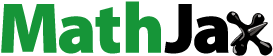
ABSTRACT
Warming in mountain regions is projected to occur three times faster than the global average. Recently, a small number of observational studies have reported species loss in mountain plant communities and have explored mechanisms facilitating the colonization by novel species. We monitored translocated mountain plant communities and their novel competitor interactions following five years of exposure to downslope climates. We found increasing species turnover under two future climate scenarios with time. Local loss of native species was followed by the colonization by novel species after a severe drought year in the third year after translocation. Here, the assumed mechanism facilitating novel species colonization in mountain communities is direct environmental filtering. We find a time lag between the local loss of native species and the colonization by novel species, which can be explained by interacting climate stressors—that is, warming and drought—pushing communities across a threshold of resistance to colonization. Interestingly, despite significant changes in species identity and strong colonization by novel species, the proportional contribution of plant functional groups to aboveground biomass stayed stable across both sites of origin and over time. Our study provides experimental evidence of local species loss in mountain plant communities prone to severe climatic change, revealing abrupt threshold dynamics.
Introduction
The climate of mountain regions is projected to warm three times faster than the global average, particularly in winter (Pepin et al. Citation2015; Körner Citation2021). Climate warming is driving shifts in species’ distributions, with native species tracking their optimal climate to higher latitudes (Pauchard et al. Citation2016), in the special case of mountain regions to higher elevations (M. J. Steinbauer et al. Citation2018) or nearby refugial microhabitats (Scherrer and Körner Citation2011; Körner and Hiltbrunner Citation2021). Few studies have reported species replacement in real-world mountain plant communities by novel species from lower elevations (Kudo et al. Citation2011; Rixen and Wipf Citation2017). In addition to the increase in species richness in mountain plant communities (M. J. Steinbauer et al. Citation2018), the thermophilization of species (Lamprecht et al. Citation2018; K. Steinbauer et al. Citation2020) and a reduction in range size and abundance of cold-adapted species (Rumpf et al. Citation2018) were found in observational studies. These observations are an early warning signal and raise the question: Why have mountain plant communities experienced such a high inertia so far? Or, in other words, why are mountain plant communities creating a so-called extinction debt? Extinction debt is defined as extinctions that are expected to occur as consequence of climate change that have not yet occurred (see Tilman et al. Citation1994; Figueiredo et al. Citation2019). Current theories explaining low extinction rates in mountain regions include (1) extinction debt driven by the long life span of mountain species (demographic inertia; Dullinger et al. Citation2012), (2) time lags between warming and novel biotic interactions (Alexander et al. Citation2018; Nomoto and Alexander Citation2021), and (3) missing novel competitors due to topography-driven isolation (M. J. Steinbauer et al. Citation2016). We theorize an additional mechanism: though communities have some resistance to warming per se, warming reduces resilience to subsequent abiotic stressors. Events such as drought can launch the “payment” of the extinction debt and create windows of opportunity (Diez et al. Citation2012) that lead to colonization by novel species. Translocation experiments, like the one presented in this study, offer a great opportunity to simulate future climate scenarios in combination with removal of dispersal barriers for novel lowland species.
In addition to temperature (see Körner and Hiltbrunner Citation2018), growing season precipitation and, more important, the precipitation regime (temporal distribution of precipitation) are benchmarks for studying mountain plant communities’ vulnerability to climate change (Müller and Bahn Citation2022). Though extreme weather events such as heat waves and droughts are rarely observed in alpine ecosystems, they will likely become more frequent at mid-elevations, the montane belt, and the subalpine belt (Spinoni et al. Citation2018; Intergovernmental Panel on Climate Change Citation2021). Not only are these elevational belts the most vulnerable from a climate change perspective (Stephan et al. Citation2021) but they are simultaneously the trailing range edges of many alpine plant species (Rixen et al. Citation2022). The impact of climatic warming interacting with drought on plant communities is likely to elicit nonadditive and nonlinear responses that are difficult to predict (Scheffer and Carpenter Citation2003; Buma Citation2015; Turner et al. Citation2020), especially in mountain environments that are underrepresented in the literature (but see Berauer et al. Citation2019). In a translocation experiment of mountain plant communities, De Boeck et al. (Citation2016) found that heat waves in combination with drought had a strong effect on aboveground productivity, whereas heat waves alone had no significant impact. Their follow-up study exploring legacy and recovery effects (De Boeck et al. Citation2018) demonstrated that the combined effect of warming and drought led to a forb-depressed state dominated by graminoid species (see also Rosbakh et al. Citation2017; Collins et al. Citation2022). Exploring such nonadditive processes is particularly important on a plant community level because species track climatic changes at different rates (Gibson‐Reinemer, Sheldon, and Rahel Citation2015; Mamantov et al. Citation2021; Rixen et al. Citation2022), leading to species turnover due to the interactions with novel species or changing outcomes of interactions among existing species over time (Alexander, Diez, and Levine Citation2015).
Therefore, investigating the variation in number, identity, and abundance of species through time plays a key role for community dynamics, which is often analyzed in light of community stability (Hector and Bagchi Citation2007; Allan et al. Citation2011; Valencia et al. Citation2020). We use two simple metrics to define turnover: species gains and losses and changes in relative abundances of single species. From a species turnover perspective, three important aspect should be considered. First, though species turnover may remain at high rates only explaining that a particular plant community is changing, the two underlying drivers (species gains and losses) may be opposing and reflect a different ecological story: colonization and local species loss (Thompson and Gonzalez Citation2017). Detecting specific time points when species appear versus when species disappear from a community reveals mechanisms underlying community reassembly and the trajectory of community structure. Second, relative abundances between species and plant functional groups (PFGs) can determine community functioning. Some studies have shown that particular PFGs tend to draw more benefits from climate change than others, consequently changing proportional PFG contributions to aboveground biomass (AGB) upon warming and drought (J. S. Hallett et al. Citation2014; Hoover, Knapp, and Smith Citation2014; Stampfli et al. Citation2018; Wilfahrt et al. Citation2021). In particular, legumes have been identified to be vulnerable to drought (Grant et al. Citation2014) likely due to their tight soil biodiversity interaction (Prudent et al. Citation2020). Ma et al. (Citation2017) found that less abundant species had little effect on community biomass stability and concluded that species diversity was an unimportant driver of community biomass stability. Yet, this conclusion ignores that changed community structure affects plant–plant interactions (Ploughe et al. Citation2019) and is likely controlling ecosystem functioning and related ecosystem services, such as water retention, including feedback loops to plant available water, feed value, slope stability, or plant–pollinator mutualisms (Schmidt et al. Citation2016; Box et al. Citation2019). More important, key measures such as aboveground primary production may remain stable under climate warming in species-rich plant communities due to compensatory effects as a result of functional redundancy (Isbell et al. Citation2015; Valencia et al. Citation2020) where species identity and abundance are crucial. Compensatory shifts in PFGs in proportion to AGB after disturbances remain underexplored (McLaren and Turkington Citation2010; Jentsch and White Citation2019). Also, knowing whether colonizing species are native or novel to a particular plant community is fundamental for biological diversity and its conservation (Alexander, Diez, and Levine Citation2015; Vetter et al. Citation2018). Thus, understanding the mechanisms behind community assembly and its implication for the stability of plant communities as well as single PFGs and a species resident status (native vs novel) is crucial to understanding the effects of climate change and its consequences for future ecosystem functioning.
There is a growing body of literature acknowledging the importance of both native species persistence and resistance to novel species under climate warming to protect ecosystem resilience and functioning (Crepaz et al. Citation2021; Usinowicz and Levine Citation2021). It has been shown that reproductive output of mountain plant species is decreasing under warmer and drier conditions (Bektaş et al. Citation2021; Schuchardt et al. Citation2021), thus changing population demography (Körner Citation2021). Moreover, seedling emergence and, even more important, seedling establishment of mountain plant species are likely to be diminished under warmer and drier conditions (Meineri et al. Citation2020; Stampfli and Zeiter Citation2020; Margreiter, Walde, and Erschbamer Citation2021), especially if highly competitive novel species are present to colonize open space (Alexander and Levine Citation2019; Vetter et al. Citation2019). The lack of competitive traits in mountain plant species against novel biotic interactions is likely increasing mountain plant communities’ vulnerability at the trailing edge under climate change.
