ABSTRACT
Permafrost-agroecosystems include all cultivation and pastoral activities in areas underlain by permafrost. These systems support local livelihoods and food production and are rarely considered in global agricultural studies but may become more relevant as climate change is increasing opportunities for food production in high latitude and mountainous areas. The exact locations and amount of agricultural production in areas containing permafrost are currently unknown, therefore we provide an overview of countries where both permafrost and agricultural activities are present. We highlight the socioecological diversity and complexities of permafrost-agroecosystems through seven case studies: (1) crop cultivation in Alaska, USA; (2) Indigenous food systems and crop cultivation in the Northwest Territories, Canada; (3) horse and cattle husbandry and Indigenous hay production in the Sakha Republic, Russia; (4) mobile pastoralism and husbandry in Mongolia; (5) yak pastoralism in the Central Himalaya, Nepal; (6) berry picking and reindeer herding in northern Fennoscandia; and (7) reindeer herding in northwest Russia. We discuss regional knowledge gaps associated with permafrost and make recommendations to policy makers and land users for adapting to changing permafrost environments. A better understanding of permafrost-agroecosystems is needed to help sustainably manage and develop these systems considering rapidly changing climate, environments, economies, and industries.
Introduction
Permafrost, ground material that remains below 0°C for two or more consecutive years (International Permafrost Association, Citation1998), is the foundation for many high latitude and mountainous socialecological systems (Vincent, Lemay, and Allard Citation2017). Agricultural systems in these regions include horticultural crops, grain cultivation, floriculture, and animal husbandry (including mobile pastoralism) on arable permafrost-affected soils and/or pasture lands; henceforth termed permafrost-agroecosystems (Ward Jones et al. Citation2022). Agricultural production in permafrost-agroecosystems is usually small scale with products consumed locally to regionally. They represent a small proportion of the global food and agricultural product supply (Unc et al. Citation2021) apart from a few niche export markets, such as Alaskan peonies (Fresco et al. Citation2021) or cashmere fibers from goats in Mongolia (Maekawa Citation2013). These agricultural systems are diverse but involve limited crop and animal species adapted to colder climates. For example, yaks, commonly raised in high mountain Asia, are not able to survive when temperatures become too high (Krishnan et al. Citation2016). Overall, permafrost-agroecosystems have a long tradition and significant cultural importance.
Approximately 24 percent of the land surface of the Northern Hemisphere (Obu Citation2021), and 0.15 percent of the Southern Hemisphere (Gruber Citation2012) fall within a permafrost region (subglacial permafrost such as in Greenland or Antarctica is not included). Regions are classified into zones that describe lateral permafrost continuity: (1) continuous permafrost covers 90–100 percent of land area; (2) discontinuous permafrost covers 50–90 percent; (3) sporadic 10–50 percent; and (4) isolated covers areas with less than 10 percent. Permafrost occurs both in high latitudes and in lower latitude mountainous areas, and underlies a seasonal thawed layer known as the active layer. Measured mean annual permafrost temperatures vary from just below freezing to −20°C (Biskaborn et al. Citation2019). The thickness and properties of high latitude permafrost are tied to glacial and climate history as well as interactions through ecological succession (Shur and Jorgenson Citation2007). Mountain permafrost forms at high altitudes and its local characteristics are dependent on slope, aspect, and other environmental conditions (Etzelmüller Citation2013).
Among the most populous permafrost-agroecosystems in Northern and Inner Asia, it is difficult to overemphasize the significance of permafrost to landbased activities, among these, pastoralism, crop production, and gardening. While pastoralism emerged thousands of years ago, with sheep, horse, and cattle being the most prominent species, largescale reindeer pastoralism commenced in comparatively recent times (Stépanoff Citation2017). Since the nineteenth century, crop production in Inner Asia has been mainly driven by nation states and their economic policies, with agricultural areas expanding and shrinking along with nation-state incentives or withdrawals (Naumov, Sidorova, and Goncharov Citation2022; Kuhmonen and Kuhmonen Citation2023). In precolonial areas of North America underlain with permafrost, wild foods, obtained through harvesting from the land and waters formed the basis of traditional Indigenous food systems. These food systems have been sustainable for generations, as Indigenous peoples have adapted to changes in seasons, migrations, and availability of plants and animals. Some high latitude Indigenous populations have been stewarding agricultural crops for several centuries, such as potato cultivation practiced by the Tlingit and Haida people of southeast Alaska and western British Columbia, Canada (Zhang et al. Citation2010). The practices and crops we often associate with the term “agriculture” came to northern North America first through Russian communities in Alaska (early to mid-nineteenth century), followed by the influx of trappers, traders, and later miners from the Gold Rush of the late 1800s (Miller Citation1951). Early high latitude agriculturalists were surprised to learn about the then mysterious impacts of permafrost degradation without necessarily knowing what permafrost was (Rockie Citation1942).
Permafrost can be directly affected by agricultural activities, such as land clearing for cultivation, which removes the overlying insulating vegetation that exposes the underlying permafrost to climate-driven thaw (Ward Jones et al. Citation2022). Other agricultural activities, such as reindeer herding, are affected by permafrost degradation, such as being forced to change migration routes (Istomin and Habeck Citation2016). How permafrost responds to thaw is dependent on ground ice content and permafrost type. System responses to permafrost thaw vary from changes to soil temperatures in ice-poor, thaw-stable permafrost to dramatic topographic changes driven by surface subsidence (known as thermokarst) through ground ice melt within ice-rich permafrost (Ward Jones et al. Citation2022). Degradation of ice-rich permafrost generates thermokarst () and waterlogging conditions creating potentially dangerous conditions that can lead to animal loss for herders (Istomin and Habeck Citation2016); for cultivation, it can lead to crop loss, damage to machinery and infrastructure, and decreases in soil fertility that can ultimately lead to field abandonment (Péwé Citation1954; Desyatkin et al. Citation2021; Ward Jones et al. Citation2022). Therefore, permafrost thaw can strongly alter drainage and affect soil cultivation, leading to additional costs and uncertainty for agriculturalists. Changes in soil hydrology, in combination with the release of previously frozen organic matter, also alter microbial activity and consequently nutrient and carbon cycling in permafrost soils (Peplau et al. Citation2022). This may be favorable for subsequent agricultural production but also problematic regarding the release of CO2 and CH4, contributing to increases in global emissions (Strauss et al. Citation2021).
Figure 1. The upper row shows historic air photos from 1951, 1984, and 2007 (United States Geological Survey Earth Explorer) that illustrate the expansion of agricultural fields of a farm in Fairbanks, Alaska. The expansion of agricultural fields removed the native insulating vegetation and exposed the underlying permafrost to climate-driven thaw. The lower row shows that this permafrost proved to be ice rich from up to 1.05 m of subsidence measured between 2011 and 2017 from Light Detection and Ranging datasets (Alaska Division of Geological and Geophysical Surveys Citation2013).
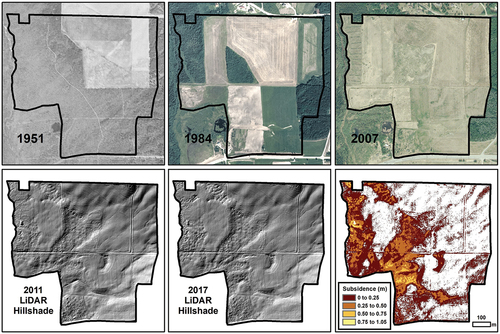
High latitude and mountainous regions are warming at an accelerated rate of two to four times faster than the global average (Arctic Monitoring and Assessment Programme [AMAP], Citation2017; Intergovernmental Panel on Climate Change [IPCC] Citation2019; Rantanen et al. Citation2022). Permafrost temperatures are increasing globally (Biskaborn et al. Citation2019). Land use changes can exacerbate thaw by removing protective native vegetation (Dyrness Citation1982) or disrupting habitat use for pasture and grazing herds (Larsen et al. Citation2016; Eftestøl et al. Citation2019). Permafrost-agroecosystems must be considered in the context of global agricultural systems, particularly in light of the expected northern expansion of agriculture (Ward Jones et al. Citation2022). Permafrost response to human activities also depends on the mode of agriculture itself as, for example, permafrost likely responds differently to crop cultivation than to animal husbandry practices. A better understanding of the diversity of permafrost-agroecosystems and the challenges, adaptations, and opportunities these systems are facing in light of climate, land use, and socioecological changes is urgently needed. Furthermore, globally coordinated research efforts focused on permafrost–agriculture interactions could benefit contemporary and future agricultural activities in high latitude and mountainous regions (Altdorff et al. Citation2021) through knowledge sharing and the development of new datasets for analysis.
In this article we present, as a coordinated effort of the International Permafrost Association’s permafrost-agroecosystem action group, the first global perspective on permafrost-agroecosystems. Our study objectives are to (1) identify data gaps and highlight countries where permafrost-agroecosystems are likely most prominent; (2) demonstrate linkages between agriculture, environmental change (particularly permafrost thaw), livelihoods, regional economies, and policies; and (3) discuss public perceptions of environmental change. We show the diversity of permafrost-agroecosystems through seven regional case studies within the United States, Canada, Russia, Mongolia, Nepal, and northern Fennoscandia. Common themes and differences across permafrost-agroecosystems informed our recommendations to policy makers and land users and aim at solutions that retain permafrost ecosystem services while also allowing for the expansion of permafrost-agroecosystems. Policy outcomes are often viewed as tradeoffs rather than complements.
Identifying countries with permafrost-agroecosystems in light of the lack of pertinent datasets
A complete global analysis of the distribution of agricultural activities in areas containing permafrost is currently impossible. Agricultural datasets in areas of permafrost are either nonexistent or are limited and patchy geographically in their occurrence. Stoessel, Moen, and Lindborg (Citation2022) provide the only known regional geospatial dataset in Fennoscandia, and other regions, such as Alaska, USA (U.S. Department of Agriculture, National Agriculture Statistics Service [USDA NASS], Citation2021) and Canada (Yukon and Northwest Territories) (Statistics Canada Citation2017), have some census-derived statistical datasets. A common dataset used for global agriculture studies is provided by Ramankutty et al. (Citation2008; Citation2010a, Citation2010b); however, its coverage is patchy and incomplete regarding permafrost-agroecosystems. While this dataset has some coverage in Central Asia, the European Alps and northern-central Canada, there are many areas, such as Alaska and most of Russia, that do not contain any data at all despite having known farmland, including in areas containing permafrost. Furthermore, the definitions of pastureland and/or silvopastoral agroforestry systems do not account for reindeer herding, which is practiced widely in Eurasia with an estimated 100,000 herders and 2.5 million semidomesticated reindeer (Magga et al. Citation2009).
As a first global estimate of countries likely to have the largest area of permafrost-agroecosystems, we assessed permafrost datasets for global coverage (Obu et al. Citation2019a, Citation2019b; Gruber Citation2012) to identify and calculate the percentage of land surface within the continuous, discontinuous, sporadic, or isolated permafrost zone for each country (). Due to the low coverage of permafrost in the Southern Hemisphere (0.15 percent; Gruber Citation2012) we focus on the Northern Hemisphere. We use Obu et al. (Citation2019a) for its higher-quality model outputs of permafrost occurrence when comparing outputs to regional field observations. The following analysis was done in ArcGIS Pro v. 2.7 (©Esri): each of the four permafrost zones was divided into its own layer and the “intercept” tool was used to clip each area per country. Permafrost zone area was calculated and exported out to Excel.
