ABSTRACT
Mountain freshwater communities are generally considered sensible to accelerated climatic changes, though their vulnerabilities have not been well evaluated. Individual species or species groups are expected to respond differently depending on their adaptations, traits, or distributions, but this has not yet been distinguished. This work used available climate change vulnerability scores (ccvs) of European Ephemeroptera, Plecoptera, and Trichoptera (EPT) species (n = 1,402) to (1) compare the vulnerability between species pools of different ecoregions including alpine species and alpine endemics, (2) contrast the vulnerability between the different insect orders, and (3) assess the altitude–vulnerability relationship within the European Alps. We revealed fifty alpine Plecoptera and Trichoptera species that are categorized highly vulnerable to climate change effects (= 31 percent of all highly vulnerable European species) with the highest proportions in species inventories of alpine endemics and high-altitude waters (51 percent of high-altitude species are classified as highly vulnerable). The ccvs analysis specifically for mountain waters shows that a disproportionately high number of alpine species, and particularly alpine endemics, will be affected by climate change and suggests that Ephemeroptera may be better prepared than Plecoptera and Trichoptera. Thus, this trait-based evaluation suggests that mountain stream invertebrate communities are undergoing disproportionate restructurings in response to climate change effects more than lowland communities are.
Introduction
Climate change effects can lead to species extinctions and thereby pose a threat to biodiversity on a global scale (Harte et al. Citation2004; Román-Palacios and Wiens Citation2020). Especially in mountain and alpine regions, which are widely distributed throughout both hemispheres, the recorded intensification of atmospheric change in recent and coming years, especially during summer (Meehl and Tebaldi Citation2004; Christidis, Jones, and Stott Citation2014; Hock et al. Citation2019), and consequential changes in mountain freshwaters (Niedrist, Psenner, and Sommaruga Citation2018; Niedrist Citation2023) will affect the living conditions for the occurring species (summarized in Hock et al. Citation2019). Freshwater species in these mountain regions are considered particularly threatened, because of their direct link to water properties and the reported significant changes in water temperature or discharge. Rapid contemporary warming has already been observed in high-altitude rivers (Niedrist and Füreder Citation2021) and larger mountain streams (Niedrist Citation2023), and shifting discharge phenology is expected for Central European rivers (Rottler et al. Citation2020). As a consequence, species distributions and community composition are expected to change, with some generalist species profiting (because these will increase their range) and some specialist species losing (because their range will shrink; Domisch, Jähnig, and Haase Citation2011; Giersch et al. Citation2015; Cauvy-Fraunié and Dangles Citation2019), depending on their physiological and behavioral adaptations. Species with specialist traits, such as the intolerance to warm waters or specific emergence periods or semivoltine life cycles, are likely to show range contractions due to the difficulty in adapting to these changes. Though the vulnerability of alpine terrestrial plant and animal species is documented (e.g., Guisan and Theurillat Citation2000) and overall changes of alpine streams as habitats or communities are well studied (e.g., Hotaling et al. Citation2017; Milner et al. Citation2017; Cauvy-Fraunié and Dangles Citation2019; Körner et al. Citation2022), knowledge on the different sensitivity of alpine aquatic species in their response to climate change is still limited (but see first physiological experiments by Hotaling et al. Citation2020). Understanding the vulnerability of species to fast environmental changes, however, is of relevance, because it will be decisive in assembling future communities and river ecosystem attributes (Leibold, Chase, and Ernest Citation2017). Furthermore, identifying the most vulnerable communities would aid freshwater conservation measures.
Trait-based vulnerability scores for climate change effects (e.g., shifting flow regimes, warming waters, changing water sources; Milner et al. Citation2017; Kneib et al. Citation2020) have been established for selected groups of European aquatic invertebrate species (Hershkovitz et al. Citation2015), with the intention to identify hotspots of climate-driven effects and particularly vulnerable species or species-groups. The resulting “climate change vulnerability score” (ccvs, ranging from 0 to 6) for aquatic insects is an aggregation of six autecological traits (endemism, micro-endemism, stream zonation preference, altitudinal preference, temperature preference, life history [voltinism]; Hershkovitz et al. Citation2015). All of these traits describe the sensitivity of aquatic species to changing conditions, physically (e.g., cold stenotherm organisms will be stressed by warming temperatures or longer generation frequencies will hinder adaptation or avoidance of climatic changes) or distributionally (endemic occurrence equals a high chance of extinction, species occurring at high altitudes will no longer be able to migrate further up), and species with specialist traits are likely to show range contractions or even local extinctions (Füreder Citation2007; Brittain Citation2008; Poff, Olden, and Strayer Citation2013). So far, many species of the three invertebrate orders Ephemeroptera (E), Plecoptera (P), and Trichoptera (T), have been evaluated using this vulnerability score, but spatial analyses and their implications in mountain regions are still missing. These insect orders occur in freshwaters worldwide and are, besides Chironomidae (no ccvs are available for nonbiting midges), the most abundant invertebrates in mountain and high-elevation streams (Füreder Citation1999; Castella et al. Citation2001; Füreder et al. Citation2002). Although EPT orders do not represent all animals in mountain streams, these are often used as indicators of environmental status and change. Without understanding their different vulnerabilities to effects of climate change and the importance of these in different habitats (e.g., low- or high-altitude rivers), however, their indicative usage remains limited and potentially most threatened habitats within ecoregions will remain hidden.
