ABSTRACT
During the 2010 Deepwater Horizon (DWH), oil spill an estimated 800 million L oil and 7.9 million L dispersant entered the environment. The potential adverse effects of the oil-dispersant mixture are poorly understood. The aim of this study was to investigate the impacts of this mixture on early development of sheepshead minnow (Cyprinodon variegatus), a small-bodied estuarine fish commonly found in the area affected by the DWH spill. Embryos were exposed to a chemically enhanced water accommodated fraction (CEWAF; 10:1 mixture of Macondo oil; Corexit 9500) for 48 hr, after which organisms were maintained in uncontaminated water for an additional 8 days. Impacts were assessed on embryonic (heart rate, development of eye pigmentation, embryonic movement measured) and larval (time to hatch, larval survival, standard length, and cyp1a gene expression) development. No significant alterations were found in survival, time to hatch or cyp1a at the end of the experiment. However, CEWAF induced significant decreases in heart rate of embryos, delayed development of eye pigmentation, reduced embryonic movement, and diminished standard length. These results indicate potential sublethal adverse consequences attributed to CEWAF exposure during early development, even in the absence of maintained cyp1a induction or survival rate, potentially affecting the fitness of organisms later in life.
Introduction
As a result of the Deepwater Horizon (DWH), oil spill an estimated 5 million barrels (approximately 800 million L) of crude oil spilled into the Gulf of Mexico (GoM) between 20 April and 19 September 2010 (Bozeman, Citation2011). In addition, approximately 7.9 million L of dispersants [Corexit 9527 (surface application) and Corexit 9500A (surface and well-head application] were applied in the aftermath of the spill (Kujawinski et al., Citation2011; Allan et al., Citation2012). Dispersants lower the interfacial tension between water and oil, thereby reducing the size of oil micelles and increasing the bioavailability of oil for microbial degradation (Kujawinski et al., Citation2011; Boopathy et al., Citation2012). The environmental distribution of the oil throughout the GoM has been significant. Shoreline assessment teams indicated that between 1,773 and 2,100 km of shoreline was affected by the oil spill (Michel et al., Citation2013; Beyer et al., Citation2016), and two years after the spill, oil was still present on 687 km of shoreline (Michel et al., Citation2013). Moreover, the crude oil, and associated polycyclic aromatic hydrocarbons (PAH), was detected in the sediment directly and 1.5 years after the spill (Kirman et al., Citation2016; Turner et al., Citation2014). Depending upon the depth of the sediment, crude oil PAH may remain in place for years (Tansel et al., Citation2011). Adverse impacts of the oil spill on organisms were noted in the field, ranging from deep-water to salt-marsh organisms. For example, a deep-water coral community in the proximity of the well exhibited widespread signs of stress including tissue loss and bleaching (White et al., Citation2012). A field study on the Gulf killifish (Fundulus grandis), a small-bodied estuarine species commonly found in the GoM, noted adverse impacts in gill morphology as well as modulation in gene expression (Whitehead et al., Citation2011). Visible oiling was reported for approximately 8.6% of shorebirds trapped during the fall 2010 to spring 2011 non-breeding season, potentially resulting in sublethal impacts, including reduced reproduction and immunosuppression, and ultimately mortality (Henkel et al., Citation2012).
There has been specific concern regarding the impact of the oil spill on early life stages of fish. These life stages were shown to be sensitive to contaminants, and adverse effects are predictive for long-term toxicity (McKim, Citation1977; Hutchinson et al., Citation1998). Several studies investigated adverse effects of the DWH oil spill on the early development of fish (Dubansky et al., Citation2013; Incardona et al., Citation2014; Rico-Martínez et al., Citation2013; Wu et al. Citation2012; Denslow et al., Citation2015). Exposure of embryonic Gulf killifish to field collected sediment resulted in smaller length at hatch as well as bradycardia (Dubansky et al., Citation2013). Exposure of a variety of large predatory pelagic fish embryos to oil from the DWH spill exhibited abnormalities in cardiac functioning (Incardona et al., Citation2014).