Here, we experimentally translocated intact mountain plant–soil communities from a semi-natural subalpine grassland and a natural alpine pasture within the European Alps downslope to a warmer and drier environment. Furthermore, the downslope translocation removed topography-driven isolation of the respective mountain plant communities and thus dispersal barriers for novel lowland species. We passively simulated warming for two different sites of origin and monitored species-specific losses of mountain species, species-specific gains of species from the local lowland communities, as well as biomass responses over five consecutive years. By pairing an extreme climatic gradient (+3.3 K and +4.4 Kaverage growing season air temperature; see ) with a natural drought event that took place in central Europe in 2018 (year 3 of experimental exposure; Bastos et al. Citation2020), we pushed mountain plant communities beyond their typical climatic envelopes. We hypothesized that (1) relative to reference dynamics in control communities, rates of species turnover over time increase under experimental warming and drier conditions; (2) species turnover in warmed communities is initially driven by species loss, followed by the colonization by novel species in subsequent years; and (3) the relative contributions of PFGs to community aboveground biomass change postcolonization (novel species belong to different PFGs as lost natives).
Table 1. Geographic and climatic characteristics of study sites from low to high elevation.
Material and methods
Experimental setup
In the spring of 2016, thirty-six intact plant–soil mesocosms (hereafter: communities) were extracted from two experimental sites. Communities from high-elevation site Furka (2,440 m.a.s.l; Switzerland) represent alpine pasture vegetation (Hefel and Stöcklin Citation2010), whereas communities from the mid-elevation site Stubai (1850 m.a.s.l.; Austria) belong to an lightly managed, semi-natural subalpine grassland (Bahn et al. Citation2009; Ingrisch et al. Citation2018). The translocated alpine pasture communities were classified as Caricetum curvulae and the subalpine grassland communities as Trisetetum flavescentis; for detailed information on dominant and characteristic species, see Berauer et al. (Citation2019).
At the high- and mid-elevation sites, one set of communities (n = 9) was reburied as climatic control, and another set of communities (n = 9) was translocated downslope to Bayreuth (350 m.a.s.l.; Germany). The plant–soil communities were 30 cm in diameter and 25 cm in depth, representing an area shown to be sufficient to study community responses and interactions in small-statured grasslands (Milbau et al. Citation2007) while maintaining belowground processes. In this study, we tracked mountain plant community dynamics annually between 2016 and 2020 (five years of novel climate exposure).
Climatic parameters
In spring 2017, a sensor for soil temperature and moisture (EcH2O 5-TM) was installed horizontally at 5-cm depth together with data loggers (EcH2O Em50) in one mesocosm at each site used in this study. The translocation gradient represents differences in many climatic parameters, including mean annual temperature (MAT), mean annual precipitation (MAP), growing season mean air temperature, growing season precipitation, and growing degree days, which were measured at 2 m using on-site climate stations.
Both warming treatments presented here are calculated as the deviation in growing season temperature between the respective climatic control site of origin and the recipient lowland site. Plant communities translocated from Furka to Bayreuth were exposed to a warming treatment of Furka +4.4 K and plant communities translocated from Stubai to Bayreuth were exposed to a warming treatment of Stubai +3.3 K (). Five-year mean (2016–2020) growing season precipitation was 166 mm at Bayreuth, 397 mm at Stubai, and 181 mm at Furka. Growing degree days during the growing season increased by a factor of 4.2 at Furka +4.4 K and by a factor 1.4 at Stubai +3.3 K.
The start of growing season was defined by the fifth day of five consecutive days with a mean daily air temperature at 2 m higher than 5.0°C. This early season temperature threshold has been shown to primarily drive the green-up of grassland species (Scherrer and Körner Citation2010; Guo et al. Citation2018). End of growing season was defined by the day of biomass harvest at peak biomass, thereby generating a clear metric for cross-site comparisons where different growing season lengths are inevitable.
Environmental parameters
AGB was harvested annually at 3 cm aboveground at site-specific peak biomass (between mid-June and beginning of August) mimicking, for example, high elevation sheep or cattle grazing. AGB was sorted to species, dried at 60°C to constant weight (minimum 48 hours), and weighed (Berauer et al. Citation2019). Each species was assigned a treatment-level resident status (“native” or “novel”) based on whether the species occurred within any experimental community of the same origin in the year of experimental setup (2016). In three cases (Geranium sylvaticum L., Gentiana punctata L., and Sibbaldia procumbens L.) where community native species were assigned as “novel,” we decided upon local expert knowledge and adjusted the species status (e.g., perennial species first seen in second year of experiment due to disturbance of translocation itself). All species names were checked according to the Global Biodiversity Information Facility (2021). One woody species (Vaccinium uliginosum L.) originating from the alpine Furka site was excluded from analysis because it occurred in only one replicate.
Data analyses
Community-specific species turnover, colonization, and species loss rate between yearly time steps were calculated using the “codyn” package v2.0.5 (L. Hallett et al. Citation2018) as
To compare turnover rates (i.e., total turnover, species loss, and colonization) across time, we conducted generalized least square models with year-to-year species turnover as a response variable and year interacting with treatment (control versus warmed) as an explanatory variable. To cope with the nonindependence of repeated sampling of mesocosms, which introduces a temporal autocorrelation, we included variance structure in the model with mesocosm nested in year. This model was performed separately for the two sites of origin (Furka, Stubai) because they consisted of two (ecological and botanical) different mountain plant communities (alpine versus subalpine). If significant, we conducted separate post hoc Tukey’s honestly significant difference tests to compare, first, between years within one treatment (temporal change of turnover for a given treatment) and, second, within each single year between treatments (effect of climatic difference between treatments). This approach was repeated for species colonization and species loss.
We calculated PFG-specific and resident status-specific species richness and proportional AGB (levels: forb native, forb novel, graminoid native, graminoid novel, legume native, legume novel) for each community for each time step. This approach allowed us to compare relative success of PFGs while excluding the effect of community-level productivity responses. To identify relationships in PFG replacement, we first calculated mean PFG-specific AGB proportions for each community and time step. Second, we used a linear regression model for each site of origin with proportional AGB as a response variable and year and treatment as explanatory variables. Third, we conducted a post hoc Tukey’s honestly significant difference test for pairwise comparisons per PFG separately for each treatment (control versus warmed) and site of origin (Furka, Stubai).
All data analysis was performed within the R programming environment (v4.1.0, R Core Team Citation2021). All assumptions of parametric modeling were verified.
Results
Species turnover
Species turnover rate was influenced by year (pStubai = .002; pFurka < .001), treatment (pStubai < .001; pFurka < .001), and their interaction (pStubai = .01; pFurka < .001) for communities from both sites of origin (degrees of freedom = 64 for both sites of origin). Species turnover rate at both climate control treatments did not significantly change over time, indicating community stability in the absence of experimental manipulation (). Species turnover rate only changed significantly at Furka control between 2017 and 2018 (p < .001), which can be explained by elevated species turnover from 2016 to 2017 as a result of the translocation process itself (e.g., cutting of roots) and a later decrease in species turnover to natural fluctuation rates. In contrast, species turnover in warmed plant communities increased significantly with time (pStubai < .001; pFurka < .001). Furthermore, species turnover of warmed mountain plant communities differed significantly from their climatic control for every year except for the alpine plant community in 2017. Averaged across four years (excluding the year of setup), annual species loss was −2.9 ± 2.7 species out of 9.4 ± 4.2 species under the Stubai +3.3 K treatment and −2.4 ± 1.6 species out of 6.3 ± 3.1 species under the Furka +4.4 K treatment, and annual species gain was 0.4 ± 2.6 and 0.7 ± 1.8 respectively. Here, high values of standard deviation highlight strong interannual differences in local species loss and colonization. Linking the average annual losses and gains of species to the average species number present in single communities across time facilitates understanding species turnover rates above 50 percent in both warming treatments ().
Figure 1. Species turnover of mountain plant communities originating from Stubai (Austria) and Furka (Switzerland) across years in two warming and drought treatments. Warming and drought caused a significant increase in species turnover in plant communities compared to climatic control sites. Species turnover increased over time in warmed communities compared to their climatic control, where species turnover remained low. Shown are mean and standard error of turnover rates. Asterisks indicate differences between warming and control per year; letters indicate differences across years per treatment and site of origin (n = 9; p < .05 for both notations).
