ABSTRACT
The Ziegler Reservoir fossil site near Snowmass Village, Colorado, provides a rare opportunity to examine environmental conditions in the Rocky Mountains during marine isotope stage (MIS) 4 (71–57 ka). Although recognized as a global-scale cold event, MIS 4 is typically absent from Rocky Mountain glacial chronologies because the geologic evidence was covered or destroyed during the subsequent, and more extensive, MIS 2 (Pinedale; 29–14 ka) glaciation. Ziegler Reservoir lies beyond the Pinedale glacial extent, which allowed for the preservation of a long-lived sequence of eolian sediments deposited in a lacustrine environment that spans from late MIS 6 (ca. 140 ka) through early MIS 3 (ca. 55 ka). Sediments dating to MIS 4 exhibit a significant increase in clay-sized particles, suggesting that the source areas, most likely nearby glacio-fluvial deposits, were enriched with fine-grained material at that time. We hypothesize that the elevated clay content was the result of rock flour production by nearby valley glaciers that were active in the Rocky Mountains during MIS 4. The results of our study illustrate how recognizing indirect evidence of glacial activity can result in a more complete record of past climate conditions than what could be achieved by the study of moraines alone.
Introduction
Efforts to reconstruct late Pleistocene glacial chronologies in the Rocky Mountains have been underway for more than a century. Blackwelder (Citation1915) investigated the glacial history of the Wind River Range in the northern Rockies () and described evidence of two large-scale, late Pleistocene glacial events that he called the Bull Lake and Pinedale stages. Subsequent researchers have utilized this framework while investigating glacial deposits at sites throughout the Rocky Mountains and have shown that the two events were separated by a considerable amount of time based on differences in the degree of erosion, soil development, and cobble weathering characteristics of Bull Lake and Pinedale moraines, till, and other glacial landforms (e.g., Holmes and Moss Citation1955; Pierce, Obradovich, and Friendman Citation1976; Colman and Pierce Citation1981; Richmond Citation1986; Hall and Shroba Citation1995). More recently, cosmogenic dating and, to a lesser degree, radiocarbon techniques have been used to determine the ages of the two glacial stages (Phillips et al. Citation1997; Dahms Citation2004; Benson et al. Citation2005; Licciardi and Pierce Citation2008, Citation2018; Dahms et al. Citation2018; Laabs et al. Citation2020). These studies have shown that the Bull Lake deposits date to marine isotope stage (MIS) 6 (ca. 191–130 ka; ka = kilo annum, thousand years; Lisiecki and Raymo Citation2005), which is temporally coincident with the Illinoian continental glaciation (), whereas Pinedale deposits are coincident with MIS 2 (29–14 ka) and the late stages of the Wisconsinan continental glaciation (Pierce, Obradovich, and Friendman Citation1976; Pierce Citation2003).
Figure 1. (a) Map of the central Rocky Mountains showing the location of our study area in west-central Colorado; inset map of North America. (b) Location of the Ziegler Reservoir fossil site just west of the town of Snowmass Village, Colorado, on the divide between Snowmass Creek Valley to the west and the Brush Creek drainage to the east. The extent of the Snowmass Creek Valley glacier during Bull Lake (MIS 6) is shown in gray (after Bryant Citation1979). Colored polygons represent areas where glacial deposits were sampled to test for provenance; Snowmass Creek Valley (blue), Roaring Fork Valley (light brown), and the Roaring Fork–Carbondale area (green). Locations of loess deposits on Mt. Sopris (Birkeland Citation1973) and the Aspen Highlands Ski property (McCalpin and Irvine Citation1995) are also shown.
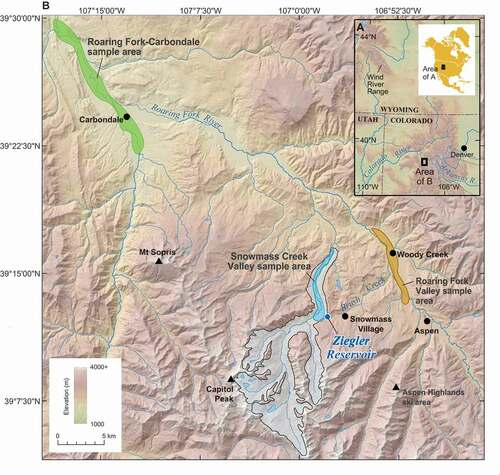
Figure 2. Oxygen isotope ratios (δ18O) of marine foraminifera over the past 200 ka (Imbrie et al. Citation1993). Age constraints for MIS 1 to 6 are based on Lisiecki and Raymo (Citation2005), with the cold stages highlighted in blue and the cooler substages of MIS 5 highlighted in light blue. The North American continental glacial episodes and Rocky Mountain glacial stages are shown below the MIS stage numbers in their approximate temporal positions (Pierce Citation2003). Finally, the age span of the Ziegler Reservoir sediments is depicted by the bracket.
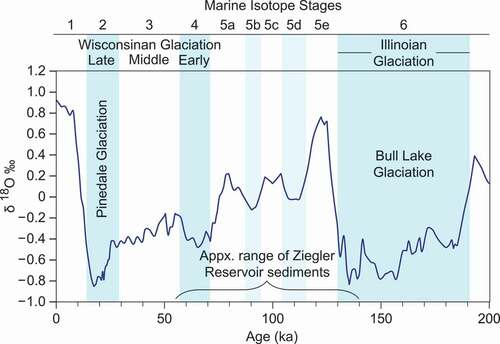
Blackwelder (Citation1915) found that Pinedale glaciers were slightly smaller than Bull Lake glaciers based on the relative positions of their moraines on the landscape. Pierce (Citation2003) later estimated that the maximum extents of Pinedale glaciers were approximately 90 percent as large as those of the Bull Lake episode, as their lateral and terminal moraines are nested just inside the older Bull Lake moraines. Because the maximum extents of the glaciers were similar, there is little space for geologic evidence of glacial activity between MIS 6 and MIS 2, specifically during MIS 4, so the typical Rocky Mountain glacial chronology consists only of the Bull Lake (MIS 6) and Pinedale (MIS 2) stages (Mears Citation1974; Chadwick, Hall, and Phillips Citation1997; Pierce Citation2003; Laabs et al. Citation2020).
Marine records show that MIS 4 was a global-scale cooling event that occurred between ca. 71 and 57 ka (Martinson et al. Citation1987; Lisiecki and Raymo Citation2005). Evidence of glacial activity during MIS 4 has been documented in Antarctica (Menking et al. Citation2019), New Zealand (McCarthy et al. Citation2008), Australia (De Deckker et al. Citation2019), Greenland (Seidenkrantz et al. Citation2019), Europe (Ivy-Ochs et al. Citation2008), northeast Asia (Glushkova Citation2011), South America (Smith and Rodbell Citation2010; Peltier et al. Citation2021), Canada (Ward, Bond, and Gosse Citation2007), and Alaska (Kaufman and Thompson Citation1998; Briner et al. Citation2005; Briner and Kaufman Citation2008; Kaufman et al. Citation2011). In the Western United States, Thackray (Citation2001) reported evidence of extensive MIS 4 glaciation on the western Olympic Peninsula in Washington, and Troost (Citation2016) found evidence that suggests that MIS 4 ice coverage in the Puget Sound lowlands in Washington may have been considerably larger than previously recognized. Similarly, on the eastern side of the Sierra Nevada in California, Bischoff and Cummins (Citation2001) identified a glacial episode that began near the close of MIS 5a that likely represents MIS 4 glaciation. Within the Great Basin, Oviatt and McCoy (Citation1992) documented high lake levels during MIS 4, which further demonstrates the cooler/wetter nature of this period.
Despite the lack of obvious MIS 4 moraines in the Rocky Mountains, researchers have identified some evidence of glacial activity during this time period. For example, Colman and Pierce (Citation1992) used weathering rind data to highlight potential MIS 4 glacial features (described as early Wisconsinan) in areas near McCall, Idaho, and the West Yellowstone region, as well as other ranges in the Western United States. In the Snake River Plain of Idaho, Forman et al. (Citation1993) linked loess deposits to MIS 4 glacial activity through luminescence dating. Similarly, Pierce et al. (Citation2011) described loess–paleosol sequences that likely date to MIS 4 near Jackson Hole, Wyoming. In the Wind River Range of Wyoming, Hall and Shroba (Citation1995) used soil properties to suggest that some moraines originally attributed to the Bull Lake glaciation may actually be MIS 4 in age. Also in the Wind River Range, Dahms (Citation2004) reported evidence of faint moraines nested between Bull Lake and Pinedale features that may have survived MIS 2. Sharp et al. (Citation2003) dated pedogenic carbonates on glacial outwash deposits in the Wind River Basin to ca. 55 ka, which implies that glaciers were producing glacio-fluvial deposits near the end of MIS 4. Finally, Douglass and Mickelson (Citation2007) used soil properties to infer a possible MIS 4 glacial event in the Uinta Mountains of Utah. These studies show that limited or indirect evidence of MIS 4 glacial activity can be used to complement glacial records based on the traditional study of moraines and establish a more complete view of past climate conditions in the Rocky Mountains.