Because Corexit was applied after the DWH spill, it is conceivable that fish were exposed to a combination of oil and Corexit. This has the potential to lead to altered responses (Rico-Martínez et al., Citation2013). Wu et al. (Citation2012) found that increased toxicity was observed in juvenile rainbow trout (Oncorhynchus mykiss) when fish were exposed to a combination of oil and Corexit compared to oil only. Similarly, exposure to Corexit 9500 and oil elevated the LC50 by 20-fold compared to crude oil exposure without dispersants in sheepshead minnow (Cyprinodon variegatus) and menidia (Menidia beryllina) (Denslow et al., Citation2015). When exposing Menidia embryos to sub-lethal concentrations, Denslow et al. (Citation2015) noted that exposure led to developmental abnormalities including lower number of heart beats/min. The goal of the current study was to investigate lethal and sublethal effects of a transient, acute exposure to a mixture of Macondo oil and Corexit 9500A on early development of sheepshead minnow, a species frequently found in the area impacted by the DWH oil spill.
Methods
Dilution water and animal holding
Parent animals were held at the Gulf Coast Research Laboratory of the University of Southern Mississippi (Ocean Springs, MS, USA) under flow-through conditions. Fish were held under estuarine conditions using artificial seawater and kept under a 16:8-hr light:dark photoperiod. Artificial seawater (ASW, 15 ppt) was synthesized using a mixture of Fritz super concentrate two-part salt (Fritz Aquatics, Mesquite, TX, USA) and Tru-Soft water softener pellets (United Salt, Houston TX, USA), and non-chlorinated well water. A stock of adult sheepshead minnow was maintained in 300-L static raceways. Water quality was monitored daily; salinity was between 14 and 16 ppt, temperature 26 ± 1°C, and dissolved oxygen between 5 and 7 mg/L. Fish were fed twice daily using Ziegler’s Maintenance flake food (Zieglers Bros., Inc., Gardners, PA, USA) and brine shrimp nauplii (Artemia franciscana).
Chemically enhanced water accommodated fraction preparation
The chemically enhanced water accommodated fraction (CEWAF) was produced following methods provided by The Chemical Response to Oil Spill: Ecological Effects Research Forum (Aurand and Coelho Citation2006). CEWAF stock (100%) was prepared by adding 1 g/L Macondo crude oil (supplied by British Petroleum) to ASW. Corexit 9500A was subsequently injected into the bottle at a dose of 10% oil by volume. This mixture was sealed with aluminum foil and then stirred for 18 hr on a stir plate at 20–25% vortex. Following the vortex period, CEWAF was allowed a 3-hr settling period in a 1L separatory funnel, after which the mixture was transferred into an amber bottle and used immediately. The first 15 ml drawn from the funnel was always discarded to ensure no separation effects from the small diameter at the bottom of the funnel. The 12.5% concentrations were made by mixing 875 ml ASW with 125 ml 100% CEWAF stock.
A SpectraMax® M5 Series Multi-Mode Microplate Reader (Molecular Devices, Sunnyvale, CA) was used to measure fluorescence emitted by PAH. At the start of the experiment, two water samples were taken from the stock solutions (Control, 12.5% and 100% CEWAF). At the end of the experiment, water samples were collected from the exposure containers (two samples/Petri dish). All samples were stored for 24–48 hr at 4°C until further analyses. Samples were analyzed using a method developed by Greer et al. (Citation2012). In summary, 3.5 ml samples CEWAF were collected and added to 3.5 ml ethanol in a glass scintillation vial. Next, samples were sonicated for 3 min to minimize hydrocarbon adhesion to the container, and subsequently centrifuged at 9,100 g for 10 min to remove salt particles. A 5-ml aliquot was drawn from the sample and placed into a quartz cuvette for analysis. In order to create a calibration curve, 750 ml serial dilutions of CEWAF were prepared. Results are shown in Table S1. Mean normalized relative fluorescence decreased at both concentrations over the 48-hr exposure period, but more rapidly in the 12.5% CEWAF concentration (reduction of 68.9% and 33.4% in the 12.5% and 100% CEWAF treatments, respectively). Due to technical and financial constraints, a full analysis of PAH concentrations was not performed for this test. However, such an analysis was performed for another experiment within 1 week of this one using identical preparation methods and oil source. For this experiment, 100% CEWAF stock synthesized from source oil was analyzed using GC-MS by ALS analytical (Kelso, WA, USA). In this identical dataset, the concentration of a suite of 50 selected PAH was measured to be 1,250 µg/L (Griffitt, personal. communication.). Using this concentration in combination with fluorescence data reported in Table S1, the sum of PAH in the low concentration exposures was estimated to be 257 µg/L at initiation of the experiment. Briefly, fluorescence data were collected from both the measured stock and the stock utilized in the current experiment. By calculating the slope of the relationship between dilution factor and fluorescence, and benchmarking the 100% stock against the measured stock, it was possible to calculate an estimated sum PAH value for the test solutions employed in this experiment.