Figure 2. Above shows the percentage of the total permafrost land per country for countries with permafrost representing 10 percent or more of their areas. Permafrost dataset (Obu et al. Citation2019a) based on modeling ground temperatures at the top of permafrost between 2000 and 2016. The graph also shows total agricultural land (defined as arable land and/or permanent pastures) in 2016 (https://datacatalog.worldbank.org/home). Below shows the total permafrost area divided into permafrost zones based on the lateral continuity of permafrost. While the likelihood of permafrost occurrence increases with increasing permafrost area and agricultural activities present, the exact location is unknown due to nonexistent and patchy datasets. *Nonglaciated areas. **Includes Svalbard within calculation of permafrost area but not in agricultural land, as there are currently no known agricultural activities on Svalbard.
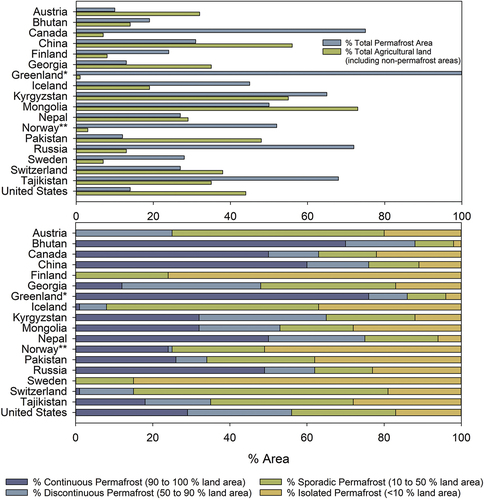
Figure 3. Distribution of permafrost within the Northern Hemisphere and locations of case studies. Geospatial data from Obu et al. Citation(2019a). Countries with permafrost representing 10 percent or more of their territories, identified in , are shaded light gray. The northern Fennoscandia study area was mapped based on Stoessel, Moen, and Lindborg (Citation2022). This analysis serves as a proxy for identifying areas with a greater likelihood of containing permafrost-agroecosystems, as the exact locations are currently unknown due to patchy or nonexistent datasets. Such geospatial datasets would allow monitoring of any growth and decline of agricultural activities; measure and monitor environmental changes, such as changes in vegetation, thermokarst, and carbon cycling; and could aid in policy development and governance.
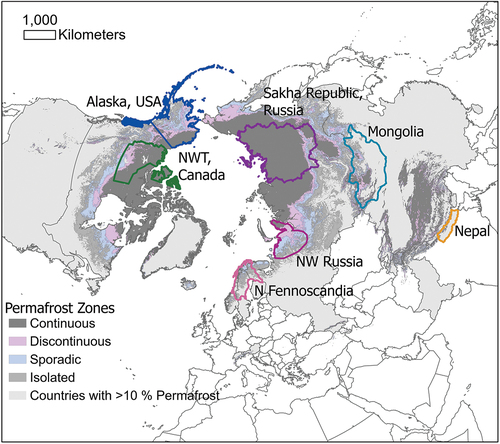
We selected countries with more than 10 percent of their landmass within any of the four permafrost zones, identifying 18 countries (): Austria, Bhutan, Canada, China, Finland, Georgia, Greenland, Iceland, Kyrgyzstan, Mongolia, Nepal, Norway, Pakistan, Russia, Sweden, Switzerland, Tajikistan, and the United States. All (except Austria, Finland, and Sweden) contain areas within all four permafrost zones. Each country has an active agriculture industry, as found in the World Bank Data Catalog (https://datacatalog.worldbank.org/home) () but quantifying the total amount of agricultural activities in areas containing permafrost is unknown due to limited and patchy datasets. Therefore, it is currently impossible to not only determine the location of permafrost-agroecosystems, which can help inform governance and policies, but also to monitor any growth or decline of agricultural activities on permafrost. Geospatial datasets would allow for upscaling of field-scale carbon measurements from permafrost soils cleared for agriculture to estimate their impact on carbon cycling (e.g., Grünzweig et al. Citation2004; Grünzweig, Valentine, and Chapin Citation2015; Peplau et al. Citation2022) and coupled with remote sensing analysis would allow the monitoring of changing environmental conditions such as shifts in vegetation that impact grazing and land clearing for cultivation and signs of thermokarst development (e.g., pond formation and subsidence).
Case studies of socioecological interactions of permafrost-agroecosystems
The condition and productivity of permafrost-agroecosystems are vulnerable to global warming trends and human land use practices and policies. These drivers cause significant impacts at multiple spatial and temporal scales. The representative selection of seven case studies below provides specific examples, not direct analytical comparisons, showcasing the diversity of permafrost-agroecosystems as well as the range of challenges and opportunities emerging from changing permafrost and environmental conditions (), changing industries, and government policies. Common themes identified between case studies, such as perceptions of environmental change, are discussed in section 4.0. The case studies were selected based on active and past research by coauthors. Data presented without citations represents original research results. Each region (Alaska, USA; Northwest Territories, Canada; Sakha Republic, Russia; Mongolia; Nepal; northern Fennoscandia; and northwest Russia) ( and ) is undergoing rapid warming, up to 3 to 4 times faster than the global average (AMAP, Citation2017; IPCC Citation2019; Rantanen et al. Citation2022).
Table 1. Overview of case studies and recent mean annual air temperature warming trends.
Alaska, USA: Crop cultivation in areas of discontinuous permafrost
The spread of conventional agricultural practices was introduced in Alaska in response to the Gold Rush when the U.S. government sent U.S. agriculture agent Charles C. Georgeson to the state to determine if there was any agriculture and horticulture potential (University of Alaska Fairbanks Agricultural and Forestry Experiment Station Citation1998). Georgeson established eight agricultural research stations across Alaska, starting in 1898, with two of the stations established on areas with permafrost: the Rampart Station, active between 1900 and 1925, and the Fairbanks Station established in 1906, still active and now belonging to the University of Alaska Fairbanks. At one time, in the mid 1950s, the state produced approximately 55 percent of its food (Snyder and Meter Citation2015). However, as imports became more reliable and less expensive than locally produced foods this self-sufficiency declined with time, and the state now imports an estimated 95 percent of its purchased foods (Meter and Goldenberg Citation2014). In recent decades, agriculture in Alaska has been characterized by small farms— owned and operated by individuals and families, with the majority of these farms (67 percent) operating on less than 50 acres (~20 ha) (USDA NASS, Citation2019). Farm numbers have been increasing in recent decades, contributing to increased local food security and access. Between 2012 and 2017, farm numbers increased in Alaska by 30 percent while simultaneously decreasing by 3 percent in the continental United States (USDA NASS, Citation2019). Approximately 80 percent of the state is underlain by permafrost that is ice rich in many areas (Jorgenson et al. Citation2008).
Although agriculture in Alaska has existed for over a century, knowledge gaps persist and resources for cultivating on permafrost-affected soils are lacking; many farmers are thus compelled to manage their fields by trial and error. In 2022, a survey was distributed online via Qualtrics and follow-up in-person interviews were conducted by members of the project research team with twelve Alaskan farmers collaborating on the U.S. National Science Foundation Permafrost Grown Project (U.S. NSF, Citation2021), eleven of which were based within the Tanana Valley of Interior Alaska (one of three agricultural hubs in the state) and one in Bethel. The survey asked questions about issues related to permafrost thawing as well as experience and/or knowledge of permafrost thaw management strategies.
Two farmers described benefits of cultivation on permafrost, including less need for irrigation as some ground ice melt produces wetter soils and accessible water supplies to fields from nearby thaw ponds. These benefits, however, are temporary and are being lost when widespread surface subsidence occurs. According to survey participants, the three most common problems relating to permafrost thaw are subsidence, changes in surface water flow, and issues related to fencing, primarily the leaning of fence posts as ice-rich permafrost thaws. Subsidence can prevent continuation of mechanized cultivation until subsided areas are graded. Fencing is often used to keep large herbivores, like moose, away from crops. Leaning fence posts on permafrost allow wildlife to enter fields, causing crop damage. Other problems related to changes in the thermal and hydrological regimes of permafrost thaw, particularly from subsidence, include not being able to plant certain crops, delayed planting and crops being killed, as well as impacts to infrastructure like barns and root cellars, and equipment like tractors getting stuck in muddy areas affected by thermokarst. The above challenges individually or in concert with one another have led to partial field abandonment for seven farmers and complete farm abandonment for one.
Although some farmers felt they had little knowledge of permafrost, eight claimed to have a moderate amount, and one said they had advanced knowledge of permafrost. Nevertheless, almost half of respondents reported feeling uninformed or unaware of any permafrost management strategies for agricultural practices. Those who claimed familiarity with some permafrost management strategies described grading the ground to level areas that had subsided, moving perennial crops out of sinking and wet areas, and utilizing no-till practices to minimize soil disturbance. Managing permafrost in farm fields can be costly; one farmer reported expending as much as 20 percent of their annual budget to manage permafrost-related disturbance through soil leveling and grading.
Despite efforts to manage permafrost issues, some farmers may inadvertently cause permafrost to thaw () by using generally recommended practices for cultivating in cold soils, such as the use of high tunnels and plastic mulches to warm soil and manage weeds. Preliminary data (Ward Jones, Gannon, and Jones Citation2024) () collected in Alaska for a range of crop and mulch types show how certain plastic mulch applications can warm soils at greater depths. For instance, a black poly-geotextile mulch applied for weed suppression warmed the soil surface at an average rate of ~0.1°C/day, and increased soil temperatures by 1.5°C at 1 m depth during the period the plastic was applied (). These common recommended practices for cultivating in colder climates have not been evaluated for their impact on permafrost: early findings from the study described above demonstrate a clear knowledge gap and need for additional research, as well as the development and adoption of management strategies that mitigate permafrost thaw.
Figure 4. (A) Plastic mulch application caused a thermokarst pit to develop along a fencepost at a farm in Fairbanks, Alaska. (B) The impact of soil heating from a 22-day geotextile mulch application during the 2022 growing season. (Data available at Ward Jones, Gannon, and Jones Citation2024.) Ground heated as much as 7.6°C at the surface and 1.5°C at 1 m depth compared to a carrot crop with no mulch.
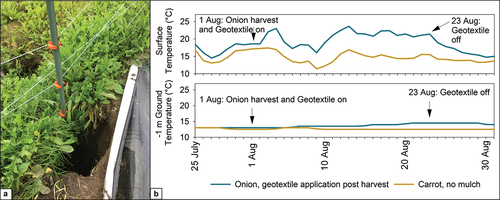
Northwest Territories, Canada: Indigenous traditional food and crop cultivation
Canada’s Northwest Territories (NWT) are ecologically dominated by boreal forest ecosystems, of which 70 percent are underlain by permafrost. Indigenous communities continue to rely on the animal and plant resources of this ecosystem to support cultural, economic, and nutritional values.
The impacts of permafrost thaw are not uniform across the territory, as the severity of change depends on the diverse ice content of the substrate. However, this fundamental change in land cover and ecology leads to issues of food access and availability for many communities in the NWT. Retrogressive thaw slumps created by thawing ice-rich permafrost can degrade water bodies, impacting aquatic ecosystems and fish populations (Kokelj and Jorgenson Citation2013). Large-scale conversion of forested ecosystems to wetlands will decrease caribou habitat through loss of spruce-lichen forests (Joly, Duffy, and Rupp Citation2012). Furthermore, changes in topography can make it more difficult for harvesters to access the land, and uneven terrain can contribute to travel safety concerns (Kokelj and Jorgenson Citation2013; IPCC Citation2019).
