ABSTRACT
In the nineteenth century, numerous settlements were established in the alpine region of Fennoscandia (the Scandes), an area that later became a major international scene for Arctic research. Here we raise awareness of this era and show that earthworm-driven bioturbation in “pristine” soils around contemporary Arctic research infrastructure is caused by soil fauna left behind during early land use. We use soil preserved under an alpine settlement to highlight that soils were not bioturbated when the first house was built at a site where bioturbation is now widespread. A review of archived material with unique site-specific chronology constrained the onset of bioturbation to the post-1871 era. Our results suggest that small-scale land use introduced earthworms that now thrive far beyond the realms of former cultivated fields. The legacy of soil fauna from this example of “ecological imperialism” still lingers and should be considered when studying soils of the Scandes.
Introduction
The alpine zone of Fennoscandia (the Scandes) is considered one of Europe’s last wildernesses that remains largely undisturbed by humans (Cretois et al. Citation2021). This area also constitutes a key region for Arctic research because the northern parts of the Scandes represent the most intensely studied Arctic environment () and thus have a disproportionally large impact on international Arctic research (Metcalfe et al. Citation2018). When asked about key processes behind Arctic environmental change, researchers active within Arctic Fennoscandia primarily list abiotic drivers controlled by climate systems (Pascual et al. Citation2021). However, recent work has emphasized the importance of soil fauna when understanding soil functions in the Arctic and that organisms dwelling in the soil may determine how ecosystems respond to ongoing warming (Blume-Werry et al. Citation2022). But is the soil fauna present within the Scandes an inherent natural property of the Arctic or a result of early human land use?
Figure 1. Map of northern Sweden with the limit of cultivation. The gray triangles surrounding the Abisko Scientific Research Station, managed by the Swedish Polar Research Secretariat, are the field huts Stordalen, Latnjajaure, Kärkevagge, and Lullihatjårro. These huts are used for accommodating researchers, and some have various monitoring infrastructures. The Maiva cabin (Jieprenkiedde) is also used in this respect but has historically been a settlement. The Tarfala Research Station is Stockholm University’s center for glaciological and alpine research. Vindelfällens research station is owned and managed by Vindelfjällens forskningssällskap. The Kilpisjärvi Biological Station is managed by the University of Helsinki. Archaeological remains from the National Heritage Board’s database (KMR), located above the limit of cultivation, are presented. The blue circles denote house foundations from recorded history (often from the nineteenth century), and orange squares indicates agrarian remains, such as fossil fields, clearance cairns, cattle sheds (Sw. fäbod), farm lots, etc. The semitransparent red polygon is approximated from Metcalfe et al. (Citation2018) and shows the area of northern Fennoscandia with the highest density of field sampling locations and citations from environmental research above the Arctic Circle.
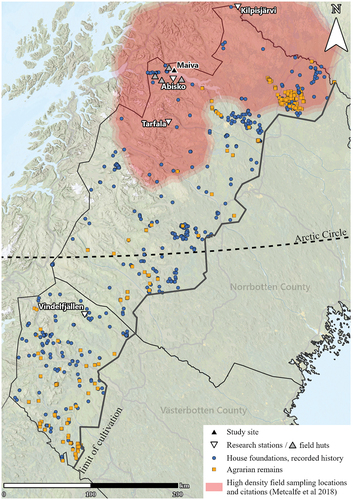
The latter question seems justified because it has been argued that earthworm species known for being able to physically rework soils (e.g., cause bioturbation) likely arrived in the Scandes with human-mediated transport (Wackett et al. Citation2018). These earthworms, mainly L. rubellus and Aporrectodea sp., dwell in the mineral soil and restructure soil nutrient pools and subsequently change conditions for other soil organisms (Lavalle et al. Citation2006). Earthworms believed to be native in the Scandes, such Dendrobaena sp., are litter dwellers, and the introduction of ecosystem engineering species has been observed to cause great mixing of well-stratified natural soils (Wackett et al. Citation2018). A scenario where humans introduced ecosystem engineering earthworms to the Scandes seems plausible, because a similar process has been documented in North America where farmers unintentionally brought in crops containing soil with earthworm cocoons, causing European worms to establish in natural forests (Hale et al. Citation2005; Cameron, Bayne, and Clapperton Citation2007; Eisenhauer et al. Citation2007; Hendrix et al. Citation2008). In these forests, the burrowing actions of earthworms have been shown to fundamentally alter the vertical distribution of soil organic matter and important nutrients, such as phosphorous (Resner et al. Citation2015). Experimental work has shown that if earthworm species now present at sites in the Arctic were historically introduced by early settlers, it would have generated great impacts on the nitrogen cycle (Blume-Werry et al. Citation2020) and soil hydrology (Klaminder et al. Citation2023). Despite the cold climate in the Scandes, it is an oversimplification to assume that this area has not been affected by farming in the past. In the mid-nineteenth century, farmers settled throughout the Scandes (Rudberg Citation1957; Arell Citation1977; Bergström Citation1979), and hay mowing by later generations has been argued to be a possible driver of Arctic vegetation change (Callaghan et al. Citation2013). As evident from archaeological observations, these settlements included areas within or adjacent to Arctic research infrastructures in the Scandes where soil processes are presumed to represent pristine Arctic conditions (). Considering the regional importance of the migration of farmers to the Scandes, surprisingly few studies have recognized that some parts of the existing soil fauna, particularly earthworms, might be a legacy from these nineteenth-century settlers. Instead, when earthworms have been observed to physically mix (bioturbated) well-stratified soils into cambisols (“brown earth”) within the Scandes, their presence has been interpreted as evidence for pockets of soil formed under a pre-twentieth-century warmer climate (Kullman Citation2007), a broad time constraint that includes warm periods such as the Mid-Holocene Warm Period or the Medieval Warm Period (Moberg et al. Citation2005). Though the view of earthworms as relicts of warmer periods or indicators of specific local conditions might be correct, it also seems plausible that nineteenth-century settlements initiated a form of belowground “ecological imperialism” (Crosby Citation1986) by introducing nonnative earthworm species.
