Abstract
Airborne asbestos fibers are associated with many serious detrimental effects on human health, while the hazard posed by waterborne fibers remains an object of debate. In adopting a precautionary principle, asbestos content in water needs to be kept as low as possible and polluting waters with asbestos should be avoided. CitationTurci et al. (2008) recently reported a method for the decontamination of asbestos-polluted waters or landfill leachates from chrysotile that combines power ultrasound (US) with oxalic acid (Ox), an acidic chelating molecule. In the previous study, the occurrence of antigorite, a polymorph of serpentine, the mineral group encompassing chrysotile asbestos, acted as a confounding factor for complete removal of chrysotile from water. The effects of US + Ox on pure chrysotile asbestos from Val Malenco, Italian Central Alps, were examined in this investigation. In the absence of mineral contaminants, a more rapid removal of pure chrysotile from water was undertaken with respect to the previous specimen. After only 12 h of combined US + Ox acid treatment, imaging (SEM) of mineral debris indicated complete loss of fibrous habit. In addition, crystallography and vibrational features of chrysotile were not detectable (x-ray powder diffraction [XRPD] and micro-Raman spectroscopy) and elemental analysis showed a low Mg/Si ratio, i.e., the loss of the brucitic layer in chrysotile (x-ray fluorescence, XRF). Some nanometric rod-shaped debris, observed in the previous study and tentatively recognized as serpentine antigorite, was now found to be made of amorphous silica, which is relatively safe and noncarcinogenic to humans, providing further assurance regarding the safety of treated product. Thus, data indicated the proposed method was effective in detoxifying waterborne chrysotile asbestos fibers.
Airborne asbestos fibers consisting of six different fibrous silicates, namely, chrysotile, amosite, crocidolite, tremolite, anthophyllite, and actinolite, are associated with serious detrimental effects on human health. Humans exposed to asbestos develop asbestosis, a debilitating and often fatal lung disease, and malignancies such as lung cancer and pleural mesothelioma, which may develop several decades after exposure (CitationMossman et al., 1990; CitationVallyathan et al., 1998). The carcinogenicity of asbestos minerals was originally considered mainly an occupational issue (CitationInternational Agency for Research on Cancer, 1977; CitationVallyathan et al., 1998), but a growing concern was expressed regarding the dispersion of natural occurring asbestos (NOA) in the environment (CitationU.S. EPA, 2003, Citation2005; CitationKazan-Allen, 2005; CitationPan et al., 2005; CitationDearwent et al., 2006; CitationVan Gosen, 2007), following reports of multiple exposure scenarios, as noted in Libby, MT (USA) (CitationPeipins et al., 2003; CitationMcDonald et al., 2004; CitationLee et al., 2008). Naturally occurring mineral fibers may be found both in large commercial deposits, close to active or abandoned mines, and in small noneconomic occurrences throughout the mountains (CitationLee et al., 2008).
Asbestos fibers are widely distributed also in the aqueous environment and may eventually contaminate drinking water through different scenarios, by (i) weathering effects on natural deposits such as serpentine rocks, (ii) release from asbestos-cement pipes, and (iii) processes associated with mining and production of certain types of iron ore (CitationCantor, 1997). Waterborne asbestos concentration is variable and ranges from 104 fibers/L to over 1011 fibers/L (CitationMillette et al., 1983). According to the World Health Organization (WHO) there is no consistent evidence that ingested asbestos is hazardous to human health (CitationWHO, 2006). However, some studies suggested possible correlation between gastrointestinal cancer and asbestos ingestion (CitationWebber, 1993; CitationKjaerheim et al., 2005). Furthermore, the occurrence of asbestos in irrigation water may generate aerosolized fibers to a concentration that is quite unpredictable, despite some reliable attempts to standardize airborne fibers per asbestos mass in suspension (U.S. EPA, 1980). The Italian Regional Agency for Environmental Protection (ARPA–Piemonte) accepted a conversion factor proposed by CitationWebber and coworkers (1988) of approximately 2 airborne fibers per liter of indoor air produced by 100,000 fibers/L in water. Following a precautionary principle, the U.S. Environmental Protection Agency (EPA) indicated a protective standard of 7 × 106 fiber/L with length >10 μm. If one considers that 80–95% of waterborne asbestos fibers are shorter than 1 μm and that U.S. EPA TEM analysis method 100.1 considers fibers longer than 0.5 μm, the U.S. EPA standard clearly underestimates the occurrence of waterborne asbestos. Since asbestos fibers, both airborne and waterborne, remain in the environment for decades or longer (CitationU.S. EPA, 2006), an environmentally responsible approach needs to be enforced in order not to disperse asbestos in surface water and groundwater. The methods currently adopted to abate waterborne asbestos are conventional water treatments such as coagulation/filtration. The filtering process of asbestos fibers requires, however, low-porosity filters, which are prone to be clogged with organic matter and other suspended inorganic particles. Furthermore, if asbestos filtration is applied with a certain success to small and controlled systems such as a drinking-water supply or landfill leachate (EU project FALL, LIFE03 ENV/IT/00323), it is not adaptable to larger scenarios such as natural release of fibers from (1) asbestos spoil dumps, (2) excavated faces in formerly quarried or mined sites, or (3) dumping of mining tailings into river or lakes (San Vittore, Balangero, Italy [CitationBuzio et al., 2000], and MABE, Kozani, Greece [CitationAnastasiadou and Gidarakos, 2007]).
