ABSTRACT
Increased northern exploration and resource extraction highlight a need for effective revegetation techniques to restore disturbed environments. This study assessed effects of indole-3-butyric acid (IBA), water extracts of Salix and smoke, soaking time, and collection time on adventitious and lateral root development of Salix species cuttings collected from Diavik Diamond Mine Inc., Northwest Territories. Over 80 percent of fall and spring cuttings developed adventitious roots, yet only 30 percent of summer cuttings rooted, indicating strong seasonal influences. Many cuttings developed extensive root system architecture in 60 days; some developed up to six orders of roots. Root length decreased with increasing root order in all seasons, and season influenced length within root orders. Application of IBA increased number of primary roots per cutting per season and number of cuttings with less than fifty secondary roots per primary root. Longer soaking times increased number of primary roots per cutting in different seasons, and soaking up to ten days increased longest root length. Salix and smoke water extract applications increased number of cuttings with twenty-five to seventy-four secondary roots. This research highlights the importance of treatment effects on adventitious and lateral root development to optimize root system architecture of cuttings from northern shrub species.
Introduction
Significant exploration and mineral resource extraction over the past century in the Canadian north have left large, long-lasting disturbances in this unique ecosystem, highlighting the need for effective revegetation techniques. Because natural recovery may take decades or centuries, reclamation practitioners have often relied on seeding early successional species such as cold-tolerant grasses and legumes to accelerate revegetation, because they can be purchased commercially (Claridge and Mirza Citation1981; Densmore Citation1992; Wright Citation2008). However, this has been unreliable for developing appropriate shrub heath tundra communities (Younkin and Martens Citation1987; Densmore Citation1992; Forbes and Jefferies Citation1999), and determining techniques that consistently promote adventitious root development on arctic shrub cuttings could lead to faster and more effective revegetation in harsh northern environments.
Plant roots play numerous structural and functional roles necessary for plant growth and survival, including anchorage and stability, resource acquisition and transportation, propagation, and storage of resources, and they provide important ecological benefits through assisting with local, regional, and global biogeochemical cycling of nutrients and water (Pendall et al. Citation2004; Bardgett, Mommer, and De Vries Citation2014; Austin and Zanne Citation2015). In northern locations, plant functions such as photosynthesis, nutrient uptake, and growth decrease as mean environmental temperature decreases and plants allocate a larger portion of total biomass to roots, reaching 70 percent or more in tundra environments (Chapin, Johnson, and McKendrick Citation1980; Poorter et al. Citation2012).
Intrinsic genetic pathways control species-specific traits such as root length and growth, branch number and pattern, and diameter, which may be modulated by changing environmental conditions (Malamy Citation2005; Jung and McCouch Citation2013; Morris et al. Citation2017). This phenotypic plasticity allows plants to adapt to their surrounding environment and influences architectural, morphological, physiological, and biotic traits of the mature root system of even genetically identical plants (Nicotra et al. Citation2010; Bardgett, Mommer, and De Vries Citation2014; Fromm Citation2019). Growth of the primary root in dicotyledons such as Salix species produces a well-developed taproot, or allorhizic system from which lateral roots may emerge forming secondary and higher orders of root branches (Atkinson et al. Citation2014; Bellini, Pacurar, and Perrone Citation2014). Regulation of different stages of lateral root formation leads to a characteristic root system architecture for different plant species (Malamy and Benfey Citation1997; Malamy Citation2005; Laplaze et al. Citation2007). Understanding these influences on root architecture can help us to develop successful revegetation techniques.
The ability to produce adventitious roots under natural or stressed circumstances such as wounding has been used by horticultural and forestry industries for food production and economic and ecological benefits (Bellini, Pacurar, and Perrone Citation2014; Steffens and Rasmussen Citation2016). In northern environments, revegetation of disturbed areas using shrub cuttings is a potentially promising reclamation technique because there are currently no commercial suppliers of seed for northern shrub species (Hagen Citation2002; Holloway and Peterburs Citation2009; Matheus and Omtzigt Citation2011; Ficko, Smith, and Zeeb Citation2015; Ficko and Naeth Citation2021). Shrub species such as Salix alaxensis (Andersson) Coville (felt leaf willow), Salix arctica Pall. (arctic willow), Salix glauca (gray willow), and Salix planifolia (diamond leaf willow) are known to quickly develop adventitious roots on cuttings with limited assistance, because they have preformed root primordia, making them preferred species for northern revegetation (Densmore, Vander Meer, and Dunkle Citation2000; Walter et al. Citation2005; Naeth and Wilkinson Citation2011; Ficko, Smith, and Zeeb Citation2015). Guidelines typically recommend collecting cuttings in fall or spring and soaking them for 24 to 48 hours before planting. However, limited research has been conducted on longer soaking times for cuttings that cannot be planted immediately due to work or environmental delays or collecting cuttings in summer if the site is inaccessible in other months.
Growth hormones such as indole-3-butyric acid (IBA), a naturally produced endogenous auxin, are commonly applied to cuttings to stimulate adventitious rooting (Davies et al. Citation2017). Auxin synthesis occurs mainly in young plant leaves and is transported by bulk flow through the vascular system to various tissues in response to environmental stimuli and interactions with other hormones and growth regulators (Overvoorde, Fukaki, and Beeckman Citation2010; Pop, Pamfil, and Bellini Citation2011). Directional movement into cells through integral membrane transport proteins creates local auxin gradients that play an essential role in regulating root architecture and development, including adventitious and lateral root formation (Malamy Citation2005; Overvoorde, Fukaki, and Beeckman Citation2010; Olatunji, Geelen, and Verstraeten Citation2017). Salix water extract contains the phytohormone salicylic acid and a number of other compounds that have fungicidal, insecticidal, antibacterial, and root promoting properties (Sati, Sati, and Singh Citation2011; Al-Amad and Qrunfleh Citation2016; Singh, Raturi, and Badoni Citation2017; Deniau et al. Citation2019; Javed, Nawaz, and Munazir Citation2020). It has commonly been used by home gardeners as an alternative treatment to improve rooting of cuttings, with several studies recently showing it can improve rooting for Olea europaea L. (European olive), Coleus scutellarioides (L.) Benth. (common coleus), Chrysanthemum sp. (chrysanthemum), Lavandula x hybrid ‘Frills’ PBR (lavender) and several arctic species (Al-Amad and Qrunfleh Citation2016; Koriesh et al. Citation2018; Wise, Gill, and Selby-Pham Citation2020; Ficko and Naeth Citation2021). Smoke water extract is another alternative treatment because karrikins in fire and smoke are known to improve germination for numerous species and to play a role in regulating root development (Chiwocha et al. Citation2009; Akeel et al. Citation2019; Swarbreck Citation2021). The only study to assess use of smoke water extract on cuttings found species-specific interactions between season and smoke water extract for seven northern species (Arctous rubra [Rehder & Wilson] Fernald [red bearberry], Empetrum nigrum L. [crowberry], Kalmia procumbens [L.] Gift & Kron & P.F. Stevens ex Galasso, Banfi & F. Conti [alpine azalea], Rhododendron tomentosum Harmaja [marsh Labrador tea], Vaccinium uliginosum L. [bog bilberry], and Vaccinium vitis-idaea L. [bog cranberry]), although results were limited by low rooting percentages (Ficko and Naeth Citation2021).
With increasing exploration and resource extraction in the north, developing a better understanding of root system architecture for Salix species grown from cuttings with different rooting techniques and how their root system architecture compares to plants grown in undisturbed tundra is expected to improve revegetation success. The main objectives of our study were to assess effects of collection time, common rooting treatments (IBA concentration, soaking length), and novel rooting treatments (water extracts of Salix and smoke) on adventitious and lateral root development of Salix spp. cuttings collected from Diavik Diamond Mine Inc., Northwest Territories, and grown under controlled conditions in a growth chamber. Because lateral root development has rarely been studied on cuttings from arctic species, determining which treatments optimize root system architecture and not just adventitious rooting can improve revegetation of disturbed northern sites.