Exposure design
Eggs at 24-hr post-fertilization (hpf) were collected from the breeding stock. Eggs were collected and kept in a 2-L Maybelle jar at 14 ppt and inside an incubator at 27°C at 16:8-hr light:dark photoperiod. At 48 hpf, 10 eggs were placed in covered glass Petri dishes (100 mm diameter) containing 200 ml of one of three treatments: Control, 12.5% CEWAF; 100% CEWAF (3 replicate Petri dishes/treatment). Exposure was conducted under static conditions for 48 hr (until 96 hpf). Eggs were maintained at 27°C, pH 8.5, DO > 6 mg/L and 14 ppt salinity. At 96 hpf, the embryos were transferred to clean ASW, with a water change 6 days after the start of the treatment [or 8 days post-fertilization (dpf)]. The developing embryos therefore experienced a 48-hr transient exposure to CEWAF from 48 to 96 hpf. The hatched larvae were fed two drops of Rotifers each day. At 10 days after initial exposure (8 days of depuration, or 12 dpf), the experiment was terminated, and larvae were stored in 1 ml RNAlater and kept in a freezer for future molecular analyses.
Effects on embryonic development were determined by quantifying heart rate, the presence of eye-pigmentation, and fin and mouth movements. At 72, 96, and 120 hpf, the embryonic heart rate was determined by placing Petri dishes under a dissection microscope at twofold magnification and counting the number of heart beats over a 10-sec period. At 72 hpf (24-hr exposure), embryonic eye pigmentation was semi-quantitatively scored as partial or full eye pigmentation. At 96 and 120 hpf, both embryonic fin and mouth movement were assessed under a dissection microscope at twofold magnification. For each individual, the absence or presence of fin and mouth movement was recorded. To assess impacts on larvae, time to hatch (assessed once a day, at the start of the day), standard length at time of sacrifice (SL: ±0.5 mm), and survival were determined.
Cytochrome P450 1A (cyp1a) expression
The effect of CEWAF on cyp1a expression was determined using qPCR, with 18S as the internal reference gene. Whole larvae from each experimental replicate were pooled (10 individuals per replicate), homogenized, and total RNA extracted using TRIzol reagent (Ambion Inc, Austin, TX.) following the manufacturer’s provided protocol. Total RNA concentration was quantified via Nanodrop 1000 (ThermoScientific, Waltham, MA), then DNAse treated (DNase I, Fermentas Inc., Burlington, ON, Canada), and reverse transcribed to cDNA using 2.5 µg total RNA per reaction and the Applied Biosystems High Capacity cDNA Reverse Transcription Kit (Applied Biosystems, Waltham, MA). The qPCR primers were according to Hendon et al. (Citation2008). Reactions were performed in a 96-well plate with a 20 μl total reaction volume comprised of 10 µl Master mix (iQ™ SYBR Green Supermix, Bio-Rad Laboratories, Hercules, CA), 10 µM forward and reverse primers, 100 ng cDNA template, and nuclease-free water to fill the remaining volume. Three biological replicates were analyzed in duplicate per treatment. All reactions were performed on a Bio-Rad CFX96 real-time PCR detection system (Bio-Rad Laboratories) using the following conditions: initial template denaturation at 95°C for 3 min, 40 cycles of 95°C for 10 sec, primer annealing at 60°C for 30 sec, product extension at 72°C for 30 sec, and final extension at 65°C to 95°C in increments of 0.5°C for 5 sec. Bio-Rad CFX 2.1 software was used for real-time qPCR data acquisition and analysis, and relative expression of each target gene was calculated by normalization to the reference gene.