To cope with such issues, an innovative method based upon synergistic action of power ultrasound (US) and an acidic metal chelator, oxalic acid (Ox), was recently proposed (CitationTurci et al., 2007b) and tested on a commercial sample of chrysotile from the Balangero mine (CitationTurci et al., 2008). In the present study, the current knowledge on the effectiveness of US and Ox in combination in detoxifying chrysotile fibers was expanded to another rather pure type of natural chrysotile. This sample, from Val Malenco, Italian Central Alps, has been thoroughly characterized by means of several physicochemical techniques, and the resulting data were used as a reference to evaluate the efficacy of the combined US and Ox remediation of waterborne chrysotile. In order to evaluate the activity of the two detoxification agents the fibers were subjected not only to the US–Ox treatment but also to US alone (in pure water suspension) or simply leached in an Ox solution. The morphology (scanning electron microscopy, SEM) and crystallographic pattern (x-ray powder diffraction, XRPD) of residuals were assessed in order to determine the persistence of the fibrous habit and occurrence of newly formed mineral phases. Spectroscopic techniques (micro-Raman and x-ray fluorescence [XRF] spectroscopy) were also employed to confirm crystallographic findings, distinguish between similar phases including chrysotile from antigorite (CitationGroppo et al., 2006) and to quantify US–Ox-driven chrysotile disruption/dissolution, by evaluating relative amount of structural elements remaining in the solid residue.
MATERIALS AND METHODS
Asbestos
Two different types of chrysotile were selected:
1. | A pure chrysotile specimen, with limited iron contamination, from Val Malenco (Italian Central Internal Alps). | ||||
2. | A commercial sample (classified 6D) from the San Vittore out-of-use asbestos mine (Balangero, Italy), kindly provided by R.S.A., the society managing the mine, already employed in previous investigations (CitationTurci et al., 2008; CitationFavero-Longo et al., 2009) and used here for comparison. |
Reagents
All reagents were purchased by Sigma-Aldrich. For all experiments ultrapure Milli-Q (Millipore, USA) water was used.
Sonochemical Treatment of Chrysotile
Chrysotile asbestos fibers (500 mg) were suspended in 50 ml ultrapure water (Milli-Q) or in 50 ml of 0.5 M Ox solution (fibers/solution ratio = 10 mg/ml) and placed into the US reactor. The cavitating tube frequency was set to 19.2 kHz and stabilized with an automated adjustment device (frequency hook). The fibers were sonicated for 12 or 21 h at an input power of approximately 150 W. The reactor was cooled in order to keep suspension temperature below 50°C and sealed in order to minimize evaporation of the liquid. In order to discriminate between the effect of acidic/chelating leaching and sonication, chrysotile (10 mg/ml) was also sonicated in pure water at the same experimental conditions or leached with Ox at 50°C under mild mixing in a thermostatic vessel.
Following the sonochemical treatments, the fibers were separated from the liquid by filtration through cellulose acetate membranes (pore size 0.45 μm), rinsed, and dried. The solid residues were subjected to structural analysis of the bulk (SEM imaging, XRPD, and micro-Raman spectroscopy), and elemental analysis via x-ray fluorescence (XRF) was performed.
