Abstract
DUP-785 (Brequinar sodium) is a potent inhibitor of the mitochondrial dihydroorotate dehydrogenase (DHO-DH), a rate-limiting enzyme in the pyrimidine de novo nucleotide synthesis. In phase I clinical studies at the maximum tolerated dose (MTD) Brequinar induced a long-term inhibition of DHO-DH in white blood cells (WBC) and a long-term depletion of plasma uridine. These two parameters were related to severe myelosuppression, so that in Phase II studies the dose of Brequinar was decreased considerably. We further characterized the mechanism of DHO-DH enzyme inhibition while in blood samples of patients entered into Phase II studies we evaluated DHO-DH inhibition in WBC and plasma uridine depletion. With Electron Spin Resonance it was demonstrated that DHO-DH produced oxygen radical formation, which was inhibited by Brequinar. In the Phase II study depending on the dose (600 to 2000 mg/m2), uridine decreased to 20% (at the highest dose) or to 80–85% (at the middle dose) or did not change, which was associated with inhibition of DHO-DH (1% activity left vs 11 and 24% left). Inhibition of DHO-DH in the tumor of the latter patient was moderate as well (12% activity left). Brequinar was inactive in all tumor types evaluated possibly because of high uridine levels in the tumor. In conclusion, Brequinar was inactive against solid tumors, but DHO-DH inhibition was associated with myeloid toxicity, which may explain its potential for treatment of leukemia or inflammatory diseases.
Introduction
Brequinar sodium (DUP-785; NSC 369390, Brequinar) was developed in the 1980s and showed a promising preclinical antitumor activity against a number of human cancer xenografts.[Citation1] When entered into the clinic its mechanism of action was unknown, although there were some indications that it might have anti-pyrimidine activity.[Citation2] In order to elucidate the mechanism of action several approaches were followed such as the “pyrazofurin” test.[Citation3] Pyrazofurin is an inhibitor of orotate phosphoribosyl transferase (OPRT)[Citation4] and its cytotoxicity can be rescued by uridine. Exposure of cells to pyrazofurin would lead to accumulation of orotic acid, which appeared to be prevented by Brequinar. Exposure of cells to radiolabeled bicarbonate with pyrazofurin led to accumulation in orotic acid, but co-incubation with Brequinar prevented this accumulation, leading to the conclusion that Brequinar inhibits an enzyme earlier in the pyrimidine de novo nucleotide synthesis (). An HPLC assay for DHO-DH[Citation5] enabled to determine that Brequinar was a potent inhibitor of the mitochondrial DHO-DH (using rat liver mitochondria biphasic kinetics for L-DHO with a Km of 56 and 12 μM). The mode of inhibition was linear type with a Ki of about 0.1 μM3. Using a different approach Chen et al.[Citation2] came to the same conclusion.
Figure 1. Pyrimidine de novo nucleotide synthesis showing the inhibition of DHO-DH by Brequinar and of orotate phosphoribosyl transferase (OPRT) by pyrazofurin (PZ). DHO-DH is a mitochondrial enzyme located at the outside of the inner membrane, depicted by the two circles (fat outside, thin inside). PRPP (5-phosphoribosyl-1-pyrophosphate) is a co-substrate for the phosphoribosylation of orotic acid to OMP, catalyzed by OPRT.