Statistical analyses
Petri dishes were used as the unit of replication to avoid pseudoreplication. All data are reported as mean ± standard error of mean (SEM) and analyzed using SPSS (IBM SPSS Statistics, v. 23, Armonk, NY). The criterion for statistical significance was set at α = 0.05. Time to hatch, cyp1a gene expression, standard length, and embryonic heart rate were analyzed using analysis of variance (ANOVA). Assumptions of normality and homogeneity of variance were checked using Shapiro–Wilk’s W-test and Levene’s test, respectively. In several cases, the assumption of normality was violated (heart rate at 72 h and 120 h, time to hatch, and length), even after data transformation (log). However, as ANOVA is robust for violation of assumptions, analyses were continued, but caution was taken if p values were close to α. If significant differences were observed, a Dunnett’s test was conducted to compare CEWAF treatments to control. For nominal response variables, eye pigmentation, mouth movement, fin movement, and survival data were analyzed by comparing treatments to controls using a Fisher’s exact test, with data grouped across replicates.
Results
CEWAF produced a significant decrease in embryo heart rate at 72, 96, and 120 hpf (). There were significantly fewer embryos with full eye pigmentation in the organisms exposed to 100% CEWAF (46.7%) compared to controls (86.7%) (). No marked differences in number of embryos with eye pigmentation were observed between organisms exposed to 12.5% CEWAF and controls (). At 96 hpf, there was a significant reduction in embryos with fin movement between controls (80% of embryos) and 100% CEWAF (30%) (). No marked differences were detected between control and 100% CEWAF at 120 hpf, and between control and 12.5% CEWAF at both time points (). The number of embryos with mouth movement was significantly reduced in fish exposed to both concentrations of CEWAF at 96 and 120 hpf ().
Table 1. Impact on embryonic Development of Sheepshead Minnow (Cyprinodon variegatus) exposed to a chemically enhanced water accommodated fraction (CEWAF).
Figure 1. Heart rate (n = 3 Petri dishes +/− SEM) at 72, 96, and 120 hours post-fertilization (hpf) in sheepshead minnow embryos exposed to 0% (controls), 12.5% and 100% chemically enhanced water accommodated fraction (CEWAF). At each individual time point, significant difference compared to control is indicated by the asterisk sign (analysis of variance followed by Dunnett’s post hoc comparisons). The criterion for significance was set at p < 0.05.
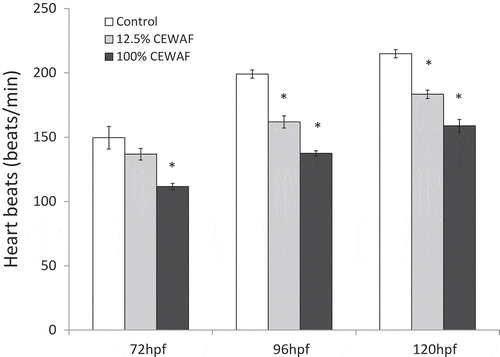
The average time to hatch was 5.5 days in controls and numerically increased to 5.8 and 6.1 when exposed to 12.5 and 100% CEWAF, respectively, but this difference was not statistically significant (). After 8 days of depuration, larvae standard length was significantly decreased by exposure to 100% CEWAF (). Follow-up post hoc analyses found a significant 7.1% reduction in standard length in larvae exposed to 100% CEWAF compared to controls with no marked difference between larvae exposed to 12.5% CEWAF vs. controls (). At the end of the experiment, no significant difference in survival was noted with survival at ≥90% in all treatments. In addition, at 11 dpf, there was no marked change in cyp1a gene expression in fish exposed to CEWAF compared to control after 8 days depuration (Figure S1).
Table 2. Impact on days to hatch (n = 3 petri dishes +/- SEM), larval survival at the end of the experiment (n = 30 Individuals), and standard length at hatch (n = 3 Petri Dishes +/− SEM) of larval sheepshead minnow (cyprinodon variegatus) exposed to chemically enhanced water accommodated fraction (CEWAF)
Discussion
Acute 48-hr exposure of sheepshead minnow embryos to a mixture of Macondo oil and Corexit 9500A resulted in sublethal alterations during embryo development including reduction in embryo movement, bradycardia, and retarded development as indicated by a fall in number of young with full eye pigmentation. Bradycardia is thought to be one of the primary responses of embryos to crude oil. Research on embryonic Pacific herring (Clupea pallasi) exposed to crude oil demonstrated a dose-dependent reduction in heart rate (Incardona et al., Citation2008). Moreover, exposure of embryos from a variety of large predatory pelagic fish [bluefin tuna (Thunnus thynnus), yellowfin tuna (Thunnus albacares), and greater amberjack (Seriola dumerili)] to DWH crude oil produced cardiac dysfunction in all three species (Incardona et al., Citation2014). Further, Gulf killifish, another important euryhaline species found in the GoM, also exhibited a bradycardic response when exposed to sediments collected at oil sites (Dubansky et al., Citation2013). Crude oil, and associated PAH, seems to exert a direct effect on cardiac conduction resulting in bradycardia (Incardona et al., Citation2004, Citation2008, Citation2011). This may lead to adverse fitness effects later in life including elevated frequency of mortality in larvae and juveniles (Incardona and Scholz, Citation2016).