Scanning Electron Microscopy (SEM)
Scanning electron microscopy (SEM) observations were performed on gold-coated fibers with a Stereoscan 410 Leica equipped with Oxford Link EDAX. The accelerating voltage was 15 kV and a secondary electron detector was used.
X-Ray Powder Diffraction (XRPD)
X-ray powder diffraction (XRPD) analyses were performed on the solid residues with a Phillips PW 1830, with θ–2θ geometry and Cu Kα radiation. The patterns obtained were compared with those contained in the Joint Committee of Powder Diffraction Standard (JCPDS) archives.
Confocal Micro-Raman Spectroscopy
Micro-Raman spectra were acquired using an integrated confocal Raman system that includes a Horiba Jobin-Yvon HR800 microspectrometer, an Olympus BX41 microscope, and a CCD air-cooled detector operating at –70°C. A polarized solid-state Nd laser operating at 532.11 nm and 80 mW was used as the excitation source. Correct calibration of the instruments was verified by measuring the Stokes and anti-Stokes bands and checking the position of the Si band at 520.7cm−1. To optimize the signal to noise ratio, spectra were acquired using an integration time of about 100 s for each spectral region.
X-Ray Florescence Spectroscopy (Micro-XRF)
All samples were analyzed using an EDAX Eagle III energy-dispersive micro-XRF (μXRF) spectrometer equipped with a Rh x-ray tube and a polycapillary exiting a circular area of nominally 30 μm diameter. Data collection occurred at each point for 45 s detector live time, with x-ray tube settings adjusted for 30% dead time. Under this experimental condition, about 1 × 106 counts were collected per scan. A matrix of at least 25 spots was analyzed for each sample.
Statistical Analysis
All data in the text and figures are provided as means ± SEM. The results were analyzed by a one-way analysis of variance (ANOVA) and Tukey's test. The criterion for significance was set at p < 0.05.
RESULTS AND DISCUSSION
Morphological Analysis
Images and micro-morphological features of natural chrysotile from Val Malenco and treated fibers are presented in and , respectively. Fibrous habit is widely recognized as a key factor in asbestos toxicity. Airborne fibers are usually not considered toxic for humans if their length is less than 5 μm. The U.S. EPA standard for waterborne asbestos fibers is calculated considering only fibers longer than 10 μm. In agreement with previous findings (CitationFavero-Longo et al., 2005; CitationTurci et al., 2007a), the long curled natural fibers of chrysotile () leached with Ox for 21 h () preserved the fibrous habit and fibers were morphologically unaltered, though some small debris were observed. Following power US for 21 h in pure Milli-Q water (), the long fibers of chrysotile were strongly reduced in length, but fibrous habit was largely preserved and fiber lengths remained within the micrometric range. Consistent with previous reports (CitationSpurny et al., 1979, Citation1980), the effect of US on asbestos fibers in pure water was mechanical in nature. Ultrasound in heterogeneous systems may act on suspended particles, i.e., asbestos fibers, through two different cavitation mechanisms, microjet impact and shock-wave damage, depending on US resonance frequency and particle size (CitationSuslick, 1990). A sketch of these two mechanisms is illustrated in . If particle size is several fold larger than resonant bubble size, the asymmetry of the near-surface environment induces a deformation of the resonant cavity during its collapse. This self-reinforcing deformation generates a high-speed stream of liquid that impacts on the surface with a velocity greater than 100 m/s. The impingement of microjets on surfaces is a well-known cause of corrosion and erosion phenomena of metals and machinery and ultrasonic cleaning of surfaces. If particle size is small enough not to disturb the liquid, the behavior of the system may be closer to that observed for homogeneous systems, and shock waves are produced following acoustic cavitation. Such waves create high-speed interparticle collisions that may induce melting of metal particles or further fragmentation of ceramic particles (CitationDoktycz & Suslick, 1990). When Ox 0.5 M is added to the chrysotile suspension, the fibrous habit of asbestos is rapidly disrupted during US for 12 and 21 h (, D and E, respectively) and the particles tend to merge in large aggregates. This finding is consistent with previously obtained data during similar treatment on the chrysotile sample from Balangero. The loss of fibrous habit on the Val Malenco chrysotile after 12 h of US–Ox treatment is even more pronounced than that reported for the Balangero one, with the former being possibly more rapidly disrupted by the US–Ox treatment. One representative high-magnification image of some particles following the US–Ox treatment for 21 h is presented in . Some apparently flat surfaces are covered with highly packed rod-shaped nanometric particles. Such particles were already observed following identical treatment of chrysotile from Balangero and were postulated to be nanofibers of antigorite, a serpentine polymorph (CitationTurci et al., 2008).