Materials and methods
Experimental design
Cuttings were collected from growing tips of shrubs in an undisturbed upland dwarf heath tundra community at Diavik Diamond Mine, Northwest Territories (64°30′41″ N, 110°17′23″ W). Salix glauca L. (grayleaf willow) and Salix planifolia Pursh (diamond leaf willow) are the two main Salix species in the community but were low in abundance relative to other shrub species, including Arctous rubra (Rehder & Wilson) Fernald (red bearberry), Betula glandulosa Michx. (bog birch), Empetrum nigrum L. (crowberry), Kalmia procumbens (L.) Gift & Kron & P.F. Stevens ex Galasso, Banfi & F. Conti (alpine azalea), Rhododendron tomentosum Harmaja (marsh Labrador tea), Vaccinium uliginosum L. (bog bilberry), and Vaccinium vitis-idaea L. (bog cranberry). Cuttings from both Salix species were collected together for the experiments, similar to how a reclamation practitioner would collect them in the field. Cuttings were not separated by species as both successfully rooted with no significant differences in a preliminary trial (70 to 100 percent; Naeth and Wilkinson Citation2011). They grew together in the plant community, making them difficult to separately identify in different seasons if leaves and/or catkins were not present. Cuttings varied in length from 10 to 40 cm based on stem length of available plants and were planted in a 50:50 by volume peat moss and potting soil mix within one week at the University of Alberta.
The effects of common horticultural and novel treatments on adventitious and lateral root development were assessed in two screening studies. Cuttings in experiment 1 were collected in summer (25–26 June), fall (19–20 September), and spring (20–21 May) and treated with soaking times (zero, one, three, five, ten, and twenty days) and IBA concentrations (0, 0.1, 0.4, 0.8 percent) for a total of 72 treatments (summer cuttings had no 0.4 percent IBA due to growth chamber space restrictions, Supplementary Table 1). There were 3, 6, and 9 cuttings per treatment group (Soak × IBA concentration) in summer, fall, and spring for a total of 54, 144, and 216 cuttings, respectively. Experiment 2 cuttings were collected in fall (27–28 September) and untreated or treated with IBA concentrations (0.1, 0.4, 0.8 percent), Salix water extract (0.5, 1, 2), or smoke water extract (0.05, 0.1, 0.5). Salix water extracts were made by placing 300, 600, and 1,200 mL of cuttings (1- to 3-cm pieces) in 2,400 mL boiling distilled water and soaking for 12 hours. Smoke water extracts were made by placing 4 L distilled water in a smoker with 1.2 kg wood chips for 4 hours and then diluting with distilled water (1:20, 1:10, 1:1 extract to distilled water by volume). There were 10 cuttings per treatment group for a total of 100 cuttings. Tap water was maintained at a depth of 5 cm in containers for soaking treatments. Approximately 5 cm of stem bottom was dipped in IBA powder prior to planting for IBA treatments, whereas for Salix and smoke water extract treatments the bottom 5 cm of cuttings was soaked for 12 hours prior to planting.
Three cuttings per species per treatment (Soaking time × IBA concentration) were planted in the same 10 × 10 × 10 cm container in each season in experiment 1 due to growth chamber space restrictions. For experiment 2, each cutting was planted in an individual 10 × 3 × 3 cm root trainer. Cuttings were placed in a growth chamber for sixty days and then roots were gently rubbed under running water to remove all substrate. Growth chamber conditions were set at 17°C/10°C for 16/8-hour photoperiods in experiment 1 and 17°C/10°C for 20/4-hour photoperiods in experiment 2 to mimic different growing conditions at Diavik.
“Primary root” refers to the adventitious root that emerged from the stem cutting; “secondary,” “tertiary,” and “quaternary” roots refer to successive orders of lateral root branches off the primary root (Lecompte and Pagès Citation2007). To assess root development patterns, primary adventitious roots on each cutting were counted; lengths of longest primary, secondary, tertiary, and quaternary roots on each cutting were measured; and number of secondary roots on each primary root were assessed under a microscope and placed in categories (0, 1–24, 25–49, 50–74, 75–99, and >100) due to significant branching. Presence of callus was noted for cuttings in experiment 1, because callus is part of the wound response and is a precursor for rooting in some (but not all) species (Davies et al. Citation2017). Shoot health and vigor of each cutting were assessed at thirty and sixty days using a five-point scale with 1 = dead, 2 = poor (plant mostly dead or dying, <30 percent live green tissue), 3 = fair (average health and growth, 30 to 60 percent live green tissue), 4 = good (plant healthy and growing, 60 to 90 percent live green tissue), and 5 = excellent (plant robust and growing vigorously, 90 to 100 percent live green tissue.
Statistical analyses
All model estimation and statistical analyses were conducted using R (v4.0.2). One fall cutting in experiment 1 was removed from the data set prior to analysis because it had eighty roots (greater than three standard deviations from the mean). Cuttings were assessed separately by season because some species root more readily at certain times of year.
Due to low number of replicates per treatment, two-thirds of cuttings with roots per season per experiment was considered the minimum requirement for analysis in a hurdle model to determine treatment effects. Fall and spring cuttings in experiment 1 and fall cuttings in experiment 2 met this condition. The first step in the model was to predict probability that a cutting produced at least one root; the second step was to predict number of roots, longest root length, and proportion of rooted cuttings with shoot health 5. Mean shoot health, longest root length for different root orders at different times of year in each experiment, number of secondary roots per primary root, and number of roots and length of root with and without callus were presented graphically using ggplot2 in R (Wickham Citation2016).
In experiment 1, a series of ten models with combinations of the independent variables IBA concentration and soaking time was constructed for each dependent variable: (1) null model (no variables); (2) IBA concentration; (3) soak; (4) IBA concentration and soak; (5) IBA concentration and IBA concentration squared; (6) soak and soak squared; (7) IBA concentration, soak, and soak squared; (8) IBA concentration, soak, and IBA concentration squared; (9) IBA concentration, soak, IBA concentration squared, and soak squared; and (10) IBA concentration, soak, and IBA concentration × Soak (interaction). All experiment 1 models were fitted with the glmmTMB package and glmmTMB function, with pot as a random variable. Models were compared using the Akaike information criterion (AIC), ranked by weight to determine probability of being the best model, and a cumulative probability of at least 0.9 was calculated for each dependent variable (Anderson Citation2008). Because rooting is binary (present or absent), logistic regression was used to determine proportion of cuttings rooted in the first step for both experiments. For step 2, mean number of roots produced by cuttings with at least one root was predicted using zero truncated negative binomial regression because it could account for overdispersion, variability in distribution, and count data. Mean length of the longest root was predicted by multiple linear regression, and proportion of cuttings with shoot health 5 was predicted by logistic regression.
In experiment 2, a fixed effects logistic regression model for proportion of cuttings that rooted with the control and nine treatments as independent variables was fitted with the MASS package and GLM function. For step 2, the Kruskal-Wallis rank sum test was used to compare the control group to nine treatment groups for mean number of roots per rooted cutting and mean length of longest root, because models developed using parametric tests fit poorly. Dunnett’s test for multiple comparisons with one control and a Hochberg adjustment was fitted from the PMCMR package to determine significant differences between the control group and each treatment group. The proportion of cuttings with shoot health 5 was predicted using logistic regression.
Results and discussion
Shoot health
Cuttings that rooted had higher shoot health by 214 percent than those that did not root regardless of treatment (Supplementary Figure 1). Shoot health of rooted cuttings in experiment 1 was 3.3 to 5 for fall and spring cuttings across IBA concentration and soaking time. Only a few summer cuttings rooted, with shoot health variable. Shoot health of unrooted cuttings was 1 to 4 for fall and spring cuttings and ≤2 for summer cuttings. In a preliminary study at Diavik, shoot health of five fall collected Salix planifolia cuttings (60 percent rooted) was 1.4 (SD 0.4) after twelve weeks in a growth chamber (Naeth and Wilkinson Citation2011). Because root development enables a plant to access nutrients and water from the soil, shoot health was stable or increased from day 30 to day 60 for rooted cuttings in both experiments in all seasons (Supplementary Figure 2). Fall and spring (but not summer) cuttings with higher shoot health at day 60 had more roots (data not shown). Using shoot health as a proxy for rooting could save time and minimize disruption to the cutting because roots do not need to be visually assessed; however, our results indicate that shoot health is not sufficiently correlated with rooting.