Similar to our results, a reduction in standard length of larvae was observed in sheepshead minnow exposed to Louisiana Crude Oil in a life cycle test, which persisted into juvenile and adult stages (Raimondo et al., Citation2016). A study on topsmelt (Atherinops affinis) exposed to Prudhoe Bay Crude Oil, either as water-accommodated fractions (WAF) or CEWAF, for 10 days showed similar adverse effects on standard length (Anderson et al., Citation2009). Data demonstrated that short-term exposure, followed by a depuration period, produced similar adverse effects on standard length. The reduction in embryos with full eye-pigmentation, fin movement, and mouth movement in organisms exposed to CEWAF indicates potential additional adverse developmental effects. Previously, Incardona et al. (Citation2004) noted reduced eye growth in zebrafish embryos exposed to PAH. A further study on beach spawning capelin (Mallotus villosus) demonstrated diminished eye-pigmentation when embryos were exposed to a water soluble fraction of crude oil (Paine et al., Citation1992). However, Frantzen et al. (Citation2012) examining the same species exposed to PAH found no marked delay or acceleration of formation of eye-pigmentation. The embryonic deformities observed did not result in increased mortality rate. However, Ron et al. (Citation2000) showed that pinked salmon (Oncorhynchus gorbuscha) which survived crude oil exposure, and even appeared healthy at hatch, demonstrated adverse impacts later in life such as delayed growth and diminished survival in the marine environment. Recent investigations by Garrido et al. (Citation2015) support these findings by reporting that smaller size at hatch resulted in an increased mortality rate later in life.
An upregulation of production of cytochrome P-4501A (CYP1A) in teleosts is a reliable bio-indicator of exposure to PAH or crude oil (van der Oost et al. Citation2003; Brewton et al., Citation2013). Field studies found significant upregulation of cyp1a in the Gulf killifish in oiled areas (Whitehead et al., Citation2011; Dubansky et al., Citation2013). No apparent studies to date have assessed cyp1a upregulation in sheepshead minnow after exposure to CEWAF, and in our study, no apparent change in cyp1a expression occurred after 8 days of depuration. It is worthwhile noting that cyp1a expression was determined at 8 dph, and fish were only exposed for a 48-hr window, between 38 hpf and 96 hpf. Data thus indicate that cyp1a upregulation in this species may only be considered an indicator during exposure, and not after depuration, but follow-up studies are required to verify this. In addition, a recent study on spotted seatrout (Cynoscion nebulosus) demonstrated a significant upregulation of cyp1a in larval spotted seatrout exposed to CEWAF compared to both controls and oil-exposed [high energy water accommodated fraction (HEWAF)] (Brewton et al., Citation2013). Interestingly, this response was not detected during juvenile stages, where a significant rise in cyp1a expression was found in HEWAF exposed juveniles compared to controls, but not in juveniles exposed to CEWAF (Brewton et al., Citation2013). Follow-up research on the use of cyp1a as a bio-indicator after depuration, as well as during different life stages, is therefore warranted.
In summary, although no marked change in mortality rate was observed in larval fish, significant sublethal effects were noted in embryos and larvae of sheepshead minnow following acute 48-hr exposure to a mixture of Macondo oil and Corexit dispersant. These sublethal effects included a reduction in embryo movement, bradycardia, and delayed development as evidenced by a decrease in number of young with full eye-pigmentation and delayed time to hatch. These impacts may consequently result in adverse effects on fitness of organisms later in life. However, follow-up research is needed to determine the long-term consequences of acute oil exposure during sheepshead minnow early development.