Ziegler Reservoir fossil site
The Ziegler Reservoir fossil site is located in the Elk Mountains near the town of Snowmass Village in west-central Colorado (). Surrounded by other high-altitude ranges, the Elk Mountains have numerous peaks that rise above 3,700 m in elevation and hosted extensive valley glaciers during the late Pleistocene (Bryant and Martin Citation1988). The bedrock geology of this area is composed predominantly of late Paleozoic and Mesozoic sedimentary rocks, including limestones, shales, and sandstones, as well as some Neogene granodiorite intrusions (Bryant Citation1972).
Ziegler Reservoir is located in a closed basin at an elevation of 2,705 m and its southeastern portion was excavated in 2010–2011 as part of a reservoir expansion project, revealing an extensive array of Pleistocene fossils (Johnson, Miller, and Pigati Citation2014; Miller et al. Citation2014; Sertich et al. Citation2014). The basin that encompasses Ziegler Reservoir was formed by a sequence of glacial moraines. The easternmost moraine was deposited at the distal margin of a small glacial lobe that overtopped a ridge between Snowmass Creek Valley to the west and the Brush Creek drainage to the east (). Subsequently, a second moraine was deposited on the western margin of the basin. Bryant (Citation1972) initially assigned the eastern moraine to the Bull Lake glaciation (MIS 6) and the western moraine to the Pinedale (MIS 2), but recent work indicates that both moraines were actually deposited during MIS 6 (Pigati et al. Citation2014). As the Bull Lake stage came to a close at the end of MIS 6, fine-grained sediments began accumulating in the basin, which continued through the early part of MIS 3 (Mahan et al. Citation2014). Sediments deposited after this time were either disturbed or removed during construction.
Figure 3. View of the Ziegler Reservoir fossil site looking to the southwest. Note the fortuitous location of the site where the Bull Lake glacier overtopped a ridge but did not descend down Brush Creek drainage to the east. Also note the mass wasting event on the Bull Lake (MIS 6) lateral moraine on the right side of the image. The location of Capitol Peak is shown in for spatial reference. Image generated using Google Earth.
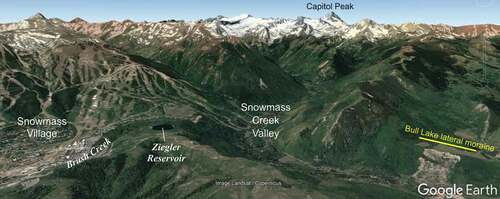
In this study, we utilized mineralogical, elemental, and particle size data, as well as other features of the fine-grained sediments previously reported by Pigati et al. (Citation2014) to better understand the sources and transport pathways of these sediments. We demonstrate that fine-grained sediments of Ziegler Reservoir are likely eolian in nature and have mineralogical and geochemical signatures similar to those of nearby glacio-fluvial deposits. In particular, we focus on deposits that date to MIS 4, which provide a rare opportunity to evaluate environmental conditions high in the Rocky Mountains between the Bull Lake and Pinedale events. These sediments exhibit an increase in clay-sized content, which we interpret as evidence of glacial activity and increased production of rock flour by surrounding valley glaciers during MIS 4.
Methods
Sample collection
Representative samples of the Ziegler Reservoir sediments were collected in 2010 and 2011 during the Snowmastodon Project (Johnson, Miller, and Pigati Citation2014). Individual stratigraphic units were measured and described both in the field and in the laboratory to create a composite stratigraphic record for the site (Pigati et al. Citation2014). To aid in unit description and characterization during that project, samples were collected from units exposed in excavation outcrops as well as sediment cores that penetrated the floor of the construction site (Supplemental Table 1a). Additional samples were collected from the impounding moraines at depths that were below the B horizon at the base of two hand-dug soil pits (each were at least 100 cm deep) to characterize the fine-grained (<2 mm) sediment of the moraines. Finally, a dust collection device was installed approximately 100 m southwest of the reservoir in 2011 to determine the character of modern dust at the site. The device was constructed using techniques described by Reheis and Kihl (Citation1995) and was sampled in October 2012 and April 2013 (Supplemental Table 1b).
Samples of potential source material for the sediments at the Ziegler Reservoir fossil site were collected from glacial deposits, mostly lateral moraines, at numerous sites in Snowmass Creek Valley, immediately west of the fossil site. Additional provenance samples were collected from glacio-fluvial outwash terraces in two parts of the Roaring Fork Valley, including a stretch between Woody Creek and Aspen located approximately 5 km to the east and an area near Carbondale located approximately 20 km to the northwest (). At each location in the Roaring Fork Valley, we targeted glacial outwash deposits that were assigned to the Bull Lake glaciation by Piety (Citation1981) but also collected sediments from Pinedale outwash terraces when the older deposits were not available (Supplemental Table 1b). All provenance samples were taken from quarries or road cuts and, like the moraine samples, were positioned well below the deposit surfaces to avoid any potential effects of pedogenesis.
Age–depth modeling
Mahan et al. (Citation2014) used the Bayesian accumulation (Bacon) software program (Blaauw and Christen Citation2011) to construct an age–depth relationship for the sediments at Ziegler Reservoir. The model uses Bayesian statistics to reconstruct histories for deposits by dividing the sediments into numerous sections and—through millions of Markov chain Monte Carlo iterations—estimates the sediment accumulation rate (SAR) for each of these sections. These accumulation rates form the basis of the age–depth model.
Particle size analysis
Particle size analysis (PSA) was conducted at the Department of Earth and Environmental Sciences Laboratory at the University of Iowa using pipette techniques on twenty-four samples representing the composite stratigraphy. Grain sizes were defined using the U.S. Department of Agriculture’s particle size classification system (U.S. Department of Agriculture, Soil Conservation Service, Soil Survey Staff Citation1993) as sand (2 mm–50 µm), silt (50–2 µm), and clay (<2 µm); the silt fraction was further subdivided into very coarse silt (50–44 µm), coarse silt (44–31 µm), medium silt (31–16 µm), fine silt (16–8 µm), and very fine silt (8–2 µm). PSA was also performed on the two dust trap samples at the U.S. Geological Survey (USGS) Soil Laboratory in Denver, Colorado, using wet dispersion laser analysis techniques (Gray, Pasternack, and Watson Citation2010).
Mineralogical and elemental analysis
Mineralogical analysis was conducted at the USGS X-ray Diffraction (XRD) Laboratory in Denver, Colorado, on the silt size fraction of the Ziegler Reservoir sediments (n = 30), sediments from the moraine soil pits (n = 2), modern dust (n = 1; the dust samples collected in 2012 and 2013 had to be combined because of their small sizes), and the three potential provenance areas (Snowmass Creek Valley, n = 11; Roaring Fork Valley, n = 13; and the Roaring Fork–Carbondale area, n = 13). All of these samples were wet sieved in steps down to 50 µm, and clay-sized particles were removed and retained for clay mineralogy analysis. The silt fractions were then immersed in 30 percent hydrogen peroxide (H2O2) to remove organic matter before being analyzed by XRD from 2° to 60° 2Θ. Mineral identification and semiquantitative interpretations of the silt fractions were based on peak intensities (Moore and Reynolds Citation1997).
Clay fractions of sediments from the Ziegler Reservoir site (n = 23), the moraine soil pits (n = 2), and the three potential provenance areas (Snowmass Creek Valley, n = 3; Roaring Fork Valley, n = 3; and the Roaring Fork–Carbondale area, n = 4) were isolated by centrifugation using Stokes’ law of settling and then dried at 60°C. Clay mineralogy was determined for air-dried, ethylene-glycolated, and heat-treated (550°C for 1 hour) aliquots using measured XRD peak heights between 2° and 35° 2Θ (Moore and Reynolds Citation1997). Semiquantitative analysis for clay minerals was based on a technique originally developed by H. D. Glass of the Illinois State Geological Survey and later modified by Hallberg, Lucas, and Goodmen (Citation1978). Counts per second (cps) from the glycolated analysis were postprocessed using a five-point running average of the diffractogram data to reduce the electronic noise. Relative abundance was estimated using the equation T = E + I + K, where T is the total, E (expandable clays/smectite) = cps at 5.2°, 2Θ, 17 Å, I (mica/illite) = 3 × cps at 8.8° 2Θ 10 Å, and K (kaolinite plus chlorite) = 2 × cps at 12.4°, 2Θ, 7.1 Å.