FIGURE 1. Images of natural and sonochemically modified chrysotile. Natural fibers (A) were leached with 0.5 M oxalic acid (Ox) solution for 21 h at 50°C (B), sonicated for 21 h with power ultrasound (US) at approximately150 W, 19.2 kHz in deionized water (C), and were simultaneously treated with US and Ox for 12 h under the same experimental conditions (D).
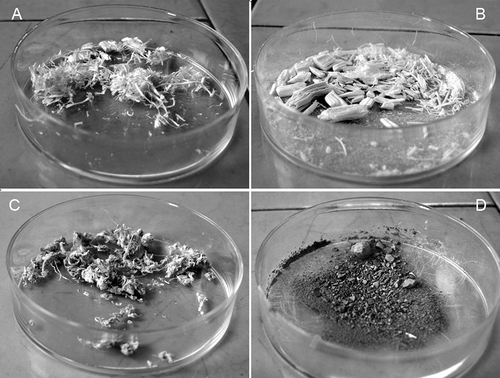
FIGURE 2. SEM image of natural and sonochemically modified chrysotile: Chrysotile fibers were untreated (A), leached with Ox for 21 h at 50°C (B), and sonicated for 21 h in deionized water (C). Synergistic effects of Ox and power US for 12 h (D) and 21 h (E) generate micrometric nonfibrous debris. Some rod-shaped nanocrystals, likely made of SiO2, are visible at higher magnification upon flat surfaces of chrysotile treated with Ox and US for 21 h (F).
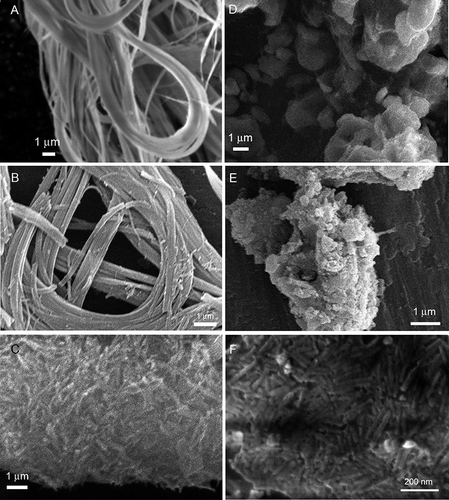
FIGURE 3. Two possible mechanisms of acoustic cavitation in liquid–solid systems: (A) Particle size is severalfold larger than resonant bubble size, and the particle creates an asymmetry of the environment near the surface, which induces, during bubble collapse, a rapidly moving stream of liquid through the cavity at the surface with velocity grater than 100 m/s. Such impacts are often used to clean surfaces and are responsible for the characteristic pitting in the surface. (B) Particles are smaller than approximately 200 μm (with US operating at approximately 20 kHz), and the shock waves created by homogeneous cavitation accelerate particles and induce high-energy interparticle collisions.
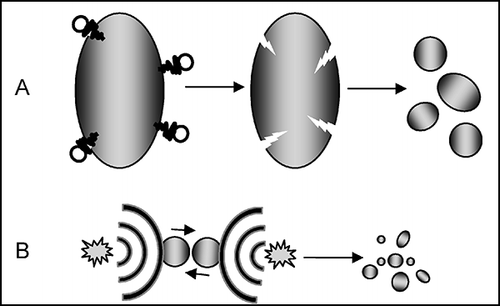
Crystallographic Modification Induced by Sonochemical Treatment
The x-ray diffraction patterns of untreated Val Malenco chrysotile fibers is illustrated (, pattern a) and compared to fibers treated with US only for 21 h (b) and to US–Ox for 12 and 21 h (c and d, respectively). Untreated fibers displayed the well-characterized x-ray diffractogram of chrysotile reported in the standards from the Joint Committee of Powder Diffraction (JCPD) archive. Serpentine main reflections (002 and 004, marked with § in the figure) were unaffected by US in pure water, which only induced a reduction in size. When Ox was added and the suspension sonicated for 12 and 21 h (patterns c and d, respectively), the crystallographic features of serpentine were suppressed and only reflexes due to magnesium oxalate were recorded (marked with asterisk in figure). Some oxalate reflexes around 2θ = 44° are superimposed with a doublet due to the occurrence of some steel debris from the ultrasonic reactor.