In this study, we assess the vulnerability of EPT species present in alpine waters using an established vulnerability score (Hershkovitz et al. Citation2015) based on a selection of climate-related traits and we compare the ratio of vulnerable species to all EPT (Ephemeroptera, Plecoptera, and Trichoptera) species within Europe and between ecoregions and different waters within ecoregions (high vs. low altitudes). Depending on their physiological constraints, assets, and preferred environmental conditions (expressed as six climate-related biological and environmental preferences and summarized as ccvs), we hypothesize that (1) taxonomically distinct groups are differently prepared for and threatened by climate change effects, with Plecoptera being the potentially most vulnerable order (Conti et al. Citation2014; Besacier Monbertrand et al. Citation2019). Further, we assume that (2) species distribution (geographical and altitudinal) is related to degree of vulnerability, with alpine taxa pools and endemic taxa pools being proportionally more threatened than the invertebrate species pool from other ecoregions, and (3) vulnerability scores are linked to traditional water quality scores (saprobity index) of the same species, with species preferring running and clearest waters (and being intolerant to oxygen depletion) being more vulnerable. In assessing these issues, we provide a basis for mitigation and conservation studies, identify special indicator species for monitoring climate change effects in mountain streams, and allow predictions about climate-driven species shifts in mountain stream communities.
Material and methods
Data sources
The sensitivity for climate-induced environmental changes is based on known ecological preferences and life history traits of European aquatic insects that are available via the www.freshwaterecology.info database (Schmidt-Kloiber and Hering Citation2015). Selections of ecological preferences of aquatic insects have been widely used as proxies for sensitivity, and climate vulnerability scores have been calculated and refined for European species of Ephemeroptera, Plecoptera, and Trichoptera (Conti et al. Citation2014; Hershkovitz et al. Citation2015). The set of ccvs, which was extracted from the database (Schmidt-Kloiber and Hering Citation2015), is based on the following six biological and environmental preference criteria (Hershkovitz et al. Citation2015): (1) endemic distribution, (2) micro-endemic distribution, (3) preference for headwater, (4) preference for high altitude, (5) preference for cold water, and (6) long-lived species and/or uni- or semivoltine development. All species were screened for matches with these criteria, and summed matches generated the vulnerability score, which ranges from 0 (invulnerable) to 6 (highly vulnerable). Though this categorization appears simplistic, these six parameters correspond to the reduced trait-based vulnerability dimensions from extensive trait-based approaches of the same species inventory (Conti et al. Citation2014).
Saprobity scores, preferences for xenosaprobic conditions, different temperature ranges (cold and warm stenothermal), water current (limnophil or rheophil), and xenosaprobic conditions, as well as the species occurrence at an ecoregion level and species altitudinal distribution ranges (<800 m, >800 m, and species without altitudinal limitations) were extracted from the same database (Schmidt-Kloiber and Hering Citation2015). Among these groups of species (EPT), we compared the relative fraction of species classified as vulnerable and nonvulnerable to climate change effects.
Data analysis
We summarized vulnerability classes and sensitiveness for species with different geographical range of occurrence (species occurring in all of Europe [Europe], in the European Alps [alpine], only in the European Alps [alpine endemics]), and only in other ecoregions around the Alps with sufficient data (Italy/Corsica/Malta, the Carpathians). These species pools were compared for their fraction of vulnerable species. Single species are generally considered as very vulnerable when classified with a ccvs of 4 or higher. Further, we related the ratio of vulnerable species with the altitudinal zonation of species occurring in the European Alps (with 4,810 m.a.s.l. being their highest peak). Potential differences in vulnerabilities between insect orders and ecoregions were tested using Mood’s median tests (by running 10,000 Monte Carlo simulations) followed by appropriate post hoc tests (pairwise Wilcoxon rank sum tests). We used the R environment (R Core Team Citation2021) for data analysis and applied functions within the packages coin (Hothorn et al. Citation2008), dplyr (Wickham et al. Citation2020), ggplot2 (Wickham Citation2016), and ggmosaic (Jeppson, Hofmann, and Cook Citation2018) for graphical illustration of key results.
Results
Among all aquatic European taxa with classifications for ecoregions (3,265 taxa within six larger taxonomic groups and sixty-five families), 59 percent were EPT taxa (1,899 taxa in forty-eight families), of which most are classified along the climate change vulnerability scale (1,402 taxa, 74 percent). For 601 of these species, the ecoregion (European) Alps is listed as part of the distribution range (ecoregion 4, www.freshwaterecology.info). Most classified alpine species belong to the order Trichoptera (326), 151 species are Plecoptera, and 124 species are Ephemeroptera.
