were more than 111 g (Hatch Citation2002). We interpret our results on body condition, as well as annual breeding effort and success (Mallory et al. Citation2017), to indicate that 2007 was likely a very good year for terns at our field site. In general, we think that terns breed in high numbers at the remote Nasaruvaalik Island because of predictably abundant, primarily invertebrate food resources in the nearby small polynya (Maftei, Davis, and Mallory Citation2015; Mallory et al. Citation2017), which allow terns to meet their energetic needs for reproduction. However, years with reduced reproductive effort or breeding skips appear to be associated with factors other than local food supply at this site (Mallory et al. Citation2017, unpublished data). Consequently, we predict that the ongoing increases in climate variability, notably warmer, wetter weather (Boisvert and Stroeve Citation2015), which leads to more snow and rain during breeding at this location, will have a more pronounced, deleterious effect on this arctic tern colony than local food-mediated influences on body condition. Importantly, we also caution that changes in the phenology and distribution of marine food supplies (e.g., Thackeray et al. Citation2010) needed during migration may be negatively influenced by a variety of factors and thereby could negatively affect this colony, irrespective of local conditions.
Acknowledgments
We thank Jason Akearok, Kelly Boadway, Josh Boadway, and Alain Fontaine for help with collections. All research activities were conducted in accordance with valid scientific and animal care permits (WL000952, NUN-SCI-06-01). Finally, thanks to three referees for very insightful and supportive comments that helped improve the article.
Disclosure statement
No potential conflict of interest was reported by the authors.
Additional information
Funding
References
- Alisauskas, R. T., and C. D. Ankney. 1985. Nutrient reserves and the energetics of reproduction in American coots. The Auk 102 (1):133–44.
- Alisauskas, R. T., and J.-M. Devink. 2015. Breeding costs, nutrient reserves, and cross-seasonal effects: Dealing with deficits in sea ducks. In Ecology and conservation of North American Sea Ducks, ed. J. P. L. Savard, D. Derksen, D. Esler, and J. Eadie, Studies in avian biology. 125–68. New York: CRC Press.
- Ankney, C. D. 1977. Feeding and digestive organ size in breeding lesser snow geese. The Auk 94 (2):275–82. doi:https://doi.org/10.1093/auk/94.2.275.
- Avery, M., D. Suddaby, P. M. Ellis, and M. W. Innes. 1992. Exceptionally low body-weights of Arctic terns Sterna paradisaea on Shetland. Ibis 134 (1):87–88. doi:https://doi.org/10.1111/j.1474-919X.1992.tb07236.x.
- BirdLife International. 2018. IUCN red list of threatened species: Arctic tern. IUCN Red List of Threatened Species. https://dx.doi.10.2305/IUCN.UK.2018-2.RLTS.T22694629A132065195.en.
- Black, A., and A. W. Diamond. 2004. Feeding areas of Arctic terns (Sterna Paradisaea) and common terns (Sterna hirundo) breeding on the Machias Seal Island, New Brunswick. In Proceedings of the 6th Bay of Fundy Workshop, Cornwallis, Nova Scotia, September 29th - October 2nd, 2004. Cornwallis, Nova Scotia: Environment Canada.
- Boisvert, L. N., and J. C. Stroeve. 2015. The Arctic is becoming warmer and wetter as revealed by the atmospheric infrared sounder. Geophysical Research Letters 42 (11):4439–46. doi:https://doi.org/10.1002/2015GL063775.
- Bryndum‐Buchholz, A., D. P. Tittensor, J. L. Blanchard, W. L. Cheung, M. Coll, E. D. Galbraith, S. Jennings, O. Maury, and H. K. Lotze. 2019. Twenty-first-century climate change impacts on marine animal biomass and ecosystem structure across ocean basins. Global Change Biology 25 (2):459–72. doi:https://doi.org/10.1111/gcb.14512.