Earthworms were likely eradicated from the Scandes when the Weichselian ice sheet covered Scandinavia, similar to the glacial eradication described for earthworms in North America (Bohlen et al. Citation2004). Determining when in time ecosystem engineering earthworms arrived to the Scandes and whether they are a natural part of the soil fauna or not is important because their actions are known for causing vegetation change (Craven et al. Citation2017), influence plant biomass (Hale et al. Citation2008), increase soils’ ability to produce greenhouse gases (Lubbers et al. Citation2013), affect grazing animals’ performance (Wurst Citation2010), and making soils drier (Klaminder et al. Citation2023). Mechanisms by which ecosystem engineering earthworms affect other organisms are largely driven by their physical mixing (bioturbation) of the soil, which not only causes a redistribution of nutrient pools but also exposes seeds to foragers and induces drought stress for plants (Frelich et al. Citation2019). In areas with podzolic soils, bioturbation is easily detected because it diminishes the organic horizon (O) and converts the eluvial (E) horizon to an organic-rich mineral soil layer (A). Though earthworm-induced bioturbation occurs in subalpine forests around gardens, composts, and farmlands in the Scandes (Wackett et al. Citation2018), any empirical evidence suggesting that this process was not part of the landscape before the arrival of the first farmers is currently lacking. This knowledge gap makes it difficult for policymakers to decide whether ecosystem engineering species are a natural part of the soil fauna found locally within the most intensely studied Arctic environment.
In this study we ask: did mineral soil dwelling earthworm species (species that drive bioturbation) exist in the Scandes prior to the arrival of nineteenth-century farmers? We provide the first empirical evidence suggesting that soil currently being strongly bioturbated by earthworms (i.e., brown earth) was podzolic prior to the arrival of farmers in the late nineteenth century. We illustrate this by comparing soil preserved under a house foundation constructed by the first settlers with a soil mapping of the surrounding subalpine birch forest. We constrain the chronology of the settlement by using documented historical sources and mapping of soil phosphorus. The land use history at the site should be viewed as being representative of other sites in the Scandes where traditional Sámi culture merged with nineteenth-century farmers and tourism.
Materials and methods
Site description
Our study focused on the area around Lake Torneträsk, situated in northern Sweden, where Arctic research is mainly conducted at an international scale (). The mean annual air temperature and precipitation in the region are 1°C and 330 mm, respectively (Swedish Meteorological and Hydrological Institute Citation2023). The rationale for focusing on this area was as follows: (1) the settlement history was uniquely well documented due to its dramatic history that attracted interest by locals and the national press but also as a cabin on the site (i.e., the Maiva cabin, 68°26′55.4″ N, 18°51′34.7″ E) was donated to the Royal Swedish Academy of Sciences; (2) the site at the northern side of Lake Torneträsk is remote (no roads) and has, in contrast to the southern side of the lake, not been strongly affected by the societies developing around the railroad that was constructed in the early twentieth century; (3) the Maiva cabin belongs to a research infrastructure provided by the Swedish Polar Research Secretariat, making the cabin a hub for monitoring and field research; (4) a survey conducted in 2016 and 2017 suggested that nonnative earthworm species (Lumbricus rubellus and Aporrectodea sp.) were widely dispersed around the cabin (Wackett et al. Citation2018). As shown in this latter study, Aporrectodea sp. frequently exceed 200 individuals m−2 near the cabin and earthworms suggested to originate from the cabin are argued to have dispersed up to 800 m into a subalpine Arctic birch forest. The birch forest around the flatter terrace on which the Maiva cabin stands has been described as unusually lush and green; Birger (Citation1909) depicted the birch forest as being park-like, and Sjögren (Citation1907) portrayed the forest meadows as rich and beautiful.
Archival sources and the ambiguity of historical sources
The biography of the northern side of Lake Torneträsk was derived from Swedish and Norwegian church archives, encompassing written sources such as birth, baptismal, wedding and death records, communion registers, catechetical examination registers (Sw. husförhörslängder), and parish records (Sw. församlingsböcker). The original documents are stored at the Swedish National Archive (Sw. Riksarkivet) and at the National Archives of Norway (No. Arkivverket), but they are also available through their search services, the Digital Research Room and the Digital Archives, respectively. Other historical sources used in the study include census records, the archive of the Sámi bailiff (Sw. lappfogde) in North Bothnia’s northern district, the Swedish Tourist Association yearbook, and the Swedish Newspaper database, which is maintained by the National Library of Sweden (Sw. Kungliga biblioteket, KB).
Archaeological survey
At the Maiva cabin site, earthworms occur in high abundances (>10 g m−2), and due to bioturbation, soils commonly lack the podzolic master horizons (O, E, B) typical for the region but instead have a structure with only A and B master horizons (Wackett et al. Citation2018). To map the extent of bioturbation in relation to the remains of a house foundation found near the Maiva cabin, we sampled soil with an open-ended soil probe (3.5-cm bore diameter and 30-cm length) using a 20 × 20 m grid sampling approach. Denser sampling grids were placed over archaeological remains. Samples for phosphate analysis, soil organic matter content (SOM), and magnetic susceptibility (MS) were consequently collected at around 5-cm depth in Ah horizons (soil affected by bioturbation), whereas in the Ap horizons (soil mixed by manual tillage) the depth averaged 10 cm; in eluviation horizons, samples were collected from the E horizon. The collected samples were analyzed in a laboratory and the different parameters are described below.