The ratio of vulnerable alpine species largely corresponded to the ratio at the European level (). Those highly vulnerable species within the Alps (n = 50) account for 32 percent of all European species being considered vulnerable (n = 137, ), yet 50 percent of all European species classified as very highly vulnerable (ccvs = 5, n = 34) are alpine endemics (n = 17, ). Compared to other endemic species pools around the Alps (Italy, the Carpathians), the Alps have a much higher proportion and number of highly vulnerable species (). The median vulnerability significantly differs between the tested insect orders (Mood’s median χ2 = 116.36, p < .001), with Plecoptera taxa ranking significantly higher (Wilcoxon rank sum test, p < .001) as shown in ).
Figure 1. Potential vulnerabilities in EPT species pools of (a) Europe, (b) the (European) Alps, and (c) endemic species of different European ecoregions. Single dots represent the vulnerability score of single species; vertical bars limit the interquartile ranges of all dots. Summary statistics are given for overall effects (χ2 and p value from Mood’s median tests and pairwise Wilcoxon rank sum tests: *** = p < .001, ** = p < .01).
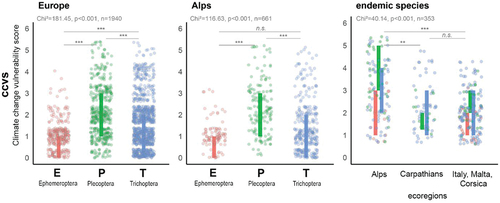
Figure 2. Classification of the EPT taxa occurring in different ecoregions (shown in different rows: Europe, Alps, alpine endemics) in different vulnerability levels (0 = invulnerable to 6 = vulnerable, with values greater than 3 being considered highly vulnerable). Bars illustrate the relative proportion of all species in the same group; corresponding values are the absolute species number.
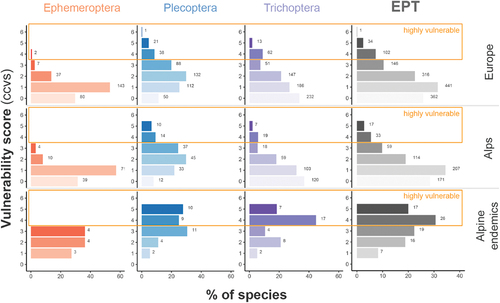
Figure 3. Classification of the EPT taxa occurring in Europe and species endemic to different European ecoregions (shown in different rows: all European species; endemics in Italy/Corsica/Malta, the European Alps, and the Carpathians) in different vulnerability levels (0 = invulnerable to 6 = vulnerable, with values greater than 3 being considered highly vulnerable). Bars illustrate the relative proportion of all species in the same group; corresponding values are the absolute species number.
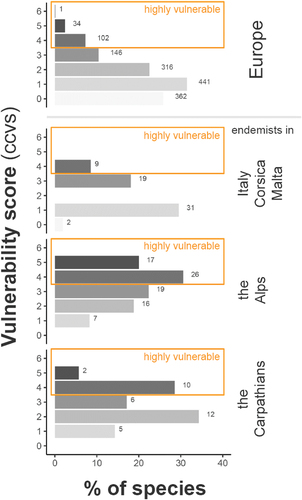
Within the EPT orders, particularly the endemic alpine Plecoptera and Trichoptera species pools have higher proportions of highly vulnerable species (ccvs ≥ 4, ). Whereas endemic Ephemeroptera species (eleven species in total) are assigned with scores up to 3 (ccvs), 53 percent of endemic Plecoptera (nineteen out of thirty-six) and 63 percent of endemic Trichoptera species (twenty-four out of thirty-eight) appeared to be very vulnerable to climate change effects (). Alpine endemic EPT taxa have significantly higher median vulnerability compared to endemics in other ecoresions ( < .001).
The breakdown of alpine species by altitudinal distribution shows that there are generally few species specialized in only occurring at higher altitudes (87 out of 400) but that this species pool also contains a disproportionally high ratio of very vulnerable species (52 percent, ). In contrast, no generalists or species restricted to low-altitude regions (<800 m.a.s.l.) are classified as vulnerable (out of 313 species).
Figure 4. Breakdown of the vulnerability scores of EPT species in the Alps ecoregion based on their altitudinal restrictions (bottom: restricted to regions <800 m.a.s.l.; center: generalists without limited altitudinal distribution; top: species occurring in regions >800 m.a.s.l.). The width of ccvs bars corresponds to the proportion of all species from the same altitudinal distribution, and the height of each row corresponds to the proportion of species having distinct altitudinal restrictions (e.g., most species in the Alps ecoregion are classified as generalists).
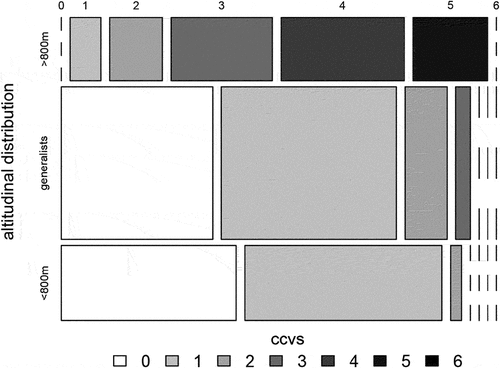
The species with ccvs-ratings between 2 and 5 are mostly classified as cold stenothermic, whereas some warm stenothermic species have lowest ccvs (0 or 1, ). Among the vulnerable species analyzed, the majority are characterized as either rheobiontic or rheophilic and very few as limnobiontic or limnophilic (). Those species with the highest vulnerability levels (2–5) have a significantly lower saprobic index scores (p < .001) and prefer xenosaprobic conditions (); that is, species that occur primarily in the clearest waters.