- Bustnes, J. O., B. Moe, D. Herzke, S. A. Hanssen, T. Nordstad, K. Sagerup, G. W. Gabrielsen, and K. Borgå. 2010. Strongly increasing blood concentrations of lipid-soluble organochlorines in High Arctic common eiders during incubation fast. Chemosphere 79 (3):320–25. doi:https://doi.org/10.1016/j.chemosphere.2010.01.026.
- Cairns, D. K. 1988. Seabirds as indicators of marine food supplies. Biological Oceanography 5 (4):261–71. doi:https://doi.org/10.1080/01965581.1987.10749517.
- Chastel, O., H. Weimerskirch, and P. Jouventin. 1995. Body condition and seabird reproductive performance: A study of three petrel species. Ecology 76 (7):2240–46. doi:https://doi.org/10.2307/1941698.
- Clayden, M. G., L. M. Arsenault, K. A. Kidd, N. J. O’Driscoll, and M. L. Mallory. 2015. Mercury bioaccumulation and biomagnification in a small Arctic polynya ecosystem. Science of the Total Environment 509–510:206–15. doi:https://doi.org/10.1016/j.scitotenv.2014.07.087.
- Devlin, C. M., A. W. Diamond, and G. W. Saunders. 2004. Sexing Arctic terns in the field and laboratory. Waterbirds 27 (3):314–20.
- Dobush, G. R., C. D. Ankney, and D. G. Krementz. 1985. The effect of apparatus, extraction time, and solvent type on lipid extractions of snow geese. Canadian Journal of Zoology 63 (8):1917–20. doi:https://doi.org/10.1139/z85-285.
- Egevang, C., I. J. Stenhouse, R. A. Phillips, A. Petersen, J. W. Fox, and J. R. D. Silk. 2010. Tracking of Arctic terns Sterna paradisaea reveals longest animal migration. Proceedings of the National Academy of Sciences 107 (5):2078–81. doi:https://doi.org/10.1073/pnas.0909493107.
- Frank, D., and P. H. Becker. 1992. Body-mass and nest reliefs in common terns Sterna hirundo exposed to different feeding conditions. Ardea 80:56–69.
- Furness, R. W., and C. J. Camphuysen. 1997. Seabirds as monitors of the marine environment. ICES Journal of Marine Science 54 (4):726–37. doi:https://doi.org/10.1006/jmsc.1997.0243.
- Furness, R. W., and M. L. Tasker. 2000. Seabird-fishery interactions: Quantifying the sensitivity of seabirds to reductions in sandeel abundance, and identification of key areas for sensitive seabirds in the North Sea. Marine Ecology Progress Series 202:253–64. doi:https://doi.org/10.3354/meps202253.
- Gabrielsen, G. W., F. Mehlum, and H. E. Karlsen. 1988. Thermoregulation in four species of Arctic seabirds. Journal of Comparative Physiology B 157 (6):703–08. doi:https://doi.org/10.1007/BF00691000.
- Gaston, A. J., H. G. Gilchrist, and M. L. Mallory. 2005. Variation in ice conditions has strong effects on the breeding of marine birds at Prince Leopold Island, Nunavut. Ecography 28 (3):331–44. doi:https://doi.org/10.1111/j.0906-7590.2005.04179.x.
- Golet, G. H., and D. B. Irons. 1999. Raising young reduces body condition and fat stores in black-legged kittiwakes. Oecologia 120 (4):530. doi:https://doi.org/10.1007/s004420050887.
- Groscolas, R., L. Schreiber, and F. Morin. 1991. The use of tritiated water to determine protein and lipid utilization in fasting birds: A validation study in incubating great-winged petrels, Pterodroma Macroptera. Physiological Zoology 64 (5):1217–33.
- Hannah, C. G., F. Dupont, and M. Dunphy. 2009. Polynyas and tidal currents in the Canadian Arctic Archipelago. Arctic 62 (1):83–95. doi:https://doi.org/10.14430/arctic115.
- Hatch, J. J. 2002. Arctic tern (Sterna paradisaea). In The birds of North America, No. 707, eds. A. Poole and F. Gill. Ithaca: Cornell Laboratory of Ornithology. doi:https://doi.org/10.2173/bna.707.