FIGURE 4. X-ray patterns of natural (a) and treated chrysotile fibers from Val Malenco. Asbestos was treated with US only for 21 h (b) or a combination US and 0.5 M Ox for 12 and 21 h (c, d). Recorded patterns were compared with natural fibers of chrysotile from Balangero (e) and treated with US + 0.5 M Ox for 21 h (f); § indicates reflections due to chrysotile, and asterisk indicates reflections due to Mg oxalate.
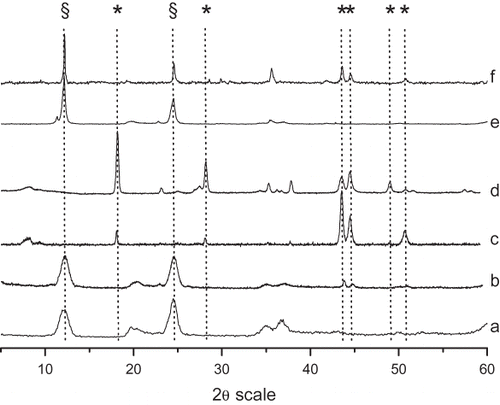
Diffractograms of chrysotile from Balangero untreated and US–Ox treated for 21 h are shown for comparison (e and f, respectively). As previously reported, the crystallographic features of serpentine (§) are still visible in the US–Ox treated sample, indicating the occurrence of some antigorite that was resistant to the sonochemical treatment. Note that XRPD did not distinguish between chrysotile and antigorite.
Spectroscopic Characterization of Solid Residues
Micro-Raman is by far the easiest spectroscopy technique able to characterize asbestos fibers and resolve serpentine polymorphs (CitationGroppo et al., 2006). In the representative spectra of chrysotile fibers untreated (a), sonicated for 21 h (b), leached for 21 h with Ox (c), and US–Ox treated for 21 h (d) are reported. Spectrum a shows the two most intense bands for SiO4 bending at 390cm−1 (antisymmetric ν5(e)) and 693cm−1 (symmetric ν2(a1)). As expected, both spectra b and c, recorded with fibers treated with US or Ox, respectively, show the chrysotile-related SiO4 vibrational features. Following US–Ox combined treatment, spectrum d, the chrysotile peaks are not recorded and only magnesium oxalate is detected (CitationFrost, 2004). It is noteworthy that in this sample, in opposite fashion to what was noted for chrysotile from Balangero, antigorite was not detected, while in both samples SEM imaging showed the occurrence of some rod-shaped nanostructure. These nanofibers were previously postulated to consist of antigorite, but these fibers also occur in the antigorite-free chrysotile, indicating that they are likely made of amorphous silica, providing further assurance regarding the safety of the treated product.
FIGURE 5. Raman spectra of chrysotile fibers that were untreated (a), sonicated for 21 h (b), leached with Ox for 21 hr (c), and treated with US–Ox for 21 h (d). A polarized solid-state Nd 80-mW laser operating at 532.11 nm was used as the excitation source. Representative spectra from at least 30 independent measurements are reported. Principal vibrational features due to chrysotile (§) and Mg oxalate (asterisk) are shown for each spectrum.