Major and trace elemental analysis was conducted on silt fractions of sediments from the Ziegler Reservoir site (n = 30), moraine soil pits (n = 2), modern dust (n = 1), and the three potential provenance areas (Snowmass Creek Valley, n = 9; Roaring Fork Valley, n = 14; and the Roaring Fork–Carbondale area, n = 13) at the University of Wisconsin Nuclear Reactor Laboratory in Madison, Wisconsin, and the USGS Instrumental Neutron Activation Analysis Laboratory in Denver, Colorado (Adams and Dams Citation1975; Brune, Forkman, and Persson Citation1984). These data were used to further characterize the sediments at the fossil site and to aid in determining the provenance of the deposits.
Results
Stratigraphy and chronology
Characterization of the Ziegler Reservoir sediments during the Snowmastodon Project revealed two distinct types of deposits (Pigati et al. Citation2014). Those located near the eastern margin of the basin are composed of poorly sorted, cobble- and boulder-rich diamictites that pinch out laterally toward the basin center and were interpreted as localized debris flows or slumps emanating from the adjacent Bull Lake moraine (). These coarse units interfinger with well-sorted, fine-grained sediments that consist of sixteen distinct stratigraphic units (3–18) that are collectively 10.5 m thick and are underlain by glacial till (unit 2) and bedrock (unit 1; ). The fine-grained sediments were all deposited in a lacustrine environment, with the exception of units 15, 16, and possibly unit 12, which were deposited in a wetland setting (Pigati et al. Citation2014).
Figure 4. Generalized cross section of the Ziegler Reservoir sedimentary sequence at the end of MIS 4 (after Pigati et al. Citation2014). The Bull Lake moraine is shown with several postdepositional debris flows, or slumps, emanating into the center of the basin where they interfinger with fine-grained basin sediments. The box shows the approximate location of the composite stratigraphy included in .
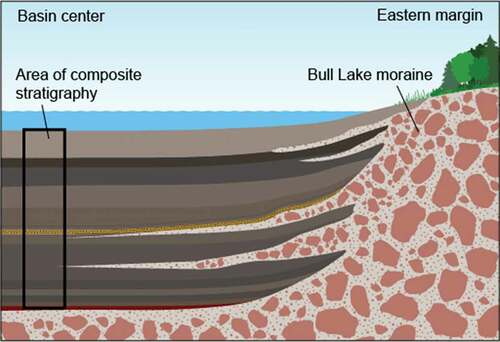
Figure 5. Composite stratigraphy, chronology, SAR, and particle size data for the fine-grained sediments of the Ziegler Reservoir fossil site. Ages for the sediments are based on the age -depth model of Mahan et al. (Citation2014). MIS are based on ages from Lisiecki and Raymo (Citation2005) and are also positioned according to the age -depth model of Mahan et al. (Citation2014). Cold MIS stages are shown in blue and the cool substages of MIS 5 are in light blue. Detailed unit descriptions can be found in Pigati et al. (Citation2014).
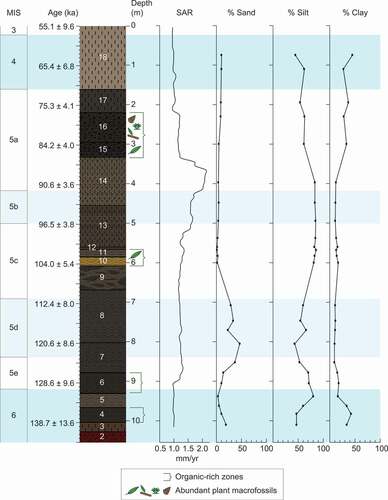
The chronology of the Ziegler Reservoir sedimentary sequence was established during the Snowmastodon Project using optically stimulated luminescence (OSL) dating and supplemented by radiocarbon, uranium series, and cosmogenic techniques (Mahan et al. Citation2014). Deposition of the fine-grained sediments occurred between ca. 140 ka (late MIS 6) and ca. 55 ka (early MIS 3). Additional age constraints within this time period were established using three significant changes in the pollen composition that are assumed to have occurred at the following climatic transitions: (1) MIS 5c–5b, 100 ka, (2) MIS 5b–5a, 89.5 ka, and (3) MIS 5a–4, 77.5 ka (Anderson et al. Citation2014; Mahan et al. Citation2014). The OSL ages and these pollen-based transitions were used as input for Bacon age–depth modeling (Blaauw and Christen Citation2011).
The age–depth model shows that the SAR at Ziegler Reservoir was fairly low and stable between late MIS 6 and early MIS 3, with the exception of MIS 5b and the early part of MIS 5a when SAR values were higher (). The modeling results also show that MIS 4 is represented by unit 18, which dates to between 71 ± 5 and 55 ± 10 ka and is the uppermost unit in the Ziegler Reservoir sedimentary sequence. We note that Anderson et al. (Citation2014) also included unit 17 in their pollen zone 4, which they interpreted as being approximately equivalent to MIS 4, despite differences in the texture and organic content of units 17 and 18. Instead, we prefer to apply the age–depth model directly when comparing the unit ages with the timing of MIS stages (Lisiecki and Raymo Citation2005). This approach results in a robust temporal correlation between unit 18 and MIS 4, with unit 17 representing a transition period between warmer conditions that prevailed during MIS 5a and the cooler conditions of MIS 4 (Anderson et al. Citation2014; Miller et al. Citation2014).
Particle size distributions
Pigati et al. (Citation2014) determined that the fine-grained sediments at Ziegler Reservoir are composed predominantly of silt, which accounts for more than 43 percent of the total in all of the samples that were analyzed (; Supplemental Tables 2a, 2b). Silt contents are highest in unit 5 and units 10 to 14, which all contain more than 80 percent silt. The amount of clay-sized particles is fairly consistent throughout most of the stratigraphic sequence, ranging from 9 to 18 percent, although we found elevated levels of 33 to 42 percent in the lowest two units (3–4) and similar values of 27 to 45 percent are present in the uppermost four units (15–18). Sand accounts for less than 15 percent of the total in all but six samples (taken from units 3, 7, and 8), which have concentrations ranging from 19 to 47 percent. The increased concentration of sand in units 7 and 8 represents the distal component of debris flows emanating from the eastern margin moraine, which were used to correlate the fine-grained deposits in the middle of the basin with the fossil-rich, coarse-grained sediments near the basin margin (Pigati et al. Citation2014).
Two samples collected from the dust trap are also mostly silt, consisting of 29 percent sand, 60 percent silt, 11 percent clay (collected in 2012) and 34 percent sand, 56 percent silt, 10 percent clay (collected in 2013; ; Supplemental Table 2a). These particle size distributions are slightly coarser than the basin sediments, which may be an accurate representation of modern dust or could possibly reflect a slight bias toward coarse material in the dust trap sampling technique (Reheis Citation2006).
Figure 6. Ternary diagram of particle size distributions for the Ziegler Reservoir sediments, loess deposits at Mt. Sopris (Birkeland Citation1973) and the Aspen Highlands ski area (McCalpin and Irvine Citation1995), loess standards from the Quaternary Materials Laboratory of the University of Iowa, and modern dust.
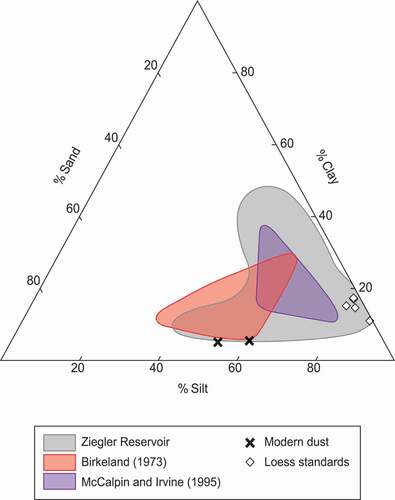
Mineralogy
Mineralogy of the silt fraction of the Ziegler Reservoir samples is dominated by quartz, which accounts for 65 to 90 percent of the total, with an average of 79 percent (Supplemental Tables 3a–3c). Feldspars, including calcium plagioclase (anorthite) and potassium feldspar, are the next most abundant minerals and account for 5 to 24 percent of the silt-sized material (). Calcite is present in a few samples where it accounts for up to 14 percent of the total, but the concentrations are highly variable. Mica content ranges from 0 to 3 percent, and chlorite is a minor constituent, reaching 1.5 percent in only one sample. Clay minerals account for less than 1.6 percent of the silt-sized grains, except for one sample from unit 14 that contains 6.5 percent clay minerals.