Discussion
Mountain ecosystems are reported as being particularly sensitive to climatic changes (e.g., Beniston and Haeberli Citation2001; Beniston Citation2005; Hock et al. Citation2019). Though most research focuses on changing abiotic conditions (summarized in Hock et al. Citation2019) and community responses are systematically monitored and predicted in terrestrial ecosystems (Engler et al. Citation2009; Gottfried et al. Citation2012), the sensitivity of water-dwelling invertebrates to climate change effects is barely known, mainly due to the lacking or inconsistent long-term observations in these environments (Jackson and Füreder Citation2006).
The effects of climate change are currently accelerating in mountain areas, with some aspects changing faster compared to the global average (Hock et al. Citation2019). For aquatic ecosystems in mountain areas, the unprecedented glacier retreat and rapidly increasing water temperatures during summer (Niedrist, Psenner, and Sommaruga Citation2018; Niedrist and Füreder Citation2021) are among the best contemporary indicators of this acceleration. Besides these well-documented changes, however, other habitat characteristics such as oxygen supply, ultraviolet radiation, or flow regime of mountain rivers are expected to change considerably in the progress of anthropogenic climate change (Elser et al. Citation2020) and will influence biotic populations in different ways (Birrell et al. Citation2020). For this reason, this study estimated potential vulnerabilities of mountain invertebrates to climate change effects using the available combination of selected species traits (those that are in conflict with projected changes; Hershkovitz et al. Citation2015) instead of single preferences (e.g., temperature preference). In combining the sensibility classification and species occurrence, we show that species pools of different ecoregions (all species in Europe, species occurring in the European Alps, and alpine endemics) possess different proportions of species vulnerable to climate change effects. Our results reveal differences between the invertebrate orders Ephemeroptera, Plecoptera, and Trichoptera in the vulnerability of their species occurring in the ecoregion European Alps, where the least impacts are to be expected for Ephemeroptera. Further, we confirm that high-altitude species pools seem to be those with highest proportions of species vulnerable or highly vulnerable to climate change consequences.
Vulnerability of species inventories from different ecoregions
This study’s results have greater precision than European-wide studies (e.g., Hershkovitz et al. Citation2015) and identify invertebrate species inventory within the ecoregion European Alps having high ratios of vulnerable species. Though the ratio of vulnerable alpine species (ccvs < 4) is comparable to the ratio at the European level, alpine species and especially alpine endemic species pools consist of much higher numbers of highly vulnerable and very highly vulnerable species (i.e., 50 percent of all European species classified as very highly vulnerable are alpine endemics). This is attributed to the general susceptibility of endemic and isolated species to climatic changes (Manes et al. Citation2021), particularly in mountain regions. The higher concentration of vulnerable species among alpine endemics in contrast to other endemic species pools (e.g., the Carpathians, Italy/Malta/Corsica) is likely due to a greater proportion of these species occurring in headwaters and high altitudes and/or having a preference for cold water temperatures.
Vulnerability of high-altitude communities
High-altitude waters and mountain headwater regions (and in particular those with glacial influence) are generally cold habitats for aquatic invertebrates (Füreder Citation1999; Jacobsen and Dangles Citation2017) but are currently warming at high rates (Niedrist and Füreder Citation2021). As this warming alters other conditions that have direct impacts on the organisms (e.g., oxygen depletion in warmer waters, higher ultraviolet penetration in less turbid glacial streams, shifting flow regimes; Birrell et al. Citation2020; Elser et al. Citation2020), life in mountain waters will be challenged in multiple aspects. Such multifactorial changes of in-stream habitat conditions and the different abilities of species to mitigate/avoid these conditions seems well covered by the ccvs (Hershkovitz et al. Citation2015).
The European Alps range from lowland to high altitudes, are drained by a variety of stream types, and retain the water in a variety of lakes at different altitudes. According to the natural (fluctuation) ranges of key habitat conditions, stenoecious species (narrow ecological tolerance) predominantly occur in high-altitude waters, whereas euryoecious species (broad ecological tolerance) colonize miscaleneous aquatic habitats across altitudinal gradients. This contrasts with the contemporary evidence for accelerated changes of habitat conditions in high-altitudes compared to lowland areas (Pepin et al. Citation2015; Hock et al. Citation2019), so that specially adapted species would have to withstand relatively large changes in habitat conditions to allow their populations to persist. Some species, including those categorized as cold stenothermal, may be able to physically tolerate higher temperatures (Castella et al. Citation2001; Niedrist and Füreder Citation2016; Hotaling et al. Citation2020), but they may experience changes in their development, emergence timing, and competition with other species that immigrate from warmer areas (Khamis et al. Citation2015; Shah et al. Citation2020). These changes could have consequences for their ability to thrive in a warmer climate. It is expected that shifts in time and phenology will lead to new interactions and ultimately to restructurings of mountain communities (Besacier Monbertrand et al. Citation2019; Shah et al. Citation2020; Timoner et al. Citation2021), but the exact mechanisms behind these changes are not yet fully understood.