As climate change continues to impact food systems in the NWT, there may be more opportunities for local agricultural production, as temperatures warm and ecosystems shift (Hannah et al. Citation2020). The NWT has a mixed history of agriculture beginning with its introduction by early settlers, fur traders, and missionaries in the eighteenth century (Piper and Sandlos Citation2007). In the first half of the twentieth century, a small network of experimental farms was established across the NWT where a wide variety of vegetable and grain crops were cultivated before this program was discontinued in the 1960s (Price Citation2023). Since then, food producers in the NWT have continued to develop successful market gardens, vegetable crops, and other agricultural products, such as eggs, mushrooms, tea, and honey. Today, small-scale subsistence agriculture is common in communities throughout the NWT () (Chen and Natcher Citation2019) and commercial agriculture is emerging as a viable operation (Lemay et al. Citation2021; Radcliffe et al. Citation2021); however, recent flooding and wildfires have destroyed some existing farms. Furthermore, for Indigenous communities across the region, agricultural knowledge and skills are relatively limited, as agriculture has not historically been part of the food system. Moreover, ongoing impacts of colonization on governance, including limited land sovereignty, the legacy of residential schools, and their association with gardens where many were forced to work, affect how farming is perceived among Indigenous communities (Spring et al. Citation2019; Johnston and Spring Citation2021; Price et al. Citation2022).
Figure 5. Raised beds by the Indigenous community of Kakisa (left) and commercial potato farm in Paradise Gardens, NWT (right).
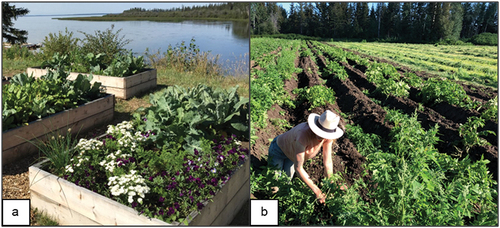
If local food production is to increase in the future, it is important to identify the areas that will become more suitable for agriculture, build capacity to grow food, and develop policies and practices that help protect the land, soils, and atmosphere. For Indigenous communities, it is crucial to ensure that agriculture is developed in a way that meets the needs of residents, is culturally appropriate, and does not diminish the overall health of the ecosystem (Unc et al. Citation2021; Price et al. Citation2022).
Central Sakha Republic, Russia: Horse and cattle husbandry, and hay production
The Sakha Republic (formerly Yakutia), which stretches over 3,000,000 km2, is primarily located in a zone of continuous permafrost with permafrost depths reaching several hundred meters. Smaller areas of discontinuous, sporadic, isolated, and no permafrost are present in southern areas. Permafrost throughout the region is commonly ice-rich.
In this region, agricultural activities occur mainly in both artificial and naturally occurring alaas meadows—areas of shallow depressions resulting either from the degradation of ice-rich permafrost (i.e., thermokarst) or drained lake basins produced from climate warming in previous millennia—most notably, about 12,000 to 9,000 years ago and between about 7,000 and 5,000 years ago (Soloviev Citation1973; Frolova et al. Citation2017; Ulrich et al. Citation2017, Citation2019). As a result of these long-term processes of uneven yet widespread permafrost degradation, steppe-like grasslands in an otherwise boreal forested terrain evolved, providing the preconditions for the horse and cattle economy of the Sakha people to develop over the last 500 to 800 years (). The economic, cultural, and spiritual significance of alaas for Sakha livelihoods in the Sakha region cannot be overemphasized (Crate et al. Citation2017; Mészáros Citation2020; Crate Citation2021).
Figure 6. Alaas amid the forest plateaus on ice-rich permafrost, such open grasslands provide suitable ecological conditions for pasture and hay-making—and thus the basis for the Sakha pastoral culture. Photo: M. Ulrich, July 2014.
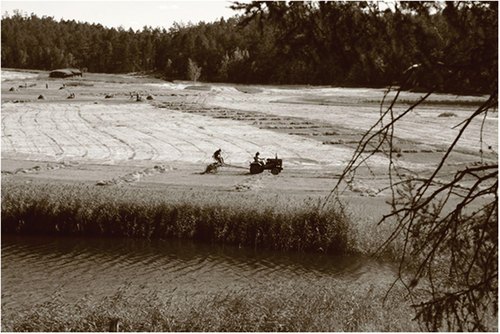
This particular form of land use underwent substantial changes in both pre-Soviet and Soviet times (Crate Citation2006; Crate et al. Citation2017) concerning ownership, the balance between subsistence and market or state-controlled production, technology, and the spatial extent of land use. Expansion of hay production and simultaneously an increasing percentage of arable land for crop farming (located partly in alaas and partly on the forested plateaus underlain with ice-rich permafrost) was made possible with the introduction of tractors () and other mechanization from the mid-1950s to the 1980s. The abrupt disbandment of the state-farm system after the fall of the Soviet Union in the early 1990s resulted in massive unemployment and major loss of food supplies, with most inhabitants developing a form of interhousehold food production described as “cows and kin” (Crate Citation2006, 298). Despite these household-level efforts, cattle numbers decreased from ~400,000 in 1990 to ~235,000 in 2012 (Crate et al. Citation2017). Due to transportation and economic restraints in the 1990s and 2000s, most households sought to use land adjacent to their settlement, resulting in the abandonment of outlying alaas areas.
The speed and the scale of recent permafrost degradation in the Sakha Republic are unprecedented in the scientific record, which is the subject of increasing public concern (Crate and Fedorov Citation2013; Crate Citation2021). An important clarification here is that although Sakha pastoralists actively acquired, expanded, and ameliorated alaas areas as pasture lands and meadows, for example through drainage and selective removal of vegetation (Crate et al. Citation2017), the rate and severity of permafrost degradation occurring today is more widespread and significant than previous episodes of agriculture-induced thaw. Those previous periods of managed permafrost degradation arose from local land-management practices occurring as early as the seventeenth century (Nikolaev Citation2009). These efforts were intensified in the Soviet period with large-scale drainage, irrigation, and logging (Habeck and Yakovlev Citation2019).
Mongolia: Sheep, cattle, horses, camels, yaks, and goats husbandry
Mongolia is another emblematic region of mobile (nomadic) pastoralism located at the southern boundary of the Eurasian permafrost (Endicott Citation2012). Northern Mongolia contains continuous permafrost and highly productive pastures along the main grain belt (Munkhjargal et al. Citation2020; Dashtseren Citation2021). Central Mongolia is underlain by discontinuous permafrost with sporadic and isolated permafrost patches in the south. Forest areas are distributed in a mosaic pattern (often on north-facing slopes), covering about 8 percent of the country, and overlapping considerably with permafrost areas.
Mobile pastoralism is culturally the most prominent activity in Mongolia and despite a certain decrease over the last 20 years, it continues to be one of the most important economic sectors, along with mining. There are approximately 300,000 pastoral households in Mongolia who mainly depend on their livestock for income and feed a large part of the country’s population. The six prominent livestock species are sheep, cattle, horses, camels, yaks, and goats. Overall, the number of livestock in Mongolia doubled between 1995 and 2015 (Gankhuyag et al. Citation2021); however, large-scale livestock die-off events do occur because of climatic events (called dzuds) such as drought, extended snow cover, and extreme cold temperatures (Pan et al. Citation2019). Herd composition varies based on the ecoregion: for example, yaks play an important role in the cooler mountain ranges of the western part (). Animal husbandry is also influenced by global trends of commerce and consumer choices (Janzen Citation2011; Ichinkhorloo Citation2018), most prominently seen in the increase of cashmere goats since the early 2000s. Because goats tend to exert a stronger impact on plant roots than do sheep, the increase in goats has exacerbated pasture degradation.
Figure 7. Yak and cattle spending a spring afternoon by an aufeis sheet in a valley near Terelj, Mongolia. Photo: J. O. Habeck, March 2019.
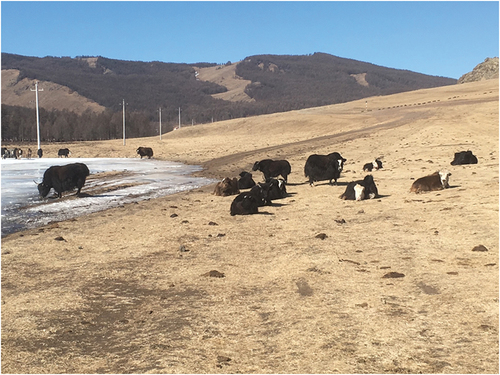
One case portrays Khuder District, a part of Mongolia where logging is an economic priority that concerns the interaction of mobile pastoralism and permafrost. The district is located in north-central Mongolia (the Selenge Region) just south of the Russian border at the margins of the continuous permafrost zone. In line with economic shifts from a socialist agropastoralism economy toward gold and iron-ore mining, commerce, and services, it is now logging—in particular illegal logging—that creates the most palpable environmental problems (Munkherdene and Sneath Citation2018). Forest cover helps preserve permafrost through effects of shade: recent borehole temperature monitoring in this region shows the forest and mountain sites to be most thermally stable (Wang et al. Citation2022). Therefore, if today’s rates of logging continue, it will have a severe impact on permafrost dynamics as it removes the protective cover and exposes permafrost to climate-driven thaw.
Permafrost thaw associated with logging or forest fires also impacts the region’s hydrological conditions; as permafrost is impermeable, water flow occurs within the summer thaw layer (Ishikawa et al. Citation2005; Kopp, Minderlein, and Menzel Citation2014; Lange et al. Citation2015). This permafrost aquatard results in more plant water availability in the surface soil and enables the growth of trees (forests) and plants of importance for animal husbandry. Degrading permafrost increases the summer thaw layer so that groundwater is no longer concentrated near the soil surface (Ishikawa et al. Citation2005). At present, this effect of permafrost thaw is less impactful in the Khuder District, where precipitation is abundant enough to generate a high degree of soil moisture, compared to regions further south in Mongolia. Local observations about environmental change suggest that, at present, the effects of the annual temperature and precipitation dynamics seem to obscure the effects of permafrost thaw and the subsequent “drying” of the land. For example, in another region of Mongolia, Khoroo, herders consistently commented on the degradation of their pasturelands and related it to the last several years of drought conditions; however, Han and Menzel (Citation2024) show that thawing permafrost can exacerbate soil drying in drought conditions.
Despite the speed and magnitude of average air temperature rise (), permafrost degradation has been comparatively slow in Mongolia (Wang et al. Citation2022). Such invisible, slow and patchy changes are beyond the perception of the public, even though they are substantially affecting rural livelihoods.
Nepal: Yak pastoralism in the Central Himalayas
Domesticated yaks (and their hybrid forms, cross breeds with other bovines) are raised all over high Mountain Asia, with 90 percent of the approximate total of 2 million found on the Tibetan Plateau in China, and between ~200,000 in the Hindu Kush Himalayan (HKH) ranges outside China (Ning et al. Citation2016a). Their wild counterparts are much rarer (~10,000) and are largely limited to the Tibetan Plateau (Kusi et al. Citation2021). Research on yak husbandry revolves largely around general herd management and associated challenges in the transboundary landscapes of the HKH (Ning et al. Citation2016b) and specific regions in Nepal (Degen et al. Citation2007; Kusi et al. Citation2021) as well as in Bhutan (Ning et al. Citation2016b; Dorji et al. Citation2020), where yak pastoralism continues to be an important source of livelihood and cultural identity. Studies on impacts of a changing climate in the HKH on yak pastoralism are limited, dealing with perceptions of climate change of herders in Sikkim (Feroze et al. Citation2019), as well as the link between glacier change and migration of herders (Meyer et al. Citation2009). While the largely negative impact of changing permafrost on ecosystems and herding in general has been documented in a few cases on the Tibetan Plateau (Baral et al. Citation2023), no study has so far investigated the link between permafrost presence or change and yak pastoralism. Here we present a first analysis on how yak herding and agriculture in high elevation areas of the HKH are potentially exposed to changing permafrost conditions, especially in light of evolving hazards, a concern previously raised by herders in the region (Degen et al. Citation2007).