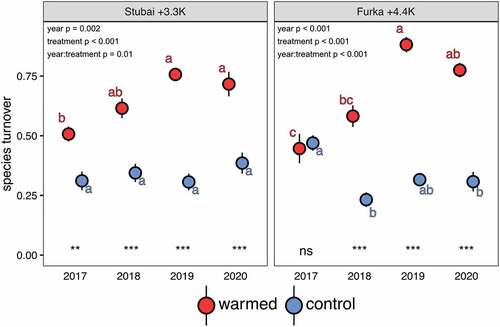
Local species loss and colonization
Species loss rates were influenced by year (pStubai = .001; pFurka = ns), treatment (pStubai < .001; pFurka < .001), and their interaction (pStubai = .003; pFurka = .024) for communities from both sites of origin. Colonization rates were influenced by year (pStubai = .001; pFurka < .001), treatment (pStubai < .001; pFurka = .017), and their interaction (pStubai = .001; pFurka < .001) for communities from both sites of origin (degrees of freedom = 64 for both sites of origin). Both warmed mountain plant communities rapidly showed high rates of local species loss (Table S1) between 2016 and 2018, followed by the increased colonization of species in 2019 (pStubai+3.3K < .001 and pFurka+4.4K < .001; ). Local species loss rates decreased from 2018 to 2019 in the Stubai +3.3K treatment (pStubai+3.3K < .001), followed by another increase from 2019 to 2020 (pStubai+3.3K < .001), whereas species loss rates remained high in the Furka +4.4K treatment throughout the entire experimental exposure. Species colonization rates decreased significantly in 2020 compared to 2019 in both treatments (pStubai+3.3K < .001 and pFurka+4.4K = .013) and leveled off at initial colonization rates.
Figure 2. (a) Yearly rates of species loss and gain in two warmed mountain plant communities. Warming combined with drought led to destabilized communities gradually losing native species, creating a window of opportunity for the colonization of novel species after the severe drought in 2018. For in situ control communities, see Figure S1. Shown are mean local rates of species loss and gain (n = 9) and standard errors (p < .05). Letters indicate differences across years per treatment. (b) Schematic of turnover of species composition at three time steps under warmed conditions: initially and after two and four years of climatic exposure. Here, the typically dense and low-statured mountain plant community from Furka (Switzerland) was disrupted by interacting stressors in 2018, creating windows of opportunity for novel species to colonize. Note that only one native species (Nardus stricta) was able to persist over a four-year warming period, and tall-statured, highly competitive novel species started to dominate the translocated communities from 2019 on, in both proportions of aboveground biomass (AGB) species richness. Sketches adapted from Jäger and Rothmaler (Citation2017).
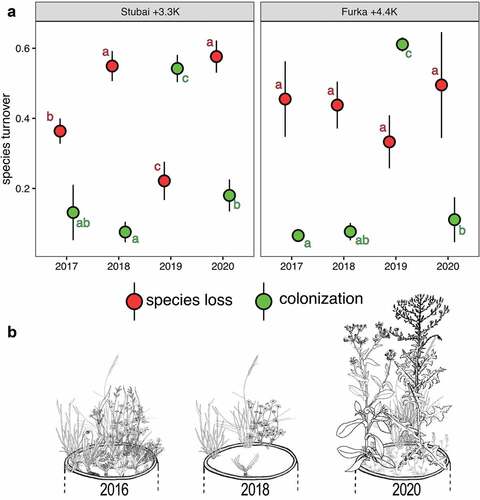
Averaged across years, species loss rates in warming treatments were higher by a factor of 2.28 compared to Stubai control and 3.07 compared to Furka control (Stubai+3.3K = 0.41 ± 0.18;
Stubaictrl = 0.18 ± 0.10;
Furka+4.4K = 0.43 ± 0.19;
Furkactrl = 0.14 ± 0.10). Colonization rates in warming treatments were higher by a factor of 1.50 compared to Stubai control and 1.26 compared to Furka control (
Stubai+3.3K = 0.24 ± 0.21;
Stubaictrl = 0.16 ± 0.10;
Furka+4.4K = 0.24 ± 0.24;
Furkactrl = 0.19 ± 0.10; Figure S1; note strong interannual differences as indicated by the high standard deviation, especially for colonization under warming).
Proportions of native vs. novel species in AGB across years
In both climatic controls at Furka and Stubai, mountain plant communities showed low colonization rates of novel species (Figure S3A and Table S1). By the end of the experiment, novel species accounted for 2.00 ± 0.04 percent and 1.00 ± 0.03 percent of mean community AGB at the control mesocosms installed in situ at Stubai and Furka, respectively (Figure S3B). In contrast, after translocation to warmer and drier conditions, mountain plant communities showed high susceptibility to the colonization by novel species particularly after the severe drought year 2018 (). In 2019, fourteen out of thirty-three and twenty-four out of twenty-six species present across all replicates were novel species in the Stubai +3.3K and Furka +4.4K treatments, respectively (Table S1). Novel species such as Lolium perenne L., Holcus lanatus L., Taraxacum sect. Ruderalia Weber, Lactuca serriola L., Sonchus asper L. Hill, and Cerastium holosteoides Fr. dominated mountain plant communities from both origins in 2019, accounting for 56 ± 1.1 percent and 63 ± 0.37 percent of mean community AGB under the Stubai +3.3K and Furka +4.4K treatments, respectively (). Whereas novel species proportion decreased in the Stubai +3.3K treatment to 39 ± 0.26 percent in 2020, it increased to 74 ± 0.31 percent in the Furka +4.4K treatment.
Figure 3. (a) Mean species richness of warmed mountain plant communities split by plant functional groups (PFG) and resident status. In both warming treatments the presence of native species is declining with time and novel species began dominating plant communities after the 2018 drought. (b) Proportional contribution of aboveground biomass (AGB) split by PFG and resident status in warmed plant communities. Relative contributions of PFGs to community productivity remain stable across years even though proportions of native and invading species have changed significantly (see also Figure S2).
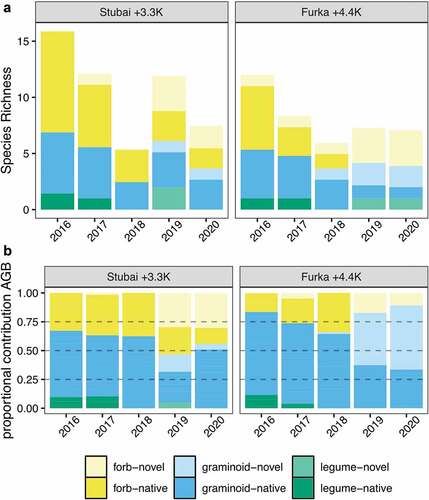
At Stubai control, the relative contribution of PFGs to community aboveground biomass remained stable (Figure S2). At Furka control only graminoids decreased their relative contribution to community AGB from 2016 to 2020 (p = .024). Forbs and one legume (Trifolium alpinum L.) showed no significant changes in relative contribution to community AGB across years at Furka control. Despite strong changes in species identity and colonization by novel species under warmed conditions, we found no significant difference in PFG proportions to AGB across years irrespective of site of origin, indicating within-PFG replacement. Though year-to-year fluctuations in PFG proportions remained insignificant, we found a tendency of increased forb proportions at cost of graminoid proportions one year after the drought in the Stubai +3.3K treatment and during the year of the drought in the Furka +4.4K treatment ( and Figure S2). Further, we found an increase in legume proportions to AGB from 2016 to 2017 (p = .017) in the Stubai +3.3K treatment (Figure S2), which is likely biased by low replicate number (n2016 = 10; n2017 = 5). Notably, there was no legume present in the year of the severe drought (2018) in the Stubai +3.3K warming treatment, whereas two novel legumes (Vicia hirsuta Fisch., Vicia tetrasperma Moris.) were found post drought in 2019.