Analytical techniques and procedure
In this study, we used standard geoarchaeological geo-prospection techniques, namely, soil phosphate content and fractionation, SOM, and MS. Soil phosphate analysis is a commonly used tool for localizing and defining settlement areas and agricultural impact as well as identifying and classifying different archaeological deposits (Goldberg and Macphail Citation2006; Holliday and Gartner Citation2007; Weiner Citation2010; French Citation2015). Phosphorous (P) is present within all soils as compounds in various inorganic and organic forms and is continuously recycled from organisms to soil and vice versa. In addition to this natural cycle, P can be introduced to soils by humans. In archaeological contexts, anthropogenic P includes human and animal waste; refuse from bone, meat, fish, and plants; burials of corpses and carcasses; organic building materials; and excreta and manure (Holliday Citation2004; Macphail and Goldberg Citation2018). In archaeology, soil P analysis is employed on the principle that human activity redistributes P. By depositing organic materials in some areas, the P levels will increase, whereas farming, harvesting, or other such practices without manuring will decrease levels in other areas (Holliday Citation2004). These activities are detectable in the archaeological record because P is resistant to leaching, is rapidly fixed and relatively immobile in most soil types, and is also rather stable in comparison to other elements within the soil system. The current levels of P can reflect the sum of past organic inputs to the soil system and also the spatial redistribution caused by various depositions; for instance, of human or animal wastes (Campbell, Moffett, and Straker Citation2011). The soil P analysis in this study is a modified version of Arrhenius’s (Citation1934) citric acid method (Engelmark and Linderholm Citation1996; Linderholm Citation2007). This includes two fractions of phosphate (inorganic [CitP] and sum of organic and inorganic [CitPOI]). The latter is an approximation of total P content but only in relation to the extraction ability of citric acid. These techniques combined with SOM, through loss on ignition (Carter Citation1993), are used to understand the local impacts of household and farming activities on site and soil formation. The procedure for analyzing MS of a substance is by exposing it to an external magnetic field to measure its ability to become magnetized. MS analysis is suitable for finding, identifying, and defining spaces with in situ burning, metallurgical deposits, and archaeological features (Engelmark and Linderholm Citation1996; Linderholm Citation2007; Milek and Roberts Citation2013). In this study, data are reported as low-frequency measurements (10−8 m3 kg−1 mass-specific susceptibility) on 10 g soil (Thompson and Oldfield Citation1986; Dearing Citation1994; Walden, Oldfield, and Smith Citation1999).
Statistics
Effects of earthworm mixing and historic land use on topsoil chemistry (concentrations of inorganic and total phosphate and SOM) were evaluated statistically by comparing the chemistry of three morphologically different mineral soil layers: E horizons (soil not affected by mixing), Ah horizons (soil affected by bioturbation), and Ap horizons (soil mixed by manual tillage). Effects on chemical properties as a function of these three mixing regimes were tested using a one-way analysis of variance (ANOVA) after validating that the data fulfilled the normality criteria (). The latter was done by using a Kolmogorov-Smirnov test of normality. Differences in topsoil chemistry between categories were tested using Tukey’s honestly significant difference post hoc test.
Table 1. Results of a one-way ANOVA for SOM, CitPOI, and CitP of the topsoil using soil mixing regime as factor.
Results
The chronological aspects of the narratives at the study site are compiled and described in detail along with their original written sources in the supplemental material, but a brief summary follows below.
In short, human activities, besides early hunter–gatherer communities, impacted the environment on the northern side of Lake Torneträsk primarily via reindeer herding until the mid-nineteenth century (). Human impact on the northern shoreline increased during the agrarian settlement era, with clearing and farming, which was, at the earliest, initiated in 1871. Note that we were able to identify the first settlers in the area by name, recollect their biographies, and construct a detailed chronology of their land use. We also located photographic evidence of cows at the northern side of the lake (), which also shows how the vegetation has changed at the Maiva site since 1946 (). Today, the landscape is primarily used for reindeer herding, tourism, and research.
Figure 2. Timeline from a Holocene perspective, with focus on the Maiva site between 1870 and 1970. The Torneträsk region was ice free at ca. 8000 BC (Geological Survey of Sweden shore displacement model), and the earliest evidence of human presence is found in the lithic material of the area, where worked pieces of quartzite and quartz generally can be dated to the Mesolithic (see supplemental material). The earliest occurrence of Sámi presence can be seen around 1100 AD, which is the typological dating of the artifact assemblage in a scree-grave at Aravuobma (Mulk, Nordqvist, and Pettersson Citation1993). However, there may be predating occurrences in the area considering the lack of archaeological surveys and excavations. The timeline of Maiva shows the different phases covering a 100-year period, from 1870 to 1970.
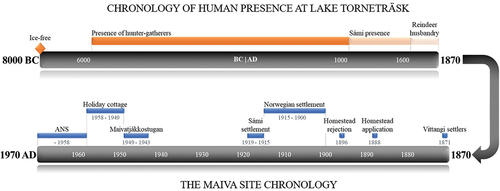
Figure 3. Photographs of the Maiva cabin in 1946 and 2020: (a) the tourist station, Maivatjåkkostugan, during the summer of 1946 with the two directors, several visitors, and the cow Mustakorva; (b) the cabin in its current state, as a research outpost; (c) the two directors walking from the cabin toward the shore with the surrounding vegetation of 1946; (d) the denser vegetation of 2020 with the cabin in the center of the photo.
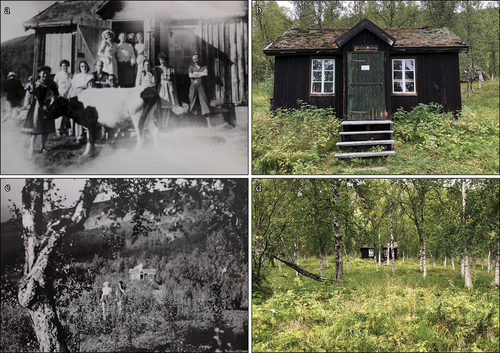
The archaeological survey
With information regarding early settlements (), use of historical maps (), and site descriptions found in archives, together with our archaeological fieldwork, we were able to locate the remains of the house that the first (Vittangi) settlers constructed in 1871 (, no. 4). The house foundation measured 11 × 6 m (west–east) with surrounding turf walls ca. 1 to 2 m wide and 0.8 to 1 m high. The house contained two clearly distinguished rooms (4 × 3 m and 3 × 2.5 m) with oven remains (Sw. spismursröse) centrally placed along the northern wall and a distinct entrance to the south.