Such a contrasting setting (being highly specialized while environmental conditions are shifting the most) and the high potential for competitive losses following immigrating species (Khamis et al. Citation2015) illustrate that high-altitude aquatic communities may face more drastic restructurings (especially due to loss of species groups) than lowland habitats in mountain regions (Shah et al. Citation2020). The link between the potential vulnerability and altitudinal distribution of aquatic invertebrate species reveals that, in particular, the species pool with occurrences in higher waters will be most affected by climate change effects. Half (52 percent) of those species restricted to habitats above 800 m.a.s.l. are categorized as highly vulnerable to climate change effects, whereas these vulnerability classes (ccvs ≥ 4) are absent for lowland species or species that occur in all elevations. Hence, our results confirm that species from higher elevations are more vulnerable to climate change effects (Besacier Monbertrand et al. Citation2019).
Unequal vulnerability between biotic groups
Ephemeroptera, Plecoptera, and Trichoptera are major orders of aquatic invertebrate communities and, along with Chironomidae, are important biotic components in mountain waters (Maiolini and Silveri Citation2010; Niedrist and Füreder Citation2016, Citation2017), whereas other invertebrates are rare (Alther et al. Citation2019). Though no Ephemeroptera species was evaluated as vulnerable (ccvs ≥ 4) within the alpine and alpine endemic species pool, we found several species of Plecoptera and Trichoptera categorized as such. Particularly within the endemic specialist species pool, more than 50 percent (nineteen) of the Plecoptera (mostly belonging to genera Leuctra and Nemoura) and more than 60 percent (twenty-four) of the Trichoptera species endemic to the Alps (mostly species within the genus Drusus) are rated with scores 4 (vulnerable) and higher (very vulnerable). A potential explanation for such differences is the differing degree of endemism and associated peculiar environmental conditions. Many endemic Plecoptera species are typically found at very cold temperatures and high altitudes, whereas endemic Ephemeroptera inhabit lower mountainous areas and tend to be eurythermal or can cope with greater variability (Brittain Citation2008). Trichoptera are often found colonizing springs near mountainous areas, making them ecologically similar to Plecoptera, but there are also endemic Trichoptera in downstream habitats with warmer temperatures. Hence, the larger ecological variability of Trichoptera (not restricted to high altitudes and/or cold waters) decreases the overall vulnerability of species within that order.
What also contributes to the effective vulnerability of aquatic invertebrates when habitat conditions become less favorable is the ability to disperse, a potentially important factor in a species’ ability to colonize new areas. Species that are capable of longer distance or more efficient flight may have an advantage in colonizing mountainous regions due to their ability to traverse difficult terrain (Bilton, Freeland, and Okamura Citation2001; Brederveld et al. Citation2011). Plecoptera in general are portrayed as passive and inferior fliers (Stewart, Stark, and Stanger Citation1993), a trait that would make them less able to relocate. In contrast, mayflies are assumed to have good flying abilities (Brittain Citation2008). However, dispersal abilities of the species studied in this article are barely available and were mostly assessed for Odonata (Schmidt-Kloiber and Hering Citation2015). Hence, a complete autecological information would be needed to improve or confirm the estimated vulnerabilities.
Conclusions
This link between aquatic invertebrate vulnerability scores and their occurrence in European ecoregions confirms that mountain stream invertebrate populations can be considered disproportionally more vulnerable to climate change effects in relation to invertebrates from other ecoregions, because alpine communities consist of larger proportions of species classified as vulnerable or very vulnerable. The comparisons between different ecoregions and endemic species pools underline that community changes and species turnover are disproportionally affected within alpine waters (and especially in rivers). Clearly, such disproportional extinction scenarios for endemic specialist populations in mountain streams are undesirable in terms of biodiversity conservation, but their estimation is essential to anticipate restructurings of invertebrate communities in these waters particularly threatened by the effects of atmospheric warming.
We want to clarify that the vulnerability score used in this article is one way to evaluate the vulnerability of aquatic invertebrate populations—but not of individuals per se—to climate change effects. We acknowledge that the index does not accurately reflect the actual vulnerability of species and orders, because it does not take into account important traits such as dispersal abilities but relies primarily on distribution and preferences for climate-relevant habitat conditions. Despite this limitation, ccvs can be useful in estimating the potential impact of changes on alpine freshwater invertebrate communities and identifying particularly sensitive and resilient species or genera. Such identifications will improve the understanding of long-term increase, stagnation, or decrease in population sizes that ultimately contributes to the survival or extinction of species. In addition to such technical options to improve community vulnerabilities to climate change effects in alpine waters, however, we recommend defining ccvs for other abundant invertebrate species in alpine waters such as Chironomidae (Castella et al. Citation2001; Füreder and Niedrist Citation2020). In general, such classifications of all invertebrates in other mountain regions (e.g., Rocky Mountains, the Andes, Himalayas) and the identification of particularly vulnerable groups would help to estimate climate change consequences and to assist local conservation efforts.