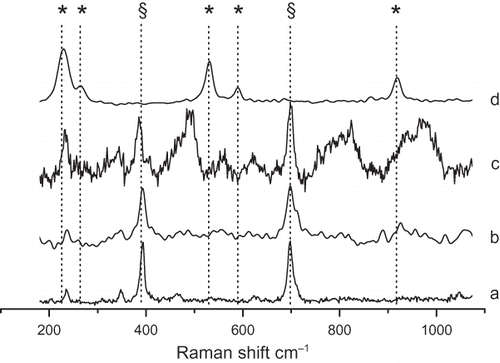
Elemental Analysis
The relative amount of Mg with respect to Si is a well-established approach to describe depletion of brucitic layer in chrysotile and investigate the congruence/incongruence of mineral dissolution (CitationFavero-Longo et al., 2005; CitationTurci et al., 2007a). X-ray fluorescence spectra of the two untreated chrysotiles and some treated samples were collected and the intensity of integrated Mg peak was normalized by the Si peak (). Val Malenco fibers were significantly affected by US in pure water (1.24 ± 0.03) with respect to the untreated mineral (1.47 ± 0.03), whereas the Balangero ones are not significantly modified by treatment. The combination of Ox and US induced an almost complete removal of Mg from the Val Malenco sample after a 12-h treatment (0.2 ± 0.2), supporting the view that the rod-shaped nanometric particles are made of amorphous silica. A similar result was obtained by leaching chrysotile under extreme conditions, i.e., HCl solution at 3 M at 373 K (CitationWang et al., 2006). The Mg/Si ratio measured on the sample from Balangero after the US–Ox treatment (0.83 ± 0.02) was significantly higher than that found for the Val Malenco one, likely due to the occurrence of antigorite which does not undergo complete solubilization during US–Ox treatment (CitationTurci et al., 2008).
CONCLUSIONS
While the toxicity of airborne asbestos fibers is well established, the hazard for humans of waterborne fibers remains unknown. Nevertheless, a precautionary principle indicates that control of waterborne asbestos fiber concentrations is required if possible. An innovative method for removing serpentine asbestos (i.e., chrysotile) from water suspensions previously reported was further validated. Elemental analyses and crystallographic patterns indicated the complete disruption of chrysotile fibres from Val Malenco (Italian Central Alps) after only 12 h of treatment. No crystalline phase or vibrational feature other than the precipitated magnesium oxalate was detected in the final product obtained. The amorphous disrupted material consisted of silica debris, as silicon is the predominant element by far. Amorphous silica is generally considered nontoxic compared to the crystalline counterpart, which produces silicosis and lung cancer (CitationInternational Agency for Research on Cancer, 1997; CitationVallyathan et al., 1998), as recently reaffirmed by the 2009 Monograph Working Group of WHO International Agency for Research on Cancer (CitationStraif et al., 2009). Amorphous silica was occasionally shown to produce transient pulmonary inflammation (CitationWarheit et al., 1995; CitationInternational Agency for Research on Cancer, 1997). In the context of nanotoxicology, some nanosized amorphous silicas were recently reported to induce pulmonary inflammation in mice and some cytotoxicity in vitro (CitationKaewamatawong et al., 2005; CitationYu et al., 2009). However, US–Ox processing of the most widespread and potent of human carcinogens, chrysotile asbestos, yielded some noncrystalline silica particles, a few of which were nanometric in size. The obtained material was thus recognized as being noncarcinogenic for humans, and this process is effective in diminishing toxicity. In vitro tests may add further evidence of the safety of the final product, which could eventually be validated in vivo. The parallel loss of fibrous habit, evidenced by SEM imaging, further accounts for the loss of potential hazard of post-treatment mineral debris. Current findings confirm previously reported data for the chrysotile specimen from Balangero (Italian Western Alps) and clarify the amorphous nature of some rod-shaped nanoparticles found in both samples upon the US–Ox treatment.
Notes
The micro-Raman and XRF measures have been obtained with the equipment acquired by the Interdepartmental Center “G. Scansetti” for Studies on Asbestos and Other Toxic Particulates with a grant from Compagnia di San Paolo, Torino, Italy. The authors acknowledge Danacamerini SAS (Torino, Italy) for engineering the US reactor.
REFERENCES
- Anastasiadou , K. and Gidarakos , E. 2007 . Toxicity evaluation for the broad area of the asbestos mine of northern Greece . J. Hazard. Mater. , 139 : 9 – 18 .
- Buzio , S. , Pesando , G. and Zuppi , G. M. 2000 . Hydrogeological study on the presence of asbestos fibres in water of northern Italy . Water Res. , 34 : 1817 – 1822 .
- Cantor , K. P. 1997 . Drinking water and cancer . Cancer Causes Control , 8 : 292 – 308 .