In this case study we investigate permafrost change and yak herding linkages in three areas in the Nepalese Himalaya: Humla, Langtang, and Yangwarak (, ), where yak pastoralism is common. Based on our field experience from the sites and supported by Google Earth imagery, we identify individual hamlets, connected agricultural lands, and huts used by yak herders (labeled as settlements, agriculture, and transhumance, respectively, in ), and compare this to permafrost extent (Obu et al. Citation2019a), investigating the lowest reach of permafrost in the watershed and the minimum distance of any of the features to permafrost (). The Humla and Langtang areas are generally higher and reach up to and beyond 7,000 m above sea level (a.s.l.), while in Yangwarak the highest elevation is just above 4,600 m a.s.l. No settlements or agricultural land exist in the latter and huts are visited by herders from regions below the minimum elevation of 783 m a.s.l. Nevertheless, in all areas, huts of yak herders are within 1 km of permafrost. In Langtang, nearly half of all huts are even located on terrain that is potentially permafrost. Yaks are left to graze freely in the pasture and return at specific times for milking. Thus, yaks can graze many kilometers beyond the herders’ huts and are therefore likely to move across permafrost terrain frequently in all areas. Agricultural land and settlements are generally further away from permafrost, except in Langtang where permafrost-affected slopes may occur above settlements.
Figure 8. (a) Thaw slumps in the lower permafrost zone in Langtang (~4,000 m a.s.l.) located between frequently used herder huts. (b) Thaw slump close to a hut in Humla. (c) One of the bigger transhumances in Langtang, threatened by debris flows from higher areas on the left and right, now lies abandoned, with the main river arm below. (d) Typical yak grazing grounds in Humla at the lower margin of permafrost occurrence.
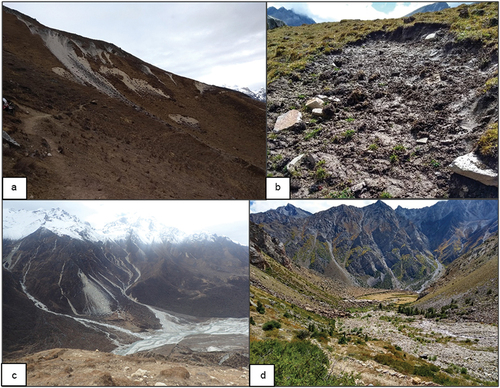
Table 2. Characteristics of the case study sites, distribution of settlement infrastructure, agricultural land and huts (transhumance) for yaks and their herders (with total number in brackets) as well as their respective distances to permafrost.
Glacier and permafrost hazards such as glacial lake outburst floods, snow avalanches, debris flows, and snowstorms have caused loss of livestock repeatedly in the region (Acharya et al. Citation2023; Shrestha et al. Citation2023). yak trails are under the threat of being eroded due to thaw slump events, detachment of the active layer, and debris flows. At the same time, thaw slumps are being used by yaks to scratch themselves, increasing erosion of fragile slopes (). On many occasions, huts of pastoralists had to be shifted after landslides or debris flows, the occurrence of which is potentially linked to changing permafrost conditions (Li et al. Citation2022, ). An indirect risk for yak pastoralism is the impact of permafrost hazards on valley floors, which are used for grazing of other livestock species, and infrastructures such as roads (Fort Citation2015), that facilitate the transportation of agricultural yield and livestock products.
Permafrost degradation and seasonal freeze–thaw cycles are often responsible for changes in plant species distribution and productivity (Schuur et al. Citation2007) and grassland expansion (Jin et al. Citation2022). A precipitation shift from snow to rain potentially contributes to these changes. These variations are likely to impact grazing practices, the nutritional profile of livestock, milk productivity and quality, as well as the relocation of grazing grounds. All these phenomena have been reported by herders at the southern fringe of Langtang as well as in Humla.
Permafrost effects on yak pastoralism will likely have economic consequences, which are currently unknown and merit further research. Nepal currently generates around 20 to 25 million USD per year in export sales alone for dog food from yak milk (Prasain Citation2023) and Yarsagumba, a medicinal plant collected in the same areas as herding, equivalent to approximately 15 percent of the country’s earnings from tourism (Shrestha, Dhital, and Gautam Citation2019).
Effects of changing permafrost in the HKH propagate downward quickly and can impact livelihoods of populations whose settlements are otherwise located outside the zone of continuous permafrost and may even have their continuous settlements more than 2,000 m a.s.l. below the lower margin of permafrost occurrence. The dependence on yak pastoralism, that during summer is carried out in permafrost environments, potentially exacerbates climate-driven thaw, with evidence from all parts of the Nepalese Himalaya.
Berry picking and reindeer herding in Northern Fennoscandia
In Fennoscandia, located mostly in the sporadic permafrost zone, permafrost occurs in the Scandes mountain range, and in less elevated and lowland parts of northern areas (Gisnås et al. Citation2017; Ruuhijärvi, Salminen, and Tuominen Citation2022). In the latter, sporadic permafrost underlies palsas () and peat plateaus (peat mounds and plateaus with perennially frozen cores that contain ice lenses, respectively), which are features of the northern Fennoscandian landscape and are disappearing due to climate change (Näkkäläjärvi, Juntunen, and Jaakkola Citation2022; Leppiniemi et al. Citation2023; Verdonen et al. Citation2023).
Figure 9. Palsa in Peera near Kilpisjärvi, Finland. This palsa is thawing fast; on the sides there are small thermokarst ponds. The top of the palsa rises 1 to 2 meters above peatland surface and creates a rather dry habitat, where lichen and dwarf shrubs are common. As the top of palsa has a thinner snow cover than the surrounding peatland, in spring they are the first snow-free spots in peatland and attract reindeer to graze there. Drone photo: T. Kumpula, August 2023.
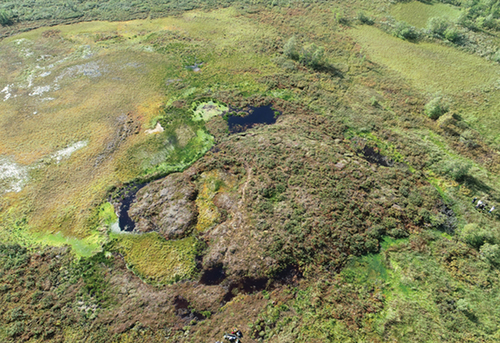
Palsas and peat plateaus are prime growing habitats of and contain substantial coverage of the highly prized cloudberry (Rubus chamaemorus L.). This berry is highly valued by locals for food consumption and to sell to jam companies for commercial production. The berry has an estimated ~2.7-million-euro annual economic value in Finland (Tahvanainen, Miina, and Kurttila Citation2019).
In addition to cloudberries, elevated palsas and peat plateaus serve as “islands” of easily available forage (lichens) for reindeer during winter as they usually have thinner snow cover compared to the surrounding areas (Seppälä Citation1994). While reindeer trampling in winter can help cool permafrost by compacting the insulating snowpack (Windirsch et al. Citation2022), intensive animal trampling in summer may contribute to the degradation of palsas via vegetation transformation, consequently reducing permafrost insulation (Holmgren et al. Citation2023; Windirsch et al. Citation2023).
Approximately 400,000 reindeer spend at least some time of the year within the areas containing permafrost in Fennoscandia (including northern areas of Norway, Sweden, Finland, and Kola Peninsula). Reindeer husbandry plays a central role in the economy and culture of this region. The ancestral lands of the Sámi cross nation-state borders of northern Fennoscandia that these Indigenous groups have been migrating across for millennia. Along with other cultural and socioeconomic aspects, reindeer herding serves as a symbol of Sámi identity (Holand et al. Citation2022). Apart from Sámi, ethnic Finns also practice reindeer husbandry. Sámi and ethnic Finns closely observe the weather, the ground, and pasture quality because seasonal conditions are decisive for all aspects of herding success (Laptander et al. Citation2023). As a livelihood, reindeer husbandry is often seen as competing with other forms of land use, such as the forestry and the energy sectors (Stoessel, Moen, and Lindborg Citation2022), which lead to fragmentation of reindeer pastures and diminished availability of certain resources (Kårtveit Citation2021; Kuokkanen Citation2023). Degradation of palsas and peat plateaus, shrubification of fjell (i.e., mountain tundra) areas, and other climate change–related processes will modify land-based activities (Markkula, Turunen, and Rasmus Citation2019). Current socioeconomic processes in northern Fennoscandia are likely to aggravate the impact of climate change on certain land-based livelihoods. Of utmost importance is thus to (1) maintain flexibility in pastoral land use and (2) endorse the position of diverse rights-holders in decision-making processes about resource use (Komu Citation2020); particularly so in ecologically sensitive areas of the boreal and tundra zone.
Reindeer herding in northwest Russia
The tundra and boreal forest zone between Kanin and Gydan Peninsulas in northwest Russia globally represent the largest reindeer herding region in terms of semidomesticated reindeer numbers, amounting to ~1.1 million, of which ~750,000 are registered in the Yamal Nenets Autonomous Okrug (YNAO) (Istomin and Habeck Citation2016). The latter region has therefore come to be seen as the most important core of north Eurasian reindeer husbandry and has been practicing it for at least 2,000 years (Istomin Citation2020). Most herders define themselves ethnically as Nenets or Komi (Habeck Citation2005; Stammler Citation2005; Laptander Citation2020).
Komi came to engage in reindeer husbandry rather recently, roughly around 1700—a process that by some has been described as an economic response to the Little Ice Age, which wrought disastrous harvests in areas where Komi had earlier depended on cattle farming, hunting, and fishing (Isotomin Citation2020). Komi adapted reindeer-herding practices from their Nenets neighbors. Mixed economy and diversified household income were (and continue to be) of crucial importance for households; however, from the mid-nineteenth century onward, some households with herds >2,000 animals became fully specialized on reindeer husbandry.
This region of northwest Russia spans over a gradient of permafrost zones, from continuous permafrost in the northern half of Yamal Peninsula to discontinuous permafrost in the southern half of Yamal and the Northern Urals, and to sporadic permafrost and isolated patches near Vorkuta toward the southwest.
Reindeer herding () in northwest Russia is a vital source of income for thousands of households and many rural communities in general (in the Komi Republic, the Nenets Autonomous District, and the YNAO). However, the management strategies and herding practices differ significantly not only from those in Fennoscandia (Magga et al. Citation2009), but also from each other. For instance, west of the Urals, transhumance is more common (with several but not all household members spending up to 9 months with the herds), whereas east of the Urals, the numbers of fully nomadic households are much higher. On both sides of the Urals, migration routes between summer and winter pastures can extend up to ~400 kilometers (or 800 kilometers both ways), and generally stretch from north to south, mirroring the zonal differences of the lowland areas and making use of both tundra and forest ecotones. Thus, the herds migrate through all zones, from continuous permafrost to isolated permafrost, twice per year. Furthermore, this part of Russia also has important oil and gas reserves, and expansion of these industries is causing herders to change migration routes (Forbes Citation2013).
Figure 10. Komi reindeer herders driving part of their herd through a spring-thaw freshet (near Khorei-Ver, Russia). Photo: J. O. Habeck, June 1999.
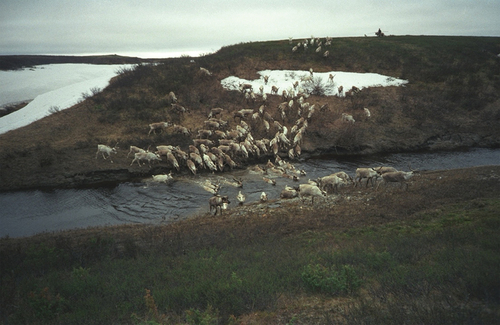
As in Fennoscandia, reindeer herders in Russia are keen observers of weather, ground, and pasture conditions (Laptander et al. Citation2023). From the reindeer herders’ point of view, permafrost dynamics are just one part of the larger context of climate change. Much more worrying to herders in the short term are the effects of rain-on-snow events, often leading to the formation of an ice layer impenetrable for reindeer and resulting in starvation and severe losses for herders (Forbes et al. Citation2016; Golovnev Citation2017; Laptander Citation2020; Stammler and Ivanova Citation2020). It is predominantly through changes in vegetation and thus albedo that reindeer herding has an impact on the soil surface, the active layer, and the permafrost horizon (Istomin and Habeck Citation2016; Holmgren et al. Citation2023). Permafrost degradation, in turn, has local and regional effects on pasture conditions, migration routes, and the timing of migration.