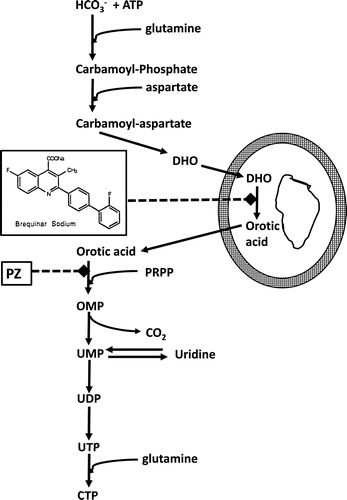
Although the uptake mechanism of Brequinar in cells has not really been characterized, injection of Brequinar to mice at its maximum tolerated dose (MTD) showed that DHO-DH activity in tumors and bone marrow cells was rapidly inhibited and remained inhibited for several days[Citation6] in relation to its pharmacokinetics. This inhibition was accompanied by a decrease in mouse plasma uridine levels (from 8.9 μM to about 3 μM) and of UTP in bone marrow; recovery of UTP pools paralleled that of DHO-DH. In tumors depletion of uridine nucleotides was only found in sensitive tumors. Based on this principle DHO-DH activity in white blood cells (WBC) and plasma uridine levels were measured in patients entered in a Phase I study to determine its MTD at a 3-weekly schedule.[Citation7,Citation8] Inhibition of DHO-DH in WBC (>90% inhibition) and especially its recovery, and the depletion of plasma uridine (initial levels between 3.0 and 6.8 μM) were dose-dependent followed by a rebound. The uridine depletion at the highest dose was associated with a prolonged DHO-DH inhibition and with a decrease of WBC UTP and CTP levels down to 42 and 16% of pretreatment levels. Most importantly these parameters appeared to be related to myelotoxicity. Brequinar was also evaluated at weekly (MTD 1500 mg/m2), bi-weekly (MTD 750 mg/m2)[Citation9] and daily (MTD 250 mg/m2)[Citation10] schedules. Subsequent testing of Brequinar in several phase II studies at different schedules demonstrated no or moderate (3/53 lung cancer patients, 1/51 colon cancer [two studies], 2/29 gastric cancer, 4/33 breast cancer) antitumor activity at weekly schedules (1200 mg/m2) in colon cancer,[Citation11] melanoma,[Citation12] head & neck cancer,[Citation13] lung cancer,[Citation14] pancreatic cancer[Citation15] and breast cancer.[Citation16] Because of the recent interest in novel analogs of Brequinar and other inhibitors of DHO-DH[Citation17,Citation18,Citation19] we reevaluated plasma samples of patients entered in some of the above-mentioned Phase II studies. Since DHO-DH is a mitochondrial enzyme and its effects are more pronounced under hypoxic conditions[Citation20] we further explored the mechanism of DHO-DH inhibition, and the relation between enzyme activity and drug sensitivity in several in vivo tumor models.
Materials and methods
Enzyme assays
For the animal experiments permissions were obtained from the local Animal Ethical Committee according to the Dutch Law on animal experimentation conforming the European Community Council Directive 2010/63/EU for laboratory animal care. DHO-DH activity was measured using an HPLC assay following the formation of orotic acid from DHO.[Citation5] DHO-DH activity was measured in tumor samples from various human xenografts from ovarian cancer (FCO, MRI and GL),[Citation21] head & neck cancer (LP and 14C)[Citation22] and murine colon cancer (Colon 26 and Colon 38).[Citation23] Mice were euthanized by cervical dislocation, tumors were excised immediately and put on ice. Thereafter, a relatively pure mitochondrial preparation was prepared as described previously[Citation5] which was used to determine DHO-DH activity. Potential inhibition by Brequinar was measured by addition of 1.5 μM Brequinar at a saturable concentration of 0.3 mM L-DHO.
DHO-DH is a mitochondrial enzyme and it was demonstrated that the DHO-DH reaction would generate superoxide.[Citation24] In order to study this mechanism mitochondria were isolated from rat liver as described earlier.[Citation5] Mitochondria were exposed to 0.3 mM L-DHO in the presence of 1 mM 5,5-dimethyl-1-pyrroline-N-peroxide (DMPO). DMPO will yield adducts with hyperfine splitting due to the β-hydrogen and the nitroxide nitrogen.[Citation25] The DMPO-trapped superoxide radicals were subsequently visualized with Electron Spin Resonance (ESR) using an ESP-300 Spectrometer in combination with an ESP 1600 Data Processing System (Bruker, Rheinstetten, FRG). ESR spectra were recorded at room temperature in an ER 4102 standard rectangular cavity (Bruker, Rheinstetten, FRG). The modulation frequency of the spectrometer was 9.78 kHz.[Citation26] Spectral intensities were calculated with the ESP 1600 Data Processing System, utilizing double integration of the first derivative signal. In order to inhibit this reaction we added 1.5 μM Brequinar.
Patients
Patients were entered in several Phase II studies of Brequinar at different schedules. These studies were performed at various European institutions (Finsen Institute, Copenhagen, Denmark; Daniel de Hoed Cancer Hospital, Rotterdam, Radboud Hospital, Nijmegen, and the VU Academic Hospital, Amsterdam, all the Netherlands); in each center the local Ethical Medical Committee approved the study. From 7 patients (4 males/3 females; median age 62, range 50–69; 2 non-small cell lung cancer, one renal cell cancer, one colon cancer, 2 head & neck cancer), we obtained WBC and plasma in which we measured DHO-DH activity and plasma uridine levels as described previously for the Phase I study.[Citation8] The amount of material from each patient was limited allowing to do one enzyme assay in duplicate (variation less than 15%). Measurement of plasma uridine was done in duplicate as well, with a variation of less than 5%). From one patient with head & neck cancer we were able to obtain one tumor sample before and one after treatment, allowing to perform one measurement of DHO-DH, each in duplicate. None of the patients experienced serious toxicity (all lower than Grade 2, so that no correlation could be established with toxicity, precluding meaningful statistics as was done in the Phase I study.[Citation8]
Since it is known that uridine may follow a circadian variation inversely related to a circadian variation in uridine phosphorylase,[Citation27] we wanted to exclude that a depletion of uridine would be a physiological variation. For that purpose plasma uridine levels were measured in 6 human volunteers over a period of 24 hr. All volunteers (4 males, 2 females; median age 30 years, range 27–31) received the same diet during this period.