Discussion
Our study provides critical insights regarding the dynamics of mountain plant community turnover when exposed to abrupt climate warming followed by an extreme drought, a combination of stressors with increasing likelihood in the near future (Turner et al. Citation2020; Intergovernmental Panel on Climate Change Citation2021; Stephan et al. Citation2021). We found increasing species turnover in mountain plant communities and thus local species loss under climate warming and observed local native species losses already after the first year of abrupt warming in 2017. We provide evidence that interacting climate change drivers, such as sudden warming processes interacting with a severe drought event, drive a threshold response of the studied plant communities. Specifically, we illustrate that climatic extremes may result in gradually thinned-out and less colonization-resistant communities (Kreyling, Jentsch, and Beierkuhnlein Citation2011), with native species first disappearing, thereby providing windows of opportunity for novel species to appear.
Native species responses
Most native species in warmed mountain communities experienced tremendous diebacks. In particular, forbs such as Campanula scheuchzeri Vill., Leontodon hispidus L., and Geum montanum L. did not persist post drought. Interestingly, the few native species, such as Nardus stricta L. persisting under Furka +4.4K, Alchemilla vulgaris L., Veronica chamaeadrys L., or Plantago lanceolata L. persisting until the end of the experiment under Stubai +3.3K, were species common in semi-natural grasslands in central Europe with distributional and elevational ranges that encompass the warmed treatment conditions or were found in only a few replicates, indicating individual-specific persistence. Further, only two native alpine graminoid species (Anthoxanthum alpinum Schur and Nardus stricta L.) persisted post drought (2019) in the Furka +4.4K treatment. Though the role of graminoids under climate change is controversial (Bardgett, Mommer, and De Vries Citation2014; Klanderud, Vandvik, and Goldberg Citation2015; Olsen et al. Citation2016; Rosbakh et al. Citation2017; Stampfli et al. Citation2018), here we found a high persistence of two species to drought, potentially due to their clonal, long-lived life cycle, representing the inertia of some mountain species and making it difficult to study long-term effects in warming experiments. However, interannual fluctuations in productivity and low presence rates of these native species in the replicates leave the exact mechanism of persistence unresolved in this study. Moreover, results may have been overestimated by our experiment, because it is likely that limited rooting space (Körner, Hoflacher, and Wiser Citation1978) in our experimental setup affected soil hydrological processes, making a direct comparison to natural mountain plant communities difficult. Further, this experimental constraint may have been extrapolated due to a general soil water depletion in the lowland study region (Landesamt für Umwelt Citation2020) after the extreme drought year (2018) followed by another extraordinarily dry year in 2019 (see Figure S4 for in situ measured soil moisture in warmed mesocosms).
In a previous study within the same experimental setup, Berauer et al. (Citation2019) did not find significantly increased productivity of plant communities under warmed conditions, most likely due to the severe drought in the experimental treatment compared to climatic controls (see Figure S4). This aligns with previous studies that found negative drought effects nullifying benefits from warming (De Boeck et al. Citation2016; Lamprecht et al. Citation2018; Tomiolo, Bilton, and Tielbörger Citation2020; Schuchardt et al. Citation2021). Yet, our observed decreases in native species’ AGB proportions and local diebacks of native species align with findings from other experimental studies (e.g., Rumpf et al. Citation2018; Oldfather and Ackerly Citation2019), critically discussed simulations (Dullinger et al. Citation2012; Cotto et al. Citation2017), and permanently installed plots at the long-term GLORIA site in Schrankogel, Austria (K. Steinbauer et al. Citation2020). In the latter study, the cover of most mountain species declined, and the local loss of native species became more frequent over time. Moreover, the same study concluded that gaps arising from native species diebacks were not immediately filled by native or novel species. This indicates that the mechanism facilitating novel species colonization in mountain communities is most likely not competitive pressure. Instead, increasing climatic unsuitability for native species (occasionally combined with local mechanical disturbance) leads to an initial loss of native species, resulting in potential windows of opportunity for novel species, given that such species have colonization opportunities.
Sensitivity of mountain plant communities to compound climatic change
Meineri et al. (Citation2020) found that mountain plant communities have low invasion resistance after disturbance because mountain species typically lack competitive traits such as tall stature, large leaves, heavy seed rain, or fast and opportunistic growth. Moreover, seedling establishment of mountain species is poor outside their maternal environment and community (Meineri, Spindelböck, and Vandvik Citation2013; Basto et al. Citation2018; Haynes et al. Citation2021), increasing the risk of unsuccessful sexual reproduction under climate warming. Further, Vázquez-Ramírez and Venn (Citation2021) concluded that warming alone had no significant impact on seed germination and establishment in most studies, whereas reduced precipitation had a strong negative impact. The high rates of local species loss between 2016 and 2018 in our study are likely the direct result of abiotic conditions, particularly interactions between warming and drought.
Another crucial and often ignored factor that may explain our observed local native species loss is the phenological cues of single species. Mountain specialists are adapted to or can at least tolerate well short growing seasons (Körner Citation2021). The extension of growing seasons in temperature-limited mountain ecosystems under climate warming may play an essential role in generating windows of opportunity for novel species from lowland: whereas mountain species senesce early in the season, novel species continue to grow and reproduce (Alexander and Levine Citation2019; K. Steinbauer et al. Citation2020). Berauer et al. (Citation2019) showed that warmed plant communities suffered severely from the persistent European-wide drought in 2018 (indicated by an early overall senescence). This can be interpreted as low resistance toward persistent drought effects, subsequently providing opportunities for colonization by novel species. Here, we report a significant increase in species colonization for mountain plant communities subjected to strong warming in the year after the severe drought relative to all other years.
This indicates that the nonadditive effect of abrupt warming and drought has gradually destabilized communities and created an ideal window of opportunity for novel, highly competitive species that occur locally at the recipient lowland site, such as Lolium perenne L., Holcus lanatus L., Taraxacum sect. Ruderalia Weber, Lactuca serriola L., Sonchus asper L. Hill, and Cerastium holosteoides Fr., irrespective of site of origin. Further, our findings suggest that once a canopy opening has formed (either through the progression of the warming treatment itself or by an additional extreme event like the drought in 2018) and potentially invading species are present in the matrix (see Lembrechts et al. Citation2018), community structural changes can happen quickly and may result in the dominance of novel species. Interestingly, our observed patterns under warming and drought did not differ between the site of a plant community’s origin (subalpine Stubai 1,850 m.a.s.l. and alpine Furka 2,440 m.a.s.l) even though community structure and phenological cues, and thus single species life cycles, differed tremendously, with only five shared native species (Campanula scheuchzeri Vill., Leontodon hispidus L., Rhinanthus glacialis Personnat, Rumex alpestris Jacq., and Scorzoneroides helvetica (Mérat) Holub) in the climatic control communities. Thus, a new research frontier is emerging in understanding system response to future climatic regimes that push plant communities across ecotones. Despite the feasibility constraints when translocating plant–soil mesocosms from pristine mountain ecosystems, our study highlights the importance of investigating climate change drivers—that is, increased warming and decreased precipitation—separately, to enhance mechanistic understanding of the relative importance of each of the climatic drivers to community stability and turnover. In our study, the effect of persistent warming, drying, and the extreme drought of 2018 could not be disentangled because our experimental setup explored the general net effect of future climate scenarios.
The documented time lag between loss of native species and colonization by novel species supports our hypothesis that canopy openings in mountain plant communities will not be filled immediately but gradually when disturbance interactions yield critical threshold conditions (e.g., due to nonadditive climate extremes or anthropogenic disturbances) suitable for novel species to appear. This is in line with K. Steinbauer et al. (Citation2020), who found that open space in high-elevation mountain communities was not filled immediately. This demonstrates that abiotic factors, namely, climatic unsuitability, were dominant over novel biotic competition. Such threshold dynamics potentially play a crucial role for the high inertia of mountain ecosystems, creating the so-called extinction debt. In our experimental translocation study, we found strong native species loss, because unlike previous studies exploring the extinction debt under natural conditions, we manipulated both climate (warmer and drier conditions) and novel competitor seed rain (from available matrix species pool at low elevation), with the latter factor actively removing the dispersal barriers that are still present in natural mountain ecosystems. It is likely that in previous studies investigating extinction debt, experimental treatments were not severe enough or focused on warming alone. Such a tendency was supported by Nomoto and Alexander (Citation2021), who found the strongest impacts of novel species on population growth under the highest warming scenario, and Lembrechts et al. (Citation2016, Citation2018), who found that anthropogenic disturbances create gaps, thereby enhancing the establishment success of novel species. This highlights that mountain plant communities show some resistance to warming per se; however, warming reduces resilience to subsequent stressors. Continuing warming (longer experimental exposure) or single events such as drought can launch the loss of native species and create windows of opportunity (Diez et al. Citation2012) that lead to colonization by novel species.