Percentage of rooted cuttings with shoot health 5 in experiment 1 was 42 to 75 for fall collected cuttings and 53 to 75 for spring. The null model had most weight in both seasons (), although soaking had a similar probability in spring, generally decreasing the percentage with shoot health 5. In experiment 2, shoots were typically very healthy, regardless of treatment. Percentage of rooted cuttings with shoot health 5 was 100 for all treatments except IBA 0.1 (89 percent) and Salix water 2 (86 percent). Neither IBA concentration nor soaking length had a strong effect on shoot health in this study. In comparison, Houle and Babeux (Citation1998) found that a 1 percent IBA concentration decreased the percentage of Salix planifolia cuttings that developed leaves, lateral shoot number, and lateral shoot biomass, and Schaff, Pezeshki, and Shields (Citation2002) found that Salix nigra cuttings soaked for ten days had higher shoot and leaf biomass than unsoaked cuttings or cuttings soaked for three days. Though scoring shoot health with a point scale is a faster method of assessing above ground growth, these results indicate that other methods such as counting shoot number or measuring shoot biomass may provide more information on treatment effects.
Table 1. Experiment 1 model rankings and probabilities for fall and spring cuttings predicting proportion of cuttings that rooted and for cuttings with at least one root, predicting number of roots, longest root length, and proportion with shoot health 5
Root development
Cuttings had consistently high rooting, with 82 percent (421 of 514 cuttings) developing adventitious roots across all experiments and treatments. Parent root elongation and subsequent lateral root branching is a complex process involving plant genetics, hormones, and environmental factors (Malamy Citation2005; Nibau, Gibbs, and Coates Citation2008; Atkinson et al. Citation2014). Cuttings generally had extensive branching, with some secondary through senary orders of lateral roots developing within sixty days. Of cuttings with primary roots, 396 developed secondary (94 percent), 360 developed tertiary (86 percent), and 117 developed quaternary roots (28 percent; quintenary and senary root orders not consistently assessed). Salix species (e.g., Salix alba L. [white willow], Salix caprea L. [goat willow], Salix cinerea L. [European gray willow], Salix eleagnos Scop. [hoary willow], Salix glabra Scop. [smooth willow], Salix pulchra Cham. [tea leaf willow], and Salix purpurea L. [basket willow]) are known to produce numerous lateral roots (Kutschera and Lichtenegger Citation2002; Iversen et al. Citation2015), indicating that intrinsic genetic factors likely determined extensive production of lateral roots in our study.
Response to season
Season was most influential on callus formation, with 75.7 ± 0.0 percent of fall cuttings, 78.2 ± 0.0 percent of spring cuttings, and 0 percent of summer cuttings producing callus, and on adventitious root development, with 30 ± 0.1 percent of summer cuttings and more than 80 percent of fall (both experiments) and spring cuttings rooted (Supplementary Table 2). Our results were similar to those of Houle and Babeux (Citation1993), with Salix planifolia cuttings having high rooting shortly before or after bud break in May and June and after dormancy in September and October and significantly lower in July and August. Holloway and Peterburs (Citation2009) found significant rooting (>80 percent) for two species in Alaska (Salix alaxensis var. longistylis [Rydb.] Schneid. [Alaska willow] and Salix arbusculoides Anderss. [little tree willow]) when collected mid- to early July and poor rooting (<25 percent) for one species (Salix bebbiana Sarg. [Bebb’s willow]) regardless of collection time. Some Salix species, especially from riparian areas, have preformed root primorida, which allow cuttings to rapidly develop roots, whereas other Salix species must undergo cell dedifferentiation before induction of new root primordia (Haissig Citation1974; Krasny, Zasada, and Vogt Citation1988; Davies et al. Citation2017). Though our cuttings were not identified to species, high rooting in fall and spring but not summer indicates a stronger influence of season on adventitious rooting than species, because all cuttings were collected in a similar manner from the same location each season. Environmental factors such as photoperiod, precipitation, and air temperature that affect physiological status and health of the donor plant prior to harvesting likely underly the effect of season on adventitious rooting (Bellini, Pacurar, and Perrone Citation2014; Davies et al. Citation2017).
Lateral roots have greatest plasticity among different root types, allowing plants to respond to changing environmental conditions such as soil water, nitrogen, and phosphorus concentrations (Postma, Dathe, and Lynch Citation2014; Robbins and Dinneny Citation2015; Chen et al. Citation2018). Longest root length decreased with successive root orders for cuttings (). Within a root order, season and experiment appeared to influence longest root length. For root branching, diameter of a parent root influences diameter and length of subsequent lateral roots (Lecompte and Pagès Citation2007; Wu, Pagès, and Wu Citation2016). Summer cuttings in our study generally had smaller adventitious roots than fall or spring cuttings and fewer and shorter lateral roots. Because all cuttings in our study were grown in a similar soil mixture, differences in lengths between seasons within a root order in experiment 1, within a root order between fall experiment 1 and 2 cuttings, and percentage of roots in secondary root categories between experiments 1 and 2 () are likely due to differences in growth chamber conditions or differences in health and resources available in donor cuttings at different times of year or in different years. In comparison, there was little difference between number of roots in secondary root categories for fall and spring cuttings in experiment 1, except category 75–99 had twice as many spring cuttings with secondary roots as fall cuttings (, Supplementary Figure 3). Because both number and length of lateral roots strongly affect root system architecture of a plant, selecting dormant Salix cuttings will likely be most successful in developing an extensive root system suitable for revegetation in the north.
Figure 1. Length of longest primary, secondary, tertiary, and quaternary roots with standard error at day 60 for rooted Salix spp. cuttings in experiments 1 and 2 at different times of year. Length of longest quaternary roots was not assessed for summer cuttings in experiment 1.
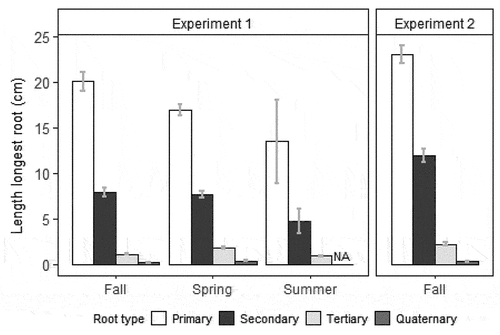
Figure 2. Percentage of fall and spring Salix spp. primary roots with secondary roots per cutting in different secondary root categories (vertical panels) in experiment 1 by IBA concentration (%; x axis) and soaking time (days; horizontal panels) with standard error. All cuttings are present in each secondary root category (vertical panel). Each bar is n = 6 (fall) and n = 9 (spring).
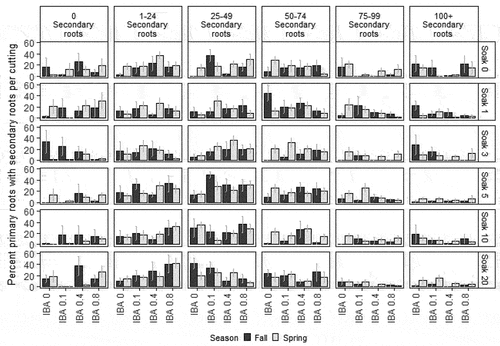
Figure 3. Percentage of fall Salix spp. primary roots with secondary roots per cutting in experiment 2 with secondary roots in different secondary root categories by treatment (x axis) with standard error. All cuttings are present in each root category. Each bar is n = 10 (all rooted cuttings are represented in each secondary root box).