- Hobson, K. A., J. Sirois, and M. L. Gloutney. 2000. Tracing nutrient allocation to reproduction with stable isotopes: A preliminary investigation using colonial waterbirds of Great Slave Lake. The Auk 117 (3). Oxford University Press:760–74.
- Jacobs, S. R., D. B. Edwards, J. Ringrose, K. H. Elliott, J.-M. Weber, and A. J. Gaston. 2011. Changes in body composition during breeding: Reproductive strategies of three species of seabirds under poor environmental conditions. Comparative Biochemistry and Physiology Part B 158 (1):77–82. doi:https://doi.org/10.1016/j.cbpb.2010.09.011.
- Jodice, P. G. R., D. D. Roby, R. M. Suryan, D. B. Irons, A. M. Kaufman, K. R. Turco, and G. H. Visser. 2003. Variation in energy expenditure among black-legged kittiwakes: Effects of activity-specific metabolic rates and activity budgets. Physiological and Biochemical Zoology 76 (3):375–88.
- Johnston, D. W. 1964. Ecologic aspects of lipid deposition in some postbreeding Arctic birds. Ecology 45 (4):848–52. doi:https://doi.org/10.2307/1934931.
- Kerr, D. C., C. D. Ankney, and J. S. Millar. 1982. The effect of drying temperature on extraction of petroleum ether soluble fats of small birds and mammals. Canadian Journal of Zoology 60 (3):470–72. doi:https://doi.org/10.1139/z82-064.
- Kitaysky, A. S., J. C. Wingfield, and J. F. Piatt. 1999. Dynamics of food availability, body condition and physiological stress response in breeding black-legged kittiwakes. Functional Ecology 13 (5):577–84. doi:https://doi.org/10.1046/j.1365-2435.1999.00352.x.
- Langham, N. P. E. 1968. The comparative biology of terns, Sterna spp. Unpublished thesis, University of Durham.
- Laxon, S., N. Peacock, and D. Smith. 2003. High interannual variability of sea ice thickness in the Arctic region. Nature 425 (6961):947–50. doi:https://doi.org/10.1038/nature02050.
- Maftei, M., S. E. Davis, and M. L. Mallory. 2015. Assessing regional populations of ground-nesting marine birds in the Canadian High Arctic. Polar Research 34 (1):25055. doi:https://doi.org/10.3402/polar.v34.25055.
- Mallory, M. L., C. M. Anderson, B. M. Braune, I. Pratte, and J. F. Provencher. 2019. Arctic cleansing diet: Sex-specific variation in the rapid elimination of contaminants by the world’s champion migrant, the Arctic tern. Science of the Total Environment 689 (11):716–24. doi:https://doi.org/10.1016/j.scitotenv.2019.06.505.
- Mallory, M. L., K. A. Boadway, S. E. Davis, M. Maftei, and A. W. Diamond. 2017. Breeding biology of Arctic terns (Sterna paradisaea) in the Canadian High Arctic. Polar Biology 40 (8):1515–25. doi:https://doi.org/10.1007/s00300-016-2072-1.
- Mallory, M. L., S. E. Davis, M. Maftei, D. T. Fife, and G. J. Robertson. 2018. Adult survival of Arctic terns in the Canadian High Arctic. Polar Research 37 (1):1537710. doi:https://doi.org/10.1080/17518369.2018.1537710.
- Mallory, M. L., and M. R. Forbes. 2013. Behavioural and energetic constraints of reproduction: Distinguishing breeding from non-breeding northern fulmars at their colony. Écoscience 20 (1):48–54. doi:https://doi.org/10.2980/20-1-3552.
- Mallory, M. L., A. J. Gaston, M. R. Forbes, H. G. Gilchrist, B. Cheney, S. Lewis, and P. M. Thompson. 2008. Flexible incubation rhythm in northern fulmars: A comparison between oceanographic zones. Marine Biology 154 (6):1031–40. doi:https://doi.org/10.1007/s00227-008-0994-z.