Discussion
Permafrost and its multiple responses to thaw and changing environmental conditions is an essential consideration for high-latitude and mountainous agricultural activities (Vincent, Lemay, and Allard Citation2017; Ward Jones et al. Citation2022). In many cases, agriculturalists’ knowledge of permafrost is limited and the responses of permafrost to management are complex, with linkages between permafrost thaw and environmental change not always obvious. There is nevertheless a general awareness of environmental change among agricultural producers and pastoralists (Poeplau et al. Citation2019). In Mongolia and Nepal, herders are monitoring how their pastures are drying, how vegetation composition is changing, and how more drought-tolerant species are moving in, even though their general awareness of permafrost may be limited. In Alaska, there is an awareness that permafrost is present, but knowledge gaps exist with regards to ground ice, and more generally, what causes the land surface to sink and how to manage it. In the Sakha Republic, Russia, agriculturalists have been actively cultivating areas resulting from thermokarst processes in previous millennia (alaas). However, the current rapid pace of change is creating uncertainty in how to move forward managing these lands for hay production. Therefore, dedicated regional and multinational research funds are needed to create scientific programs involving transdisciplinary research teams (spanning both physical and social sciences) and local experts to gain fundamental knowledge in how to cultivate and manage permafrost-agroecosystems while maintaining the landscapes biodiversity and capacity to store carbon (Unc et al. Citation2021; Ward Jones et al. Citation2022). This knowledge must be codeveloped with Indigenous and local land users, so that permafrost-conscious practices and management strategies can be utilized.
Climate change is creating both opportunities and challenges that vary regionally. The accelerated rate of warming in permafrost regions is potentially driving opportunities for expanded agricultural activities; however, recent agriculture expansion studies do not account for permafrost (King et al. Citation2018; Hannah et al. Citation2020; Fresco et al. Citation2021; KC et al. Citation2021; Čengić et al. Citation2023). Approximately a quarter of the northern hemisphere land area falls within a permafrost region (Obu Citation2021), so its presence and dynamics may become more relevant as agroecosystems intensify, with potential future geographic shifts in food production (Ward Jones et al. Citation2022). Hannah et al. (Citation2020) estimate that high-latitude and mountainous regions will gain the largest land area, over 10 million km2 and ~1 million km2, respectively, under RCP 8.5 by 2060–2080, to grow globally important crops. However, despite the low population density, much of this land is already being used for food production. For example, within North America, vast tracts of land are utilized by Indigenous peoples to access and harvest traditional wild foods. Different land-use practices for food production are likely to conflict with large-scale agricultural development in high-latitude regions, as is occurring with the Nenana-Totchaket Agricultural Project in Alaska (DeMarban Citation2022). In Canada, legacies of colonialism, such as residential schools, and the preponderance of farming as a settlers’ activity, may prevent the desire to adopt and expand agricultural activities. Furthermore, these opportunities may be challenged by problems arising from degrading permafrost that will accelerate as warming continues. While opportunities for agriculture will increase climatically, one cannot expect the scale of cultivation and techniques developed in southern latitudes will automatically work in areas of permafrost due to its different physical properties and processes, especially under thaw conditions (Ward Jones et al. Citation2022), nor are the goals of agricultural production necessarily the same in northern communities (Price et al. Citation2022). Alternatively, climate change is negatively impacting pasture lands through shifts in vegetation composition, such as in Northern Fennoscandia, where permafrost patches provide food sources for reindeer in winter. Pastureland availability is being fragmented or compressed for growing industrial sectors such as logging and mining in areas such as Northern Fennoscandia, northwest Russia, and Mongolia. In high Mountain Asia, climate change has also resulted in the drying of soils where permafrost has thawed while increasing mass movements like landslides and debris flows, causing people to relocate for a lack of adequate and safe agricultural space (Baral et al. Citation2023).
These potentially competing land interests between growing industry sectors and traditional livelihoods will, in face of environmental changes, drive tradeoffs between individual livelihoods and local, regional, and national economic interests. Furthermore, while climatic opportunities may increase food production in high latitude and mountainous areas, these opportunities also come at the cost of tradeoffs with other ecosystem services and environmental consequences (Klöffel et al. Citation2022). Many of these tradeoffs are yet to be fully assessed; for instance, how Indigenous or pastoralist land-use practices are likely to be impacted by future expansion of industrial activities. In particular, land use changes that result in an increase in provisioning ecosystem services may result in a loss of biodiversity, increased carbon emissions from newly cultivated land, and shifts in downstream nutrient concentrations (King et al. Citation2018; Hannah et al. Citation2020; Unc et al. Citation2021). So far, only a few studies have investigated such mechanisms, but they share the insight that carbon losses from permafrost soils upon deforestation are much higher than those from nonpermafrost soils (Grünzweig, Valentine, and Chapin Citation2015; Peplau et al. Citation2022). Research involving agriculturalists and pastoralists, especially those who are trained and situated locally, is needed for high latitude and mountainous communities to develop effective solutions (Ward Jones et al. Citation2022) that are supported by local land use policies (Unc et al. Citation2021). Furthermore, policy makers can be proactive in developing policies that minimize potential future conflicts and take into account tradeoffs between agricultural expansion and permafrost protection. These policies must be formulated in cooperation with local communities, rightsholders, and stakeholders.
Additional actions policy makers can take to support permafrost-agroecosystems include devoting resources to create baseline datasets (both census and geospatial) related to permafrost-agroecosystem location, agricultural activity type, agriculturalist demographics, and other social–ecological characteristics. Policy makers should also be mindful that permafrost presence can be viewed ambivalently, as an asset or a burden (Ward Jones et al. Citation2022), and the varying policies that result from this ambivalence do not necessarily exclude each other; rather, they may coexist. In mountainous areas, thawing permafrost can create hazards, such as from landslide wasting events. Also, subsidence and other permafrost effects can lead to crop failure and economic losses due to the absence of relief programs and lack of insurance coverage for permafrost-related damages. Policies, including financial incentives, can be developed to support permafrost preservation; for instance, through permafrost-conscious cultivation practices that are developed to suit local conditions. Another opportunity could be expanding climate-smart agricultural practices to include permafrost and extend carbon credit programs that support permafrost preservation by cultivation and pastoralism. Regardless, developing effective permafrost-conscious cultivation and pastoral practices is only possible if dedicated scientific efforts endorse long-term transdisciplinary research programs in cooperation with local agriculturalists.
Conclusion
Climate change will shift agricultural production globally, especially in high latitude and mountainous regions that are commonly underlain by permafrost and expected to experience the largest gains in arable land area. However, it is currently impossible to incorporate these regions in global analysis and monitor these environmental shifts as geospatial datasets of permafrost-agroecosystems are nonexistent or are limited and patchy in occurrence. We identified 18 countries in the Northern Hemisphere whose territory contains 10 percent or more land within a permafrost region. Each country identified also has an active agricultural sector, although the extent of agricultural activities in permafrost-containing areas globally is currently unknown.
The creation of datasets and dedicated research programs is necessary to better understand permafrost-agroecosystems, so they can be sustainably managed and developed as new areas of land become better suited to agricultural activities and some areas decline with permafrost degradation. Permafrost conditions vary geographically, with ground ice content being a primary control. Therefore, the environmental and societal impacts of thawing permafrost on agricultural activities will vary geographically and have different responses depending on permafrost type. For example, thawing ice-rich permafrost leads to increased soil moisture content and waterlogging, whereas permafrost with poor ice content is associated with land drying; both responses further generate complex cascading interactions and feedback within their respective environment. Our case studies show the complex socioecological linkages between agricultural activities and environmental changes arising from permafrost thaw and climate change. Although there is diversity between the seven regional agroecosystem case studies, we found many common themes, such as an overall awareness of environmental changes and permafrost knowledge gaps. Accounting for these complex socioecological linkages and ecosystem and land use tradeoffs while filling in knowledge gaps is needed to sustainably manage and develop locally relevant, climate-positive permafrost-agroecosystems within the context of globally changing climate, policy, industry, and economies.
Author contributions and divisions of labor
This article represents a coordinated effort by the International Permafrost-Agroecosystem Action Group. The initial ideas, conceptualization, and discussion of the article were discussed during virtual group meetings. Individual case studies were led and written by subteams within the authorship team and then completed drafts of the article were revised by the whole authorship team. The order of authorship reflects a combination of the number of tasks contributed by a coauthor as well as the order of case study subteams that generally match the order of case studies in the article. Specific tasks are as follows: MWJ led coordination and revisions of the article, conducted the analysis in section “Identifying countries with permafrost-agroecosystems” in light of the lack of pertinent datasets contributed first drafts of text outside the case studies, and contributed to the Alaska case study. JOH contributed the northwest Russia, and, with MU and SC, to the Sakha Republic, Russia, and Mongolia cases studies. GG, TS, BJ, MK, YS, BG, and NP contributed to the Alaska case study. PB, AM, and JS, contributed to the Nepal case study. AS, MJP, and DB contributed to the NWT, Canada case study. BF, MV, TK, JS, and TW contributed to the northern Fennoscandia and northwest Russia case studies. CP, FT, MT, SG, AU, and NB contributed text outside case studies sections.
Acknowledgments
This article is a coordinated effort of the Permafrost-Agroecosystem Action Group, supported by the International Permafrost Association. We thank the two anonymous reviewers for their comments and feedback that improved the final article.
Disclosure statement
No potential conflict of interest was reported by the author(s).
Additional information
Funding
References
- Acharya, A., J. F. Steiner, K. M. Walizada, S. Ali, Z. H. Zakir, A. Caiserman, and T. Watanabe. 2023. Snow and ice avalanches in high mountain Asia – Scientific, local and indigenous knowledge. Natural Hazards and Earth System Sciences 23 (7): 2569–23.
- Altdorff, D., N. Borchard, E. H. Young, L. Galagedara, J. Sorvali, S. Quideau, and A. Unc. 2021. Agriculture in boreal and Arctic regions requires an integrated global approach for research and policy. Agronomy for Sustainable Development 41 (2): 1–13. doi:10.1007/s13593-021-00676-1.
- Arctic Monitoring and Assessment Programme. 2017. Snow, Water, Ice and Permafrost in the Arctic (SWIPA). Oslo, Norway: Arctic Monitoring and Assessment Programme.
- Baral, P., S. Allen, J. F. Steiner, T. R. Gurung, and G. McDowell. 2023. Climate change impacts and adaptation to permafrost change in High Mountain Asia: A comprehensive review. Environmental Research Letters 18: 093005.
- Biskaborn, B. K., S. L. Smith, J. Noetzli, H. Matthes, G. Vieira, D. A. Streletskiy, P. Schoeneich, et al. 2019. Permafrost is warming at a global scale. Nature Communications 10 (1): 264. doi:10.1038/s41467-018-08240-4.
- Čengić, M., Z. J. N. Steinmann, P. Defourny, J. C. Doelman, C. Lamarche, E. Stehfest, A. M. Schipper, and M. A. J. Huijbregts. 2023. Global maps of agricultural expansion potential at a 300 m resolution. Land 12 (3): 579. doi:10.3390/land12030579.