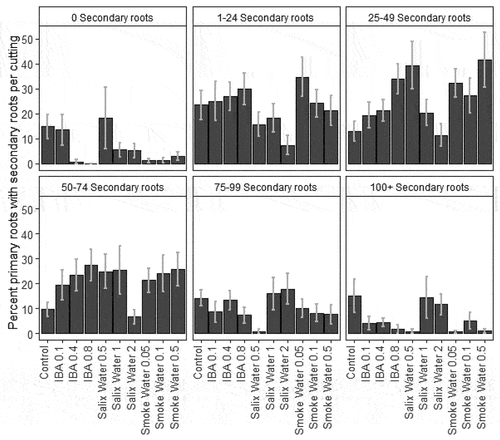
Formation of callus and new roots requires input of carbohydrates solubilized from local sources or transported from distal portions of the cutting. Stored carbohydrates provide energy to enable a cutting to survive until it develops new roots and building blocks for root formation (da Costa et al. Citation2013; Davies et al. Citation2017). Dormant cuttings are thought to have higher carbohydrate reserves, although concentrations are not consistently correlated with rooting for different species (Fege and Brown Citation1984; Haissig Citation1986; Davies et al. Citation2017; Tsafouros et al. Citation2019). Further research is required to determine factors affecting carbohydrate partitioning within a cutting at different times of year and in different species and how this influences adventitious and lateral root development and callus formation. Though summer is the best time for reclamation practitioners to work, our results indicate that collecting cuttings from dormant Salix species will likely lead to more successful revegetation because cuttings have much higher rooting percentages and more developed root system architecture.
Response to IBA And soaking
Fall and spring cuttings had sufficient adventitious rooting for treatment effects analysis; fall models generally had weaker treatment effects. Percentage of rooted cuttings in a dependent variable treatment group (IBA concentration or soaking time) in experiment 1 was seventy-one to ninety-two for fall cuttings and eighty-three to ninety-two for spring (Supplementary Figure 4). The null model had most weight in both seasons (), although for fall cuttings soaking had similar weighting to the null model, indicating that soaking may increase rooting percentages. In experiment 2, most fall cuttings rooted, regardless of treatment. Nine out of ten control cuttings, IBA 0.1 percent, and IBA 0.4 percent rooted, and ten out of ten IBA 0.8 percent rooted.
Endogenous auxin concentrations naturally vary in plants throughout the growing season, and in cuttings, an increase in endogenous auxin at the cut surface from polar auxin transport or application of different exogenous auxin concentrations stimulates adventitious rooting in many species (Nanda and Anand Citation1970; Wiesman, Riov, and Epstein Citation1988; Ludwig-Müller Citation2000; Štefančič, Štampar, and Osterc Citation2005). Application of exogenous IBA in different forms and concentrations has species-specific effects on numerous root parameters, including callusing, timing of root emergence, number of primary and secondary roots, root length, dry weight, and field survival rates (Sharma and Aier Citation1989; Abdel-Rahman Citation2020; Rehana et al. Citation2020). Because cuttings in our study were not treated for up to seven days after collection, rewounding the cuttings prior to treatment may have resulted in different adventitious root percentages in different seasons based on interactions with seasonal endogenous auxin concentrations (Nanda and Anand Citation1970; Howard Citation1971; Blakesley, Weston, and Elliott Citation1991). For instance, Nanda, Kumar, and Kochhar (Citation1974) found that Populus robusta Schneid. cuttings developed more roots when treated with IBA at day 0 than at day 7 or 14. However, Lodama et al. (Citation2016) found that delaying hormone application by one to two weeks improved rooting in Lobostemon fruticosus (L.) H. Buek (pajama bush). Follow-up research investigating endogenous auxin concentrations in Salix species from our study throughout the growing season can determine whether interactions between endogenous and exogenous growth hormones are influencing root development.
Cuttings with callus in our study produced eight more roots (56 percent) on average than those without, and roots were 8.7 cm (82.3%) longer (). Cuttings treated with IBA that callused developed 80.3 and 41.2 percent more roots in fall and spring, respectively, than untreated cuttings that callused, although roots were 19.2 and 13 percent shorter, respectively (). IBA increased number of roots and root length for cuttings without callus relative to untreated cuttings. Soaking increased the mean number of roots by 21.3 percent for spring cuttings that callused and decreased root length by 15.9 percent relative to unsoaked cuttings that callused. Soaking decreased number of roots and root length for spring cuttings without callus and all fall cuttings. In some species, such as Lobostemon fruticosus and Arabidopsis thaliana, root initials always developed from callus tissue (Ludwig-Müller, Vertocnik, and Town Citation2005; Lodama et al. Citation2016), but in Prunus GiSelA 5 (dwarf cherry), cuttings with callus had fewer and shorter roots than cuttings without callus (Štefančič, Štampar, and Osterc Citation2005). In two poplar species, the easy-to-root Populus × euramericana (Dode) Guinier cv. 1–78 developed roots from preformed root primordia and callus, whereas in the hard to root species, Populus tremula L., cuttings developed callus but not roots (Okoro and Grace Citation1976). Ludwig-Müller, Vertocnik, and Town (Citation2005) and Nanda, Kumar, and Kochhar (Citation1974) both found that timing and concentrations of different auxins influenced whether cuttings developed callus and then roots or just callus. Because callused Salix cuttings had more developed root system architecture in our study, assessing factors that influence callus formation could improve root initiation and development in future.
Table 2. Mean difference (Diff) in rooting and percentage change between IBA concentration (0.1, 0.4, 0.8 percent) and control (0 percent) and soaking length (one, three, five, ten, and twenty days) and control (zero days) for number of roots and root length (cm) for Salix cuttings in fall and spring in experiment 1
Figure 4. Number of roots (a) and length longest root (b) with standard error at day 60 for all Salix spp. cuttings in experiment 1 that had callus (solid line) and did not callus (dashed line) by IBA concentration (%; x axis), season (horizontal panels), and soaking time (days; vertical panels).
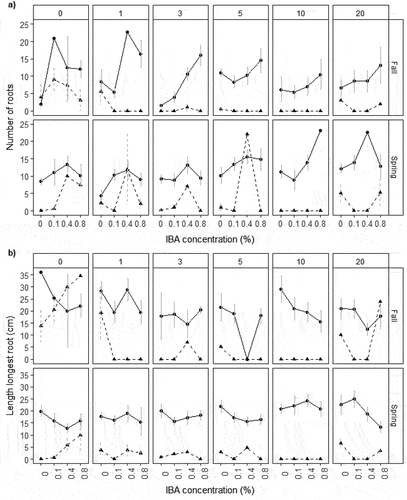
Treatment effects varied for number of roots and root length and between experiments for rooted cuttings. In experiment 1, the linear model with IBA concentration best represented number of roots on rooted fall cuttings; cuttings treated with IBA had 3.9 (63 percent) more roots on average than untreated cuttings (, ). The model with linear and quadratic terms for both IBA concentration and soaking length best represented spring cuttings. Number of roots increased by 22.3, 68.5, and 46.6 percent for 0.1, 0.4, and 0.8 percent IBA, respectively, for spring cuttings; decreased by 11.8 and 4.9 percent with shorter soaking (1, 3 days, respectively) than no soaking; and increased by 39.9, 48.3, and 36.5 percent with longer soaking (5, 10, 20 days, respectively; , Supplementary Table 3). Higher IBA concentrations in both seasons produced some cuttings with many roots (). For longest root length, the null model had most weight for fall cuttings, followed by a linear soaking term (, Supplementary Figure 5). Soaking generally decreased longest root length for fall cuttings by 3.3 cm (14.5 percent) relative to no soaking (, Supplementary Table 3). The model with linear concentration and soaking and quadratic concentration and soaking best represented root length for spring cuttings (, Supplementary Figure 5). Increasing IBA concentration decreased longest root length by up to 3.2 cm (17.4 percent), whereas increasing soaking time up to ten days increased longest root length by 7.1 cm (47.8 percent) relative to no soaking (, Supplementary Table 3). In experiment 2, IBA 0.4 and 0.8 percent had similar numbers of roots and root length as the control, while IBA 0.1 percent had fewer and shorter roots (, Supplementary Table 3, Supplementary Figure 5e). Number of roots had only a weak correlation with longest root length for cuttings in both experiments (Supplementary Figure 6).