Figure 4. (a) the Torneträsk region with archaeological sites registered in KMR. Sámi and undefined nomadic sites are marked with yellow circles whilst agrarian and industrial sites are marked with orange squares. (b) The section of a land from a surveyor’s map (25-kij-18:66) covering the Maiva cabin and its surroundings. Meadows are delimited in green, arable land in yellow, and wetland areas for haymaking in orange. The flatter terrace on which the cabin stands is called Jebrenkedde or Jèbrènkèddē (Wiklund Citation1910, 58), with kèddē or kiedde meaning meadow, camping ground, reindeer corralling/milking ground, abandoned milking ground with ample pasture (Collinder Citation1964, 84). The river west of Maiva is called Snuvrejohka or Snūrijocka and the river east of Maiva is called Jiebrenjohka or Jèbrènjocka. The mountain just north of Maiva is called Jiebrenčorru, which is connected to a larger range consisting of two peaks, Jiebrenčohkka and Láirečorru (Snuoratjåkko and Airavare), which together form the Làìretjohkko (Lerfjället or Clay Mountain).
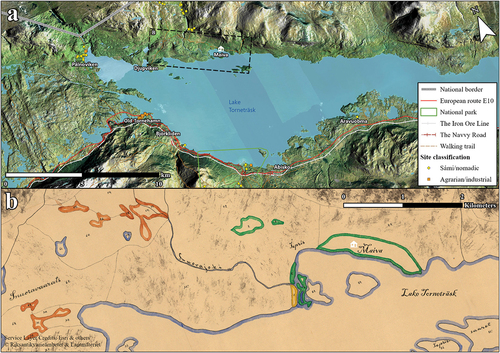
Figure 5. (a) Overview of the study site with constructions, foundations, and remains marked with white and numbered as follows: 1, peat goahti; 2, the Maiva cabin; 3, outbuilding; 4, house foundation; 5, herder cabin; 6, peat goahti remains; 7, house foundation. The base map depicts soil moisture and is based on data from the Land Survey (Lantmäteriet), Geological Survey of Sweden (Sveriges geologiska undersökning), and the Swedish Meteorological and Hydrological Institute (Sveriges meteorologiska och hydrologiska institut). Red pixels indicate dry soil and blue indicates wet soil. Samples are marked with circles for Ah horizons, black dots for Ap horizons, and gray squares for E horizons. A paleosol (a buried E horizon) is marked with an X. The extent of maps B and C are marked with dotted lines in map A. (b) The northern part of the site. (c) The southern part of the site. The isolines ranging from 50 to 80 are interpolated MS values from soil samples. The point symbols represent the CitPOI accumulation in parts per million P and are distributed according to size and color in three classes. SP1, paleosol; SP2, active cambisol.
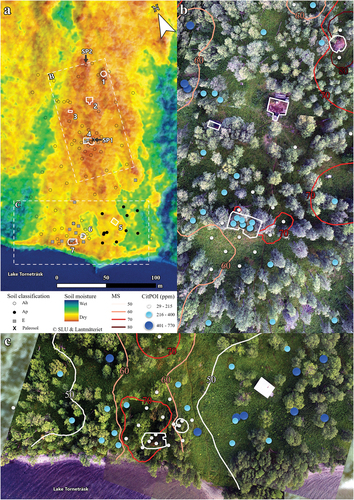
About 40 m further north stands the Maiva cabin (, no. 2). Through information derived from newspaper clippings in combination with archival material, we were able to infer that this cabin originally belonged to an old fisherman (Anders Andersson), before it was turned into a tourist station. West of the cabin is an outbuilding serving as an outhouse and storehouse (, no. 3), and north of the cabin stands a peat goahti (, no. 1, a Sámi hut or tent). In the southeastern part of the area is a contemporary reindeer herder’s cabin (, no. 5). Another house foundation is located in the southwest (, no. 7); here, fragmented parts of the wooden roofing were still visible on the surrounding mound. This building consisted of one room and did not exhibit any remains of an oven. Photographs and information from a diary kept by one of the managers of the tourist station identified this building as a stable. Close to the stable were the remains of a goahti (, no. 6). Note that we found information stating that Anders Andersson lived in a peat goahti (HLA B III/1 1905) before he constructed a permanent building (the Maiva cabin).
The environmental archaeology
Bioturbation was a widespread feature at the site, and 86 percent of the sampled soil was classified as mixed mineral soil (Ah or Ap). Only patches of less mixed mineral soil with an E horizon still present were found near the shoreline. This latter soil also contained earthworm burrows and was slightly bioturbated but not enough to be classified as an Ah. Anthropogenic reworking of the upper 20 cm of the soil was apparent in the southeast, around the herder cabin, where soil horizons were classified as Ap (); that is, we use the suffix “p” to denote horizons that were judged to have been plowed or manually tilled down to ca. 20 cm depth. Inside the house foundation of the Vittangi settlers, beneath remains of a sill beam in the northern turf wall, a paleosol (buried podzol) was identified (). The finding is important considering that all contemporary soil adjacent to the cabin was mixed (), whereas the paleosol buried under the house fundament was well structured and lacked visible earthworm burrows. The geographical distribution of Ah and Ap horizons had no apparent connection to moisture modeled from the topography (); hence, the morphological types were found at both dry and wet sites (differences in hydrology seemed unlikely to explain their distribution). Possible influence of iron-rich hearth rakes detected as increased MS was detected adjacent (east) of the Vittangi house and west of the collapsed peat goahti ().
Figure 6. Paleosol (SP1) consisting of a podzol buried beneath a house foundation and active cambisol (SP2) in close proximity to the house foundation. Note that the Ah of the paleosol (SP1) has an anthropogenic origin (deposit from the house construction) and how earthworm activity has reworked the E (and B) horizon in the cambisol outside the house construction (SP2).