Figure 7. (a) Mineralogy (excluding quartz) of silt from the Ziegler Reservoir sediments (n = 30) compared to silt mineralogy from the three potential provenance areas (Snowmass Creek Valley, n = 11; Roaring Fork Valley, n = 13; and the Roaring Fork–Carbondale area, n = 13), silt from the moraine surrounding the basin, and modern dust. (b) Clay mineralogy for the Ziegler Reservoir (n = 23) sediments compared to the potential provenance areas (Snowmass Creek Valley, n = 3; Roaring Fork Valley, n = 3; and the Roaring Fork–Carbondale area, n = 4) and the impounding moraine (n = 2). The dust trap samples were not large enough for clay mineral analysis. (c), (d) Ratios of select elemental concentrations from the silt fraction of the Ziegler Reservoir sediments (n = 30), provenance areas (Snowmass Creek Valley, n = 9; Roaring Fork Valley, n = 14; and the Roaring Fork–Carbondale area, n = 13), the impounding moraine (n = 2), and modern dust (n = 1).
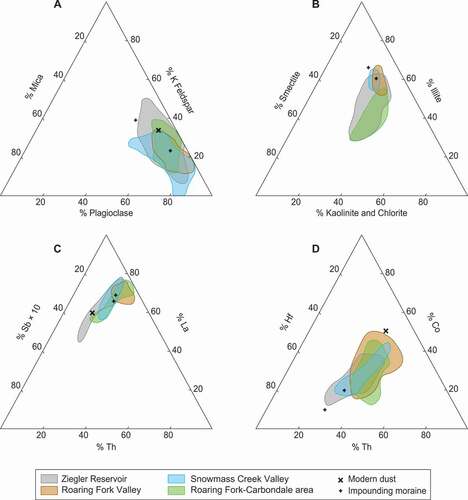
The mineralogy of silt from the moraine soil pits is similar to that of the basin sediments: 85 to 94 percent quartz, 5 to 14 percent feldspars, approximately 1 percent mica, and less than 1 percent clay minerals (; Supplemental Tables 3a–3c). Minerals of the silt size fraction of modern dust are also approximately the same: 84 percent quartz, 10 percent feldspars, 5 percent calcite, 1 percent micas, and 1 percent clay minerals (; Supplemental Tables 3a–3c).
Silt extracted from the provenance samples of glacial deposits in Snowmass Creek Valley and outwash terraces from the two areas in the Roaring Fork Valley exhibit mineralogical assemblages that are comparable to those found in the basin sediments. In these samples, quartz is the most abundant mineral (42–94 percent), feldspars are the next most abundant (4–33 percent), and mica averages approximately 0 to 2 percent. Calcite concentrations are once again highly variable, ranging from 0 to 44 percent, and clay minerals account for only a small portion of the provenance samples, generally less than 2 percent of the total (; Supplemental Tables 3a–3c).
Finally, clay mineral analysis of the basin sediments show that concentrations of smectites (12–38 percent), illites (31–58 percent), and kaolinites/chlorites (28–38 percent) are relatively uniform for units 3 to 14, above which the illites decrease and smectites increase in relative abundance (; Supplemental Table 3d). Clay mineralogy of the Snowmass Creek Valley samples revealed that smectite concentrations are 13 to 17 percent, illite is 56 to 63 percent, and kaolinite/chlorite is 24 to 28 percent. In the Roaring Fork Valley samples, smectite concentrations are 10 to 15 percent, illite is 53 to 65 percent, and kaolinite/chlorite is 25 to 34 percent. Finally, clay minerals in the Roaring Fork–Carbondale samples include 13 to 40 percent smectites, 29 to 53 percent illites, and 31 to 40 percent kaolinite/chlorites (; Supplemental Table 3d).
Elemental data
Major and trace elemental data of silt from the Ziegler Reservoir basin sediments exhibit concentrations that are indistinguishable from those collected from the three potential provenance areas, although we note that the provenance samples generally had slightly wider ranges (; Supplemental Table 4). As one example, lanthanum (La) concentrations ranged from 27 to 43 ppm with an average of 32 ppm in the Ziegler Reservoir samples, 32 to 54 ppm with an average of 42 ppm in the Snowmass Creek Valley samples, 40 to 69 ppm with an average of 50 ppm in the Roaring Fork Valley samples, and 28 to 85 ppm with an average of 48 ppm in the Roaring Fork–Carbondale samples. These averages are broadly similar to an average La value of 30 ppm determined for evolved upper continental crust (Taylor and McLennan Citation1985). Similar patterns and overlap between the Ziegler Reservoir sediments and provenance areas were observed in the elemental data for many other major and trace elements (; Supplemental Table 4).
Discussion
The bulk of the sediment that filled the Ziegler Reservoir basin consists of well-sorted silt that was deposited into a lake or wetland setting. Characterizing the physical and chemical nature of these fine-grained sediments, examining their potential source areas, and determining the possible transport mechanisms provide important insight into the environmental conditions that prevailed in the area during the time of deposition.
Evidence of eolian transport
Several lines of evidence suggest that the majority of the fine-grained sediments were introduced to the Ziegler Reservoir fossil site by eolian processes. First, the reservoir is located in a small (~14.2 ha), closed watershed that sits upon a saddle that separates Snowmass Creek Valley from the Brush Creek drainage (). The Bull Lake moraines that impound the basin have very few boulders exposed on the surface, but boulders were encountered below the surface when digging the soil pits, which implies that fine-grained material has been added to the moraine surfaces since their initial formation. Field observations reveal no signs of fluvial transport, either on the moraine surfaces or within the basin, and the lacustrine and wetland units are laminar to massive with no signs of crossbedding or fining upward sequences that would be expected in a fluvial or alluvial system (Pigati et al. Citation2014).
Second, the fine-grained sediments of the Ziegler Reservoir sedimentary sequence are quartz rich, which is typical of glacially derived loess (Taylor, McLennan, and McCulloch Citation1983; Pye Citation1995). In addition, the particle size distributions of the sediments, which consist of up to 90 percent silt, are similar to the University of Iowa loess standard, as well as loess deposits found at other high-altitude settings in the vicinity of the Ziegler Reservoir fossil site (). These sites include loess that was trapped on the surfaces of rock glaciers on the flanks of Mt. Sopris above the Roaring Fork Valley approximately 20 km to the northwest (; Birkeland Citation1973) and loess found in structural depressions that were exposed in construction trenches at the Aspen Highlands ski area roughly 12 km to the east (; McCalpin and Irvine Citation1995).
Finally, measurements conducted as part of OSL dating efforts at Ziegler Reservoir showed a low scatter in the equivalent dose data, which is characteristic of eolian sediments (Mahan et al. Citation2014). Together, the OSL properties, lack of evidence for fluvial processes, physical characteristics of the silt grains, and similar particle size distributions of the basin sediments, loess standards, and loess deposits in the local area support the hypothesis that the silt-rich material found at Ziegler Reservoir was introduced to the site primarily through eolian transport.
Evidence of provenance
If the fine-grained sediments found at the fossil site were largely the result of eolian transport, where did they originate? Glaciated alpine environments, and the outwash plains that extend beyond them, are often sources for locally derived eolian sediments (Pye Citation1987, Citation1995; Bullard Citation2013). Late Pleistocene glaciers in the mountains surrounding the Ziegler Reservoir fossil site produced extensive outwash plains in the Roaring Fork Valley and left behind glacial deposits in many other valleys (Piety Citation1981). These deposits contain abundant fine-grained materials, which are the result of glacial erosion and grinding of the sedimentary rocks and other types of bedrock located up valley. We compared the mineralogical and elemental data from these deposits with the Ziegler Reservoir sediments to determine whether one or more of these valleys could be identified as the dominant source area. The mineral composition of silt from the Ziegler Reservoir sediments is similar to that of silt from glacio-fluvial deposits at all three potential source areas, and the mineralogy of all three areas is similar to each other (). The mineralogical and elemental data do not exhibit unique signatures that allow for differentiation of one potential source area over another, but collectively the data suggest that these local deposits have similar signatures and are likely sources for the Ziegler Reservoir sediments. Clay minerals and major and trace elemental data in the basin sediments are also similar to the potential provenance areas (), although we note that the Ziegler Reservoir silts are slightly more quartz rich and exhibit a small reduction in the range of variation in elemental concentrations compared to the provenance areas, which is commonly observed in glacially derived loess (Taylor, McLennan, and McCulloch Citation1983; Pye Citation1995).