- Dearwent , S. M. , Mumtaz , M. M. , Godfrey , G. , Sinks , T. and Falk , H. 2006 . Health effects of hazardous waste . Ann. NY Acad. Sci. , 1076 : 439 – 448 .
- Doktycz , S. J. and Suslick , K .S. 1990 . Interparticle collisions driven by ultrasound . Science , 247 : 1067 – 1069 .
- Favero-Longo , S. E. , Turci , F. , Tomatis , M. , Castelli , D. , Bonfante , P. , Hochella , M. F. , Piervittori , R. and Fubini , B. 2005 . Chrysotile asbestos is progressively converted into a non-fibrous amorphous material by the chelating action of lichen metabolites . J. Environ. Monit. , 7 : 764 – 766 .
- Favero-Longo , S. E. , Turci , F. , Tomatis , M. , Compagnoni , R. , Piervittori , R. and Fubini , B. 2009 . The effect of weathering on ecopersistence, reactivity, and potential toxicity of naturally occurring asbestos and asbestiform minerals . J. Toxicol. Environ. Health A , 72 : 305 – 314 .
- Frost , R. L. 2004 . Raman spectroscopy of natural oxalates . Anal. Chim. Acta , 517 : 207 – 214 .
- Groppo , C. , Rinaudo , C. , Cairo , S. and Gastaldi , D. 2006 . Micro-Raman spectroscopy for a quick and reliable identification of serpentine minerals from ultramarics . Eur. J. Mineral. , 18 : 319 – 329 .
- International Agency for Research on Cancer . 1977 . Monographs on the evaluation of the carcinogenic risk of chemicals to man: Asbestos , 14th , 1 – 106 . Lyon, , France : IARC .
- International Agency for Research on Cancer . 1997 . Monograph on the evaluation of the carcinogenic risk of chemicals to humans: Silica, some silicates, coal dusts para-amid fibrils , 1 – 475 . Lyon, , France : IARC .
- Kaewamatawong , T. , Kawamura , N. , Okajima , M. , Sawada , M. , Morita , T. and Shimada , A. 2005 . Acute pulmonary toxicity caused by exposure to colloidal silica: Particle size dependent pathological changes in mice . Toxicol. Pathol. , 33 : 743 – 749 .
- Kazan-Allen , L. 2005 . Asbestos and mesothelioma: Worldwide trends . Lung Cancer , 49 ( suppl. 1 ) : S3 – S8 .
- Kjaerheim , K. , Ulvestad , B. , Martinsen , J. I. and Andersen , A. 2005 . Cancer of the gastrointestinal tract and exposure to asbestos in drinking water among lighthouse keepers (Norway) . Cancer Causes Control , 16 : 593 – 598 .
- Lee , R. J. , Strohmeier , B. R. , Bunker , K. L. and Van Orden , D. R. 2008 . Naturally occurring asbestos: a recurring public policy challenge . J. Hazard. Mater. , 153 : 1 – 21 .
- McDonald , J. C. , Harris , J. and Armstrong , B. 2004 . Mortality in a cohort of vermiculite miners exposed to fibrous amphibole in Libby, Montana . Occup. Environ. Med. , 61 : 363 – 366 .
- Millette , J. R. , Clark , P. J. , Stober , J. and Rosenthal , M. 1983 . Asbestos in water supplies of the United States . Environ. Health Perspect. , 53 : 45 – 48 .
- Mossman , B. T. , Bignon , J. , Corn , M. , Seaton , A. and Gee , J. B. 1990 . Asbestos: scientific developments and implications for public policy . Science , 247 : 294 – 301 .
- Pan , X. L. , Day , H. W. , Wang , W. , Beckett , L. A. and Schenker , M. B. 2005 . Residential proximity to naturally occurring asbestos and mesothelioma risk in California . Am. J. Respir. Crit. Care Med. , 172 : 1019 – 1025 .
- Peipins , L. A. , Lewin , M. , Campolucci , S. , Lybarger , J.A . , Miller , A. , Middleton , D. , Weis , C. , Spence , M. , Black , B. and Kapil , V. 2003 . Radiographic abnormalities and exposure to asbestos-contaminated vermiculite in the community of Libby, Montana, USA . Environ. Health Perspect. , 111 : 1753 – 1759 .