Data availability
The data used for this study are available via the KNB depository (Niedrist Citation2020).
Acknowledgments
We acknowledge freshwaterecology.info for managing the collection of autecological information from freshwater invertebrates all over Europe. We are grateful for the constructive criticism and comments of four anonymous reviewers on an earlier version of the article. For the purpose of open access, the author has applied a CC BY public copyright license to any author accepted manuscript version arising from this submission.
Disclosure statement
No potential conflict of interest was reported by the authors.
Additional information
Funding
References
- Alther, R., C. Thompson, B. Lods-Crozet, and C. T. Robinson. 2019. Macroinvertebrate diversity and rarity in non-glacial Alpine streams. Aquatic Sciences 81:42. doi:10.1007/s00027-019-0642-3.
- Beniston, M. 2005. Warm winter spells in the Swiss Alps: Strong heat waves in a cold season? A study focusing on climate observations at the Saentis high mountain site. Geophysical Research Letters 32:L01812. doi:10.1029/2004GL021478.
- Beniston, M., W. Haeberli. 2001. Sensitivity of mountain regions to climatic change. In Climate of the 21st century: Changes and risks, ed. J. Lozan, H. Grassl, and P. Hupfer, 237–10. Hamburg: Wissenschaftliche Auswertungen.
- Besacier Monbertrand, A.-L., P. Timoner, K. Rahman, P. Burlando, S. Fatichi, Y. Gonseth, A. Lehmann, E. Castella, and A. Lehmann. 2019. Assessing the vulnerability of aquatic macroinvertebrates to climate warming in a mountainous watershed: Supplementing presence-only data with species traits. Water 11:636. doi:10.3390/w11040636.
- Bilton, D. T., J. R. Freeland, and B. Okamura. 2001. Dispersal in freshwater invertebrates. Annual Review of Ecology and Systematics 32:159–81. doi:10.1146/annurev.ecolsys.32.081501.114016.
- Birrell, J. H., A. A. Shah, S. Hotaling, J. J. Giersch, C. E. Williamson, D. Jacobsen, and H. A. Woods. 2020. Insects in high‐elevation streams: Life in extreme environments imperiled by climate change. Global Change Biology 26 (12):6667–84. doi:10.1111/GCB.15356.
- Brederveld, R. J., S. C. Jähnig, A. W. Lorenz, S. Brunzel, and M. B. Soons. 2011. Dispersal as a limiting factor in the colonization of restored mountain streams by plants and macroinvertebrates. Journal of Applied Ecology 48:1241–50. doi:10.1111/j.1365-2664.2011.02026.x.
- Brittain, J. E. 2008. Mayflies, biodiversity and climate change. In International advances in the ecology, zoogeography, and systematics of mayflies and stoneflies, ed. F. R. Hauer and A. Welch, 1–14. Oakland, CA: University of California Press. doi:10.1525/california/9780520098688.003.0001.
- Castella, E., H. Adalsteinsson, J. E. Brittain, G. M. Gislason, A. Lehmann, V. Lencioni, B. Lods-Crozet, et al. 2001. Macrobenthic invertebrate richness and composition along a latitudinal gradient of European glacier-fed streams. Freshwater Biology 46:1811–31. doi:10.1046/j.1365-2427.2001.00860.x.
- Cauvy-Fraunié, S., and O. Dangles. 2019. A global synthesis of biodiversity responses to glacier retreat. Nature Ecology & Evolution 3:1675–85. doi:10.1038/s41559-019-1042-8.
- Christidis, N., G. S. Jones, and P. A. Stott. 2014. Dramatically increasing chance of extremely hot summers since the 2003 European heatwave. Nature Climate Change 5:46–50. doi:10.1038/nclimate2468.
- Conti, L., A. Schmidt-Kloiber, G. Grenouillet, and W. Graf. 2014. A trait-based approach to assess the vulnerability of European aquatic insects to climate change. Hydrobiologia 721:297–315. doi:10.1007/s10750-013-1690-7.
- Domisch, S., S. C. Jähnig, and P. Haase. 2011. Climate-change winners and losers: Stream macroinvertebrates of a submontane region in Central Europe. Freshwater Biology 56:2009–20. doi:10.1111/j.1365-2427.2011.02631.x.
- Elser, J. J., C. Wu, A. L. González, D. H. Shain, H. J. Smith, R. Sommaruga, C. E. Williamson, et al. 2020. Key rules of life and the fading cryosphere: Impacts in alpine lakes and streams. Global Change Biology gcb.15362. doi:10.1111/gcb.15362.
- Engler, R., C. F. Randin, P. Vittoz, T. Czáka, M. Beniston, N. E. Zimmermann, and A. Guisan. 2009. Predicting future distributions of mountain plants under climate change: Does dispersal capacity matter? Ecography 32:34–45. doi:10.1111/j.1600-0587.2009.05789.x.
- Füreder, L. 2007. Life at the edge: Habitat condition and bottom fauna of Alpine running waters. International Review of Hydrobiology 92:491–513. doi:10.1002/iroh.200610987.