Statistics
When feasible and relevant we evaluated the treatment related effects using the Student’s t test for paired and unpaired samples.
Results
DHO-DH activity in model systems and inhibition by Brequinar
DHO-DH activity was measured in several tumors previously characterized for their sensitivity to Brequinar. We found a 4-fold variation in DHO-DH activity, with the lowest activity in the head & neck xenograft LP and the highest in Colon 26. Inhibition by Brequinar was most pronounced in the head & neck cancer xenografts with the lowest remaining activity (); inhibition of DHO-DH in the Colon 38 was significantly less than in the ovarian cancer tumors (p < 0.05). Earlier we observed a relationship between DHO-DH inhibition and sensitivity to Brequinar in vitro,[Citation28] but in the in vivo models this relationship was less evident.
Figure 2. Activity of DHO-DH in several human xenografts from ovarian cancer and head & neck squamous cell carcinoma (SCC) and in the murine colon cancer models Colon 26 (CT26) and Colon 38 (CT38). Inhibition of DHO-DH was achieved by addition of 1.5 μM Brequinar (DUP), a concentration present for several days in plasma of patients treated with Brequinar.[Citation31] Values are means of 4–7 separate tumors ±. SE. Inhibition by Breq was significant for all tumors (paired t-test; p < 0.0005).
![Figure 2. Activity of DHO-DH in several human xenografts from ovarian cancer and head & neck squamous cell carcinoma (SCC) and in the murine colon cancer models Colon 26 (CT26) and Colon 38 (CT38). Inhibition of DHO-DH was achieved by addition of 1.5 μM Brequinar (DUP), a concentration present for several days in plasma of patients treated with Brequinar.[Citation31] Values are means of 4–7 separate tumors ±. SE. Inhibition by Breq was significant for all tumors (paired t-test; p < 0.0005).](/cms/asset/fe9cdddd-7dd5-470d-8d77-e1b92eb7ab55/lncn_a_1508692_f0002_b.jpg)
Incubation of rat liver mitochondria with DHO demonstrated a clear superoxide radical formation with typical DMPO-OOH and DMPO-OH spectra with an AN of 14.9 G (). Addition of Brequinar to the reaction completely inhibited this radical formation.
Figure 3. Superoxide formation after incubation of isolated rat liver mitochondria with 0.3 mM L-DHO for 30 min. Instrumental conditions: magnetic field, center field 3480 G; sweep width 10 G; modulation frequency, 9.78 kHz; modulation amplitude, 0.990 G; gain, 3.20 × 105; power, 50 mW; conversion time, 10.24 ms; number of scans, 20; scan time, 40 s. DMPO was used to trap superoxide which is shown in the typical spectrum in the upper figure. Inhibition of DHO-DH was achieved by addition of 1.5 μM Brequinar. One representative experiment out of 3 is shown.
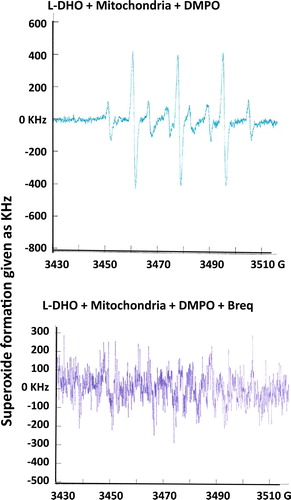
DHO-DH activity in patients
In 4 patients entered in a Phase II study with Brequinar we also measured the DHO-DH during treatment with Brequinar at different dose levels (). In all patients DHO-DH in WBC was almost completely inhibited (remaining activity 1% at the highest dose vs 11 and 24% at the lower doses) within 4 hr, which was retained until at least 24 hr. After 11 days, DHO-DH either showed an overshoot or returned to normal pretreatment levels. Interestingly in the tumor sample DHO-DH activity decreased to 12% of pretreatment levels.