- Spurny , K. R. , Stoeber , W. , Opiela , H. and Weiss , G. 1979 . Size-selective preparation of inorganic fibers for biological experiments . Am. Ind. Hyg. Assoc. J. , 40 : 20 – 38 .
- Spurny , K. R. , Stoeber , W. , Opiela , H. and Weiss , G. 1980 . On the problem of milling and ultrasonic treatment of asbestos and glass fibers in biological and analytical applications . Am. Ind. Hyg. Assoc. J. , 41 : 198 – 203 .
- Straif , K. , Brahim-Tallaa , L. , Baan , R. , Grosse , Y. , Secretan , B. , El Ghissassi , F. , Bouvard , V. , Guha , N. , Freeman , C. , Galichet , L. and Cogliano , V. 2009 . A review of human carcinogens—Part C: Metals, arsenic, dusts, and fibres . Lancet Oncol. , 10 : 453 – 454 .
- Suslick , K. S. 1990 . Sonochemistry . Science , 247 : 1439 – 1445 .
- Turci , F. , Favero-Longo , S. E. , Tomatis , M. , Martra , G. , Castelli , D. , Piervittori , R. and Fubini , B. 2007a . A biomimetic approach to the chemical inactivation of chrysotile fibres by lichen metabolites . Chemistry , 13 : 4081 – 4093 .
- Turci , F. , Tomatis , M. , Mantegna , S. , Cravotto , G. and Fubini , B. 2007b . The combination of oxalic acid with power ultrasound fully degrades chrysotile asbestos fibres . J. Environ. Monit. , 9 : 1064 – 1066 .
- Turci , F. , Tomatis , M. , Mantegna , S. , Cravotto , G. and Fubini , B. 2008 . A new approach to the decontamination of asbestos-polluted waters by treatment with oxalic acid under power ultrasound . Ultrason. Sonochem. , 15 : 420 – 427 .
- U.S. Environmental Protection Agency . 2003 . Asbestos mechanisms of toxicity workshop Chicago 12–13 June 2003
- U.S. Environmental Protection Agency . 2005 . Mechanisms of action of inhaled fibers, particles, and nanoparticles in lung and cardiovascular disease , Research Triangle Park, NC : EPA Conference Center . 25–28 October 2005
- U.S. Environmental Protection Agency. 2006. National primary drinking water regulations— Consumer fact sheet on: Asbestos http://www.epa.gov/ogwdw/dwh/c-ioc/asbestos.html
- Vallyathan , V. , Green , F. , Ducatman , B. and Schulte , P. 1998 . Roles of epidemiology, pathology, molecular biology and biomarkers in the investigation of occupational lung cancer . J. Toxicol. Environ. Health B , 1 : 91 – 116 .
- Van Gosen , B. S. 2007 . The geology of asbestos in the United States and its practical applications . Environ. Eng. Geosci. , 13 : 55 – 68 .
- Wang , L. , Lu , A. , Wang , C. , Zheng , X. , Zhao , D. and Liu , R. 2006 . Nano-fibriform production of silica from natural chrysotile . J. Colloid Interface Sci. , 295 : 436 – 439 .
- Warheit , D. B. , Mchugh , T. A. and Hartsky , M. A. 1995 . Differential pulmonary responses in rats inhaling crystalline, colloidal or amorphous silica dusts . Scand. J. Work Environ. Health , 21 : 19 – 21 .
- Webber , J. S. 1993 . “ Asbestos-contaminated drinking water ” . In Handbook of hazardous materials , Edited by: Corn , M. 29 – 44 . San Diego, CA, , USA : Academic Press, Inc .
- Webber , J. S. , Syrotynski , S. and King , M. V. 1988 . Asbestos-contaminated drinking water: Its impact on household air . Environ. Res. , 46 : 153 – 167 .
- World Health Organization . 2006 . Guidelines for drinking-water quality, Vol. 1, Recommendations , 3rd , Geneva, , Switzerland : WHO Press .
- Yu , K. O. , Grabinski , C. M. , Schrand , A. M. , Murdock , R. C. , Wang , W. , Gu , B. H. , Schlager , J. J. and Hussain , S. M. 2009 . Toxicity of amorphous silica nanoparticles in mouse keratinocytes . J. Nanoparticle Res. , 11 : 15 – 24 .