Evidence of glacial activity during MIS 4
Unit 18 of the Ziegler Reservoir sedimentary sequence is noticeably different than the underlying stratigraphic units and was referred to informally as the “clay cap.” This 1.6-m-thick unit is much lighter in color than the other units, with dry Munsell colors ranging from light gray (10 YR 7/1) to yellowish brown (10 YR 5/6). It is also massive and does not exhibit bedding features and, unlike most of the other units, it does not contain well-preserved plant remains. In fact, the near lack of organic material in unit 18 holds true even at the microscopic level, as only a few grains of pollen were identified (Anderson et al. Citation2014). Field recognition of a significant amount of clay-sized material in this unit was confirmed by the PSA data, which showed that clay accounted for up to 45 percent of the total, nearly four times higher than what was found in most of the sedimentary sequence (). Because unit 18 is so different than the other units present at the site, and because it corresponds temporally to MIS 4, a time when glacial records are exceptionally rare in the Rocky Mountains, we decided to further investigate the environmental conditions that prevailed during its deposition.
Clay-rich sediments (i.e., those containing more than 20 percent clay) first appear at the base of the Ziegler Reservoir stratigraphic sequence in units 3 and 4, which are positioned directly above the Bull Lake till (). We interpret the elevated clay content in these units to reflect the input of glacially derived rock flour that became available when the Bull Lake glacier began to recede at the end of MIS 6 and the basin began to fill with sediment. Clay content is low in units 5 through 14, which are all dominated by silt-sized particles, and then once again clay becomes prominent in units 15 to 18 (). Units 15 and 16 both consist predominantly of well-preserved peat, contain little mineral material, and are extremely porous, whereas overlying units 17 and 18 are clay rich and dense. Therefore, we interpret the relatively high percentages of clay in units 15 and 16 to reflect translocation from the clay-rich units above, so they were not considered further in our analysis.
The transition from the peat of unit 16 to the clayey silt of unit 17 is abrupt, and coarse organic material is absent above this contact. Unit 17 exhibits weak, blocky ped structures and is very dark grayish brown (10 YR 3/2) with a clay content of 37 percent. The base of this unit marks a dramatic change in pollen, characterized by an increase in Pinus and nonarboreal pollen, especially Artemisia, and Cyperaceae (Anderson et al. Citation2014). Fire history from microscopic charcoal recovered from the basin sediments shows a decrease in forest fire activity at this time (Anderson et al. Citation2020), and both plant macrofossils and invertebrates are rare in unit 17 (Elias Citation2014; Haskett and Porinchu Citation2014; Strickland et al. Citation2014). Trends in these environmental indicators continue into unit 18, which exhibits a lack of plant macrofossils and invertebrates, is lighter in color, and also contains abundant clay-sized particles.
The increase in the clay content of units 17 and 18 could be explained by several possible scenarios including changes in the source area(s), transport pathways, and/or depositional environment, as well as changes within the source sediments themselves. To determine whether a change in the source area(s) was responsible for a shift from silt-rich to clay-rich sediments at the beginning of MIS 4, for example, through the new addition of sediment from a far traveled source, we divided the Ziegler Reservoir samples into groups (sand-rich, silt-rich, and clay-rich) based on PSA data for the unit from which they originated and compared the resulting mineralogical and elemental data. The mineralogy and elemental abundances of these subgroups show no significant differences (), which suggests that the source area(s) of the Ziegler Reservoir sediments did not change between late MIS 6 and early MIS 3.
Figure 8. Results of (a) particle size analysis, (b) mineralogical determinations, and (c) select trace elements for subgroups of the Ziegler Reservoir sediments. The subgroups were based on the dominant grain size of the units from which the samples were taken and include sand-rich, silt-rich, and clay-rich sediments. Mineralogical and elemental data are based on results from the silt-sized particles from each subgroup. The lack of differentiation between these groups suggests that the source areas likely did not change between late MIS 6 and early MIS 3.
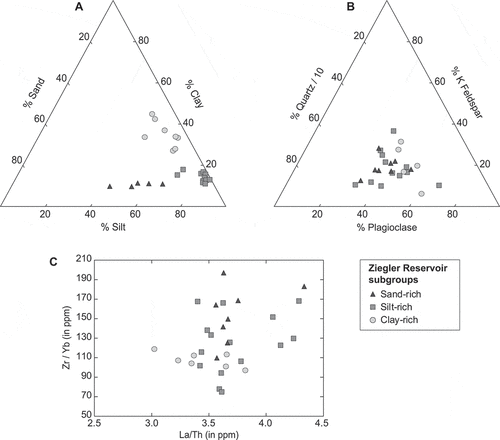
A shift in the dominant transport mechanism for units 17 and 18 is also an unlikely explanation for the increase in clay because, although the clay content is high in these units, silt remains a significant component and the sediments continue to exhibit loess-like textures that are characteristic of eolian deposits. In addition, there is a lack of crossbedding and fining upward sequences in these units and an absence of erosion in the surrounding terrain, which would be expected if the transport pathway changed to a dominantly non-eolian (e.g., alluvial and/or fluvial) system during MIS 4.
Similarly, a change in the depositional surface does not appear to be a plausible explanation for the increased clay content in the uppermost units because all of the fine-grained sediments at Ziegler Reservoir (including units 17 and 18) were deposited in a lacustrine environment, with the exception of units 15, 16, and possibly unit 12 (Pigati et al. Citation2014). Although the lake levels certainly would have varied over time, such changes would not have affected the size of the sediments deposited in the basin.
In our view, the most plausible explanation for the increase in clay in units 17 and 18 is an increase in both the proportion and volume of clay-sized material in the source area(s) during MIS 4. We hypothesize that unit 17 (ca. 77–71 ka) represents the transition from warm conditions during MIS 5a to cooler conditions during MIS 4, when local valley glaciers began to abrade the landscape, including sedimentary bedrock units, producing newly available, clay-rich rock flour that was carried downstream and deposited in glacio-fluvial settings. The clay-rich sediments were then entrained by winds and transported by eolian processes to the Ziegler Reservoir basin. This scenario likely continued through the entirety of MIS 4 (ca. 71–55 ka; unit 18), when glacial-like conditions prevailed (Miller et al. Citation2014; Anderson et al. Citation2020). Interestingly, the lack of environmental indicators (pollen, conifer cones, insects, ostracodes) in units 17 and 18 was also observed in units 3 and 4 at the base of the Ziegler Reservoir stratigraphic section, which suggests that conditions at the site during MIS 4 may have resembled those that prevailed at the end of MIS 6 (Miller et al. Citation2014).
Geologic mapping of the area revealed four distinct moraine deposits that Bryant (Citation1972) called Qma, Qmb, Qmc, and Qmd. Since then, Qma has been assigned to the Pinedale glacial stage (MIS 2), Qmc correlates with the Bull Lake stage (MIS 6), and Qmd is pre–Bull Lake in age (i.e., older than MIS 6; Pigati et al. Citation2014). In Snowmass Creek Valley, Qmb deposits extend at least 2 km beyond the limits of Qma (Pinedale) but not as far as Qmc (Bull Lake), which suggests that this unit was deposited during the early part of MIS 2 or possibly during MIS 4. Additional work is necessary to explore this possibility and should include cosmogenic dating of the Qmb moraine boulders, which has proven successful to identifying MIS 4 moraines worldwide (Doughty et al. Citation2021). If this unit is found to correlate with MIS 4, this would provide a rare opportunity to investigate surficial geologic deposits in the Rocky Mountains that date to this time period.