Figure 5. Violin and jitter plots for number of roots in fall (a), (b), (e) and spring (c), (d) on rooted Salix spp. cuttings from experiment 1 for IBA concentration (%) (a), (c); soaking time (days) (b), (d); and from experiment 2 by treatment (e). Closed circles represent individual roots; open circles represent treatment means. Each closed circle in the jitter plot had a small value (between 0 and 0.2) added to the value on the x axis to visually separate points. Black lines for each violin plot use density curves to show data distribution, with wider areas having higher frequency of data points than narrower areas.
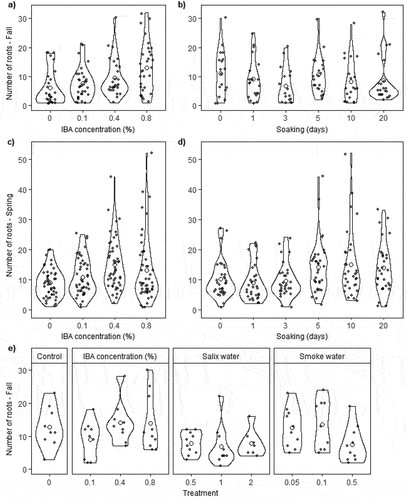
Root development and architecture is a complex interaction between internal and external factors that is still not well understood, particularly for how multiple environmental constraints affect root allocation and growth (Ho et al. Citation2005). For example, more roots with increasing IBA concentration and soaking time would create more opportunities to explore the substrate for resources. However, IBA concentration and soaking had opposite effects on root length for spring cuttings. For IBA concentrations similar to those in our study, Miller-Adamany, Gerber, and Thomsen (Citation2017) found no effects of application of 0.3 percent IBA on belowground biomass of Salix exigua Nutt. (coyote willow) cuttings, and Hoag and Short (Citation1992) found no improvement in rooting after applying 0.1 percent IBA to cuttings from ten willow species. In a subarctic study, Houle and Babeux (Citation1998) found time of year did not affect rooting of Salix planifolia cuttings treated with 0.1 percent IBA, although fall dormant cuttings produced more roots than August cuttings. Application of 0.01 and 0.1 percent IBA to late May cuttings had rooting similar to dormant cuttings in our study. Higher concentrations of IBA (1 percent), above the concentration used in our study, decreased rooted cuttings and number of roots, indicating a potential auxin threshold for Salix species (Houle and Babeux Citation1994; Lund, Smith, and Hackett Citation1996; Ricci et al. Citation2008).
For soaking duration, only one other study was found that specifically investigated effects of season (fall, spring) and soaking time (0, 14 days) on Salix cuttings (Salix amygdaloides Andersson [peach leaf willow] and Salix exigua cuttings; Tilley and Hoag Citation2009). Soaking did not influence rooting percentages but increased shoot and root biomass of fall cuttings. Ficko and Naeth (Citation2021) found species-specific responses to soaking and season for seven other shrub species from Diavik, but rooting percentages were low. In another study, soaking dormant Salix nigra cuttings for ten days doubled cutting survival and resulted in higher root, shoot, and leaf biomass than zero or three days of soaking, which was similar to the pattern for number of roots on spring cuttings in our study (Schaff, Pezeshki, and Shields Citation2002). Miller-Adamany, Gerber, and Thomsen (Citation2017) found that soaking and storing Salix exigua cuttings for seventeen days increased aboveground biomass, with no differences between soaked and unsoaked cuttings for belowground biomass; in a large field study, Martin, Pezeshki, and Shields (Citation2005) found that soaking Salix nigra cuttings for fourteen days significantly improved cutting survival over thirty-four weeks. Soaking was hypothesized to improve cutting water status, which enabled faster root development and better contact with soil even with low soil water conditions. Given that soaking did not strongly influence rooting, reclamation practitioners can likely leave Salix cuttings soaking for up to three weeks without significant adverse effects if logistical issues arise prior to planting. Further research is necessary to decipher the physiological mechanism underlying how soaking time impacts rooting and how this varies by season.
Endogenous IAA concentrations in the rooting zone are known to affect all stages of lateral root initiation, primordia development, and emergence (Bhalerao et al. Citation2002; De Smet et al. Citation2007). In our study, application of exogenous IBA had a variable effect on lateral root development by generally increasing number of cuttings with fewer than fifty secondary roots and decreasing number of cuttings with greater than seventy-five secondary roots, although this varied by season and experiment (, Supplementary Figure 7). Soaking time did not strongly influence number of secondary roots (Supplementary Figure 8). In Arabidopsis thaliana, lateral root formation is promoted by increasing exogenous auxin concentrations until a maximum after which further lateral root formation is decreased or inhibited (Péret et al. Citation2009; Ivanchenko, Napsucialy‐Mendivil, and Dubrovsky Citation2010). In Cucurbita pepo L. (pumpkin), application of exogenous auxins decreased primary root growth but had no effect on lateral root initiation (Ilina et al. Citation2018). IBA application in our study likely decreased the rate of parent root elongation and potentially exceeded the maximum concentration that decreased number of secondary roots. Given that root system architecture of cuttings is shaped by both adventitious and lateral root development, use of auxins must be carefully balanced to optimize both adventitious and lateral root development to cultivate plants with healthy and well-developed root systems suitable for reclamation.
Response to salix and smoke water extracts
In experiment 2, 100 percent of cuttings rooted for all three smoke water treatments and 0.5 and 1 Salix water extracts relative to 90 percent for control cuttings. However, the highest concentration of Salix water extract (2) only had 70 percent of cuttings root. Ficko and Naeth (Citation2021) found differences in rooting percentages between season and Salix water extract and season and smoke water extract for seven northern species also collected from Diavik, but rooting percentages were generally less than 25 percent. All concentrations of Salix water and the highest concentration of smoke water had fewer roots than the control, and only smoke water 0.1 had longer roots than the control. Smoke water extract and low concentrations of Salix water extract increased number of secondary roots between twenty-five and seventy-four relative to the control ().
In our study, Salix cuttings may not have responded strongly to Salix water extract if they already contained the same compounds (salicylic acid) as those in the extract or if the Salix water extracts were not optimal concentrations to stimulate adventitious rooting. Recent research has determined that karrikins, found in smoke water extract, use similar signaling pathways as strigolactones, a newly discovered class of plant hormone. Karrikins and strigolactones inhibited adventitious and lateral root formation in Arabidopsis thaliana, although low phosphorus conditions or high auxin concentrations overrode the inhibitory effect on lateral root formation (Kapulnik et al. Citation2011; Ruyter-Spira et al. Citation2011; Rasmussen et al. Citation2012). Given that there were differences in response between adventitious and lateral root development in our study, understanding how compounds in these extracts affect different pathways in the plant may improve revegetation efforts in the future by selecting treatments that optimize root system architecture for the local soil conditions of the reclamation site.
Conclusions
For successful northern revegetation, cuttings must develop an appropriate root system architecture that can respond to harsh and changing environmental conditions. Given the necessity of developing roots for cuttings to grow, most research to date with arctic cuttings has focused primarily on the effect of different treatments on percentage rooting and sometimes number of adventitious roots, without assessing how treatments also affect other root parameters such as lateral root development or which parameters influence success after planting in the field. In our study, Salix spp. cuttings developed extensive root system architecture within sixty days, particularly for dormant cuttings, with some cuttings developing up to six orders of roots. For primary root development, IBA and soaking influenced root number and root length in spring but only had a weak effect for fall cuttings, indicating seasonal influences of treatments. Because soaking length did not generally have a large effect on root development, Salix cuttings may be soaked for up to three weeks if logistical factors delay planting. However, we observed differences in response to treatment between primary and secondary root behavior, which indicates that reclamation practitioners must select treatments that optimize root system architecture for the local environmental conditions to maximize revegetation success at disturbed northern sites.