Figure 4. Inhibition of DHO-DH in WBC (4A) and the effect of Brequinar on plasma uridine levels (4B) of patients with solid tumors entered in several Phase II studies at weekly doses of Brequinar at 1500 mg/m2 (#1 and #5), 2000 (#2), 1200 (#3) and 1200 (#4), and twice weekly doses of 600 (# 6) and 750 (#7). (A) Enzyme activities before treatment were set at 100%. From patient #1 we were also able to evaluate two tumor biopsy specimen, one before and one after drug administration. (B) The concentration of plasma uridine before treatment (samples taken in the morning between 9 and 10 am) was 4.9 ± 1.2 μM (range 4.03–6.8 μM); values were calculated by setting the pretreatment level at 100%. The doses of 600 and 750 mg/m2, given twice weekly, showed a slight decrease of uridine after 72 hr for #7 (from 128% to 76%) and even less for #6, possibly within the natural circadian variation.
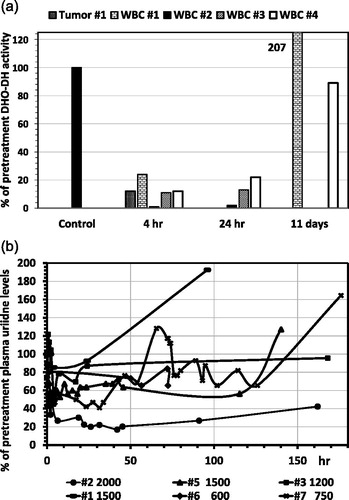
Uridine concentration
In order to exclude that uridine depletion was due to a physiological circadian variation of plasma uridine, blood samples from 6 human volunteers were evaluated over a 24 hr period. Care was taken that all volunteers received the same diet over this period in order to exclude food induced variations. Interestingly, in all volunteers plasma uridine levels reached a trough midafternoon and a peak in the evening around midnight (). Overall variation between peak-trough levels was 1.5–2.6-fold and significantly different (P < 0.005).
Figure 5. Circadian variation of plasma uridine levels in 6 healthy human volunteers All volunteers received the same diet (evening local Chinese dishes) during this period. The difference between peak and trough concentrations was significant (paired t-test; p < 0.001).
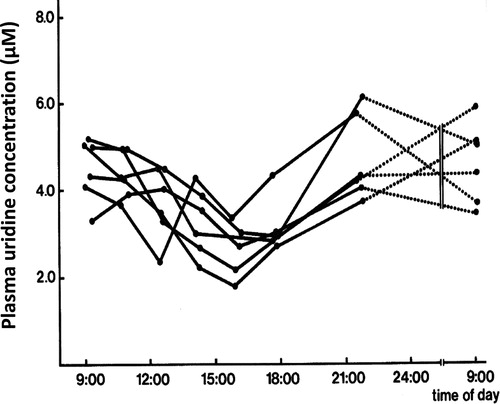
Plasma uridine levels in patients following Brequinar treatment was quite variable, but exceeded that of the natural circadian variation in healthy human volunteers, which was not observed in cancer patients.[Citation29] In one patient a depletion of uridine down to 20% (at the highest dose) of pretreatment levels was found, but in the other patients a moderate depletion (down to 40–50%) or to 80–85% (at the lowest dose), or even an increase was found ().
Discussion
In this study we demonstrated that the DHO-DH reaction is mediated by oxygen radical formation which is inhibited by Brequinar in agreement with the earlier reported superoxide formation of the DHO-DH reaction and its inhibition by Brequinar.[Citation24] Uptake of Brequinar into cells, tumors and subsequently in mitochondria is rapid and its elimination from tissues follows blood elimination.[Citation30] Brequinar has a triphasic decay of plasma concentrations with half-life ranges of 11.1–36.6 min, 1.7–6.9 h and 12.5–25.0 h.[Citation31] The long half-life is likely to reflect tissue accumulation with a slow release to the blood, explaining the relatively long DHO-DH inhibition. The ESR data also seem to indicate that Brequinar forms a complex with the enzyme explaining the relatively long-term inhibition. Although in vitro a correlation was found between the enzyme activity, the potential DHO-DH inhibition and the sensitivity to Brequinar,[Citation23,Citation28] we could not establish a similar relationship for the in vivo tumors. This is possibly due to the different in vivo behavior of Brequinar compared to in vitro. In vitro cells are all exposed to a similar concentration of Brequinar, while in vivo this exposure is likely to be different between the various tumors tested. Another explanation for the differences might be the tissue/tumor concentration of uridine. Darnowski et al.[Citation32] demonstrated that tissues have a higher uridine content than plasma, which was also found for our colon tumor models, in which the sensitive Colon 38 had a higher uridine level than the insensitive Colon 26, but a similar dose of Brequinar (50 mg/kg) hardly affected the uridine levels in Colon 26, but more pronounced (90%) in Colon 38.[Citation6]
There appear to be several differences between the patients evaluated in the Phase I and Phase II studies. In the Phase I study a pronounced and long-term inhibition of DHO-DH and a long term depletion of plasma uridine were only found in patients receiving a high dose of Brequinar (2000–2250 mg/m2); even at 1800 mg/m2 the effects were moderate.[Citation8] However, doses in the Phase II study were much lower (600 in the bi-weekly and 1200 and 1500 in the weekly schedules); only the patient receiving 2000 mg/m2 showed an 80% depletion and the highest DHO-DH inhibition. However, in all other Phase II studies lower doses were used. In another Phase II study uridine depletion at doses from 300 to 800 mg/m2 was moderate (25% at 300 and 70% at 400 mg/m2) and short lasting (recovered at 15 hr).[Citation33] Since plasma uridine mainly reflects what is happening in normal tissues and considering the high uridine levels in solid tumors (compared to plasma), the lack of antitumor activity of Brequinar can possibly be explained by insufficient inhibition of DHO-DH in the tumor. This might hold for novel DHO-DH inhibitors as well. Despite the renewed interest in DHO-DH inhibitors it seems unlikely that they will be able to effectively inhibit solid tumor DHO-DH without serious systemic side effects.