UTEH_A_1340208_Supplementary.docx
Download MS Word (29.4 KB)Acknowledgments
We would like to thank Robin Vroom, Binnaz Bailey, Danielle Simning, Jeremy Jackson, Jean Jovonovich Alvillar and Nancy Brown-Peterson for help during the conduction of the experiments. In addition, we would like to extend our thanks to the editor and reviewers, who provided valuable and detailed feedback on an earlier version of the manuscript.
Funding
This research was made possible by a grant from BP/The Gulf of Mexico Research Initiative (award number SA 13-01/GoMRI-009) awarded to TB, MSS, RJG, SDG and CP. The funders had no role in the design, execution, or analyses of this project. The data are archived at Gulf of Mexico Research Initiative Information and Data Cooperative.
Supplemental data
Supplemental data for this article can be accessed on the publisher’s website.
Additional information
Funding
References
- Allan, S. E., Smith, B. W., and Anderson, K. A. 2012. Impact of the Deepwater Horizon oil spill on bioavailable polycyclic aromatic hydrocarbons in Gulf of Mexico coastal waters. Environ. Sci. Technol. 46: 2033–2039.
- Anderson, B. S., Arenella-Parkerson, D., Phillips, B. M., Tjeerdema, R. S., and Crane, D. 2009. Preliminary investigation of the effects of dispersed Prudhoe Bay Crude Oil on developing topsmelt embryos, Atherinops affinis. Environ. Pollut. 157:1058–1061.
- Aurand D., Coelho, G. 2006. Cooperative aquatic toxicity testing of dispersed oil and the chemical response to oil spill: Ecological effects research forum (CROSERF). Technical Report 07-03. Ecosystems Management & Associates, Inc., Lusby, MD, p. 105.
- Beyer, J., Trannum, H. C., Bakke, T., Hodson, P. V., and Collier, T. K. 2016. Environmental effects of the Deepwater Horizon oil spill: A review. Mar. Pollut. Bull. 110: 28–51.
- Boopathy, R., Shields, S., and Nunna, S. 2012. Biodegradation of crude oil from the BP oil spill in the marsh sediments of southeast Louisiana, USA. Appl. Biochem. Biotechnol. 167: 1560–1568.
- Bozeman, B. 2011. The 2010 BP Gulf of Mexico oil spill: Implications for theory of organizational disaster. Technol. Soc. 33: 244–252.
- Brewton, R. A., Fulford, R., and Griffitt, R. J. 2013. Gene expression and growth as indicators of effects of the bp deepwater horizon oil spill on spotted seatrout (Cynoscion nebulosus). J. Toxicol. Environ. Health A 76: 1198–1209.
- Denslow, N. D., Adeyemo, O. K., and Kroll, K. J. 2015. Effect of oil and dispersants from the Gulf of Mexico on estuarine fish species. J. Environ. Indicat. 9: 10–11.
- Dubansky, B., Whitehead, A., Miller, J. T., Rice, C. D., and Galvez, F. 2013. Multitissue molecular, genomic, and developmental effects of the deepwater horizon oil spill on resident gulf killifish (Fundulus grandis). Environ. Sci. Technol. 47: 5074–5082.
- Frantzen, M., Falk-Petersen, I.-B., Nahrgang, J., Smith, T. J., Olsen, G. H., Hangstad, T. A., and Camus, L. 2012. Toxicity of crude oil and pyrene to the embryos of beach spawning capelin (Mallotus villosus). Aquat. Toxicol. 108:42–52.
- Garrido, S., Ben-Hamadou, R., Santos, A. M. P., Ferreira, S., Teodósio, M. A., Cotano, U., Irigoien, X., Peck, M. A., Saiz, E., and Ré, P. 2015. Born small, die young: Intrinsic, size-selective mortality in marine larval fish. Sci. Rep. 5: 17065.
- Greer, C.D., Hodson, P.V., Li, Z., King, T., and Lee, K. 2012. Toxicity of crude oil chemically dispersed in a wave tank of Atlantic herring (Clupea harengus). Environ. Toxicol. Chem. 31: 1324–1333.