- Chen, A., and D. Natcher. 2019. Greening Canada’s Arctic food system: Local food procurement strategies for combating food insecurity. Canadian Food Studies/Revue Canadienne Des Études Sur L’alimentation 6 (1): 140–54. doi:10.15353/cfs-rcea.v6i1.301.
- Crate, S. 2021. Once upon the permafrost: Knowing culture and climate change in Siberia. Tucson: University of Arizona Press.
- Crate, S. A. 2006. Investigating local definitions of sustainability in the Arctic: Insights from post-Soviet Sakha villages. Arctic 59 (3): 294–310.
- Crate, S. A., and A. N. Fedorov. 2013. A methodological model for exchanging local and scientific climate change knowledge in northeastern Siberia. Arctic 66 (3): 338–50. doi:10.14430/arctic4312.
- Crate, S., M. Ulrich, J. O. Habeck, A. R. Desyatkin, R. V. Desyatkin, A. N. Fedorov, T. Hiyama, et al. 2017. Permafrost livelihoods: A transdisciplinary review and analysis of thermokarst-based systems of indigenous land use. Anthropocene 18: 89–104.
- Czerniawska, J., and J. Chlachula. 2020. Climate-change induced permafrost degradation in Yakutia, East Siberia. Arctic 73 (4): 509–28. doi:10.14430/arctic71674.
- Dashtseren, A. 2021. Permafrost in Mongolia. In The Physical Geography of Mongolia, ed. B. Yembuu, 119–33. Cham: Springer International Publishing.
- Degen, A. A., M. Kam, S. B. Pandey, C. R. Upreti, S. Pandey, and P. Regmi. 2007. Transhumant pastoralism in yak production in the Lower Mustang District of Nepal. Nomadic Peoples 11 (2): 57–85. doi:10.3167/np.2007.110204.
- DeMarban, A. 2022. Tribe protests effort to develop agricultural project in wilderness west of Nenana. Anchorage Daily News, November 4, 2022. https://www.adn.com/alaska-news/2022/11/03/tribe-protests-effort-to-develop-agricultural-project-in-wilderness-west-of-nenana/
- Desyatkin, R., N. Filippov, A. Desyatkin, D. Konyushkov, and S. Goryachkin. 2021. Degradation of arable soils in Central Yakutia: Negative consequences of global warming for Yedoma landscapes. Frontiers in Earth Science 9:795.
- DGGS Staff. 2013. Elevation datasets of Alaska: Alaska division of geological & geophysical surveys digital data series 4, https://elevation.alaska.gov/
- Dhital, Y. P., S. Jia, J. Tang, X. Liu, X. Zhang, R. R. Pant, and B. Dawadi. 2023. Recent warming and its risk assessment on ecological and societal implications in Nepal. Environmental Research Communications 5 (3): 031010. doi:10.1088/2515-7620/acc56e.
- Dorji, N., M. Derks, P. Dorji, P. W. G. Groot Koerkamp, and E. A. M. Bokkers. 2020. Herders and livestock professionals’ experiences and perceptions on developments and challenges in yak farming in Bhutan. Animal Production Science 60 (17): 2004–20. doi:10.1071/AN19090.
- Dyrness, C. T. 1982. Control of depth to permafrost and soil temperature by the forest floor in black spruce/feathermoss communities, Vol. 396. U.S. Department of Agriculture, Forest Service, Pacific Northwest Forest and Range Experiment Station.
- Eftestøl, S., K. Flydal, D. Tsegaye, and J. E. Colman. 2019. Mining activity disturbs habitat use of reindeer in Finnmark, Northern Norway. Polar Biology 42 (10): 1849–58. doi:10.1007/s00300-019-02563-8.
- Endicott, E. 2012. Agriculture, mining, tourism: Competing interests in land use. In A history of land use in Mongolia: The thirteenth century to the present, ed. E. Endicott, 129–57. New York: Palgrave Macmillan US.
- Etzelmüller, B. 2013. Recent advances in mountain permafrost research. Permafrost and Periglacial Processes 24 (2): 99–107. doi:10.1002/ppp.1772.
- Feroze, S. M., L. I. Ray, K. J. Singh, and R. Singh. 2019. Pastoral yak rearing system is changing with change in climate: An exploration of North Sikkimin Eastern Himalaya. Climatic Change 157 (3): 483–98. doi:10.1007/s10584-019-02551-1.
- Forbes, B. C. 2013. Cultural resilience of social-ecological systems in the Nenets and Yamal-Nenets Autonomous Okrugs, Russia: A focus on reindeer nomads of the tundra. Ecology and Society 18 (4). doi:10.5751/ES-05791-180436.
- Forbes, B. C., T. Kumpula, N. Meschtyb, R. Laptander, M. Macias-Fauria, P. Zetterberg, M. Verdonen, et al. 2016. Sea ice, rain-on-snow and tundra reindeer nomadism in Arctic Russia. Biology Letters 12 (11):20160466. doi:10.1098/rsbl.2016.0466.
- Fort, M. 2015. Natural hazards versus climate change and their potential impacts in the dry, Northern Himalayas: Focus on the Upper Kali Gandaki (Mustang District, Nepal). Environmental Earth Sciences 73 (2): 801–14. doi:10.1007/s12665-014-3087-y.
- Fresco, N., A. Bennett, P. Bieniek, and C. Rosner. 2021. Climate change, farming, and gardening in Alaska: Cultivating opportunities. Sustainability 13 (22): 12713. doi:10.3390/su132212713.
- Frolova, L. A., A. G. Ibragimova, M. Ulrich, and S. Wetterich. 2017. Reconstruction of the history of a thermokarst lake in the Mid-Holocene based on an analysis of subfossil Cladocera (Siberia, Central Yakutia). Contemporary Problems of Ecology 10 (4): 423–30. doi:10.1134/S1995425517040023.
- Gankhuyag, G., F. Ceacero, A. Yansanjav, P. Hejcmanová, L. Davaa, S. Namkhaidorj, and B. Č. Bolfíková. 2021. Long-term trends in livestock and wildlife interactions: Do livestock numbers predict recent trends of wolves, foxes, and rodents’ populations in Mongolian rangelands? Journal for Nature Conservation 60: 125969. doi:10.1016/j.jnc.2021.125969.
- Gisnås, K., B. Etzelmüller, C. Lussana, J. Hjort, A. B. K. Sannel, K. Isaksen, S. Westermann, et al. 2017. Permafrost map for Norway, Sweden and Finland. Permafrost and Periglacial Processes 28 (2): 359–78. doi:10.1002/ppp.1922.
- Golovnev, A. V. 2017. Challenges to Arctic nomadism: Yamal Nenets facing climate change era calamities. Arctic Anthropology 54 (2): 40–51. doi:10.3368/aa.54.2.40.
- Gruber, S. 2012. Derivation and analysis of a high-resolution estimate of global permafrost zonation. The Cryosphere 6: 221–33. doi:10.5194/tc-6-221-2012.
- Grünzweig, J. M., S. D. Sparrow, D. Yakir, and F. Stuart Chapin III. 2004. Impact of agricultural land‐use change on carbon storage in boreal Alaska. Global Change Biology 10 (4): 452–72. doi:10.1111/j.1365-2486.2004.00738.x.
- Grünzweig, J. M., D. W. Valentine, and F. S. Chapin. 2015. Successional changes in carbon stocks after logging and deforestation for agriculture in interior Alaska: Implications for boreal climate feedbacks. Ecosystems 18 (1): 132–45. doi:10.1007/s10021-014-9817-x.
- Habeck, J. O. 2005. What it means to be a herdsman: The practice and image of reindeer husbandry among the Komi of northern Russia. Münster: LIT.
- Habeck, J. O., and I. A. Yakovlev. 2019. Land improvement under conditions of permafrost: Melioratsiia and intended forms of environmental change in Soviet Yakutia. Northeast Asian Studies 23: 71–92.
- Han, L., and L. Menzel. 2024. Hydrological response to long-lasting dry spell at the southern edge of Siberian permafrost. Science of the Total Environment 922: 171330. doi:10.1016/j.scitotenv.2024.171330.
- Hannah, L., P. R. Roehrdanz, K. C. Krishna Bahadur, E. D. Fraser, C. I. Donatti, L. Saenz, T. M. Wright, et al. 2020. The environmental consequences of climate-driven agricultural frontiers. PLOS ONE 15 (2): e0228305. doi:10.1371/journal.pone.0228305.
- Holand, Ø., T. Horstkotte, J. Kumpula, and J. Moen. 2022. Reindeer pastoralism in Fennoscandia. In Reindeer husbandry and global environmental change, ed. T. Horstkotte, Ø. Holand, J. Kumpula, and J. Moen, 7–47. New York: Routledge.
- Holmgren, M., F. Groten, M. R. Carracedo, S. Vink, and J. Limpens. 2023. Rewilding risks for peatland permafrost. Ecosystems 26 (8): 1806–18. doi:10.1007/s10021-023-00865-x.
- Ichinkhorloo, B. 2018. Collaboration for survival in the age of the market: Diverse economic practices in postsocialist Mongolia. Central Asian Survey 37 (3): 386–402. doi:10.1080/02634937.2018.1501347.
- Intergovernmental Panel on Climate Change. 2019. IPCC special report on the ocean and cryosphere in a changing climate, 755. [H.-O. Pörtner, D.C. Roberts, V. Masson-Delmotte, P. Zhai, M. Tignor, E. Poloczanska, K. Mintenbeck, A. Alegría, M. Nicolai, A. Okem, J. Petzold, B. Rama, N.M. Weyer (eds.)]. Cambridge, UK: Cambridge University Press. doi:10.1017/9781009157964.
- International Permafrost Association. 1998. Multi-language glossary of permafrost and related ground-ice terms. Edited by R.O. van Everdingen. Calgary: Arctic Institute of North America. Version 2.0.
- Ishikawa, M., N. Sharkhuu, Y. Zhang, T. Kadota, and T. Ohata. 2005. Ground thermal and moisture conditions at the southern boundary of discontinuous permafrost, Mongolia. Permafrost and Periglacial Processes 16 (2): 209–16. doi:10.1002/ppp.483.
- Istomin, K. V. 2020. Post-Soviet reindeer herders: Between family and collective herding. Region 9 (1): 25–52. doi:10.1353/reg.2020.0005.
- Istomin, K. V., and J. O. Habeck. 2016. Permafrost and indigenous land use in the northern Urals: Komi and Nenets reindeer husbandry. Polar Science 10 (3): 278–87. doi:10.1016/j.polar.2016.07.002.
- Janzen, H. H. 2011. What place for livestock on a re-greening earth? Animal Feed Science and Technology 166: 783–96. doi:10.1016/j.anifeedsci.2011.04.055.
- Jin, X., H. Jin, D. Luo, Y. Sheng, Q. Wu, J. Wu, W. Wang, et al. 2022. Impacts of permafrost degradation on hydrology and vegetation in the source area of the Yellow River on Northeastern Qinghai-Tibet Plateau, Southwest China. Frontiers in Earth Science 10: 845824. doi:10.3389/feart.2022.845824.
- Johnston, C., and A. Spring. 2021. Grassroots and global governance: Can global-local linkages foster food system resilience for small northern Canadian communities? Sustainability 13 (4): 2415. doi:10.3390/su13042415.
- Joly, K., P. A. Duffy, and T. S. Rupp. 2012. Simulating the effects of climate change on fire regimes in Arctic biomes: Implications for caribou and moose habitat. Ecosphere 3 (5): 1–18. doi:10.1890/ES12-00012.1.
- Jorgenson, M. T., K. Yoshikawa, M. Kanevskiy, Y. Shur, V. Romanovsky, S. Marchenko, G. Grosse, J. Brown, and B. Jones. 2008. Permafrost characteristics of Alaska. In Proceedings of the Ninth International Conference on Permafrost. Vol. 3, 121–2. Fairbanks: University of Alaska.