The major side effect of Brequinar was myelosuppression,[Citation7–9] indicating a specific effect on certain hematopoietic cells. Indeed we found pronounced inhibition of DHO-DH in mouse bone marrow cells.[Citation6] Apparently inhibition of DHO-DH leads to a specific effect on myeloid cells, which was also found in some recent studies focusing on models for acute myeloid leukemia.[Citation17,Citation18] This is in line with the efficacy of the DHO-DH inhibitors leflunomide and teriflunomide against the auto-immune disease acute rheumatoid arthritis.[Citation34,Citation35] It has been shown that inhibition of DHO-DH not only leads to suppression of interleukin 1 and tumor necrosis factor alpha, it also inhibits T-cell activation.[Citation36] DHO-DH boosts the rate of cytokine release such as Il-17 and interferon-ϒ, which is inhibited by DHO-DH inhibitors such as vidofludimus (4SC-101, now developed as IMU-838).[Citation37] Therefore a future role for DHO-DH inhibitors might be in the field of inflammatory diseases, not only rheumatoid arthritis, but also inflammatory bowel disease.[Citation37] It was also demonstrated that Brequinar can inhibit the phosphorylation of tyrosine on proteins such as p59[Citation36]. Current efforts on development of new DHO-DH inhibitors should focus on these applications. Indeed based on the 4SC technology, Immunic AG is developing IMU-838 for these applications. Other possibilities include targeting acute myeloid leukemia (AML), since DHO-DH inhibitors may induce differentiation.[Citation18] Targeting of malarial[Citation38] and viral[Citation39] infections might also be an interesting option, since these organisms have an altered pyrimidine metabolism making them more susceptible to inhibitors of pyrimidine de novo synthesis than mammalian cells.[Citation40] An important point in the application of Brequinar or other DHO-DH inhibitors is the protection of tumor cells by uridine, which has a high concentration in tumors (10–100 μM), although the normal plasma concentration (5 μM) is already high enough to reverse the effect of Brequinar. This can be overcome by the use of a nucleoside transport inhibitor, such as dipyridamole.[Citation41]
In conclusion, Brequinar possibly failed in Phase II clinical development showing no effect against solid tumors, due to insufficient DHO-DH inhibition as could be concluded from the moderate to no effect on uridine levels. Insufficient inhibition of DHO-DH might be related to a dose that might be too low, or a schedule that might not be frequent enough. The best anti-tumor effect in mice and the best in vitro growth inhibition, was achieved at daily (at least 5 days) administration and at prolonged (at least 3–4 days) drug exposure, respectively. The specific properties of DHO-DH (oxygen radical) might be a new lead for other application such as hematological malignancies or inflammatory diseases.
Acknowledgements
Patients described in this paper were entered in various Phase II studies performed at the VU University Medical Center in Amsterdam, the Radboud Medical Center in Nijmegen, the Erasmus Medical Center in Rotterdam, all in the Netherlands (current names), and The Finsen Institute in Copenhagen, Denmark. Some of the principal investigators are retired or deceased. Additional contributions were from Jorge Nadal, Emile Laurensse, and Dr. J.M.S. van Maanen (ESR, deceased).
References
- Dexter, D. L.; Hesson, D. P.; Ardecky, R. J.; Rao, G. V.; Tippett, D. L.; Dusak, B. A.; Paull, K. D.; Plowman, J.; DeLarco, B. M.; Narayanan, V. L.; Forbes, M. Activity of a Novel 4-Quinolinecarboxylic Acid, NSC 368390 [6-Fluoro-2-(2'-Fluoro-1,1'-Biphenyl-4-yl)-3-Methyl-4-Quinolinecarb Oxylic Acid Sodium Salt], against Experimental Tumors. Cancer Res. 1985, 45, 5563–5568.