Finally, the use of rock flour as a proxy of alpine glaciation has been applied at sites throughout the Western United States (e.g., Menounos and Reasoner Citation1997; Bischoff and Cummins Citation2001; Rosenbaum and Reynolds Citation2004; Rosenbaum and Heil Citation2009). In these studies, glacially derived rock flour was transported by fluvial processes and deposited directly in lacustrine basins. Although glacial systems are well known for producing silt (Smalley Citation1966; Pye Citation1987, Citation1995; Bullard Citation2013), Bischoff and Cummins (Citation2001) found that clay material less than 2 µm in diameter was also present in glacially derived rock flour deposits that originated from plutonic bedrock. We contend that the clay-rich material found in units 17 and 18 was similarly derived from glacial grinding of local bedrock, especially sedimentary units, that produced clay-rich rock flour. Our conceptual model for sediment transport and deposition at Ziegler Reservoir only requires the additional step of being transported by eolian processes between the glacio-fluvial outwash plains and the fossil site, which is permitted by the mineralogical, elemental, and physical evidence. The results of our study show that indirect evidence of past glacial activity can be used to complement studies based on glacial moraines to provide a more complete view of past glacial activity, especially for MIS 4, in this part of North America.
Conclusions
In the Rocky Mountains, geologic evidence of glacial activity during MIS 4 was typically covered or destroyed by the subsequent, and larger, Pinedale glacial episode (MIS 2). Rocky Mountain glacial chronologies therefore frequently understate or altogether miss the effects of cooling during this time period, resulting in an incomplete picture of the response of high-elevation environments to climatic oscillations in the region. Utilizing indirect evidence of glacial activity during MIS 4 has the potential to add detail to the climatic history of the area and help shed light on this “missing” glaciation.
The Ziegler Reservoir fossil site in west-central Colorado contains a rare record of high-alpine climatic conditions in the Rockies that spans from the end of MIS 6 through the beginning of MIS 3. Fine-grained sediments at this closed-basin site are interpreted as eolian based on grain size distributions that are comparable to loess standards and other known loess deposits in the area, as well as a lack of evidence of alluvial or fluvial activity within the basin itself. Mineralogical and elemental data indicate that these sediments likely originated from one or more local sources, such as glacial moraines, glacio-fluvial terraces, and outwash plains.
A change from silt-rich to clay-rich sediments in the uppermost units of the Ziegler Reservoir stratigraphic record is temporally correlative with a cooling climate leading into MIS 4 and likely reflects a change in the dominant grain size in the source area(s). We hypothesize that production of rock flour caused by an increase in nearby glacial activity during MIS 4 provided a large quantity of fine-grained sediments that were deposited in nearby glacio-fluvial outwash plains. These grains were then entrained by local winds and deposited in the closed basin of the Ziegler Reservoir fossil site.
Although the MIS 4 glaciers in the region were not as large as those of the subsequent Pinedale (MIS 2) event, it appears that they were large enough to produce a considerable amount of clay-size material for transport to the surrounding region. Further work is necessary to quantify the precise timing and extents of glacial activity during MIS 4 within the Rocky Mountains and should include examination of indirect evidence of glacial activity. Combined with the continued study of glacial moraines, including possible MIS 4 moraines in Snowmass Creek Valley, this approach will improve our understanding of how alpine environments responded to changes in climatic conditions during the late Pleistocene.
Supplemental Material
Download PDF (1 MB)Acknowledgments
The authors recognize the significant contributions of Ken Pierce to our understanding of the glacial history within the Rocky Mountains; he will be missed. We thank Kathleen Springer, Janet Slate, and two anonymous reviewers for providing constructive reviews of this article. We also thank Jeremy Havens for assistance with the figures.
Disclosure statement
No potential conflict of interest was reported by the authors.
Supplementary material
Supplemental material for this article can be accessed on the publisher’s website
Additional information
Funding
References
- Adams, F., and R. Dams. 1975. Applied gamma-ray spectrometry, 294. 2nd ed. New York, NY: Pergamon Press.
- Anderson, R. S., G. Jiménez-Moreno, M. Belanger, and C. Briles. 2020. Fire history of the unique high-elevation Snowmastodon (Ziegler Reservoir) site during MIS 6–4, with comparisons of TII to TI in the southern Colorado Rockies. Quaternary Science Reviews 232:106213. doi:https://doi.org/10.1016/j.quascirev.2020.106213.
- Anderson, R. S., G. Jiménez-Moreno, T. Ager, and D. Porinchu. 2014. High-elevation paleoenvironmental change during MIS 6–4 in the central Rockies of Colorado as determined from pollen analysis. Quaternary Research 82 (3):542–52. doi:https://doi.org/10.1016/j.yqres.2014.03.005.
- Benson, L., R. Madole, G. Landis, and J. Gosse. 2005. New data for late Pleistocene Pinedale alpine glaciation from southwestern Colorado. Quaternary Science Reviews 24 (1–2):49–65. doi:https://doi.org/10.1016/j.quascirev.2004.07.018.
- Birkeland, P. W. 1973. Use of relative age-dating methods in a stratigraphic study of rock glacier deposits, Mt. Sopris, Colorado. Arctic and Alpine Research 5 (4):401–16. doi:https://doi.org/10.2307/1550131.
- Bischoff, J. L., and K. Cummins. 2001. Wisconsin glaciation of the Sierra Nevada (79,000–15,000 yr B.P.) as recorded by rock flour in sediments of Owens Lake, California. Quaternary Research 55 (1):14–24. doi:https://doi.org/10.1006/qres.2000.2183.
- Blaauw, M., and J. A. Christen. 2011. Flexible paleoclimate age-depth models using an autoregressive gamma process. Bayesian Analysis 6 (3):457–74. doi:https://doi.org/10.1214/ba/1339616472.
- Blackwelder, E. 1915. Post-Cretaceous history of the mountains of central western Wyoming. The Journal of Geology 23 (4):307–40. doi:https://doi.org/10.1086/622243.
- Briner, J. P., and D. S. Kaufman. 2008. Late Pleistocene mountain glaciation in Alaska: Key chronologies. Journal of Quaternary Science 23 (6–7):659–70. doi:https://doi.org/10.1002/jqs.1196.
- Briner, J. P., D. S. Kaufman, W. F. Manley, R. C. Finkel, and M. W. Caffee. 2005. Cosmogenic exposure dating of late Pleistocene moraine stabilization in Alaska. Geological Society of America Bulletin 117 (7):1108–20. doi:https://doi.org/10.1130/B25649.1.
- Brune, D., B. Forkman, and B. Persson. 1984. Nuclear analytical chemistry, Chapter 10. Sweden: Chartwell-Bratt Ltd.
- Bryant, B. 1972. Geologic map of the Highland Peak quadrangle, Pitkin County, Colorado. U.S. Geological Survey Geologic Quadrangle Map GQ-932, 1:24,000 scale.
- Bryant, B. 1979. Geology of the Aspen 15-minute quadrangle, Pitkin and Gunnison Counties, Colorado: U.S. Geological Survey Professional Paper 1073, 146.
- Bryant, B., and P. L. Martin. 1988. The geologic story of the Aspen region: U.S. Geological Survey Bulletin 1603, 53 pp.
- Bullard, J. E. 2013. Contemporary glacigenic inputs to the dust cycle. Earth and Planetary Science Letters 38:71–89.
- Chadwick, O. A., R. D. Hall, and F. M. Phillips. 1997. Chronology of Pleistocene glacial advances in the central Rocky Mountains. Geological Society of America Bulletin 109 (11):1443–52. doi:https://doi.org/10.1130/0016-7606(1997)109<1443:COPGAI>2.3.Co;2.
- Colman, S. M., and K. L. Pierce. 1981. Weathering rinds on andesitic and basaltic stones as a Quaternary age indicator, western United States. U.S. Geological Survey Professional Paper 1210, 56.
- Colman, S. M., and K. L. Pierce. 1992. Varied records of early Wisconsinan alpine glaciation in the western United States derived from weathering-rind thicknesses. In The last interglacial-glacial transition in North America, ed. P. U. Clark and P. D. Lea, 269–78. Boulder, Colorado: The Geologic Society of America, Inc.
- Dahms, D. E. 2004. Relative and numeric age data for Pleistocene glacial deposits and diamictons in and near Sinks Canyon, Wind River Range, Wyoming, U.S.A. Arctic, Antarctic, and Alpine Research 36 (1):59–77. doi:https://doi.org/10.1657/1523-0430(2004)036[0059:RANADF]2.0.CO;2.
- Dahms, D. E., M. Egli, D. Fabel, J. Harbor, D. Brandová, R. de Castro Portes, and M. Christl. 2018. Revised Quaternary glacial succession and post-LGM recession, southern Wind River Range, Wyoming, USA. Quaternary Science Reviews 192:167–84. doi:https://doi.org/10.1016/j.quascirev.2018.05.020.