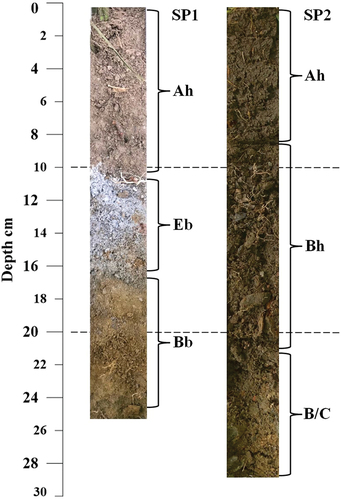
About 74 percent of the of the 102 soil samples was bioturbated (Ah horizon), 15 percent was unmixed (E horizon remaining), and 12 percent was part of an abandoned farmland (Ap horizon). We compared the chemical properties of these three categories (unmixed, bioturbated, and cultivated) to assess to what extent difference in mixing affected the topsoil chemistry (). Soil mixing had an impact on the SOM content (F2,112 = 42.382, p < .001), where the post hoc test suggested a higher SOM content in the cultivated soil (mean ± SE, 35 ± 4 percent) in comparison to the bioturbated soil (11 ± 1 percent) and morphological unmixed soil (6 ± 1 percent). There was no statistical difference (p > .78) in SOM between the unmixed and the bioturbated soil. However, total P (CitPOI) differed between all mixing regimes (F2,112 = 27.695, p < .001), where the lowest concentration occurred in the unmixed soil (121 ± 21 ppm), which was lower than both the cultivated soil (469 ± 40 ppm) and the bioturbated soil (270 ± 14 ppm). There was also a significant difference in inorganic phosphorous (CitP) as a result of soil mixing regimes (F2,112 = 9.599, p < .001). Here, the post hoc analysis revealed that soil not physically mixed by earthworms had a lower inorganic P concentration (59 ± 15 ppm) than the bioturbated soil (111 ± 6 ppm), and the bioturbated soil had higher concentration than the relict farmland (61 ± 7 ppm).
Figure 7. Topsoil chemistry as a result of soil mixing regimes (unmixed, cultivated, and bioturbated). Data include soil organic matter content (upper panel), total phosphorous (middle panel), and inorganic phosphorous (lower panel). Bars indicate mean values and the standard errors are highlighted as error bars. Individual data points are shown to the right of the bar along with the inferred normal distribution. Different letters (a, b, c) above the bars indicate statistical differences (p < .05) between mixing regimes according to Tukey’s honestly significant difference post hoc test, where similar letters imply no statistical difference (p > .05) between mixing regimes.
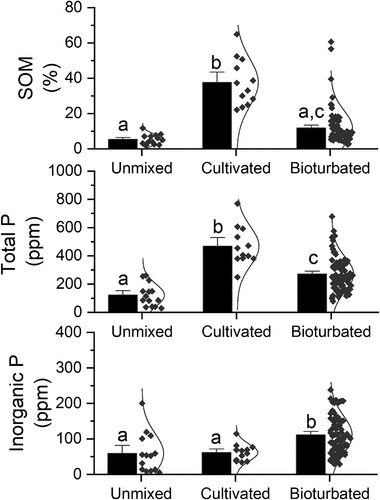
Discussion
Arctic researchers only occasionally recognize that early land use may influence the contemporary Arctic environment. In the case of the Scandes, these exceptions include acknowledgment of possible vegetation effects caused by hay mowing during the early twentieth century (Callaghan et al. Citation2013). Another example from the Scandes involves sites used for milking reindeer until the early twentieth century that have left a visible impact on vegetation (Egelkraut Citation2017; Egelkraut et al. Citation2018). To date, eventual impacts from alpine farming, fishermen, and early twentieth-century tourism are not commonly discussed as a driver of contemporary features in the Arctic. The land use history outlined for the northern side of Torneträsk in our work brings awareness to the diverse, but largely forgotten, land use in one of the remote parts of the Scandes. The written documentation that we found highlights that the northern side of Lake Torneträsk has been used not only by prehistoric hunter–gatherers and traditional Sámi herding cultures but also by generations of farmers, hunters, fishers, and early tourists. Moreover, as our survey illustrates, soil underlying meadows and a subalpine birch forest near one of the settlements have been reworked not only manually by human tillage, as evident from findings of an old, cultivated field, but also by bioturbation from earthworms. Our study suggests that bioturbation by earthworms was a process that was unleashed during the post-settlement era, as indicated by the finding of unmixed podzolic soils preserved under the house foundation of house 4, a construction that our historical documentation predicts was constructed in 1871 by settlers from Vittangi; hence, this date serves as a terminus ante quem for the earthworm introduction. Our chemical analysis of the topsoil reveals that ecosystem engineering earthworm species arriving after the Vittangi settlement have boosted the soil fertility by greatly increasing the concentration of phosphorous (both inorganic P and total P) in the topsoil. It is noteworthy to highlight that the effect on SOM and total P is still higher locally due to historic land use relating to cultivation but that the soil fertility effect caused by earthworms extends far from the rim of the relict farmland.
It is evident from contemporary observations that ecosystem engineering earthworm species are found at a distance of 800 m from the old, cultivated field (Wackett et al. Citation2018). It is known that grazing cattle can facilitate transport of earthworm cocoons from farms into surrounding environments via soil caught by their hooves (Hoogerkamp, Rogaar, and Eijsackers Citation1983), and documentation of past usage of at least cows at the site raise the possibility that forest grazing have contributed to the dispersion of earthworms far from the settlement. However, earthworms have been shown to disperse at rates of 5 to 10 m yr−1 in American forests (Gundale, Jolly, and Deluca Citation2005), which makes it possible that they have reached a distance of 1 km from their original introduction point since 1871 by their own means.
Our observations are in line with previous studies emphasizing that earthworms can hitchhike with soil and crops brought by farmers, cattle, or fishermen using worms as bait (James and Hendrix Citation2004). Whether the post-settlement introduction of earthworms to the Maiva area was intentional or unintentional is not known. The often-cited example of Aristotle calling them “intestines of the earth” does not appear to be connected to soil fertility (Rota Citation2011), but during the eighteenth century, by the latest, the positive effects of earthworms for farming were well recognized (van Groenigen et al. Citation2019), and it seems possible that the generations of nineteenth- and twentieth-century farmers intentionally brought earthworms to Maiva. Earthworms are also effective bait for anglers, and it cannot be excluded that fishermen or even later tourists or researchers contributed to the establishment of earthworms in the early twentieth century. Earthworms seem most likely to have been established, at the latest, in the early twentieth century when Anders Andersson settled in the area, engaging with agriculture, animal husbandry, and fishing.