- Chen, S. F.; Ruben, R. L.; Dexter, D. L. Mechanism of Action of the Novel Anticancer Agent 6-Fluoro-2-(2'-Fluoro-1,1'-Biphenyl-4-yl)-3-Methyl-4-Quinolinecarbo Xylic Acid Sodium Salt (NSC 368390): Inhibition of de Novo Pyrimidine Nucleotide Biosynthesis. Cancer Res. 1986, 46, 5014–5019.
- Peters, G. J.; Sharma, S. L.; Laurensse, E.; Pinedo, H. M. Inhibition of Pyrimidine de Novo Synthesis by DUP-785 (NSC 368390). Invest New Drugs 1987, 5, 235–244.
- Cadman, E. C.; Dix, D. E.; Handschumacher, R. E. Clinical, Biological, and Biochemical Effect of Pyrazofurin. Cancer Res. 1978, 38, 682–688.
- Peters, G. J.; Laurensse, E.; Leyva, A.; Pinedo, H. M. A Sensitive, Non-Radiometric Assay for Dihydroorotic Acid Dehydrogenase Using Anion-Exchange High-Performance Liquid Chromatography. Anal. Biochem. 1987, 161, 32–38.
- Peters, G. J.; Nadal, J. C.; Laurensse, E. J.; De Kant, E.; Pinedo, H. M. Retention of in Vivo Antipyrimidine Effects of Brequinar Sodium (DUP-785; NSC368390) in Murine Liver, Bone Marrow and Colon Cancer. Biochem. Pharmacol. 1990, 39, 135–144.
- Schwartsmann, G.; Dodion, P.; Vermorken, J. B.; ten Bokkel Huinink, W. W.; Joggi, J.; Winograd, B.; Gall, H.; Simonetti, G.; van der Vijgh, W. J. F.; van Hennik, M. B.; et al. Phase I Study of Brequinar Sodium (NSC 368390) in Patients with Solid Malignancies. Cancer Chemother. Pharmacol. 1990, 25, 345–351.
- Peters, G. J.; Schwartsmann, G.; Nadal, J. C.; Laurensse, E. J.; Van Groeningen, C. J.; Van der Vijgh, W. J. F.; Pinedo, H. M. In Vivo Inhibition of the Pyrimidine de Novo Enzyme Dihydroorotic Acid Dehydrogenase by Brequinar Sodium (DUP-785; NSC 368390) in Mice and Patients. Cancer Res. 1990, 50, 4644–4649.
- Bork, E.; Vest, S.; Hansen, H. H. A Phase I Clinical and Pharmacokinetic Study of Brequinar Sodium, DUP 785 (NSC 368390), Using a Weekly and a Biweekly Schedule. Eur. J. Cancer Clin. Oncol. 1989, 25, 1403–1411.
- Arteaga, C. L.; Brown, T. D.; Kuhn, J. G.; Shen, H. S.; O'Rourke, T. J.; Beougher, K.; Brentzel, H. J.; Von Hoff, D. D.; Weiss, G. R. Phase I Clinical and Pharmacokinetic Trial of Brequinar Sodium (DuP 785; NSC 368390). Cancer Res. 1989, 49, 4648–4653.
- Dodion, P. F.; Wagener, T.; Stoter, G.; Drozd, A.; Lev, L. M.; Skovsgaard, T.; Renard, J.; Cavalli, F. Phase II Trial with Brequinar (DUP-785, NSC 368390) in Patients with Metastatic Colorectal Cancer: A Study of the Early Clinical Trials Group of the EORTC. Ann. Oncol. 1990, 1, 79–80.
- Natale, R.; Wheeler, R.; Moore, M.; Dallaire, B.; Lynch, W.; Carlson, R.; Grillo-Lopez, A.; Gyves, J. Multicenter Phase II Trial of Brequinar Sodium in Patients with Advanced Melanoma. Ann. Oncol. 1992, 3, 659–660.
- Urba, S.; Doroshow, J.; Cripps, C.; Robert, F.; Velez-Garcia, E.; Dallaire, B.; Adams, D.; Carlson, R.; Grillo-Lopez, A.; Gyves, J. Multicenter Phase II Trial of Brequinar Sodium in Patients with Advanced Squamous-Cell Carcinoma of the Head and Neck. Cancer Chemother. Pharmacol. 1992, 31, 167–169.
- Maroun, J.; Ruckdeschel, J.; Natale, R.; Morgan, R.; Dallaire, B.; Sisk, R.; Gyves, J. Multicenter Phase II Study of Brequinar Sodium in Patients with Advanced Lung Cancer. Cancer Chemother. Pharmacol. 1993, 32, 64–66.