Extreme events may facilitate novel species establishment in semi-natural grassland communities, and the positive and stabilizing effect of species diversity on native species recovery of AGB was nullified under novel species presence (Vetter et al. Citation2020). In line with suppressed species recovery potential (see also De Boeck et al. Citation2018), our results show that disturbed mountain communities that are dominated by novel species likely remain unstable; for example, local species loss rates for mountain plant communities subjected to strong climate warming remained high during the postdrought recovery phase. A potential explanation for the second highest species turnover rates in the last year of our experiment is the dominance of annual ruderal species that took advantage of free space and resources in the year after the drought but did not persist longer than one growing season, irrespective of site of origin. An important factor that likely influenced our findings is the limited recovery potential of native species due to (1) a lack of native species seed rain from the matrix and (2) limited reproduction from native species within the mesocosm due to the applied land use regime (the annual harvest of AGB in mid-June to the beginning of August). The latter point is important for late-flowering species originating from the Furka site with no historical harvest adaptation except extensive sheep grazing. However, we consider the harvest influence minor due to the fast and early annual phenological cycle of alpine species.
Given the limited recovery potential of native species in our experimental setup, it is likely that communities shift toward an alternative stable state dominated by novel perennial species in the future. In natural mountain ecosystems, postdisturbance recovery will highly depend on the return interval of interacting climate stressors and propagule dispersal of both novel and native species.
Plant functional group replacement
Overall, we found strong changes in community structure as evidenced by local loss of native species, colonization by novel species, and shifts toward community productivity being dominated by novel species under warming. In previous studies, native species recovery was found to be suppressed under novel species presence in experimentally planted communities (Vetter et al. Citation2020) or after disturbances favoring growth of novel species over natives (Pinto and Ortega Citation2016). Here we found that two native graminoid species (Poa pratensis L. and Lolium perenne L.) as well as one native forb (Plantago lanceolata L.) were able to increase their postdrought dominance in 2020 in the Stubai +3.3K treatment, whereas none of the native species regained their predisturbance dominance in the Furka +4.4K treatment. The only legume native to Furka +4.4K (Trifolium alpinum L.) was lost during the severe drought in 2018. Trifoilim repens L. and Trifolium pratense L., common species in the Stubai +3.3K treatment during the first years of the experiment, were lost in the year of the severe drought (2018). One native (Vicia hirsuta Fisch.) and one novel (Vicia tetrasperma Moris.) legume were found post drought in 2019, indicating both species-specific recovery potentials and limitations (Isbell et al. Citation2015; Wilfahrt et al. Citation2021). Further, the loss of legumes after the severe drought may be partially attributed to the loss of mycorrhizal soil mutualists suppressing legumes post drought recovery (Prudent et al. Citation2020; Yang et al. Citation2021), though we lack the data to test this. A potential reason for the high colonization by novel species after drought might be the lack of a mountain species seed pool in the surrounding matrix (no native species seed rain). Yet, it is likely that lowland species, which are known to be highly competitive, would have outcompeted mountain species. Nevertheless, this mechanism was excluded by the experimental design.
Interestingly, relative contributions of graminoids versus forbs to community productivity did not change significantly over time and irrespective of site of origin (subalpine Stubai 1,850 m.a.s.l. and alpine Furka 2,440 m.a.s.l) due to self-replacement of species within the same PFG. Others have found functional dissimilarity of traits between native and novel species to be crucial to allow novel species colonization in warmed but undisturbed mountain communities (Catford, Jansson, and Nilsson Citation2009; Alexander, Diez, and Levine Citation2015“”). This highlights that under less severe climate scenarios, novel invading species might be forced into competitive niche carving. The observed PFG self-replacement irrespective of site of origin is likely the result of disturbance-induced windows of opportunity that promote novel species similar to natives for replacement. Further, our findings emphasize the need to focus on underlying functional traits and stronger—threshold- and biome-crossed—experimental gradients in future studies to detect mechanisms that may become critical in the future.
Conclusion
The diverse alpine and subalpine plant communities of mountain regions are vulnerable to nonadditive effects of interacting stressors such as warming and drought, especially once single species are close to their respective trailing edge. We report clear threshold responses in community dynamics, namely, abrupt increases in the dominance of novel species, when prone to sudden and compound climatic change. We suggest that competitive pressure from novel species on the native community is not the sole mechanism by which such abrupt changes occur. Instead, we show that climatic extreme events destabilized mountain plant community structure and provided windows of opportunity for novel species to establish. Notably, lost native species were replaced by novel species of the same plant functional group, with legumes being most adversely affected by warming and drought. Interacting climatic stressors are likely to increase the vulnerability of mountain plant communities in the near future.
Author contributions
AJ developed and designed the research project, developed and framed the research question and contributed to data collection and the writing of the article. MAS developed and framed the research question and contributed to data collection and data analysis and the writing of the article. JG developed and framed the research question and contributed to data collection and data analysis and the writing of the article. BJB developed and framed the research question and contributed to data collection and data analysis and the writing of the article. MB developed and framed the research question and contributed to data collection and the writing of the article. AvH contributed to data collection and the writing of the article. NY contributed to data analysis and the writing of the article.
Supplemental Material
Download Zip (2.2 MB)Acknowledgments
We thank Erika Hiltbrunner and Christian Körner for climate data and valuable feedback on an earlier version of the article, as well as Peter Wilfahrt and Franklin Alongi for fruitful discussions.
Disclosure statement
No potential conflict of interest was reported by the authors.
Supplementary material
Supplemental data for this article can be accessed online at https://doi.org/10.1080/15230430.2023.2174282.
Additional information
Funding
References
- Alexander, J. M., L. Chalmandrier, J. Lenoir, T. I. Burgess, F. Essl, S. Haider, C. Kueffer, K. McDougall, A. Milbau, M. A. Nuñez, et al. 2018. Lags in the response of mountain plant communities to climate change. Global Change Biology 24 (2):563–15. doi:10.1111/gcb.13976.
- Alexander, J. M., J. M. Diez, and J. M. Levine. 2015. Novel competitors shape species’ responses to climate change. Nature 525 (7570):515–18. doi:10.1038/nature14952.
- Alexander, J. M., and J. M. Levine. 2019. Earlier phenology of a nonnative plant increases impacts on native competitors. Proceedings of the National Academy of Sciences 116 (13):6199–204. doi:10.1073/pnas.1820569116.
- Allan, E., W. Weisser, A. Weigelt, C. Roscher, M. Fischer, and H. Hillebrand. 2011. More diverse plant communities have higher functioning over time due to turnover in complementary dominant species. Proceedings of the National Academy of Sciences 108 (41):17034–39. doi:10.1073/pnas.1104015108.
- Bahn, M., M. Schmitt, R. Siegwolf, A. Richter, and N. Brüggemann. 2009. Does photosynthesis affect grassland soil-respired CO2 and its carbon isotope composition on a diurnal timescale? New Phytologist 182 (2):451–60. doi:10.1111/j.1469-8137.2008.02755.x.
- Bardgett, R. D., L. Mommer, and F. T. De Vries. 2014. Going underground: Root traits as drivers of ecosystem processes. Trends in Ecology & Evolution 29 (12):692–99. doi:10.1016/j.tree.2014.10.006.
- Bastos, A., P. Ciais, P. Friedlingstein, S. Sitch, J. Pongratz, L. Fan, J. P. Wigneron, U. Weber, M. Reichstein, Z. Fu, et al. 2020. Direct and seasonal legacy effects of the 2018 heat wave and drought on European ecosystem productivity. Science Advances 6 (24):eaba2724. doi:10.1126/sciadv.aba2724.
- Basto, S., K. Thompson, J. P. Grime, J. D. Fridley, S. Calhim, A. P. Askew, and M. Rees. 2018. Severe effects of long-term drought on calcareous grassland seed banks. Npj Climate and Atmospheric Science 1 (1):1–7. doi:10.1038/s41612-017-0007-3.