Revisiting previous interpretations of earthworm presence in the Scandes
We note that sites with earthworm-bioturbated soils previously have been interpreted as relict features of warmer periods; that is, trees growing in this soil were judged to have been established prior to the twentieth century (Kullman Citation2007). With our results in mind, an alternative explanation for earthworm-induced bioturbation includes a scenario where earthworms originally dispersed from a nearby nineteenth-century alpine settlement named Tjatjasen (KMR L1946:5752) or from other houses and cattle sheds further down the valley. The tree line at this site is well within the reach of earthworm migration seen in our study (<800 m) because the Tjatjasen settlement includes remnants of agriculture and is situated about 500 m from the tree line. Moreover, a Swedish newspaper from 1886 described the presence of cows, sheep, goats, and horses near the farm (see supplemental material); hence, earthworm dispersal across the landscape prior to the twentieth century could have been mediated by the hooves of these animals as transport agents. Though we cannot resolve the origin of earthworms for other sites, the contrasting predictions made using existing theories about their origin is worth noting. The “relict soil” hypothesis suggests that earthworm bioturbation is a diminishing process in the Scandes since the twentieth century because podzolic soil is interpreted to be closing in (Kullman Citation2007). In contrast, our findings suggest that bioturbation is a process that has expanded over the same timeframe and, thus, bioturbation did not exist on the site prior to 1871.
Ecosystem engineering earthworms that are present around Maiva have also been found near present-day settlements in harsh Arctic climates, including finds in Greenland and the North American Arctic (Blume-Werry et al. Citation2020). Nevertheless, climatic parameters such as temperature and precipitation are important for explaining earthworm diversity (Phillips et al. Citation2019), and the Scandes has experienced a 1°C to 2°C warming in annual temperatures since the 1980s (Rantanen et al. Citation2022). Indeed, this warming has likely increased earthworm activities during the last decades and, thus, we cannot exclude that the ongoing bioturbation rates are currently more pronounced than at the time of the settlers. In fact, a scenario where the dispersal of ecosystem engineering earthworms introduced by early settlers has accelerated during the last decades due to improved climatic conditions seems likely. Nevertheless, our findings, which constrain bioturbation to the post-settlement era, inform policymakers of the anthropogenic, nonnative origin of bioturbating earthworm species in the contemporary environment in the Scandes.
Conclusions
The land use history described for the north side of Lake Torneträsk was not unique but rather a narration repeated across the Scandes. Nevertheless, the environmental legacy of pre-twentieth-century land use in the Scandes is rarely discussed. That early land use is not recognized as a driver of environmental change may be due to a limited understanding about the extent of alpine farming within the Scandes or due to an assumption that the land use was too small scale to make an impact. However, the latter view likely centers on the belief that impacts were solely restricted to human use of tools (axes, shovels, and plows), fire, and grazing livestock (reindeer, cows, etc.). It is evident from our study that bioturbation improved the nutrient status of the topsoil, by more than doubling the concentration of inorganic P, and that the extent of the impact from an aerial perspective were more extensive than impacts generated directly by human land use. That is, we estimate that the nutrient content of about 74 percent of the mapped soil at the study site was increased by bioturbation, whereas only 12 percent was impacted be manual reworking. Importantly, our estimate of earthworm-affected soil is likely an underestimate given that earthworms have also been detected hundreds of meters outside the mapped area (Wackett et al. Citation2018). Given the recognized role of soil nutrients and bioturbation as drivers of environmental change, it seems plausible that small-scale land use generated an ecological impact far beyond former cultivated fields by mediating the dispersal of nonnative earthworms. For example, experimental work with tundra soil suggests that, at the time of their arrival, earthworm species that are currently present at the study site likely boosted the growth of graminoid vegetation (Blume-Werry et al. Citation2020) and created more porous and dry soil (Klaminder et al. Citation2023).
The study area is part of the most intensively studied region of the Arctic (Metcalfe et al. Citation2018). Here, research on interactions between Arctic soils and our planet’s climate system has been devoted substantial scientific attention (Dorrepaal et al. Citation2009). Interestingly, the soil fauna community, including the occurrence of earthworms, has been argued to be central when predicting how Arctic soils respond to climate change (Blume-Werry et al. Citation2022). With this in mind, it seems urgent to determine drivers of their current distribution in the Scandes to improve our ability to predict their future distribution.
Supplemental Material
Download Zip (131.1 KB)Disclosure statement
No potential conflict of interest was reported by the authors.
Supplementary material
Supplemental data for this article can be accessed online at https://doi.org/10.1080/15230430.2023.2274650
Additional information
Funding
References
- Arell, N. 1977. Reindeer nomadism in Torne Lappmark: Land use during the colonization period in Enontekis parish. Umeå: Umeå University. (In Swedish)
- Arrhenius, O. 1934. Phosphate content in Scanian soils. Stockholm: SGU. (In Swedish)
- Bergström, S. O. 1979. Colonization of Kronoparks in Norrbotten 1894–1950. Umeå: Umeå University. (In Swedish)
- Birger, S. 1909. Jebrenjokkstugan. In Svenska Turistföreningens årsskrift 1909, and R. Melander, 373–5. Stockholm: Centraltryckeriet. (In Swedish)
- Blume-Werry, G., J. Klaminder, E. J. Krab, and S. Monteux. 2022. Ideas and perspectives: Alleviation of functional limitations by soil organisms is key to climate feedbacks from Arctic soils. Biogeosciences 20: 1979–13. doi:10.5194/bg-20-1979-2023.2023.
- Blume-Werry, G., E. J. Krab, J. Olofsson, M. K. Sundqvist, M. Väisänen, and J. Klaminder. 2020. Invasive earthworms unlock Arctic plant nitrogen limitation. Nature Communications 11: 1766. doi:10.1038/s41467-020-15568-3.
- Bohlen, P. J., S. Scheu, C. M. Hale, M. A. McLean, S. Migge, P. M. Groffman, and D. Parkinson. 2004. Non-native invasive earthworms as agents of change in northern temperate forests. Frontiers in Ecology and the Environment 2: 427–35. doi:10.1890/1540-9295(2004)002[0427:NIEAAO]2.0.CO;2.
- Callaghan, T. V., C. Jonasson, T. Thierfeilder, Z. Yang, H. Hedenås, M. Johansson, U. Molau, et al. 2013. Ecosystem change and stability over multiple decades in the Swedish subarctic: Complex processes and multiple drivers. Philosophical Transactions of the Royal Society 368: 20120488. doi:10.1098/rstb.2012.0488.