- De Deckker, P., L.J., A., van der Kaars, S., Bayon, G., Stuut, J.-B. W., Perner, K., dos Santos, R. L., Uemura, R., and Demuro, M. 2019. Marine Isotope Stage 4 in Australasia: A full glacial culminating 65,000 years ago - global connections and implications for human dispersal. Quaternary Science Reviews 204: 187–207. doi: https://doi.org/10.1016/j.quascirev.2018.11.017.
- Doughty, A. M., M. R. Kaplan, C. Peltier, and S. Barker. 2021. A maximum in global glacier extent during MIS 4. Quaternary Science Reviews 261:106948. doi:https://doi.org/10.1016/j.quascirev.2021.106948.
- Douglass, D. C., and D. M. Mickelson. 2007. Soil development and glacial history, west fork of Beaver Creek, Uinta Mountains, Utah. Arctic, Antarctic, and Alpine Research 39 (4):592–602. doi:https://doi.org/10.1657/1523-0430(06-093)[DOUGLASS]2.0.CO;2.
- Elias, S. A. 2014. Environmental interpretation of fossil insect assemblages from MIS 5 at Ziegler Reservoir, Snowmass Village, Colorado. Quaternary Research 82 (3):592–603. doi:https://doi.org/10.1016/j.yqres.2014.01.005.
- Forman, S. L., R. P. Smith, W. R. Hackett, J. A. Tullis, and P. A. McDaniel. 1993. Timing of late Quaternary glaciations in the western United States based on the age of loess on the eastern Snake River Plain, Idaho. Quaternary Research 40 (1):30–37. doi:https://doi.org/10.1006/qres.1993.1053.
- Glushkova, O. Y. 2011. Late Pleistocene glaciations in North-East Asia. In Developments in Quaternary science, ed. J. Van der Meer, Vol. 15, 865–75. Amsterdam: Elsevier.
- Gray, A. B., G. B. Pasternack, and E. B. Watson. 2010. Hydrogen peroxide treatment effects on the particle size distribution of alluvial and marsh sediments. The Holocene 20 (2):293–301. doi:https://doi.org/10.1177/0959683609350390.
- Hall, R. D., and R. R. Shroba. 1995. Soil evidence for a glaciation intermediate between the Bull Lake and Pinedale Glaciations at Fremont Lake, Wind River Range, Wyoming, USA. Arctic, Antarctic, and Alpine Research 27 (1):89–98. doi:https://doi.org/10.2307/1552071.
- Hallberg, G. R., J. R. Lucas, and C. M. Goodmen. 1978. Semi-quantitative analysis of clay mineralogy. In Standard procedures for evaluation of Quaternary materials in Iowa, ed. G. R. Hallberg, 5–22. Iowa City: Iowa Geological Survey.
- Haskett, D. R., and D. F. Porinchu. 2014. A quantitative midge-based reconstruction of mean July air temperature from a high-elevation site in central Colorado, USA, for MIS 6 and 5. Quaternary Research 82 (3):580–91. doi:https://doi.org/10.1016/j.yqres.2014.05.002.
- Holmes, G. W., and J. H. Moss. 1955. Pleistocene geology of the southwestern Wind River Mountains, Wyoming. Geological Society of America Bulletin 66 (6):629–64. doi:https://doi.org/10.1130/0016-7606(1955)66[629:PGOTSW]2.0.CO;2.
- Imbrie, J., A. Berger, E. A. Boyle, S. C. Clemens, A. Duffy, W. R. Howard, G. Kukla, J. Kutzbach, D. G. Martinson, A. McIntyre, et al. 1993. On the structure and origin of major glaciation cycles 2. The 100,000-year cycle. Paleoceanography and Paleoclimatology 8 (6):699–735. doi:https://doi.org/10.1029/93PA02751.
- Ivy-Ochs, S., H. Kerschner, A. Reuther, F. Preusser, K. Heine, M. Maisch, P. W. Kubik, and C. Schluchter. 2008. Chronology of the last glacial cycle in the European Alps. Journal of Quaternary Science 23 (6–7):559–73. doi:https://doi.org/10.1002/jqs.1202.
- Johnson, K. R., I. M. Miller, and J. S. Pigati. 2014. Introduction to the Snowmastodon project. Quaternary Research 82 (3):473–76. doi:https://doi.org/10.1016/j.yqres.2013.12.010.
- Kaufman, D. S., and C. H. Thompson. 1998. Re-evaluation of pre-late Wisconsin glacial deposits, lower Naknek River Valley, Southwestern Alaska, USA. Arctic, Antarctic, and Alpine Research 30 (2):473–76. doi:https://doi.org/10.2307/1552129.
- Kaufman, D. S., N. E. Young, J. P. Briner, and W. F. Manley. 2011. Alaska Palaeo-Glacier Atlas (version 2). In Developments in Quaternary Science, eds J. Van der Meer, vol. 15, 427–45. Amsterdam: Elsevier.
- Laabs, B. J. C., J. M. Licciardi, E. M. Leonard, J. S. Munroe, and D. W. Marchetti. 2020. Updated cosmogenic chronologies of Pleistocene mountain glaciation in the western United States and associated paleoclimate inferences. Quaternary Science Reviews 242:106427. doi:https://doi.org/10.1016/j.quascirev.2020.106427.
- Licciardi, J. M., and K. L. Pierce. 2008. Cosmogenic exposure-age chronologies of Pinedale and Bull Lake glaciations in greater Yellowstone and the Teton Range, USA. Quaternary Science Reviews 27 (7–8):814–31. doi:https://doi.org/10.1016/j.quascirev.2007.12.005.
- Licciardi, J. M., and K. L. Pierce. 2018. History and dynamics of the greater Yellowstone glacial system during the last two glaciations. Quaternary Science Reviews 200:1–33. doi:https://doi.org/10.1016/j.quascirev.2018.08.027.
- Lisiecki, L. E., and M. E. Raymo. 2005. A Pliocene-Pleistocene stack of 57 globally distributed benthic δ18O records. Paleoceanography 2:1–17.
- Mahan, S. A., H. J. Gray, J. S. Pigati, J. Wilson, N. A. Lifton, J. B. Paces, and M. Blaauw. 2014. A geochronologic framework for the Ziegler Reservoir fossil site, Snowmass Village, Colorado. Quaternary Research 82 (3):490–503. doi:https://doi.org/10.1016/j.yqres.2014.03.004.
- Martinson, D. G., N. G. Pisias, J. D. Hays, J. Imbrie, T. C. Moore Jr., and N. J. Shackleton. 1987. Age dating and the orbital theory of the ice ages: Development of a high-resolution 0 to 300,000-yr chronostratigraphy. Quaternary Research 2:71–29.
- McCalpin, J. P., and J. R. Irvine. 1995. Sackungen at the Aspen Highlands Ski Area, Pitkin County, Colorado. Environmental and Engineering Geoscience I (3):277–90. doi:https://doi.org/10.2113/gseegeosci.I.3.277.
- McCarthy, A., A. Mackintosh, U. Rieser, and D. Fink. 2008. Mountain Glacier chronology from Boulder Lake, New Zealand, indicates MIS 4 and MIS 2 ice advances of similar extent. Arctic, Antarctic, and Alpine Research 40 (4):695–708. doi:https://doi.org/10.1657/1523-0430(06-111)[MCCARTHY]2.0.CO;2.
- Mears, B. 1974. The evolution of the Rocky Mountain glacial model. In Glacial geomorphology, publications in geomorphology, ed. D. R. Coates, 11–40. Binghamton: State University of New York.
- Menking, J. A., E. J. Brook, S. A. Shackleton, J. P. Severinghaus, M. N. Dyonisius, V. Petrenko, J. R. McConnell, R. H. Rhodes, T. K. Bauska, D. Baggenstos, et al. 2019. Spatial pattern of accumulation at Taylor Dome during marine isotope stage 4: Stratigraphic constraints from Taylor Glacier. Climate of the Past 15 (4):1537–56. doi:https://doi.org/10.5194/cp-15-1537-2019.
- Menounos, B., and M. A. Reasoner. 1997. Evidence for cirque glaciation in the Colorado Front Range during the Younger Dryas chronozone. Quaternary Research 48 (1):38–47. doi:https://doi.org/10.1006/qres.1997.1902.
- Miller, I. M., J. S. Pigati, R. S. Anderson, K. R. Johnson, S. A. Mahan, T. A. Ager, R. G. Baker, M. Blaauw, J. Bright, P. M. Brown, et al. 2014. Summary of the Snowmastodon project special volume: A high-elevation, multi-proxy biotic and environmental record of MIS 6–4 from the Ziegler Reservoir fossil site, Snowmass Village, Colorado, USA. Quaternary Research 82 (3):618–34. doi:https://doi.org/10.1016/j.yqres.2014.07.004.