- Kårtveit, B. 2021. Green colonialism. In Stories of change and sustainability in the Arctic Regions: The interdependence of local and global, ed. R. Sørly, T. Ghaya, and B. Kårtveit, 157–77. New York: Routledge.
- KC, K. B., A. G. Green, D. Wassmansdorf, V. Gandhi, K. Nadeem, and E. D. Fraser. 2021. Opportunities and trade-offs for expanding agriculture in Canada’s North: An ecosystem service perspective. FACETS 6 (1): 1728–52. doi:10.1139/facets-2020-0097.
- King, M., D. Altdorff, P. Li, L. Galagedara, J. Holden, and A. Unc. 2018. Northward shift of the agricultural climate zone under 21st-century global climate change. Scientific Reports 8 (1): 1–10. doi:10.1038/s41598-018-26321-8.
- Kivinen, S., S. Rasmus, K. Jylhä, and M. Laapas. 2017. Long-term climate trends and extreme events in Northern Fennoscandia (1914–2013). Climate 5 (1): 16. doi:10.3390/cli5010016.
- Klöffel, T., E. H. Young, N. Borchard, J. D. Vallotton, E. Nurmi, N. J. Shurpali, F. U. Tenorio, X. Liu, G. H. F. Young, and A. Unc. 2022. The challenges fraught opportunity of agriculture expansion into boreal and Arctic regions. Agricultural Systems 203: 103507. doi:10.1016/j.agsy.2022.103507.
- Kokelj, S. V., and M. T. Jorgenson. 2013. Advances in thermokarst research. Permafrost and Periglacial Processes 24, no. 2: 108–19. doi:10.1002/ppp.1779.
- Komu, T. 2020. Pursuing the good life in the north: Examining the coexistence of reindeer herding, extractive industries and nature-based tourism in northern Fennoscandia. Doctoral dissertation, University of Oulu, Finland. https://oulurepo.oulu.fi/bitstream/handle/10024/36672/isbn978-952-62-2502-9.pdf
- Kopp, B. J., S. Minderlein, and L. Menzel. 2014. Soil moisture dynamics in a mountainous headwater area in the discontinuous permafrost zone of northern Mongolia. Arctic, Antarctic, and Alpine Research 46 (2): 459–70. doi:10.1657/1938-4246-46.2.459.
- Krishnan, G., V. Paul, S. S. Hanah, J. Bam, and P. J. Das. 2016. Effects of climate change on yak production at high altitude. The Indian Journal of Animal Sciences 86 (6): 621–6. doi:10.56093/ijans.v86i6.59033.
- Kuhmonen, I., and T. Kuhmonen. 2023. Transitions through the dynamics of adaptive cycles: Evolution of the Finnish agrifood system. Agricultural Systems 206: 103604. doi:10.1016/j.agsy.2023.103604.
- Kuokkanen, R. 2023. Are reindeer the new buffalo? Climate change, the Green Shift, and manifest destiny in Sápmi. Meridians 22 (1): 11–33. doi:10.1215/15366936-10220458.
- Kusi, N., P. Manandhar, H. Senn, J. Joshi, M. Ghazali, K. D. Hengaju, S. P. Suwal, et al. 2021. Phylogeographical analysis shows the need to protect the wild yaks’ last refuge in Nepal. Ecology and Evolution 11 (12): 8310–8. doi:10.1002/ece3.7660.
- Lange, J., B. J. Kopp, M. Bents, and L. Menzel. 2015. Tracing variability of run‐off generation in mountainous permafrost of semi‐arid north‐eastern Mongolia. Hydrological Processes 29 (6): 1046–55. doi:10.1002/hyp.10218.
- Laptander, R. 2020. When we got reindeer, we moved to live to the tundra: The spoken and silenced history of the Yamal Nenets. Doctoral dissertation, University of Lapland, Rovaniemi, Finland. https://luc.finna.fi/ulapland/Record/lauda.10024_64168?sid=3507968563
- Laptander, R., T. Horstkotte, J. O. Habeck, S. Rasmus, T. Komu, H. Matthes, H. Tømmervik, K. Istomin, J. T. Eronen, and B. C. Forbes. 2023. Critical seasonal conditions in the reindeer-herding year: A synopsis of factors and events in Fennoscandia and northwestern Russia. Polar Science 39:101016. doi:10.1016/j.polar.2023.101016.
- Larsen, R. K., K. Raitio, P. Sandström, A. Skarin, M. Stinnerbom, J. Wik-Karlsson, S. Sandström, C. Österlin, and Y. Buhot. 2016. Cumulative effects of exploitations on reindeer husbandry: What needs to be done in permit processes. Report from the Swedish Environmental Protection Agency (Springer).
- Lemay, M. A., J. Radcliffe, D. Bysouth, and A. Spring. 2021. Northern food systems in transition: The role of the emerging agri-food industry in the Northwest Territories (Canada) food system. Frontiers in Sustainable Food Systems 5. doi:10.3389/fsufs.2021.661538.
- Leppiniemi, O., O. Karjalainen, J. Aalto, M. Luoto, and J. Hjort. 2023. Environmental spaces for palsas and peat plateaus are disappearing at a circumpolar scale. The Cryosphere 17 (8): 3157–76. doi:10.5194/tc-17-3157-2023.
- Li, D., X. Lu, D. E. Walling, T. Zhang, J. F. Steiner, R. J. Wasson, S. Harrison, et al. 2022. High Mountain Asia hydropower systems threatened by climate-driven landscape instability. Nature Geoscience 15 (7): 520–30. doi:10.1038/s41561-022-00953-y.
- Maekawa, A. 2013. The cash in cashmere: Herders’ incentives and strategies to increase the goat population in post-socialist Mongolia. The Mongolian Ecosystem Network: Environmental Issues under Climate and Social Changes: 233–45.
- Magga, O. H., S. D. Mathiesen, R. W. Corell, and A. Oskal. 2009. Reindeer herding, traditional knowledge, adaptation to climate change and loss of grazing land. IPY EALÁT Project Executive Summary Report, Arctic Council Sustainable Development working Group. 7th Arctic Council Ministerial Meeting, Nuuk, Greenland.
- MARCC (Mongolia Second Assessment Report on Climate Change). 2014. Mongolia second assessment report on climate change 2014, ed. D. Dagvadorj, Z. Batjargal, and L. Natsagdorj. Ulaanbaatar, Mongolia: Ministry of Environmental and Green Development of Mongolia.
- Markkula, I., M. Turunen, and S. Rasmus. 2019. A review of climate change impacts on the ecosystem services in the Saami Homeland in Finland. Science of the Total Environment 692: 1070–85. doi:10.1016/j.scitotenv.2019.07.272.
- Marshall, K. E., and J. L. Baltzer. 2015. Decreased competitive interactions drive a reverse species richness latitudinal gradient in subarctic forests. Ecology 96 (2): 461–70. doi:10.1890/14-0717.1.
- Mészáros, C. 2020. Arctic pastoralist Sakha: Ethnography of evolution and microadaptation in Siberia. Sibirica 19 (2): 99–103. doi:10.3167/sib.2020.190206.
- Meter, K., and M. P. Goldenberg. 2014. Building food security in Alaska [Report]. Crossroads Resource Center Minneapolis, commissioned by the Alaska Department of Health and Social Services with collaboration from the Alaska Food Policy Council. https://akfoodpolicycouncil.files.wordpress.com/2013/07/14-09-17_building-food-security-in-ak_ken-meter_lr.pdf (accessed July 28, 2014).
- Meyer, M. C., C. Hofmann, A. M. D. Gemmell, E. Haslinger, H. Häusler, and D. Wangda. 2009. Holocene Glacier: Fluctuations and migration of Neolithic Yak pastoralists into the high valleys of Northwest Bhutan. Quaternary Science Reviews 28 (13): 1217–37. doi:10.1016/j.quascirev.2008.12.025.
- Miller, E. W. 1951. Agricultural developments in Interior Alaska. The Scientific Monthly 73 (4):245–54.
- Munkherdene, G., and D. Sneath. 2018. Enclosing the gold-mining commons of Mongolia: The vanishing ninja and the development project as resource. Current Anthropology 59 (6): 814–38. doi:10.1086/700961.
- Munkhjargal, M., G. Yadamsuren, J. Yamkhin, and L. Menzel. 2020. Ground surface temperature variability and permafrost distribution over mountainous terrain in northern Mongolia. Arctic, Antarctic, and Alpine Research 52 (1): 13–26. doi:10.1080/15230430.2019.1704347.
- Näkkäläjärvi, K., S. Juntunen, and J. J. Jaakkola. 2022. Cultural perception and adaptation to climate change among reindeer saami communities in Finland. In Climate cultures in Europe and North America, 103–25. London: Routledge.
- Naumov, A., D. Sidorova, and R. Goncharov. 2022. Farming on Arctic margins: Models of agricultural development in northern regions of Russia, Europe and North America. Regional Science Policy & Practice 14 (1): 174–86. doi:10.1111/rsp3.12273.
- Nikolaev, S. I. 2009. Sakha People. Yakutsk: Yakutsk Territory.
- Ning, W., I. Muhammad, Y. Shaoliang, S. Joshi, F. M. Qameer, and N. Bisht. 2016a. Coping with borders: Yak raising in transboundary landscapes of The Hindu Kush Himalayan Region. In Yak on the move— Transboundary challenges and opportunities for yak raising in a changing Hindu Kush Himalayan Region. Kathmandu, Nepal: International Centre for Integrated Mountain Development.
- Ning, W., Y. Shaoliang, S. Joshi, and N. Bisht. 2016b. Yak on the move: Transboundary challenges and opportunities for yak raising in a changing Hindu Kush Himalayan Region, 3–22. Kathmandu, Nepal: International Centre for Integrated Mountain Development.
- Obu, J. 2021. How much of the earth’s surface is underlain by permafrost? Journal of Geophysical Research: Earth Surface 126, no. 5: e2021JF006123. doi:10.1029/2021JF006123.
- Obu, J., S. Westermann, A. Bartsch, N. Berdnikov, H. H. Christiansen, A. Dashtseren, R. Delaloye, et al. 2019a. Northern Hemisphere permafrost map based on TTOP modelling for 2000–2016 at 1 km2 scale. Earth-Science Reviews 193: 299–316. doi:10.1016/j.earscirev.2019.04.023.
- Obu, J., S. Westermann, A. Kääb, and A. Bartsch. 2019b. Ground temperature map, 2000–2016, Andes, New Zealand and East African Plateau Permafrost. University of Oslo, Pangaea. doi:10.1594/PANGAEA.905512.
- Pan, C. G., J. S. Kimball, M. Munkhjargal, N. P. Robinson, E. Tijdeman, L. Menzel, and P. B. Kirchner. 2019. Role of surface melt and icing events in livestock mortality across Mongolia’s semi-arid landscape. Remote Sensing 11 (20): 2392. doi:10.3390/rs11202392.
- Peplau, T., J. Schroeder, E. Gregorich, and C. Poeplau. 2022. Subarctic soil carbon losses after deforestation for agriculture depend on permafrost abundance. Global Change Biology 28 (17): 5227–42. doi:10.1111/gcb.16307.
- Péwé, T. L. 1954. Effect of permafrost on cultivated fields, Fairbanks area, Alaska (No. 989). US Government Printing Office.
- Piper, L., and J. Sandlos. 2007. A broken frontier: Ecological imperialism in the Canadian North. Environmental History 12 (4): 759–95. doi:10.1093/envhis/12.4.759.
- Poeplau, C., J. Schroeder, E. Gregorich, and I. Kurganova. 2019. Farmers’ perspective on agriculture and environmental change in the circumpolar north of Europe and America. Land 8 (12): 190. doi:10.3390/land8120190.