Supplemental Material
Download Zip (546.7 KB)Acknowledgments
We gratefully acknowledge Dr. Peter Blenis and Dr. Sasha van der Klein for statistical analysis support and the many people who assisted with the various aspects of this research.
Disclosure statement
No potential conflict of interest was reported by the author(s).
Supplementary material
Supplemental material for this article can be accessed on the publisher’s website.
Additional information
Funding
References
- Abdel-Rahman, S. 2020. Influence of rooting media and indole-3-butyric-acid (IBA) concentration on rooting and growth of different types of Conocarpus erectus L. stem cuttings. Scientific Journal of Flowers and Ornamental Plants 7:199–219. doi:10.21608/sjfop.2020.109363.
- Akeel, A., M. M. A. Khan, H. Jaleel, and M. Uddin. 2019. Smoke-saturated water and karrikinolide modulate germination, growth, photosynthesis and nutritional values of carrot (Daucus carota L.). Journal of Plant Growth Regulation 38:1387–401. doi:10.1007/s00344-019-09941-w.
- Al-Amad, I., and M. Qrunfleh. 2016. Effect of Babylon weeping willow (Salix babylonica L.) extracts on rooting of stem cuttings of olive (Olea europaea L.) ‘Nabali.’ Acta Horticulturae 391–96. doi:10.17660/ActaHortic.2016.1130.58.
- Anderson, D. R., editor. 2008. Quantifying the evidence about science hypotheses. In The life sciences: A primer on evidence, pp. 83–104. New York, NY: Springer.
- Atkinson, J. A., A. Rasmussen, R. Traini, U. Voß, C. Sturrock, S. J. Mooney, D. M. Wells, and M. J. Bennett. 2014. Branching out in roots: Uncovering form, function, and regulation. Plant Physiology 166:538–50. doi:10.1104/pp.114.245423.
- Austin, A. T., and A. E. Zanne. 2015. Whether in life or in death: Fresh perspectives on how plants affect biogeochemical cycling. Journal of Ecology 103:1367–71. doi:10.1111/1365-2745.12486.
- Bardgett, R. D., L. Mommer, and F. T. De Vries. 2014. Going underground: Root traits as drivers of ecosystem processes. Trends in Ecology & Evolution 29:692–99. doi:10.1093/jxb/eri208.
- Bellini, C., D. I. Pacurar, and I. Perrone. 2014. Adventitious roots and lateral roots: Similarities and differences. Annual Review of Plant Biology 65:639–66. doi:10.1146/annurev-arplant-050213-035645.
- Bhalerao, R. P., J. Eklöf, K. Ljung, A. Marchant, M. Bennett, and G. Sandberg. 2002. Shoot-derived auxin is essential for early lateral root emergence in Arabidopsis seedlings. The Plant Journal 29:325–32. doi:10.1046/j.0960-7412.2001.01217.x.
- Blakesley, D., G. D. Weston, and M. C. Elliott. 1991. Endogeneous levels of indole-3-acetic acid and abscisic acid during the rooting of Cotinus coggygria cuttings taken at different times of the year. Plant Growth Regulation 10:1–12. doi:10.1007/BF00035126.
- Chapin, F. S., D. A. Johnson, and J. D. McKendrick. 1980. Seasonal movement of nutrients in plants of differing growth form in an Alaskan tundra ecosystem: Implications for herbivory. Journal of Ecology 68:189–209. doi:10.2307/2259251.
- Chen, Y., Y. Xie, C. Song, L. Zheng, X. Rong, L. Jia, L. Luo, C. Zhang, X. Qu, and W. Xuan. 2018. A comparison of lateral root patterning among dicot and monocot plants. Plant Science 274:201–11. doi:10.1016/j.plantsci.2018.05.018.
- Chiwocha, S. D. S., K. W. Dixon, G. R. Flematti, E. L. Ghisalberti, D. J. Merritt, D. C. Nelson, J.-A. M. Riseborough, S. M. Smith, and J. C. Stevens. 2009. Karrikins: A new family of plant growth regulators in smoke. Plant Science 177:252–56. doi:10.1016/j.plantsci.2009.06.007.
- Claridge, F. B., and A. M. Mirza. 1981. Erosion control along transportation routes in northern climates. Arctic 34:147–57. doi:10.14430/arctic2516.
- da Costa, C. T., M. R. de Almeida, C. M. Ruedell, J. Schwambach, F. D. S. Maraschin, and A. G. Fett-Neto. 2013. When stress and development go hand in hand: Main hormonal controls of adventitious rooting in cuttings. Frontiers in Plant Science 4. doi:10.3389/fpls.2013.00133.
- Davies, F., Jr, R. Geneve, S. Wilson, H. Hartmann, and D. Kester. 2017. Hartmann & Kester’s plant propagation: Principles and practices. 9th ed. New York, NY: Pearson.
- De Smet, I., T. Tetsumura, B. De Rybel, N. F. Dit Frey, L. Laplaze, I. Casimiro, R. Swarup, M. Naudts, S. Vanneste, D. Audenaert, et al. 2007. Auxin-dependent regulation of lateral root positioning in the basal meristem of Arabidopsis. Development 134:681–90. doi:10.1242/dev.02753.
- Deniau, M. G., R. Bonafos, M. Chovelon, C.-E. Parvaud, A. Furet, C. Bertrand, and P. A. Marchand. 2019. Willow extract (Salix cortex), a basic substance of agronomical interests. International Journal of Bio-Resource & Stress Management 10:408–18. doi:10.23910/IJBSM/2019.10.4.2009.
- Densmore, R. V. 1992. Succession on an Alaskan tundra disturbance with and without assisted revegetation with grass. Arctic and Alpine Research 24:238–43. doi:10.2307/1551663.
- Densmore, R. V., M. E. Vander Meer, and N. G. Dunkle. 2000. Native plant revegetation manual for Denali National Park and Preserve. Anchorage, Alaska: US Geological Survey, Biological Resources Division.
- Fege, A. S., and G. N. Brown. 1984. Carbohydrate distribution in dormant Populus shoots and hardwood cuttings. Forest Science 30:999–1010.
- Ficko, S. A., and M. A. Naeth. 2021. Root development on cuttings of seven Arctic shrub species for revegetation. Arctic, Antarctic, and Alpine Research 53:237–51. doi:10.1080/15230430.2021.1976711.
- Ficko, S. A., B. Smith, and B. Zeeb. 2015. Assisted revegetation following contaminated site remediation in the Arctic: Four-year case study of a former radar site. American Journal of Plant Sciences 6:1301–12. doi:10.4236/ajps.2015.68130.
- Forbes, B. C., and R. L. Jefferies. 1999. Revegetation of disturbed Arctic sites: Constraints and applications. Biological Conservation 88:15–24. doi:10.1016/S0006-3207(98)00095-0.
- Fromm, H. 2019. Root plasticity in the pursuit of water. Plants 8:236. doi:10.3390/plants8070236.
- Hagen, D. 2002. Propagation of native Arctic and alpine species with a restoration potential. Polar Research 21:37–47. doi:10.1111/j.1751-8369.2002.tb00065.x.
- Haissig, B. E. 1974. Origins of adventitious roots. New Zealand Journal of Forestry Science 4:299–310.
- Haissig, B. E. 1986. Metabolic processes in adventitious rooting in cuttings. In New root formation in plants and cuttings, ed. M. B. Jackson, 141–89. Dordrecht: Martinus Nijhoff Publishers.
- Ho, M. D., J. C. Rosas, K. M. Brown, J. P. Lynch, M. D. Ho, J. C. Rosas, K. M. Brown, and J. P. Lynch. 2005. Root architectural tradeoffs for water and phosphorus acquisition. Functional Plant Biology 32:737–48. doi:10.1071/FP05043.
- Hoag, J. C., and H. Short. 1992. Use of willow and cottonwood cuttings for vegetating shorelines and riparian areas. Aberdeen, ID: USDA-NRCS Interagency Riparian/Wetland Plant Development Project. Page 15.