- Bektaş, B., W. Thuiller, A. Saillard, P. Choler, J. Renaud, M.-P. Colace, R. Della Vedova, and T. Münkemüller. 2021. Lags in phenological acclimation of mountain grasslands after recent warming. Journal of Ecology 109 (9):3396–410. doi:10.1111/1365-2745.13727.
- Berauer, B. J., P. A. Wilfahrt, M. A. S. Arfin-Khan, P. Eibes, A. Von Heßberg, J. Ingrisch, M. Schloter, M. A. Schuchardt, and A. Jentsch. 2019. Low resistance of montane and alpine grasslands to abrupt changes in temperature and precipitation regimes. Arctic, Antarctic, and Alpine Research 51 (1):215–31. doi:10.1080/15230430.2019.1618116.
- Box, J. E., W. T. Colgan, T. R. Christensen, N. M. Schmidt, M. Lund, F.-J. W. Parmentier, R. Brown, U. S. Bhatt, E. S. Euskirchen, V. E. Romanovsky, et al. 2019. Key indicators of Arctic climate change: 1971–2017. Environmental Research Letters 14 (4):045010. doi:10.1088/1748-9326/aafc1b.
- Buma, B. 2015. Disturbance interactions: Characterization, prediction, and the potential for cascading effects. Ecosphere 6 (4):1–15. doi:10.1890/ES15-00058.1.
- Catford, J. A., R. Jansson, and C. Nilsson. 2009. Reducing redundancy in invasion ecology by integrating hypotheses into a single theoretical framework. Diversity and Distributions 15 (1):22–40. doi:10.1111/j.1472-4642.2008.00521.x.
- Collins, C. G., S. C. Elmendorf, J. G. Smith, L. Shoemaker, M. Szojka, M. Swift, and K. N. Suding. 2022. Global change re-structures alpine plant communities through interacting abiotic and biotic effects. Ecology Letters 25:1813–26. doi:10.1111/ele.14060.
- Cotto, O., J. Wessely, D. Georges, G. Klonner, M. Schmid, S. Dullinger, W. Thuiller, and F. Guillaume. 2017. A dynamic eco-evolutionary model predicts slow response of alpine plants to climate warming. Nature Communications 8 (1):15399. doi:10.1038/ncomms15399.
- Crepaz, H., G. Niedrist, J. Wessely, M. Rossi, and S. Dullinger. 2021. Resident vegetation modifies climate-driven elevational shift of a mountain sedge. Alpine Botany 131 (1):13–25. doi:10.1007/s00035-020-00243-6.
- De Boeck, H. J., S. Bassin, M. Verlinden, M. Zeiter, and E. Hiltbrunner. 2016. Simulated heat waves affected alpine grassland only in combination with drought. New Phytologist 209 (2):531–41. doi:10.1111/nph.13601.
- De Boeck, H. J., E. Hiltbrunner, M. Verlinden, S. Bassin, and M. Zeiter. 2018. Legacy effects of climate extremes in Alpine grassland. Frontiers in Plant Science 9. doi:10.3389/fpls.2018.01586.
- Diez, J. M., C. M. D’Antonio, J. S. Dukes, E. D. Grosholz, J. D. Olden, C. J. Sorte, D. M. Blumenthal, B. A. Bradley, R. Early, I. Ibáñez, et al. 2012. Will extreme climatic events facilitate biological invasions? Frontiers in Ecology and the Environment 10 (5):249–57. doi:10.1890/110137.
- Dullinger, S., A. Gattringer, W. Thuiller, D. Moser, N. E. Zimmermann, A. Guisan, W. Willner, C. Plutzar, M. Leitner, T. Mang, et al. 2012. Extinction debt of high-mountain plants under twenty-first-century climate change. Nature Climate Change 2 (8):619–22. doi:10.1038/nclimate1514.
- Figueiredo, L., J. Krauss, I. Steffan-Dewenter, and J. Sarmento Cabral. 2019. Understanding extinction debts: Spatio–temporal scales, mechanisms and a roadmap for future research. Ecography 42 (12):1973–90. doi:10.1111/ecog.04740.
- Gibson‐Reinemer, D. K., K. S. Sheldon, and F. J. Rahel. 2015. Climate change creates rapid species turnover in montane communities. Ecology and Evolution 5 (12):2340–47. doi:10.1002/ece3.1518.
- Global Biodiversity Information Facility. 2021. https://www.gbif.org/
- Grant, K., J. Kreyling, H. Heilmeier, C. Beierkuhnlein, and A. Jentsch. 2014. Extreme weather events and plant–plant interactions: Shifts between competition and facilitation among grassland species in the face of drought and heavy rainfall. Ecological Research 29 (5):991–1001. doi:10.1007/s11284-014-1187-5.
- Guo, L., J. Chen, E. Luedeling, J. -S. He, J. Cheng, Z. Wen, and C. Peng. 2018. Early-spring soil warming partially offsets the enhancement of alpine grassland aboveground productivity induced by warmer growing seasons on the Qinghai-Tibetan Plateau. Plant and Soil 425:177–88. doi:10.1007/s11104-018-3582-0.
- Hallett, L., M. Avolio, S. Jones, A. MacDonald, D. Flynn, P. Slaughter, J. Ripplinger, S. Collins, C. Gries, and M. Jones. 2018. codyn: Community dynamics metrics R package (version 2). KNB data repository. doi:10.5063/F1N877Z6.
- Hallett, J. S. Hsu, E. E. Cleland, S. L. Collins, T. L. Dickson, E. C. Farrer, L. A. Gherardi, K. L. Gross, R. J. Hobbs, L. Turnbull, et al. 2014. Biotic mechanisms of community stability shift along a precipitation gradient. Ecology 95 (6):1693–700. doi:10.1890/13-0895.1.
- Haynes, K. R., J. Friedman, J. C. Stella, and D. J. Leopold. 2021. Assessing climate change tolerance and the niche breadth-range size hypothesis in rare and widespread alpine plants. Oecologia 196 (4):1233–45. doi:10.1007/s00442-021-05003-9.
- Hector, A., and R. Bagchi. 2007. Biodiversity and ecosystem multifunctionality. Nature 448 (7150):188–90. doi:10.1038/nature05947.
- Hefel, C., and J. Stöcklin. 2010. Flora der Furka. Bauhinia 22:33–59.
- Hoover, D. L., A. K. Knapp, and M. D. Smith. 2014. Resistance and resilience of a grassland ecosystem to climate extremes. Ecology 95 (9):2646–56. doi:10.1890/13-2186.1.
- Ingrisch, J., S. Karlowsky, A. Anadon-Rosell, R. Hasibeder, A. König, A. Augusti, G. Gleixner, and M. Bahn. 2018. Land use alters the drought responses of productivity and CO2 fluxes in mountain grassland. Ecosystems 21 (4):689–703. doi:10.1007/s10021-017-0178-0.
- Intergovernmental Panel on Climate Change. 2021. Climate change 2021: The physical science basis. (V. Masson-Delmotte, P. Zhai, A. Pirani, S. L. Connors, C. Péan, S. Berger, N. Caud, Y. Chen, L. Goldfarb, & M. I. Gomis, Eds.). Cambridge University Press. https://www.ipcc.ch/report/ar6/wg1/downloads/report/IPCC_AR6_WGI_Full_Report.pdf
- Isbell, F., D. Craven, J. Connolly, M. Loreau, B. Schmid, C. Beierkuhnlein, T. M. Bezemer, C. Bonin, H. Bruelheide, E. de Luca, et al. 2015. Biodiversity increases the resistance of ecosystem productivity to climate extremes. Nature 526 (7574):574–77. doi:10.1038/nature15374.
- Jäger, E. J., and W. Rothmaler, Eds. 2017. Rothmaler - Exkursionsflora von Deutschland. 2: Gefäßpflanzen: Grundband (21., durchgesehene Auflage). Berlin Heidelberg: Springer Verlag.
- Jentsch, A., and P. White. 2019. A theory of pulse dynamics and disturbance in ecology. Ecology 100 (7):e02734. doi:10.1002/ecy.2734.