- Cameron, E. K., E. M. Bayne, and M. J. Clapperton. 2007. Human-facilitated invasion of exotic earthworms into northern boreal forests. Écoscience 14, no. 4: 482–90. doi:10.2980/1195-6860(2007)14[482:HIOEEI]2.0.CO;2.
- Campbell, G., L. Moffett, and V. Straker. 2011. Environmental Archaeology. A guide to the theory and practice of methods, from sampling and recovery to post-excavation. 2nd ed. Portsmouth: English Heritage.
- Carter, M. R. 1993. Soil sampling and methods of analysis. London: Lewis Publishers.
- Collinder, B. 1964. Dictionary of Sweden’s Sámi place names. Uppsala: Kungliga Ortnamnskomissionen. (In Swedish)
- Craven, D., M. P. Thakur, E. K. Cameron, L. E. Frelich, R. Beauséjour, R. B. Blair, B. Blossey, et al. 2017. The unseen invaders: Introduced earthworms as drivers of change in plant communities in North American forests (a meta-analysis). Global Change Biology 23: 1065–74. doi:10.1111/gcb.13446.
- Cretois, B., J. D. C. Linnell, B. van Moorter, P. Kaczensky, E. B. Nilsen, J. Parada, and J. K. Rod. 2021. Coexistence of large mammals and humans is possible in Europe’s anthropogenic landscapes. iScience 24. doi:10.1016/j.isci.2021.103083.
- Crosby, A. W. 1986. Ecological imperialism: The biological expansion of Europe, 900–1900. Cambridge: Cambridge University Press.
- Dearing, J. 1994. Environmental magnetic susceptibility. Kenilworth: Chi Publishing.
- Dorrepaal, E., S. Toet, R. van Logtestijn, E. Swart, M. J. van de Weg, T. V. Callaghan, and R. Aerts. 2009. Carbon respiration from subsurface peat accelerated by climate warming in the subarctic. Nature 460: 616–9. doi:10.1038/nature08216.
- Egelkraut, D. 2017. Long-lasting ecological legacies of reindeer on tundra vegetation. Umeå: Umeå University.
- Egelkraut, D., K.-Å. Aronsson, A. Allard, M. Åkerholm, S. Stark, and J. Olofsson. 2018. Multiple feedbacks contribute to a centennial legacy of reindeer on tundra vegetation. Ecosystems 21: 1545–63. doi:10.1007/s10021-018-0239-z.
- Eisenhauer, N., S. Partsch, D. Parkinson, and S. Scheu. 2007. Invasion of a deciduous forest by earthworms: Changes in soil chemistry, microflora, microarthropods and vegetation. Soil Biology & Biochemistry 39, no. 5: 1099–110. doi:10.1016/j.soilbio.2006.12.019.
- Engelmark, R., J. Linderholm. 1996. Prehistoric land management and cultivation: A soil chemical study. In 6th Nordic Conference on the application of scientific methods in Archaeology, Esbjerg 1993, ed. V. Mejdahl and P. Siemen, 315–22. Esbjerg: Esbjerg Museum.
- Frelich, L. E., B. Blossey, E. K. Cameron, A. Dávalos, N. Eisenhauer, T. Fahey, O. Ferlian, et al. 2019. Side-swiped: Ecological cascades emanating from earthworm invasions. Frontiers in Ecology and the Environment 17, no. 9: 502–10. doi:10.1002/fee.2099.
- French, C. 2015. A handbook of geoarchaeological approaches for investigating landscapes and settlement sites. Oxford: Oxbow Books.
- Goldberg, P., and R. I. Macphail. 2006. Practical and theoretical geoarchaeology. Malden: Blackwell.
- Gundale, M. J., W. M. Jolly, and T. H. Deluca. 2005. Susceptibility of a northern hardwood forest to exotic earthworm invasion. Conservation Biology 19: 1075–83. doi:10.1111/j.1523-1739.2005.00103.x.
- Hale, C. M., L. E. Frelich, P. B. Reich, and J. Pastor. 2005. Effects of European earthworm invasion on soil characteristics in northern hardwood forests of Minnesota, USA. Ecosystems 8: 911–27. doi:10.1007/s10021-005-0066-x.
- Hale, C. M., L. E. Frelich, P. B. Reich, and J. Pastor. 2008. Exotic earthworm effects on hardwood forest floor, nutrient availability and native plants: A mesocosm study. Oecologia 155: 509–18. doi:10.1007/s00442-007-0925-6.
- Hendrix, P. F., M. A. Callaham Jr., J. M. Drake, C.-Y. Huang, S. W. James, B. A. Snyder, and W. Zhang. 2008. Pandora’s Box contained bait: The global problem of introduced earthworms. Annual Review of Ecology, Evolution, and Systematics 39, no. 1: 593–613. doi:10.1146/annurev.ecolsys.39.110707.173426.
- Holliday, V. T. 2004. Soils in archaeological research. Oxford: Oxford University Press.
- Holliday, V. T., and W. G. Gartner. 2007. Methods of soil P analysis in archaeology. Journal of Archaeological Science 34: 301–33. doi:10.1016/j.jas.2006.05.004.
- Hoogerkamp, M., H. Rogaar, H. J. P. Eijsackers. 1983. Effect of earthworms on grassland on recently reclaimed polder soils in the Netherlands. In Earthworm ecology from Darwin to vermiculture, ed. J. E. Satchell, 85–105. London: Chapman and Hall.
- James, S. W., P. F. Hendrix. 2004. Invasion of exotic earthworms into North America and other regions. In Earthworm ecology, 2nd ed., ed. C. A. Edwards, 75–88. Boca Raton: CRC Press.
- Klaminder, J., E. J. Krab, M. Larsbo, H. Jonsson, J. Fransson, and J. Koestel. 2023. Holes in the tundra: Invasive earthworms alter soil structure and moisture in tundra soils. Science of the Total Environment 859: 1–8. doi:10.1016/j.scitotenv.2022.160125.