- Holloway, P. S., and M. R. Peterburs. 2009. Propagation of twelve Alaska native plants by summer stem cuttings. Journal of Environmental Horticulture 27:207–10. doi:10.24266/0738-2898-27.4.207.
- Houle, G., and P. Babeux. 1993. Temporal variations in the rooting ability of cuttings of Populus balsamifera and Salix planifolia from natural clones-populations of subarctic Quebec. Canadian Journal of Forest Research 23:2603–08. doi:10.1139/x93-323.
- Houle, G., and P. Babeux. 1994. Variations in rooting ability of cuttings and in seed characteristics of five populations of Juniperus communis var. depressa from subarctic Quebec. Canadian Journal of Botany 72:493–98. doi:10.1139/b94-066.
- Houle, G., and P. Babeux. 1998. The effects of collection date, IBA, plant gender, nutrient availability, and rooting volume on adventitious root and lateral shoot formation by Salix planifolia stem cuttings from the Ungava Bay area (Quebec, Canada). Canadian Journal of Botany 76:1687–92. doi:10.1139/b98-177.
- Howard, B. H. 1971. Nursery experiment report: The response of cuttings to basal wounding in relation to time of auxin treatment. Plant Propagator 21:267–74.
- Ilina, E. L., A. S. Kiryushkin, V. A. Semenova, N. P. Demchenko, K. Pawlowski, and K. N. Demchenko. 2018. Lateral root initiation and formation within the parental root meristem of Cucurbita pepo: Is auxin a key player? Annals of Botany 122:873–88. doi:10.1093/aob/mcy052.
- Ivanchenko, M. G., S. Napsucialy‐Mendivil, and J. G. Dubrovsky. 2010. Auxin-induced inhibition of lateral root initiation contributes to root system shaping in Arabidopsis thaliana. The Plant Journal 64:740–52. doi:10.1111/j.1365-313X.2010.04365.x.
- Iversen, C. M., V. L. Sloan, P. F. Sullivan, E. S. Euskirchen, A. D. McGuire, R. J. Norby, A. P. Walker, J. M. Warren, and S. D. Wullschleger. 2015. The unseen iceberg: Plant roots in Arctic tundra. New Phytologist 205:34–58. doi:10.1111/nph.13003.
- Javed, B., K. Nawaz, and M. Munazir. 2020. Phytochemical analysis and antibacterial activity of tannins extracted from Salix alba L. against different gram-positive and gram-negative bacterial strains. Iranian Journal of Science and Technology, Transactions A: Science 44:1303–14. doi:10.1007/s40995-020-00937-w.
- Jung, J. K. H. M., and S. R. M. McCouch. 2013. Getting to the roots of it: Genetic and hormonal control of root architecture. Frontiers in Plant Science 4. doi:10.3389/fpls.2013.00186.
- Kapulnik, Y., P.-M. Delaux, N. Resnick, E. Mayzlish-Gati, S. Wininger, C. Bhattacharya, N. Séjalon-Delmas, J.-P. Combier, G. Bécard, E. Belausov, et al. 2011. Strigolactones affect lateral root formation and root-hair elongation in Arabidopsis. Planta 233:209–16. doi:10.1007/s00425-010-1310-y.
- Koriesh, E. M., Y. M. Abd El-Fattah, I. H. Abo El-Soud, and M. F. Khalil. 2018. Effects of different nutrient solution formulations supplemented with willow bark or juvenile branches decoction on growth of Coleus plants. Hortscience Journal of Suez Canal University 7:11–17. doi:10.21608/hjsc.2018.58328.
- Krasny, M. E., J. C. Zasada, and K. A. Vogt. 1988. Adventitious rooting of four Salicaceae species in response to a flooding event. Canadian Journal of Botany 66:2597–98. doi:10.1139/b88-352.
- Kutschera, L., and E. Lichtenegger. 2002. Wurzelatlas mitteleuropäischer Waldbäume und Sträucher. Graz - Stuttgart, Austria: Leopold Stocker Verlag.
- Laplaze, L., E. Benkova, I. Casimiro, L. Maes, S. Vanneste, R. Swarup, D. Weijers, V. Calvo, B. Parizot, M. B. Herrera-Rodriguez, et al. 2007. Cytokinins act directly on lateral root founder cells to inhibit root initiation. The Plant Cell 19:3889–900. doi:10.1105/tpc.107.055863.
- Lecompte, F., and L. Pagès. 2007. Apical diameter and branching density affect lateral root elongation rates in banana. Environmental and Experimental Botany 59:243–51. doi:10.1016/j.envexpbot.2006.01.002.
- Lodama, K. E., E. S. du Toit, J. M. Steyn, H. T. Araya, G. Prinsloo, C. P. du Plooy, and P. J. Robbertse. 2016. Improving rooting of Lobostemon fruticosus L. cuttings with delayed auxin treatment. South African Journal of Botany 105:111–15.
- Ludwig-Müller, J. 2000. Indole-3-butyric acid in plant growth and development. Plant Growth Regulation 32:219–30. doi:10.1023/A:1010746806891.
- Ludwig-Müller, J., A. Vertocnik, and C. D. Town. 2005. Analysis of indole-3-butyric acid-induced adventitious root formation on Arabidopsis stem segments. Journal of Experimental Botany 56:2095–105.
- Lund, S. T., A. G. Smith, and W. P. Hackett. 1996. Cuttings of a tobacco mutant, rae, undergo cell divisions but do not initiate adventitious roots in response to exogenous auxin. Physiologia Plantarum 97:372–80. doi:10.1034/j.1399-3054.1996.970223.x.
- Malamy, J. E. 2005. Intrinsic and environmental response pathways that regulate root system architecture. Plant, Cell & Environment 28:67–77. doi:10.1111/j.1365-3040.2005.01306.x.
- Malamy, J. E., and P. N. Benfey. 1997. Organization and cell differentiation in lateral roots of Arabidopsis thaliana. Development 124:33–44. doi:10.1242/dev.124.1.33.
- Martin, L. T., S. R. Pezeshki, and F. D. Shields. 2005. Soaking treatment increases survival of black willow posts in a large-scale field study. Ecological Restoration 23:95–98. doi:10.3368/er.23.2.95.
- Matheus, P., and T. Omtzigt. 2011. Yukon revegetation manual: Practical approaches and methods. Whitehorse, YT: Mining and Petroleum Environmental Research Group.
- Miller-Adamany, A. A., D. T. Gerber, and M. Thomsen. 2017. Maximizing stake performance: Storage, soaking, scoring, and auxin as pre-planting techniques for sandbar willow (Salix exigua) stakes. Natural Areas Journal 37:412–18. doi:10.3375/043.037.0315.
- Morris, E. C., M. Griffiths, A. Golebiowska, S. Mairhofer, J. Burr-Hersey, T. Goh, D. von Wangenheim, B. Atkinson, C. J. Sturrock, J. P. Lynch, et al. 2017. Shaping 3D root system architecture. Current Biology 27:R919–R930. doi:10.1016/j.cub.2017.06.043.
- Naeth, M. A., and S. R. Wilkinson. 2011. Reclamation at the Diavik Diamond Mine in the Northwest Territories: Substrates, soil amendments and native plant community development. In Phase II final report, 1–31. Edmonton: University of Alberta.
- Nanda, K. K., and V. K. Anand. 1970. Seasonal changes in auxin effects on rooting of stem cuttings of Populus nigra and its relationship with mobilization of starch. Physiologia Plantarum 23:99–107. doi:10.1111/j.1399-3054.1970.tb06396.x.
- Nanda, K. K., P. Kumar, and V. K. Kochhar. 1974. Role of auxins, antiauxin and phenol in the production and differentiation of callus on stem cuttings of Populus robusta. New Zealand Journal of Forestry Science 4:338–46.
- Nibau, C., D. J. Gibbs, and J. C. Coates. 2008. Branching out in new directions: The control of root architecture by lateral root formation. New Phytologist 179:595–614. doi:10.1111/j.1469-8137.2008.02472.x.