- Mallory, M. L., H. G. Gilchrist, M. Janssen, H. L. Major, F. Merkel, J. F. Provencher, and H. Strøm. 2018. Financial costs of conducting science in the Arctic: Examples from seabird research. Arctic Science 4 (4):624–33. doi:https://doi.org/10.1139/as-2017-0019.
- Mallory, M. L., S. A. Hatch, and D. N. Nettleship. 2012. Northern fulmar (Fulmarus glacialis). In The birds of North America Online, No. 361, ed. A. Poole. Ithaca: Cornell Lab of Ornithologyhttps://birdsoftheworld.org/bow/species/norful/cur/introduction.
- Mallory, M. L., N. J. Karnovsky, A. J. Gaston, K. A. Hobson, J. F. Provencher, M. R. Forbes, G. L. Hunt Jr, T. Byers, and T. A. Dick. 2010. Temporal and spatial patterns in the diet of northern fulmars Fulmarus glacialis in the Canadian High Arctic. Aquatic Biology 10 (2):181–91. doi:https://doi.org/10.3354/ab00277.
- Mallory, M. L., S. A. Robinson, C. E. Hebert, and M. R. Forbes. 2010. Seabirds as indicators of aquatic ecosystem conditions: A case for gathering multiple proxies of seabird health. Marine Pollution Bulletin 60 (1):7–12. doi:https://doi.org/10.1016/j.marpolbul.2009.08.024.
- Mathot, K. J., E. M. A. Kok, J. B. Burant, A. Dekinga, P. Manche, D. Saintonge, and T. Piersma. 2019. Evolutionary design of a flexible, seasonally migratory, avian phenotype: Why trade gizzard mass against pectoral muscle mass? Proceedings of the Royal Society B: Biological Sciences 286 (1903):20190518. doi:https://doi.org/10.1098/rspb.2019.0518.
- Moe, B., L. Stempniewicz, D. Jakubas, F. Angelier, O. Chastel, F. Dinessen, G. W. Gabrielsen, F. Hanssen, N. J. Karnovsky, B. Rønning, et al. 2009. Climate change and phenological responses of two seabird species breeding in the High-Arctic. Marine Ecology Progress Series 393 (October):235–46. doi:https://doi.org/10.3354/meps08222.
- Monaghan, P., J. D. Uttley, M. D. Burns, C. Thaine, and J. Blackwood. 1989. The relationship between food supply, reproductive effort and breeding success in Arctic terns Sterna paradisaea. Journal of Animal Ecology 58 (1):261–74. doi:https://doi.org/10.2307/4999.
- Moreno, J. 1989. Strategies of mass change in breeding birds. Biological Journal of the Linnean Society 37 (4):297–310. doi:https://doi.org/10.1111/j.1095-8312.1989.tb01907.x.
- Nagy, K. A. 1983. The doubly labeled water (3HH180) method: a guide to its use. Publication 12-1417. University of California, Los Angeles, USA.
- Nisbet, I. C. T., J. M. Arnold, S. A. Oswald, P. Pyle, and M. A. Patten. 2017. Common Tern (Sterna hirundo). In The Birds of North America Online, ed. P. G. Rodewald. Ithaca: Cornell Lab of Ornithology. https://birdsoftheworld.org/bow/species/comter/cur/introduction
- Parsons, M., I. Mitchell, A. Butler, N. Ratcliffe, M. Frederiksen, S. Foster, and J. B. Reid. 2008. Seabirds as indicators of the marine environment. ICES Journal of Marine Science 65 (8):1520–26. doi:https://doi.org/10.1093/icesjms/fsn155.
- Percy, J. A., and F. J. Fife. 1981. The biochemical composition and energy content of Arctic marine macrozooplankton. Arctic 34 (4):307–13.
- Perrow, M., E. Skeate, and J. Gilroy. 2011. Visual tracking from a rigid-hulled inflatable boat to determine foraging movements of breeding terns. Journal of Field Ornithology 82 (1):68–72. doi:https://doi.org/10.1111/j.1557-9263.2010.00309.x.