- Moore, M.; Maroun, J.; Robert, F.; Natale, R.; Neidhart, J.; Dallaire, B.; Sisk, R.; Gyves, J. Multicenter Phase II Study of Brequinar Sodium in Patients with Advanced Gastrointestinal Cancer. Invest. New Drugs. 1993, 11, 61–65.
- Cody, R.; Stewart, D.; DeForni, M.; Moore, M.; Dallaire, B.; Azarnia, N.; Gyves, J. Multicenter Phase II Study of Brequinar Sodium in Patients with Advanced Breast Cancer. Am. J. Clin. Oncol. 1993, 16, 526–528.
- Lewis, T. A.; Sykes, D. B.; Law, J. M.; Muñoz, B.; Rustiguel, J. K.; Nonato, M. C.; Scadden, D. T.; Schreiber, S. L. Development of ML390: A Human DHODH Inhibitor That Induces Differentiation in Acute Myeloid Leukemia. ACS Med. Chem. Lett. 2016, 7, 1112–1117.
- Sykes, D. B.; Kfoury, Y. S.; Mercier, F. E.; Wawer, M. J.; Law, J. M.; Haynes, M. K.; Lewis, T. A.; Schajnovitz, A.; Jain, E.; Lee, D.; et al. Inhibition of Dihydroorotate Dehydrogenase Overcomes Differentiation Blockade in Acute Myeloid Leukemia. Cell 2016, 167, 171–186.
- Vyas, V. K.; Ghate, M. Recent Developments in the Medicinal Chemistry and Therapeutic Potential of Dihydroorotate Dehydrogenase (DHODH) Inhibitors. Mrmc. Chem. 2011, 11, 1039–1055.
- Löffler, M. The “Anti-Pyrimidine Effect” of Hypoxia and Brequinar Sodium (NSC 368390) Is of Consequence for Tumor Cell Growth. Biochem. Pharmacol. 1992, 43, 2281–2287.
- Boven, E.; Winograd, B.; Fodstad, O.; Lobbezoo, M. W.; Pinedo, H. M. Preclinical Phase II Studies in Human Tumor Lines: A European Multicenter Study. Eur. J. Cancer Clin. Oncol. 1988, 24, 567–573.
- Braakhuis, B. J. M.; van Dongen, G. A.; Peters, G. J.; van Walsum, M.; Snow, G. B. Antitumor Activity of Brequinar Sodium (Dup-785) against Human Head and Neck Squamous Cell Carcinoma Xenografts. Cancer Lett. 1990, 49, 133–137.
- Peters, G. J.; Laurensse, E.; de Kant, E.; Nadal, J. C.; Pinedo, H. M. The Relationship between Dihydroorotic Acid Dehydrogenase and in Vitro and in Vivo Cytostatic Effects of Brequinar Sodium (DUP-785; NSC 368390). Adv. Exp. Med. Biol. 1989, 253B, 375–382.
- Hey-Mogensen, M.; Goncalves, R. L.; Orr, A. L.; Brand, M. D. Production of Superoxide/H2O2 by Dihydroorotate Dehydrogenase in Rat Skeletal Muscle Mitochondria. Free Radic. Biol. Med. 2014, 72, 149–155.
- Finkelstein, E.; Rosen, G. M.; Rauckman, E. J. Spin Trapping of Superoxide and Hydroxyl Radical: Practical Aspects. Arch. Biochem. Biophys. 1980, 200, 1–16.
- Mans, D. R.; Retèl, J.; van Maanen, J. M.; Lafleur, M. V.; van Schaik, M. A.; Pinedo, H. M.; Lankelma, J. Role of the Semi-Quinone Free Radical of the anti-Tumour Agent Etoposide (VP-16-213) in the Inactivation of Single- and Double-Stranded Phi X174 DNA. Br. J. Cancer. 1990, 62, 54–60.
- El Kouni, M. H.; Naguib, F. N. M.; Kyung Sun, P.; Sungman, C.; Darnowskl, J. W.; Soong, S.-J. Circadian Rhythm of Hepatic Uridine Phosphorylase Activity and Plasma Concentration of Uridine in Mice. Biochem. Pharmacol. 1990, 40, 2479–2485.
- de Kant, E.; Pinedo, H. M.; Laurensse, E.; Peters, G. J. The Relation between Inhibition of Cell Growth and of Dihydroorotic Acid Dehydrogenase by Brequinar Sodium. Cancer Lett. 1989, 46, 123–127.