- Nicotra, A. B., O. K. Atkin, S. P. Bonser, A. M. Davidson, E. J. Finnegan, U. Mathesius, P. Poot, M. D. Purugganan, C. L. Richards, F. Valladares, et al. 2010. Plant phenotypic plasticity in a changing climate. Trends in Plant Science 15:684–92. doi:10.1016/j.tplants.2010.09.008.
- Okoro, O. O., and J. Grace. 1976. The physiology of rooting Populus cuttings. Physiologia Plantarum 36:133–38. doi:10.1111/j.1399-3054.1976.tb03923.x.
- Olatunji, D., D. Geelen, and I. Verstraeten. 2017. Control of endogenous auxin levels in plant root development. International Journal of Molecular Sciences 18:2587. doi:10.3390/ijms18122587.
- Overvoorde, P., H. Fukaki, and T. Beeckman. 2010. Auxin control of root development. Cold Spring Harbor Perspectives in Biology 2:a001537. doi:10.1101/cshperspect.a001537.
- Pendall, E., S. Bridgham, P. J. Hanson, B. Hungate, D. W. Kicklighter, D. W. Johnson, B. E. Law, Y. Luo, J. P. Megonigal, M. Olsrud, et al. 2004. Below-ground process responses to elevated CO2 and temperature: A discussion of observations, measurement methods, and models. New Phytologist 162:311–22. doi:10.1111/j.1469-8137.2004.01053.x.
- Péret, B., B. De Rybel, I. Casimiro, E. Benková, R. Swarup, L. Laplaze, T. Beeckman, and M. J. Bennett. 2009. Arabidopsis lateral root development: An emerging story. Trends in Plant Science 14:399–408. doi:10.1016/j.tplants.2009.05.002.
- Poorter, H., K. J. Niklas, P. B. Reich, J. Oleksyn, P. Poot, and L. Mommer. 2012. Biomass allocation to leaves, stems and roots: Meta‐analyses of interspecific variation and environmental control. New Phytologist 193:30–50. doi:10.1111/j.1469-8137.2011.03952.x.
- Pop, T. I., D. Pamfil, and C. Bellini. 2011. Auxin control in the formation of adventitious roots. Notulae Botanicae Horti Agrobotanici Cluj-Napoca 39:307–16. doi:10.15835/nbha3916101.
- Postma, J. A., A. Dathe, and J. P. Lynch. 2014. The optimal lateral root branching density for maize depends on nitrogen and phosphorus availability. Plant Physiology 166:590–602. doi:10.1104/pp.113.233916.
- Rasmussen, A., M. G. Mason, C. D. Cuyper, P. B. Brewer, S. Herold, J. Agusti, D. Geelen, T. Greb, S. Goormachtig, T. Beeckman, et al. 2012. Strigolactones suppress adventitious rooting in Arabidopsis and pea. Plant Physiology 158:1976–87. doi:10.1104/pp.111.187104.
- Rehana, S., M. Madhavi, A. D. Rao, and P. Subbaramamma. 2020. Effect of cutting types and IBA treatments on success of vegetative propagation in crossandra (Crossandra infundibuliformis L.) var. Arka shravya. Journal of Pharmacognosy and Phytochemistry 9:1469–75.
- Ricci, A., E. Rolli, L. Dramis, and C. Diaz-Sala. 2008. N,N′-bis-(2,3-methylenedioxyphenyl)urea and N,N′-bis-(3,4-methylenedioxyphenyl)urea enhance adventitious rooting in Pinus radiata and affect expression of genes induced during adventitious rooting in the presence of exogenous auxin. Plant Science 175:356–63. doi:10.1016/j.plantsci.2008.05.009.
- Robbins, N. E., II, and J. R. Dinneny. 2015. The divining root: Moisture-driven responses of roots at the micro- and macro-scale. Journal of Experimental Botany 66:2145–54. doi:10.1093/jxb/eru496.
- Ruyter-Spira, C., W. Kohlen, T. Charnikhova, A. van Zeijl, L. van Bezouwen, N. de Ruijter, C. Cardoso, J. A. Lopez-Raez, R. Matusova, R. Bours, et al. 2011. Physiological effects of the synthetic strigolactone analog GR24 on root system architecture in Arabidopsis: Another belowground role for strigolactones? Plant Physiology 155:721–34. doi:10.1104/pp.110.166645.
- Sati, S. C., M. D. Sati, and H. Singh. 2011. Screening of insecticidal activity of Salix babylonica roots against mustard aphid. Universities’ Journal of Phytochemistry and Ayurvedic Heights 2:28–31.
- Schaff, S. D., S. R. Pezeshki, and F. D. Shields. 2002. Effects of pre-planting soaking on growth and survival of black willow cuttings. Restoration Ecology 10:267–74. doi:10.1046/j.1526-100X.2002.02035.x.
- Sharma, S. D., and N. B. Aier. 1989. Seasonal rooting behaviour of cuttings of plum cultivars as influenced by IBA treatments. Scientia Horticulturae 40:297–303. doi:10.1016/0304-4238(89)90103-9.
- Singh, H., R. Raturi, and P. P. Badoni. 2017. Isolation of secondary metabolites from the roots of Salix babylonica. IOP Conference Series: Materials Science and Engineering 225:012094. doi:10.1088/1757-899X/225/1/012094.
- Štefančič, M., F. Štampar, and G. Osterc. 2005. Influence of IAA and IBA on root development and quality of Prunus “GiSelA 5” leafy cuttings. HortScience 40:2052–55. doi:10.21273/HORTSCI.40.7.2052.
- Steffens, B. and A. Rasmussen. 2016. The physiology of adventitious roots. Plant Physiology 170:603–617. doi: 10.1104/pp.15.01360.
- Swarbreck, S. M. 2021. Phytohormones interplay: Karrikin signalling promotes ethylene synthesis to modulate roots. Trends in Plant Science 26:308–11. doi:10.1016/j.tplants.2021.02.004.
- Tilley, D. J., and J. C. Hoag. 2009. Evaluation of fall versus spring dormant planting of hardwood willow cuttings with and without soaking treatment. Native Plants Journal 10:288–94.
- Tsafouros, A., A. Frantzeskaki, A. Assimakopoulou, and P. A. Roussos. 2019. Spatial and temporal changes of mineral nutrients and carbohydrates in cuttings of four stone fruit rootstocks and their contribution to rooting potential. Scientia Horticulturae 253:227–40. doi:10.1016/j.scienta.2019.04.049.
- Walter, J., D. Hughes, N. J. Moore, and G. Muhlberg. 2005. Streambank revegetation and protection: A guide for Alaska. Juneau: Alaska Department of Fish and Game.
- Wickham, H. 2016. ggplot2: Elegant graphics for data analysis. New York: Springer-Verlag.
- Wiesman, Z., J. Riov, and E. Epstein. 1988. Comparison of movement and metabolism of indole-3-acetic acid and indole-3-butyric acid in mung bean cuttings. Physiologia Plantarum 74:556–60. doi:10.1111/j.1399-3054.1988.tb02018.x.
- Wise, K., H. Gill, and J. Selby-Pham. 2020. Willow bark extract and the biostimulant complex Root Nectar® increase propagation efficiency in chrysanthemum and lavender cuttings. Scientia Horticulturae 263:109108. doi:10.1016/j.scienta.2019.109108.
- Wright, S. J. 2008. A revegetation manual for Alaska. Palmer: Alaska Plant Materials Center, Division of Agriculture, Alaska Department of Natural Resources.
- Wu, Q., L. Pagès, and J. Wu. 2016. Relationships between root diameter, root length and root branching along lateral roots in adult, field-grown maize. Annals of Botany 117:379–90. doi:10.1093/aob/mcv185.
- Younkin, W. E., and H. E. Martens. 1987. Long-term success of seeded species and their influence on native species invasion at abandoned rig site A-01 Caribou Hills, N.W.T., Canada. Arctic and Alpine Research 19:566–71. doi:10.2307/1551426.