- Pratte, I., K. A. Boadway, A. W. Diamond, and M. L. Mallory. 2018. Changes in isotopic niches across stages of the annual cycle in the Arctic tern (Sterna paradisaea). Arctic 71 (3). doi: https://doi.org/10.14430/arctic4731.
- Provencher, J. F., J. Ammendolia, C. M. Rochman, and M. L. Mallory. 2019. Assessing plastic debris in aquatic food webs: What we know and don’t know about uptake and trophic transfer. Environmental Reviews 27 (3):304–17. doi:https://doi.org/10.1139/er-2018-0079.
- Provencher, J. F., B. M. Braune, H. G. Gilchrist, M. R. Forbes, and M. L. Mallory. 2014. Trace element concentrations and gastrointestinal parasites of Arctic terns breeding in the Canadian High Arctic. Science of the Total Environment 476–477 (4):308–16. doi:https://doi.org/10.1016/j.scitotenv.2014.01.016.
- Raveling, D. G. 1979. The annual cycle of body composition of Canada geese with special reference to control of reproduction. The Auk 96 (2):234–52.
- Robinson, R. A., H. Q. P. Crick, J. A. Learmonth, I. M. D. Maclean, C. D. Thomas, F. Bairlein, M. C. Forchhammer, C. M. Francis, J. A. Gill, B. J. Godley, et al. 2009. Travelling through a warming world: Climate change and migratory species. Endangered Species Research 7 (2):87–99. doi:https://doi.org/10.3354/esr00095.
- RStudio Team. 2019. RStudio: Integrated development environment for R. Boston, MA: RStudio, Inc. http://www.rstudio.com/.
- Scott, C. L., S. Kwasniewski, S. Falk-Petersen, and J. R. Sargent. 2000. Lipids and life strategies of Calanus finmarchicus, Calanus glacialis and Calanus hyperboreus in late autumn, Kongsfjorden, Svalbard. Polar Biology 23 (7):510–16. doi:https://doi.org/10.1007/s003000000114.
- Stirling, I. 1997. The importance of polynyas, ice edges, and leads to marine mammals and birds. Journal of Marine Systems 10 (1):9–21. doi:https://doi.org/10.1016/S0924-7963(96)00054-1.
- Suddaby, D., and N. Ratcliffe. 1997. The effects of fluctuating food availability on breeding Arctic terns (Sterna paradisaea). The Auk 114 (3):524–30.
- Thackeray, S. J., T. H. Sparks, M. Frederiksen, S. Burthe, P. J. Bacon, J. R. Bell, M. S. Botham, T. M. Brereton, P. W. Bright, L. Carvalho, et al. 2010. Trophic level asynchrony in rates of phenological change for marine, freshwater and terrestrial environments. Global Change Biology 16 (12):3304–13. doi:https://doi.org/10.1111/j.1365-2486.2010.02165.x.
- Uttley, J., P. Tatner, and P. Monaghan. 1994. Measuring the daily energy expenditure of free-living Arctic terns (Sterna paradisaea). The Auk 111 (2):453–59. doi:https://doi.org/10.2307/4088609.
- Van Gils, J. A., P. F. Battley, T. Piersma, and R. Drent. 2005. Reinterpretation of gizzard sizes of red knots world-wide emphasises overriding importance of prey quality at migratory stopover sites. Proceedings of the Royal Society B: Biological Sciences 272 (1581):2609–18. doi:https://doi.org/10.1098/rspb.2005.3245.
- Watson, R. A., and A. Tidd. 2018. Mapping nearly a century and a half of global marine fishing: 1869–2015. Marine Policy 93 (7):171–77. doi:https://doi.org/10.1016/j.marpol.2018.04.023.
- Wendeln, H., and P. H. Becker. 1996. Body mass change in breeding common terns Sterna hirundo. Bird Study 43 (1):85–95. doi:https://doi.org/10.1080/00063659609460998.