- Van Kuilenburg, A. B. P.; Poorter, R. L.; Peters, G. J.; Van Gennip, A. H.; Van Lenthe, H.; Stroomer, A. E. M.; Smid, K.; Noordhuis, P.; Bakker, P. J. M.; Veenhof, C. H. N. No, Circadian Variation of Dihydropyrimidine Dehydrogenase, Uridine Phosphorylase, ß-Alanine and 5-Fluorouracil during Continuous Infusion of 5-Fluoruoracil. Adv. Exp. Med. Biol. 1998; 431, 815-816. Eur. J. Cancer 1995, 31, S198.
- Shen, H. S.; Chen, S. F.; Behrens, D. L.; Whitney, C. C.; Dexter, D. L.; Forbes, M. Distribution of the Novel Anticancer Drug Candidate Brequinar Sodium (DuP 785, NSC 368390) into Normal and Tumor Tissues of Nude Mice Bearing Human Colon Carcinoma Xenografts. Cancer Chemother. Pharmacol. 1988, 22, 183–186.
- Schwartsmann, G.; van der Vijgh, W. J. F.; van Hennik, M. B.; Klein, I.; Vermorken, J. B.; Dodion, P.; ten Bokkel Huinink, W. W.; Joggi, G.; Gall, H.; Crespeigne, N.; et al. Pharmacokinetics of Brequinar Sodium (NSC 368390) in Patients with Solid Tumors during a Phase I Study. Eur. J. Cancer Clin. Oncol. 1989, 25, 1675–1681.
- Darnowski, J. W.; Handschumacher, R. E. Tissue Uridine Pools: Evidence in Vivo of a Concentrative Mechanism for Uridine Uptake. Cancer Res. 1986, 46, 3490–3494.
- Buzaid, A. C.; Pizzorno, G.; Marsh, J. C.; Ravikumar, T. S.; Murren, J. R.; Todd, M.; Strair, R. K.; Poo, W. J.; Hait, W. N. Biochemical Modulation of 5-Fluorouracil with Brequinar: Results of a Phase I Study. Cancer Chemother. Pharmacol. 1995, 36, 373–378.
- Schultz, M.; Keeling, S. O.; Katz, S. J.; Maksymowych, W. P.; Eurich, D. T.; Hall, J. J. Clinical Effectiveness and Safety of Leflunomide in Inflammatory Arthritis: A Report from the RAPPORT Database with Supporting Patient Survey. Clin. Rheumatol. 2017, 36, 1471–1478.
- Breedveld, F. C.; Dayer, J. M. Leflunomide: Mode of Action in the Treatment of Rheumatoid Arthritis. Ann. Rheum. Dis. 2000, 59, 841–849.
- Xu, X.; Williams, J. W.; Shen, J.; Gong, H.; Yin, D. P.; Blinder, L.; Elder, R. T.; Sankary, Finnega, H.; Chong, A. S. In Vitro and In Vivo Mechanisms of Action of the Antiproliferative and Immunosuppressive Agent, Brequinar Sodium. J. Immunol. 1998, 160, 846–853.
- Fitzpatrick, L. R.; Deml, L.; Hofmann, C.; Small, J. S.; Groeppel, M.; Hamm, S.; Lemstra, S.; Leban, J.; Ammendola, A. 4SC-101, A Novel Immunosuppressive Drug, Inhibits IL-17 and Attenuates Colitis in Two Murine Models of Inflammatory Bowel Disease. Inflamm. Bowel Dis. 2010, 16, 1763–1777.
- Boa, A. N.; Canavan, S. P.; Hirst, P. R.; Ramsey, C.; Stead, A. M.; McConkey, G. A. Synthesis of Brequinar Analogue Inhibitors of Malaria Parasite Dihydroorotate Dehydrogenase. Bioorg Med Chem 2005, 13, 1945–1967.
- Adcock, R. S.; Chu, Y. K.; Golden, J. E.; Chung, D. H. Evaluation of anti-Zika Virus Activities of Broad-Spectrum Antivirals and NIH Clinical Collection Compounds Using a Cell-Based, High-Throughput Screen Assay. Antiviral Res. 2017, 138, 47–56.
- Seymour, K. K.; Lyons, S. D.; Phillips, L.; Rieckmann, K. H.; Christopherson, R. I. Cytotoxic Effects of Inhibitors of de Novo Pyrimidine Biosynthesis upon Plasmodium falciparum. Biochemistry 1994, 3;33, 5268–5274.
- Peters, G. J.; Kraal, I.; Pinedo, H. M. In Vitro and in Vivo Studies on the Combination of Brequinar Sodium (DUP-785; NSC 368390) with 5-Fluorouracil; Effects of Uridine. Br. J. Cancer. 1992, 65, 229–233.