- Klanderud, K., V. Vandvik, and D. Goldberg. 2015. The importance of biotic vs. abiotic drivers of local plant community composition along regional bioclimatic gradients. PLOS ONE 10 (6):e0130205. doi:10.1371/journal.pone.0130205.
- Körner, C. 2021. Alpine plant life: Functional plant ecology of high mountain ecosystems. 3rd ed. Berlin Heidelberg: Springer Verlag.
- Körner, C., and E. Hiltbrunner. 2018. The 90 ways to describe plant temperature. Special Issue on Alpine and Arctic Plant Communities: A Worldwide Perspective 30:16–21. doi:10.1016/j.ppees.2017.04.004.
- Körner, C., and E. Hiltbrunner. 2021. Why is the alpine flora comparatively robust against climatic warming? Diversity 13 (8):383. doi:10.3390/d13080383.
- Körner, C., H. Hoflacher, and G. Wieser 1978. Untersuchungen zum Wasserhaushalt von Almflächen in Gasteiner-Tal. In Ökologische Analysen von Almflächen im Gasteiner Tal. Universitätsverlag, 2, ed. A. Cernusca, 67–79. WagnerWagner: Innsbruck. Veröffentlichungen des Österreichischen MaB-Hochgebirgsprogramms Hohe Tauern.
- Körner, C., G. Wieser, and H. Hoflacher. 1978. In Untersuchungen zum Wasserhaushalt von Almflächen in Gasteiner-Tal, ed. A. Cernusca, Vol. 2, 67–76. Universitätsverlag Wagner Innsbruck.
- Kreyling, J., A. Jentsch, and C. Beierkuhnlein. 2011. Stochastic trajectories of succession initiated by extreme climatic events. Ecology Letters 14 (8):758–64. doi:10.1111/j.1461-0248.2011.01637.x.
- Kudo, G., Y. Amagai, B. Hoshino, and M. Kaneko. 2011. Invasion of dwarf bamboo into alpine snow-meadows in northern Japan: Pattern of expansion and impact on species diversity. Ecology and Evolution 1 (1):85–96. doi:10.1002/ece3.9.
- Lamprecht, A., P. R. Semenchuk, K. Steinbauer, M. Winkler, and H. Pauli. 2018. Climate change leads to accelerated transformation of high-elevation vegetation in the central Alps. New Phytologist 220 (2):447–59. doi:10.1111/nph.15290.
- Landesamt für Umwelt. 2020. Niedrigwasser 2018 und 2019 – Analysen und Auswirkungen für Bayern. https://www.bestellen.bayern.de/application/eshop_app000007?SID=389222430&ACTIONxSESSxSHOWPIC(BILDxKEY:%27lfu_was_00198%27,BILDxCLASS:%27Artikel%27,BILDxTYPE:%27PDF%27)
- Lembrechts, J. J., J. Lenoir, M. A. Nuñez, A. Pauchard, C. Geron, G. Bussé, A. Milbau, and I. Nijs. 2018. Microclimate variability in alpine ecosystems as stepping stones for non-native plant establishment above their current elevational limit. Ecography 41 (6):900–09. doi:10.1111/ecog.03263.
- Lembrechts, J. J., A. Pauchard, J. Lenoir, M. A. Nuñez, C. Geron, A. Ven, P. Bravo-Monasterio, E. Teneb, I. Nijs, and A. Milbau. 2016. Disturbance is the key to plant invasions in cold environments. Proceedings of the National Academy of Sciences 113 (49):14061–66. doi:10.1073/pnas.1608980113.
- Ma, Z., H. Liu, Z. Mi, Z. Zhang, Y. Wang, W. Xu, L. Jiang, and J.-S. He. 2017. Climate warming reduces the temporal stability of plant community biomass production. Nature Communications 8 (1):15378. doi:10.1038/ncomms15378.
- Mamantov, M. A., D. K. Gibson‐Reinemer, E. B. Linck, and K. S. Sheldon. 2021. Climate‐driven range shifts of montane species vary with elevation. Global Ecology and Biogeography 30 (4):784–94. doi:10.1111/geb.13246.
- Margreiter, V., J. Walde, and B. Erschbamer. 2021. Competition-free gaps are essential for the germination and recruitment of alpine species along an elevation gradient in the European Alps. Alpine Botany 131:135–50. doi:10.1007/s00035-021-00264-9.
- McLaren, J. R., and R. Turkington. 2010. Ecosystem properties determined by plant functional group identity. Journal of Ecology 98 (2):459–69. doi:10.1111/j.1365-2745.2009.01630.x.
- Meineri, E., K. Klanderud, J. Guittar, D. E. Goldberg, and V. Vandvik. 2020. Functional traits, not productivity, predict alpine plant community openness to seedling recruitment under climatic warming. Oikos 129 (1):13–23. doi:10.1111/oik.06243.
- Meineri, E., J. Spindelböck, and V. Vandvik. 2013. Seedling emergence responds to both seed source and recruitment site climates: A climate change experiment combining transplant and gradient approaches. Plant Ecology 214 (4):607–19. doi:10.1007/s11258-013-0193-y.
- Milbau, A., D. Reheul, B. De Cauwer, and I. Nijs. 2007. Factors determining plant–neighbour interactions on different spatial scales in young species-rich grassland communities. Ecological Research 22:242–47. doi:10.1007/s11284-006-0018-8.
- Müller, L. M., and M. Bahn. 2022. Drought legacies and ecosystem responses to subsequent drought. Global Change Biology 28:5086–103. doi:10.1111/gcb.16270.
- Nomoto, H. A., and J. M. Alexander. 2021. Drivers of local extinction risk in alpine plants under warming climate. Ecology Letters 24 (6):1157–66. doi:10.1111/ele.13727.
- Oldfather, M. F., and D. D. Ackerly. 2019. Increases in thermophilus plants in an arid alpine community in response to experimental warming. Arctic, Antarctic, and Alpine Research 51 (1):201–14. doi:10.1080/15230430.2019.1618148.
- Olsen, S. L., J. P. Töpper, O. Skarpaas, V. Vandvik, and K. Klanderud. 2016. From facilitation to competition: Temperature-driven shift in dominant plant interactions affects population dynamics in seminatural grasslands. Global Change Biology 22 (5):1915–26. doi:10.1111/gcb.13241.
- Pauchard, A., A. Milbau, A. Albihn, J. Alexander, T. Burgess, C. Daehler, G. Englund, F. Essl, B. Evengård, G. B. Greenwood, et al. 2016. Non-native and native organisms moving into high elevation and high latitude ecosystems in an era of climate change: New challenges for ecology and conservation. Biological Invasions 18 (2):345–53. doi:10.1007/s10530-015-1025-x.
- Pepin, N., H. F. Diaz, M. Baraer, E. B. Caceres, N. Forsythe, H. Fowler, G. Greenwood, M. Z. Hashmi, X. D. Liu, J. R. Miller, et al. 2015. Elevation-dependent warming in mountain regions of the world. Nature Climate Change 5:424.
- Pinto, S. M., and Y. K. Ortega. 2016. Native species richness buffers invader impact in undisturbed but not disturbed grassland assemblages. Biological Invasions 18 (11):3193–204. doi:10.1007/s10530-016-1208-0.
- Ploughe, L. W., E. M. Jacobs, G. S. Frank, S. M. Greenler, M. D. Smith, and J. S. Dukes. 2019. Community Response to Extreme Drought (CRED): A framework for drought-induced shifts in plant–plant interactions. New Phytologist 222 (1):52–69. doi:10.1111/nph.15595.
- Prudent, M., S. Dequiedt, C. Sorin, S. Girodet, V. Nowak, G. Duc, C. Salon, and P.-A. Maron. 2020. The diversity of soil microbial communities matters when legumes face drought. Plant, Cell & Environment 43 (4):1023–35. doi:10.1111/pce.13712.
- R Core Team. 2021. R: A language and environment for statistical computing. https://www.R-project.org/
- Rixen, C., and S. Wipf. 2017. Non-equilibrium in alpine plant assemblages: Shifts in Europe’s summit floras. In High mountain conservation in a changing world, ed. J. Catalan, J. M. Ninot, and M. M. Aniz, 285–303. Springer International Publishing. doi:10.1007/978-3-319-55982-7_12.