- Kullman, L. 2007. Long-term geobotanical observations of climate change impacts in the Scandes of West-Central Sweden. Nordic Journal of Botany 24: 445–67. doi:10.1111/j.1756-1051.2004.tb02209.x.
- Lavalle, P., T. Decaëns, M. Aubert, S. Barot, M. Blouin, F. Bureau, P. Margerie, P. Mora, and J.-P. Rossi. 2006. Soil invertebrates and ecosystem services. European Journal of Soil Biology 42: 3–15. doi:10.1016/j.ejsobi.2006.10.002.
- Linderholm, J. 2007. Soil chemical surveying: A path to a deeper understanding of prehistoric sites and societies in Sweden. Geoarchaeology 22: 417–38. doi:10.1002/gea.20159.
- Lubbers, I., K. van Groenigen, S. Fonte, J. Six, L. Brussaard, and J. W. van Groenigen. 2013. Greenhouse-gas emissions from soils increased by earthworms. Nature Climate Change 3: 187–94. doi:10.1038/nclimate1692.
- Macphail, R. I., and P. Goldberg. 2018. Applied soils and micromorphology in archaeology. Cambridge: Cambridge University Press.
- Metcalfe, D. B., T. D. G. Hermans, J. Ahlstrand, M. Becker, M. Berggren, R. G. Björk, M. P. Björkman, et al. 2018. Patchy field sampling biases understanding of climate change impacts across the Arctic. Nature Ecology & Evolution 2: 1443–8. doi:10.1038/s41559-018-0612-5.
- Milek, K. B., and H. M. Roberts. 2013. Integrated geoarchaeological methods for the determination of site activity areas: A study of a Viking Age house in Reykjavik, Iceland. Journal of Archaeological Science 40: 1845–65. doi:10.1016/j.jas.2012.10.031.
- Moberg, A., D. M. Sonechkin, K. Holmgren, N. M. Datsenko, and W. Karlén. 2005. Highly variable Northern Hemisphere temperatures reconstructed from low- and high-resolution proxy data. Nature 433: 613–7. doi:10.1038/nature03265.
- Mulk, I.-M., L. Nordqvist, and M. Pettersson. 1993. The grave at Aravuobma: An archaeological investigation of a scree-grave at Torneträsk, Jukkasjärvi parish, Kiruna municipality, Lapland. Jokkmokk: Ájtte Svenskt Fjälloch Samemuseum. (In Swedish)
- The National Board of Surveying and Mapping. 1892. Lantmäterimyndigheternas arkiv. Avvittring, Jukkasjärvi kronoöverloppsmark, file designation 25-kij-18:66.
- Pascual, D., J. Åkerman, M. Becher, T. V. Callaghan, T. R. Christensen, E. Dorrepaal, U. Emanuelsson, et al. 2021. The missing pieces for better future predictions in subarctic ecosystems: A Torneträsk case study. Ambio 50: 375–92. doi:10.1007/s13280-020-01381-1.
- Phillips, H. R. P., C. A. Guerra, M. L. B. Bartz, M. J. I. Briones, G. Brown, T. W. Crowther, O. Ferlian, et al. 2019. Global distribution of earthworm diversity. Science 366: 480–5. doi:10.1126/science.aax4851.
- Rantanen, M., A. Y. Karpechko, A. Lipponen, K. Nordling, O. Hyvärinen, K. Ruosteenoja, T. Vihma, and A. Laaksonen. 2022. The Arctic has warmed nearly four times faster than the globe since 1979. Communications Earth & Environment 3. doi:10.1038/s43247-022-00498-3.
- Resner, K., K. Yoo, S. D. Sebestyen, A. Aufdenkampe, C. Hale, A. Lyttle, and A. Blum. 2015. Invasive earthworms deplete key soil inorganic nutrients (Ca, Mg, K, and P) in a northern hardwood forest. Ecosystems 18: 89–102. doi:10.1007/s10021-014-9814-0.
- Riksantikvarieämbetets Kulturmiljöregister (KMR). L1946:5752. (In Swedish)
- Rota, E. 2011. Early oligochaete science, from Aristotle to Francesco Redi. Archives of Natural History 38: 136–63. doi:10.3366/anh.2011.0011.
- Rudberg, S. 1957. Wilderness and peripheral settlements in interior Northern Sweden: A discussion of certain causal relationship behind the urban and non-urban distribution. Uppsala: Almqvist & Wiksell. (In Swedish)
- Sjögren, O. 1907. Abisko and excursions from there. Stockholm: Centraltryckeriet. (In Swedish)
- Swedish Meteorological and Hydrological Institute (SMHI). 2023. Meteorological data from Abisko Observatory: Air temperature hourly value, 2022-05-31 to 2023-06-01, Daily precipitation, 2021-03-01 to 2022-02-28. https://www.smhi.se/data/meteorologi/ladda-ner-meteorologiska-observationer#stationid=188800,param=airtemperatureInstant,stations=core.
- Thompson, R., and F. Oldfield. 1986. Environmental magnetism. London: Allen & Unwin.
- van Groenigen, J. W., K. J. van Groenigen, G. F. Koopmans, L. Stokkermans, H. M. J. Vos, and I. M. Lubbers. 2019. How fertile are earthworm casts? A meta-analysis. Geoderma 338: 525–35. doi:10.1016/j.geoderma.2018.11.001.
- Wackett, A. A., K. Yoo, J. Olofsson, and J. Klaminder. 2018. Human-mediated introduction of geoengineering earthworms in the Fennoscandian Arctic. Biological Invasions 20: 1377–86. doi:10.1007/s10530-017-1642-7.
- Walden, J., F. Oldfield, and J. Smith. 1999. Environmental magnetism: A practical guide. London: Quaternary Research Association.
- Weiner, S. 2010. Microarchaeology: Beyond the visible archaeological record. Cambridge: Cambridge University Press.
- Wiklund, K. B. 1910. The Sámi and Finnish place names at Kiruna and Torneträsk. Uppsala: Akademiska Bokhandeln. (In Swedish)
- Wurst, S. 2010. Effects of earthworms on above- and belowground herbivores. Applied Soil Ecology 45: 123–30. doi:10.1016/j.apsoil.2010.04.005.