- Prasain, K. 2023. Dog chew exports likely to hit Rs3 billion this fiscal. The Kathmandu Post. https://kathmandupost.com/money/2023/06/07/dog-chew-exports-likely-to-hit-rs3-billion-this-fiscal
- Price, M. J. 2023. Seeing Green: Lifecycles of an Arctic Agricultural Frontier. Rural Sociology 88 (4): 941–71. doi:10.1111/ruso.12506.
- Price, M. J., A. Latta, A. Spring, J. Temmer, C. Johnston, L. Chicot, J. Jumbo, and M. Leishman. 2022. Agroecology in the North: Centering Indigenous food sovereignty and land stewardship in agriculture “frontiers.” Agriculture and Human Values 39: 1191–206. doi:10.1007/s10460-022-01312-7.
- Radcliffe, J., K. Skinner, A. Spring, L. Picard, F. Benoit, and W. Dodd. 2021. Virtual barriers: Unpacking the sustainability implications of online food spaces and the Yellowknife Farmers market’s response to COVID-19. Nutrition Journal 20 (1): 12. doi:10.1186/s12937-021-00664-x.
- Ramankutty, N., A. T. Evan, C. Monfreda, and J. A. Foley. 2008. Farming the planet: 1. Geographic distribution of global agricultural lands in the year 2000. Global Biogeochemical Cycles 22 (1): GB1003. doi:10.1029/2007GB002952.
- Ramankutty, N., A. T. Evan, C. Monfreda, and J. A. Foley. 2010a. Global agricultural lands: Croplands. Palisades, NY: National Aeronautics and Space Administration Socioeconomic Data and Applications Center.
- Ramankutty, N., A. T. Evan, C. Monfreda, and J. A. Foley. 2010b. Global agricultural lands: Pastures, 2000. Palisades, NY: National Aeronautics and Space Administration Socioeconomic Data and Applications Center. doi:10.7927/H47H1GGR.
- Rantanen, M., A. Y. Karpechko, A. Lipponen, K. Nordling, O. Hyvärinen, K. Ruosteenoja, T. Vihma, and A. Laaksonen. 2022. The Arctic has warmed nearly four times faster than the globe since 1979. Communications Earth & Environment 3 (1): 168. doi:10.1038/s43247-022-00498-3.
- Rockie, W. A. 1942. Pitting on Alaskan farmlands: A new erosion problem. Geographical Review 32 (1): 128–34. doi:10.2307/210363.
- Ruuhijärvi, R., P. Salminen, and S. Tuominen. 2022. Palsasoiden levinneisyys, rakennetyypit ja tila Suomessa 2010-luvulla [Distribution range, morphological types, and state of palsa mires in Finland in the 2010s]. Suo 73 (1): 1–32.
- Schuur, E. A. G., K. G. Crummer, J. G. Vogel, and M. C. MacK. 2007. Plant species composition and productivity following permafrost thaw and thermokarst in Alaskan Tundra. Ecosystems 10 (2): 280–92. doi:10.1007/s10021-007-9024-0.
- Seppälä, M. 1994. Palsan selittäminen–esimerkki teorian muodostuksesta. Terra 106, no. 3: 204–8.
- Shrestha, U. B., K. R. Dhital, and A. P. Gautam. 2019. Economic dependence of mountain communities on Chinese caterpillar fungus Ophiocordyceps sinensis (yarsagumba): A case from western Nepal. Oryx 53 (2): 256–64. doi:10.1017/S0030605317000461.
- Shrestha, F., J. F. Steiner, R. Shrestha, Y. Dhungel, S. P. Joshi, S. Inglis, A. Ashraf, S. Wali, K. M. Walizada, and T. Zhang. 2023. A comprehensive and version-controlled database of glacial lake outburst floods in High Mountain Asia. Earth System Science Data 15 (9): 3941–61. doi:10.5194/essd-15-3941-2023.
- Shur, Y. L., and M. T. Jorgenson. 2007. Patterns of permafrost formation and degradation in relation to climate and ecosystems. Permafrost and Periglacial Processes 18, no. 1: 7–19. doi:10.1002/ppp.582.
- Snyder, E. H., and K. Meter. 2015. Food in the last frontier: Inside Alaska’s food security challenges and opportunities. Environment: Science and Policy for Sustainable Development 57 (3): 19–33.
- Soloviev, P. A. 1973. Thermokarst phenomena and landforms due to frost heaving in Central Yakutia. Biuletyn Peryglacjalny 23: 135–55.
- Spring, A., K. Skinner, M. Simba, E. Nelson, J. Baltzer, H. Swanson, and M. Turetsky. 2019. Taking care of the land: An interdisciplinary approach to community-based food systems assessment in Kakisa, Northwest Territories, Canada. In Sustainable food system assessment: lessons from global practice, ed. A. Blay-Palmer, D. Conaré, K. Meter, and A. Di Battista, 42–65. New York, NY: Routledge.
- Stammler, F. 2005. Reindeer nomads meet the market: Culture, property and globalisation at the “end of the land,” Vol. 6. Münster: LIT Verlag.
- Stammler, F. M., and A. Ivanova. 2020. From spirits to conspiracy? Nomadic perceptions of climate change, pandemics and disease. Anthropology Today 36 (4): 8–12. doi:10.1111/1467-8322.12589.
- Statistics Canada. 2017. Farm and farm operator data: Yukon and the Northwest Territories agricultural trends. https://www150.statcan.gc.ca/n1/pub/95-640-x/2016001/article/14810-eng.htm
- Stépanoff, C. 2017. The rise of reindeer pastoralism in Northern Eurasia: Human and animal motivations entangled. Journal of the Royal Anthropological Institute 23, no. 2: 376–96. doi:10.1111/1467-9655.12612_1.
- Stoessel, M., J. Moen, and R. Lindborg. 2022. Mapping cumulative pressures on the grazing lands of northern Fennoscandia. Scientific Reports 12 (1): 16044. doi:10.1038/s41598-022-20095-w.
- Strauss, J., B. Abbott, G. Hugelius, E. A. G. Schuur, C. Treat, M. Fuchs, C. Schädel, et al. 2021. Permafrost. In Recarbonizing global soils – A technical manual of recommended management practices, 127–47. Rome, Italy: Food and Agriculture Organization of the United Nations. doi:10.4060/cb6378en.
- Tahvanainen, V., J. Miina, and M. Kurttila. 2019. Climatic and economic factors affecting the annual supply of wild edible mushrooms and berries in Finland. Forests 10 (5): 385. doi:10.3390/f10050385.
- Thoman, R., J. E. Walsh, and H. R. McFarland. 2019. Alaska’s changing environment: Documenting Alaska’s physical and biological changes through observations. Fairbanks, AK, USA: International Arctic Research Center, University of Alaska Fairbanks.
- Ulrich, M., H. Matthes, L. Schirrmeister, J. Schütze, H. Park, Y. Iijima, and A. N. Fedorov. 2017. Differences in behavior and distribution of permafrost‐related lakes in Central Yakutia and their response to climatic drivers. Water Resources Research 53 (2): 1167–88. doi:10.1002/2016WR019267.
- Ulrich, M., H. Matthes, J. Schmidt, A. N. Fedorov, L. Schirrmeister, C. Siegert, B. Schneider, J. Strauss, and C. Zielhofer. 2019. Holocene thermokarst dynamics in Central Yakutia–A multi-core and robust grain-size endmember modeling approach. Quaternary Science Reviews 218: 10–33. doi:10.1016/j.quascirev.2019.06.010.
- Unc, A., D. Altdorff, E. Abakumov, S. Adl, S. Baldursson, M. Bechtold, D. J. Cattani, et al. 2021. Expansion of agriculture in northern cold-climate regions: A cross-sectoral perspective on opportunities and challenges. Frontiers in Sustainable Food Systems 5: 663448. doi:10.3389/fsufs.2021.663448.
- University of Alaska Fairbanks Agricultural and Forestry Experiment Station. 1998. University of Alaska Agricultural and Forestry Experiment Station. Agroborealis 30 (1): Catalogue #30–1.
- U.S. Department of Agriculture, National Agriculture Statistics Service. 2019. 2017 Census of Agriculture: Alaska State and Area Data. Vol 1, Part 2, AC-17-A-2. https://www.nass.usda.gov/Publications/AgCensus/2017/Full_Report/Volume_1,_Chapter_1_State_Level/Alaska/akv1.pdf
- U.S. Department of Agriculture, National Agriculture Statistics Service. 2021. USDA’s national agricultural statistics service, Alaska Field office database, https://www.nass.usda.gov/Statistics_by_State/Alaska/index.php
- U.S. National Science Foundation. 2021. Award Abstract # 2126965, NNA research: Permafrost grown: Cultivating convergence between farmers and researchers to foster sustainability for intensifying permafrost-agroecosystems. https://www.nsf.gov/awardsearch/showAward?AWD_ID=2126965
- Verdonen, M., L. T. Berner, B. C. Forbes, and T. Kumpula. 2020. Periglacial vegetation dynamics in Arctic Russia: Decadal analysis of tundra regeneration on landslides with time series satellite imagery. Environmental Research Letters 15 (10): 105020. doi:10.1088/1748-9326/abb500.
- Verdonen, M., A. Störmer, E. Lotsari, P. Korpelainen, B. Burkhard, A. Colpaert, and T. Kumpula. 2023. Permafrost degradation at two monitored palsa mires in north-west Finland. The Cryosphere 17 (5): 1803–19. doi:10.5194/tc-17-1803-2023.
- Vincent, W. F., M. Lemay, and M. Allard. 2017. Arctic permafrost landscapes in transition: Towards an integrated Earth system approach. Arctic Science 3 (2): 39–64. doi:10.1139/as-2016-0027.
- Wang, Q., T. Okadera, M. Watanabe, T. Wu, and B. Ochirbat. 2022. Ground warming and permafrost degradation in various terrestrial ecosystems in northcentral Mongolia. Permafrost and Periglacial Processes 33 (4): 406–24. doi:10.1002/ppp.2161.
- Ward Jones, M., G. Gannon, and B. Jones. 2024. Temperature monitoring of various crop with and without seasonal extension techniques during the 2022 growing season in Fairbanks, Alaska. Arctic Data Center. doi:10.18739/A26T0GZ0N.
- Ward Jones, M., T. Schwoerer, G. Gannon, B. M. Jones, M. Z. Kanevskiy, I. Sutton, B. St. Pierre, C. St. Pierre, J. Russell, and D. Russell. 2022. Climate-driven expansion of northern agriculture must consider permafrost. Nature Climate Change 12 (8): 699–703. doi:10.1038/s41558-022-01436-z.
- Windirsch, T., B. C. Forbes, G. Grosse, J. Wolter, S. Stark, C. Treat, M. Ulrich, et al. 2023. Impacts of reindeer on soil carbon storage in the seasonally frozen ground of northern Finland: A pilot study. Boreal Environment Research 28 (1–6): 207–26.
- Windirsch, T., G. Grosse, M. Ulrich, B. C. Forbes, M. Göckede, J. Wolter, M. Macias-Fauria, J. Olofsson, N. Zimov, and J. Strauss. 2022. Large herbivores on permafrost—A pilot study of grazing impacts on permafrost soil carbon storage in Northeastern Siberia. Frontiers in Environmental Science 10. doi:10.3389/fenvs.2022.893478.
- Zhang, L., C. R. Brown, D. Culley, B. Baker, E. Kunibe, H. Denney, C. Smith, N. Ward, T. Beavert, J. Coburn, et al. 2010. Inferred origin of several Native American potatoes from the Pacific Northwest and Southeast Alaska using SSR markers. Euphytica 174 (1): 15–29. doi:10.1007/s10681-009-0092-4.