- Rixen, C., S. Wipf, S. B. Rumpf, J. Giejsztowt, J. Millen, J. W. Morgan, A. B. Nicotra, S. Venn, S. Zong, K. J. M. Dickinson, et al. 2022. Intraspecific trait variation in alpine plants relates to their elevational distribution. Journal of Ecology 110:860–75. doi:10.1111/1365-2745.13848.
- Rosbakh, S., A. Leingärtner, B. Hoiss, J. Krauss, I. Steffan-Dewenter, and P. Poschlod. 2017. Contrasting effects of extreme drought and snowmelt patterns on mountain plants along an elevation gradient. Frontiers in Plant Science 8:1478. doi:10.3389/fpls.2017.01478.
- Rumpf, S. B., K. Hülber, G. Klonner, D. Moser, M. Schütz, J. Wessely, W. Willner, N. E. Zimmermann, and S. Dullinger. 2018. Range dynamics of mountain plants decrease with elevation. Proceedings of the National Academy of Sciences 115 (8):1848–53. doi:10.1073/pnas.1713936115.
- Scheffer, M., and S. R. Carpenter. 2003. Catastrophic regime shifts in ecosystems: Linking theory to observation. Trends in Ecology & Evolution 18 (12):648–56. doi:10.1016/j.tree.2003.09.002.
- Scherrer, D., and C. Körner. 2010. Infra-red thermometry of alpine landscapes challenges climatic warming projections. Global Change Biology 16:2602–13. doi:10.1111/j.1365-2486.2009.02122.x.
- Scherrer, D., and C. Körner. 2011. Topographically controlled thermal-habitat differentiation buffers alpine plant diversity against climate warming: Topographical control of thermal-habitat differentiation buffers alpine plant diversity. Journal of Biogeography 38 (2):406–16. doi:10.1111/j.1365-2699.2010.02407.x.
- Schmidt, N. M., J. B. Mosbacher, P. S. Nielsen, C. Rasmussen, T. T. Høye, and T. Roslin. 2016. An ecological function in crisis? The temporal overlap between plant flowering and pollinator function shrinks as the Arctic warms. Ecography 39 (12):1250–52. doi:10.1111/ecog.02261.
- Schuchardt, M. A., B. J. Berauer, A. Heßberg, P. Wilfahrt, and A. Jentsch. 2021. Drought effects on montane grasslands nullify benefits of advanced flowering phenology due to warming. Ecosphere 12:7. doi:10.1002/ecs2.3661.
- Spinoni, J., J. V. Vogt, G. Naumann, P. Barbosa, and A. Dosio. 2018. Will drought events become more frequent and severe in Europe? International Journal of Climatology 38 (4):1718–36. doi:10.1002/joc.5291.
- Stampfli, A., J. M. G. Bloor, M. Fischer, and M. Zeiter. 2018. High land-use intensity exacerbates shifts in grassland vegetation composition after severe experimental drought. Global Change Biology 24 (5):2021–34. doi:10.1111/gcb.14046.
- Stampfli, A., and M. Zeiter. 2020. The impact of seed deficiency on productivity and on negative drought effect in semi-natural grassland. Journal of Vegetation Science 31 (6):1066–78. doi:10.1111/jvs.12889.
- Steinbauer, M. J., R. Field, J.-A. Grytnes, P. Trigas, C. Ah-Peng, F. Attorre, H. J. B. Birks, P. A. V. Borges, P. Cardoso, C.-H. Chou, et al. 2016. Topography-driven isolation, speciation and a global increase of endemism with elevation. Global Ecology and Biogeography 25 (9):1097–107. doi:10.1111/geb.12469.
- Steinbauer, M. J., J.-A. Grytnes, G. Jurasinski, A. Kulonen, J. Lenoir, H. Pauli, C. Rixen, M. Winkler, M. Bardy-Durchhalter, E. Barni, et al. 2018. Accelerated increase in plant species richness on mountain summits is linked to warming. Nature 556 (7700):231–34. doi:10.1038/s41586-018-0005-6.
- Steinbauer, K., A. Lamprecht, P. Semenchuk, M. Winkler, and H. Pauli. 2020. Dieback and expansions: Species-specific responses during 20 years of amplified warming in the high Alps. Alpine Botany 130 (1):1–11. doi:10.1007/s00035-019-00230-6.
- Stephan, R., M. Erfurt, S. Terzi, M. Žun, B. Kristan, K. Haslinger, and K. Stahl. 2021. An inventory of Alpine drought impact reports to explore past droughts in a mountain region. Natural Hazards and Earth System Sciences 21 (8):2485–501. doi:10.5194/nhess-21-2485-2021.
- Thompson, P. L., and A. Gonzalez. 2017. Dispersal governs the reorganization of ecological networks under environmental change. Nature Ecology & Evolution 1 (6):0162. doi:10.1038/s41559-017-0162.
- Tilman, D., R. M. May, C. L. Lehman, and M. A. Nowak. 1994. Habitat destruction and the extinction debt. Nature 371 (6492):65–66. doi:10.1038/371065a0.
- Tomiolo, S., M. C. Bilton, and K. Tielbörger. 2020. Plant community stability results from shifts in species assemblages following whole community transplants across climates. Oikos 129 (1):70–80. doi:10.1111/oik.06536.
- Turner, M. G., W. J. Calder, G. S. Cumming, T. P. Hughes, A. Jentsch, S. L. LaDeau, T. M. Lenton, B. N. Shuman, M. R. Turetsky, Z. Ratajczak, et al. 2020. Climate change, ecosystems and abrupt change: Science priorities. Philosophical Transactions of the Royal Society B: Biological Sciences 375 (1794):20190105. doi:10.1098/rstb.2019.0105.
- Usinowicz, J., and J. M. Levine. 2021. Climate-driven range shifts reduce persistence of competitors in a perennial plant community. Global Change Biology 27 (9):1890–903. doi:10.1111/gcb.15517.
- Valencia, E., F. de Bello, T. Galland, P. B. Adler, J. Lepš, A. E-Vojtkó, R. van Klink, C. P. Carmona, J. Danihelka, J. Dengler, et al. 2020. Synchrony matters more than species richness in plant community stability at a global scale. Proceedings of the National Academy of Sciences 117 (39):24345–51. doi:10.1073/pnas.1920405117.
- Vázquez-Ramírez, J., and S. E. Venn. 2021. Seeds and seedlings in a changing world: A systematic review and meta-analysis from high altitude and high latitude ecosystems. Plants 10 (4):768. doi:10.3390/plants10040768.
- Vetter, V. M. S., J. Kreyling, J. Dengler, I. Apostolova, M. A. S. Arfin‐Khan, B. J. Berauer, S. Berwaers, H. J. De Boeck, I. Nijs, M. A. Schuchardt, et al. 2020. Invader presence disrupts the stabilizing effect of species richness in plant community recovery after drought. Global Change Biology 26 (6):3539–51. doi:10.1111/gcb.15025.
- Vetter, V. M. S., N. B. Tjaden, A. Jaeschke, C. Buhk, V. Wahl, P. Wasowicz, and A. Jentsch. 2018. Invasion of a legume ecosystem engineer in a cold biome alters plant biodiversity. Frontiers in Plant Science 9. doi:10.3389/fpls.2018.00715.
- Vetter, V. M. S., J. Walter, P. A. Wilfahrt, C. Buhk, M. Braun, S. Clemens, E. Dinkel, M. Dubbert, A. Schramm, F. Wegener, et al. 2019. Invasion windows for a global legume invader are revealed after joint examination of abiotic and biotic filters. Plant Biology 21 (5):832–43. doi:10.1111/plb.12987.
- Wilfahrt, P. A., A. H. Schweiger, N. Abrantes, M. A. S. Arfin-Khan, M. Bahn, B. J. Berauer, M. Bierbaumer, I. Djukic, M. van Dusseldorp, P. Eibes, et al. 2021. Disentangling climate from soil nutrient effects on plant biomass production using a multispecies phytometer. Ecosphere 12 (8):e03719. doi:10.1002/ecs2.3719.
- Yang, G., J. Roy, S. D. Veresoglou, and M. C. Rillig. 2021. Soil biodiversity enhances the persistence of legumes under climate change. New Phytologist 229 (5):2945–56. doi:10.1111/nph.17065.