- Moore, D. M., and R. C. Reynolds. 1997. X-ray diffraction and the identification and analysis of clay minerals. 2nd ed. New York, NY: Oxford University Press.
- Oviatt, C. G., and W. D. McCoy. 1992. Early Wisconsin lakes and glaciers in the Great Basin, USA. In The last interglacial-glacial transition in North America, ed. P. U. Clark and P. D. Lea, Geological Society of America Special Paper 270, 279–87. Boulder, Colorado: The Geologic Society of America, Inc.
- Peltier, C., M. R. Kaplan, S. D. Birkel, R. L. Soteres, E. A. Sagredo, J. C. Aravena, J. P. I. M. Araos, P. I. Moreno, R. Schwartz, and J. M. Schaefer. 2021. The large MIS 4 and long MIS 2 glacier maxima on the southern tip of South America. Quaternary Science Reviews 262:106858. doi:https://doi.org/10.1016/j.quascirev.2021.106858.
- Phillips, F. M., M. G. Zreda, J. C. Gosse, J. Klein, E. B. Evenson, R. D. Hall, O. A. Chadwick, and P. Sharma. 1997. Cosmogenic 36Cl and 10Be ages of Quaternary glacial and fluvial deposits of the Wind River Range, Wyoming. Geological Society of America Bulletin 109 (11):1453–63. doi:https://doi.org/10.1130/0016-7606(1997)109<1453:CCABAO>2.3.CO;2.
- Pierce, K. L. 2003. Pleistocene glaciations of the Rocky Mountains. Developments in Quaternary Science 1:63–76.
- Pierce, K. L., D. R. Muhs, M. A. Fosberg, S. A. Mahan, J. G. Rosenbaum, J. M. Licciardi, and M. J. Pavich. 2011. A loess–paleosol record of climate and glacial history over the past two glacial–interglacial cycles (~150 ka), southern Jackson Hole, Wyoming. Quaternary Research 76 (1):119–41. doi:https://doi.org/10.1016/j.yqres.2011.03.006.
- Pierce, K. L., J. D. Obradovich, and I. Friendman. 1976. Obsidian hydration dating and correlation of Bull Lake and Pinedale Glaciations near West Yellowstone, Montana. Geological Society of America Bulletin 87 (5):703–10. doi:https://doi.org/10.1130/0016-7606(1976)87<703:OHDACO>2.0.CO;2.
- Piety, L. A. 1981. Relative dating of terrace deposits and tills in the Roaring Fork valley, Colorado, MS Thesis. University of Colorado, Boulder. 209 pp.
- Pigati, J. S., I. M. Miller, K. R. Johnson, J. S. Honke, P. E. Carrara, D. R. Muhs, G. Skipp, and B. Bryant. 2014. Geologic setting and stratigraphy of the Ziegler Reservoir fossil site, Snowmass Village, Colorado. Quaternary Research 82 (3):477–89. doi:https://doi.org/10.1016/j.yqres.2013.12.011.
- Pye, K. 1987. Aeolian dust and dust deposits. London: Academic Press.
- Pye, K. 1995. The nature, origin and accumulation of loess. Quaternary Science Reviews 14 (7–8):653–67. doi:https://doi.org/10.1016/0277-3791(95)00047-X.
- Reheis, M. C. 2006. A 16-year record of eolian dust in southern Nevada and California, USA: Controls on dust generation and accumulation. Journal of Arid Environments 67 (3):487–520. doi:https://doi.org/10.1016/j.jaridenv.2006.03.006.
- Reheis, M. C., and R. Kihl. 1995. Dust deposition in southern Nevada and California, 1984–1989: Relations to climate, source area, and source lithology. Journal of Geophysical Research 100 (D5):8893–918. doi:https://doi.org/10.1029/94JD03245.
- Richmond, G. M. 1986. Stratigraphy and correlation of glacial deposits of the Rocky Mountains, the Colorado Plateau and the ranges of the Great Basin. Quaternary Science Reviews 5:99–127. doi:https://doi.org/10.1016/S0277-3791(86)80013-0.
- Rosenbaum, J. G., and C. W. Heil Jr. 2009. The glacial/deglacial history of sedimentation in Bear Lake, Utah and Idaho. In Paleoenvironments of Bear Lake, Utah and Idaho, and its catchment. Geological Society of America Special Paper 450, ed. J. G. Rosenbaum and D. S. Kaufman, 247–61. Boulder, Colorado: The Geologic Society of America.
- Rosenbaum, J. G., and R. L. Reynolds. 2004. Record of late Pleistocene glaciation and deglaciation in the southern cascade range. II. Flux of glacial flour in a sediment core from Upper Klamath Lake, Oregon. Journal of Paleolimnology 31 (2):235–52. doi:https://doi.org/10.1023/B:JOPL.0000019229.75336.7a.
- Seidenkrantz, M. S., A. Kuijpers, J. Olsen, C. Pearce, S. Lindblom, J. Ploug, P. Przybyło, and I. Snowball. 2019. Southwest Greenland shelf glaciation during MIS 4 more extensive than during the last glacial maximum. Nature Scientific Reports 9:15617. doi:https://doi.org/10.1038/s41598-41019-51983-41593.
- Sertich, J. J. W., R. K. Stucky, H. G. McDonald, C. Newton, D. C. Fisher, E. Scott, J. R. Demboski, C. Lucking, B. K. McHorse, and E. B. Davis. 2014. High-elevation late Pleistocene (MIS 6–5) vertebrate faunas from the Ziegler Reservoir fossil site, Snowmass Village, Colorado. Quaternary Research 82 (3):504–17. doi:https://doi.org/10.1016/j.yqres.2014.08.002.
- Sharp, W. D., K. R. Ludwig, O. A. Chadwick, R. Amundson, and L. L. Glaserd. 2003. Dating fluvial terraces by 230Th/U on pedogenic carbonate, Wind River Basin, Wyoming. Quaternary Research 59 (2):139–50. doi:https://doi.org/10.1016/S0033-5894(03)00003-6.
- Smalley, I. J. 1966. The properties of glacial loess and the formation of loess deposits. SEPM Journal of Sedimentary Research 36:669–76. doi:https://doi.org/10.1306/74D7153C-2B21-11D7-8648000102C1865D.
- Smith, J. A., and D. T. Rodbell. 2010. Cross-cutting moraines reveal evidence for North Atlantic influence on glaciers in the tropical Andes. Journal of Quaternary Science 25 (3):243–48. doi:https://doi.org/10.1002/jqs.1393.
- Strickland, L. E., R. G. Baker, R. S. Thompson, and D. M. Miller. 2014. Last interglacial plant macrofossils and climates from Ziegler Reservoir, Snowmass Village, Colorado, USA. Quaternary Research 82 (3):553–66. doi:https://doi.org/10.1016/j.yqres.2014.07.008.
- Taylor, S. R., and S. M. McLennan. 1985. The continental crust: Its composition and evolution: An examination of the geochemical record preserved in sedimentary rocks, 312. Oxford: Blackwell Scientific Publications.
- Taylor, S. R., S. M. McLennan, and M. T. McCulloch. 1983. Geochemistry of loess, continental crustal composition and crustal model ages. Geochimica et Cosmochimica Acta 47 (11):1897–905. doi:https://doi.org/10.1016/0016-7037(83)90206-5.
- Thackray, G. D. 2001. Extensive early and middle Wisconsin glaciation on the western Olympic Peninsula, Washington, and the variability of Pacific moisture delivery to the northwestern United States. Quaternary Research 55 (3):257–70. doi:https://doi.org/10.1006/qres.2001.2220.
- Troost, K. G. 2016. Chronology, lithology and paleoenvironmental interpretations of the penultimate ice-sheet advance into the Puget Lowland, Washington State, earth and space sciences, 239. Seattle, Washington: University of Washington.
- U.S. Department of Agriculture, Soil Conservation Service, Soil Survey Staff. 1993. National soils handbook. Washington, DC: U.S. Department of Agriculture.
- Ward, B. C., J. D. Bond, and J. C. Gosse. 2007. Evidence for a 55–50 ka (early Wisconsin) glaciation of the Cordilleran ice sheet, Yukon Territory, Canada. Quaternary Research 68 (1):141–50. doi:https://doi.org/10.1016/j.yqres.2007.04.002.