- Füreder, L. 1999. High alpine streams: Cold habitats for insect larvae. In Cold-adapted organisms, ed. R. Margesin and F. Schinner, 181–96. Berlin, Heidelberg: Springer. doi:10.1007/978-3-662-06285-2_10.
- Füreder, L., and G. H. Niedrist. 2020. Glacial stream ecology: Structural and functional assets. Water 12:376. doi:10.3390/w12020376.
- Füreder, L., C. Vacha, K. Amprosi, S. Bühler, C. M. E. Hansen, and C. Moritz. 2002. Reference conditions of Alpine streams: Physical habitat and ecology. Water, Air and Soil Pollution: Focus 2:275–94. doi:10.1023/A:1020171129760.
- Giersch, J. J., S. Jordan, G. Luikart, L. A. Jones, F. R. Hauer, and C. C. Muhlfeld. 2015. Climate-induced range contraction of a rare alpine aquatic invertebrate. Freshwater Science 34:53–65. doi:10.1086/679490.
- Gottfried, M., H. Pauli, A. Futschik, M. Akhalkatsi, P. Barančok, J. L. Benito Alonso, G. Grabherr, J. Dick, B. Erschbamer, and M. R. Fernández Calzado. 2012. Continent-wide response of mountain vegetation to climate change. Nature Climate Change 2:111–15. doi:10.1038/nclimate1329.
- Guisan, A., and J.-P. Theurillat. 2000. Assessing alpine plant vulnerability to climate change: A modeling perspective. Integrated Assessment 1:307–20. doi:10.1023/A:1018912114948.
- Harte, J., A. Ostling, J. L. Green, and A. Kinzig. 2004. Biodiversity conservation: Climate change and extinction risk. Nature 430:145–48. doi:10.1038/nature02718.
- Hershkovitz, Y., V. Dahm, A. W. Lorenz, and D. Hering. 2015. A multi-trait approach for the identification and protection of European freshwater species that are potentially vulnerable to the impacts of climate change. Ecological Indicators 50:150–60. doi:10.1016/j.ecolind.2014.10.023.
- Hock, R., G. Rasul, C. Adler, B. Cáceres, S. Gruber, Y. Hirabayashi, M. Jackson, et al. 2019. High mountain areas. In IPCC special report on the ocean and cryosphere in a changing climate, ed. H.-O. Pörtner, D. C. Roberts, V. Masson-Delmotte, P. Zhai, M. Tignor, E. Poloczanska, K. Mintenbeck et al., 72. Geneva, Switzerland: IPCC.
- Hotaling, S., D. S. Finn, J. Joseph Giersch, D. W. Weisrock, and D. Jacobsen. 2017. Climate change and alpine stream biology: Progress, challenges, and opportunities for the future. Biological Reviews 92. doi:10.1111/brv.12319.
- Hotaling, S., A. A. Shah, K. L. McGowan, L. M. Tronstad, J. J. Giersch, D. S. Finn, H. A. Woods, et al. 2020. Mountain stoneflies may tolerate warming streams: Evidence from organismal physiology and gene expression. Global Change Biology gcb.15294. doi:10.1111/gcb.15294.
- Hothorn, T., K. Hornik, M. A. van de Wiel, and A. Zeileis. 2008. Implementing a class of permutation tests: The coin package. Journal of Statistical Software 28. doi:10.18637/jss.v028.i08.
- Jackson, J. K., and L. Füreder. 2006. Long-term studies of freshwater macroinvertebrates: A review of the frequency, duration and ecological significance. Freshwater Biology 51:591–603. doi:10.1111/j.1365-2427.2006.01503.x.
- Jacobsen, D., and O. Dangles. 2017. Ecology of high altitude waters, 305. Oxford: Oxford University Press.
- Jeppson, H., H. Hofmann, and D. Cook. 2018. ggmosaic: Mosaic plots in the ‘ggplot2’ framework. R package version 0.2.0.
- Khamis, K., L. E. Brown, D. M. Hannah, and A. M. Milner. 2015. Experimental evidence that predator range expansion modifies alpine stream community structure. Freshwater Science 34:66–80. doi:10.1086/679484.
- Kneib, M., S. Cauvy-Fraunié, N. Escoffier, M. Boix Canadell, Å. Horgby, and T. J. Battin. 2020. Glacier retreat changes diurnal variation intensity and frequency of hydrologic variables in Alpine and Andean streams. Journal of Hydrology 583:124578. doi:10.1016/j.jhydrol.2020.124578.
- Körner, C., U.-G. Berninger, A. Daim, T. Eberl, F. F. Mendoza, L. Füreder, S. Wickham, E. Hainzer, R. Kaiser, and E. Meyer. 2022. Long-term monitoring of high-elevation terrestrial and aquatic ecosystems in the Alps – A five-year synthesis. Eco.Mont (Journal on Protected Mountain Areas Research) 14:48–69. doi:10.1553/ECO.MONT-14-2S48.
- Leibold, M. A., J. M. Chase, and S. K. M. Ernest. 2017. Community assembly and the functioning of ecosystems: How metacommunity processes alter ecosystems attributes. Ecology 98:909–19. doi:10.1002/ecy.1697.
- Maiolini, B., and L. Silveri. 2010. EPT species distribution in 108 Alpine springs in Trentino (Italy). SIL Proceedings 1922-2010 (30):1639–42. doi:10.1080/03680770.2009.11902392.
- Manes, S., M. J. Costello, H. Beckett, A. Debnath, E. Devenish-Nelson, K.-A. Grey, M. M. Vale, T. M. Khan, W. Kiessling, and C. Krause. 2021. Endemism increases species’ climate change risk in areas of global biodiversity importance. Biological Conservation 257:109070. doi:10.1016/j.biocon.2021.109070.
- Meehl, G. A., and C. Tebaldi. 2004. More intense, more frequent, and longer lasting heat waves in the 21st century. Science 305:994–97. doi:10.1126/science.1098704.
- Milner, A. M., K. Khamis, T. J. Battin, J. E. Brittain, N. E. Barrand, L. Füreder, L. E. Brown, G. M. Gíslason, D. Jacobsen, and D. M. Hannah. 2017. Glacier shrinkage driving global changes in downstream systems. Proceedings of the National Academy of Sciences of the United States of America 114:9770–78. doi:10.1073/pnas.1619807114.
- Niedrist, G. H. 2020. Summarized vulnerability classifications of European EPT species to climate change effects [Data set]. Knowledge Network for Biocomplexity. doi:10.5063/9K48M0.
- Niedrist, G. H. 2023. Substantial warming of Central European mountain rivers under climate change. Regional Environmental Change. doi:10.1007/s10113-023-02037-y.
- Niedrist, G. H., and L. Füreder. 2016. Towards a definition of environmental niches in alpine streams by employing chironomid species preferences. Hydrobiologia 781:143–60. doi:10.1007/s10750-016-2836-1.
- Niedrist, G. H., and L. Füreder. 2017. Trophic ecology of alpine stream invertebrates: Current status and future research needs. Freshwater Science 36:466–78. doi:10.1086/692831.
- Niedrist, G. H., and L. Füreder. 2021. Real-time warming of Alpine streams: (re)defining invertebrates’ temperature preferences. River Research and Applications 37:283–93. doi:10.1002/rra.3638.
- Niedrist, G. H., R. Psenner, and R. Sommaruga. 2018. Climate warming increases vertical and seasonal water temperature differences and inter-annual variability in a mountain lake. Climatic Change 151:473–90. doi:10.1007/s10584-018-2328-6.
- Pepin, N., R. S. Bradley, H. F. Diaz, M. Baraer, E. B. Caceres, N. Forsythe, H. Fowler, et al. 2015. Elevation-dependent warming in mountain regions of the world. Nature Climate Change 5. doi:10.1038/nclimate2563.
- Poff, N. L. R., J. D. Olden, and D. L. Strayer. 2013. Climate change and freshwater fauna extinction risk. Saving a Million Species: Extinction Risk from Climate Change 309–36. doi:10.5822/978-1-61091-182-5_17/FIGURES/7_17.
- R Core Team. 2021: R: A language and environment for statistical computing. R Foundation for Statistical Computing, Vienna.
- Román-Palacios, C., and J. J. Wiens. 2020. Recent responses to climate change reveal the drivers of species extinction and survival. Proceedings of the National Academy of Sciences of the United States of America 117:4211–17. doi:10.1073/pnas.1913007117.
- Rottler, E., T. Francke, G. Bürger, and A. Bronstert. 2020. Long-term changes in central European river discharge for 1869-2016: Impact of changing snow covers, reservoir constructions and an intensified hydrological cycle. Hydrology and Earth System Sciences 24:1721–40. doi:10.5194/HESS-24-1721-2020.
- Schmidt-Kloiber, A., and D. Hering. 2015. www.freshwaterecology.info – An online tool that unifies, standardises and codifies more than 20,000 European freshwater organisms and their ecological preferences. Ecological Indicators 53:271–82. doi:10.1016/J.ECOLIND.2015.02.007.
- Shah, A. A., M. E. Dillon, S. Hotaling, and H. A. Woods. 2020. High elevation insect communities face shifting ecological and evolutionary landscapes. Current Opinion in Insect Science 41:1–6. doi:10.1016/j.cois.2020.04.002.
- Stewart, K. W., B. P. Stark, and J. A. Stanger. 1993. Nymphs of North American stonefly genera (Plecoptera), 460. Denton, Tex: University of North Texas Press.
- Timoner, P., M. Fasel, S. S. Ashraf Vaghefi, P. Marle, E. Castella, F. Moser, and A. Lehmann. 2021. Impacts of climate change on aquatic insects in temperate alpine regions: Complementary modeling approaches applied to Swiss rivers. Global Change Biology 27:3565–81. doi:10.1111/gcb.15637.
- Wickham, H. 2016. ggplot2: Elegant graphics for data analysis. New York: Springer-Verlag.
- Wickham, H., R. François, L. Henry, and K. Müller 2020: dplyr: A grammar of data manipulation. R package version 1.0.1. https://cran.r-project.org/package=dplyr.