- Hendon, L. A., Carlson, E. A., Manning, S., and Brouwer, M. 2008. Molecular and developmental effects of exposure to pyrene in the early life-stages of Cyprinodon variegatus. Comp. Biochem. Physiol. C: Toxicol. Pharmacol. 147: 205–215.
- Henkel, J. R., Sigel, B. J., and Taylor, C. M. 2012. Large-scale impacts of the Deepwater Horizon oil spill: Can local disturbance affect distant ecosystems through migratory shorebirds? Bioscience 62: 676–685.
- Hutchinson, T. H., Solbe, J., and Kloepper-Sams, P. J. 1998. Analysis of the ecetoc aquatic toxicity (EAT) database III — Comparative toxicity of chemical substances to different life stages of aquatic organisms. Chemosphere 36: 129–142.
- Incardona, J. P., Carls, M. G., Day, H. L., Sloan, C. A., Bolton, J. L., Collier, T. K., and Scholz, N. L. 2008. Cardiac arrhythmia is the primary response of embryonic Pacific herring (Clupea pallasi) exposed to crude oil during weathering. Environ. Sci. Technol. 43: 201–207.
- Incardona, J. P., Collier, T. K., and Scholz, N. L. 2004. Defects in cardiac function precede morphological abnormalities in fish embryos exposed to polycyclic aromatic hydrocarbons. Toxicol. Appl. Pharmacol. 196: 191–205.
- Incardona, J. P., Collier, T. K., and Scholz, N. L. 2011. Oil spills and fish health: Exposing the heart of the matter. J. Expo. Sci. Environ. Epidemiol. 21: 3–4.
- Incardona, J. P., Gardner, L. D., Linbo, T. L., Brown, T. L., Esbaugh, A. J., Mager, E. M., Stieglitz, J. D., French, B. L., Labenia, J. S., and Laetz, C. A. 2014. Deepwater Horizon crude oil impacts the developing hearts of large predatory pelagic fish. Proc. Nat. Acad. Sci. USA 111: E1510–E1518.
- Incardona, J. P., and Scholz, N. L. 2016. The influence of heart developmental anatomy on cardiotoxicity-based adverse outcome pathways in fish. Aquat. Toxicol. 177: 515–525.
- Kirman, Z. D., Sericano, J. L., Wade, T. L., Bianchi, T. S., Marcantonio, F., and Kolker, A. S. 2016. Composition and depth distribution of hydrocarbons in Barataria Bay marsh sediments after the Deepwater Horizon oil spill. Environ. Pollut. 214: 101–113.
- Kujawinski, E. B., Kido Soule, M. C., Valentine, D. L., Boysen, A. K., Longnecker, K., and Redmond, M. C. 2011. Fate of dispersants associated with the Deepwater Horizon oil spill. Environ. Sci. Technol. 45: 1298–1306.
- McKim, J. M. 1977. Evaluation of tests with early life stages of fish for predicting long-term toxicity. J. Fish. Res. Board Can. 34: 1148–1154.
- Michel, J., Owens, E. H., Zengel, S., Graham, A., Nixon, Z., Allard, T., Holton, W., Reimer, P. D., Lamarche, A., and White, M. 2013. Extent and degree of shoreline oiling: Deepwater Horizon oil spill, Gulf of Mexico, USA. PLoS One 8: e65087.
- Paine, M. D., Leggett, W. C., McRuer, J. K., and Frank, K. T. 1992. Effects of Hibernia crude oil on Capelin (Mallotus villosus) embryos and larvae. Mar. Environ. Res. 33: 159–187.
- Raimondo, S., Hemmer, B. L., Lilavois, C. R., Krzykwa, J., Almario, A., Awkerman, J. A., and Barron, M. G. 2016. Effects of Louisiana crude oil on the sheepshead minnow (Cyprinodon variegatus) during a life-cycle exposure to laboratory oiled sediment. Environ. Toxicol. 31: 1627–1639.
- Rico-Martínez, R., Snell, T. W., and Shearer, T. L. 2013. Synergistic toxicity of Macondo crude oil and dispersant Corexit 9500A® to the Brachionus plicatilis species complex (Rotifera). Environ. Pollut. 173: 5–10.
- Ron, A. H., Stanley, D. R., Alex, C. W., Robert, F. B., Frank, P. T., John, E. J., and Jeffrey, W. S. 2000. Delayed effects on growth and marine survival of pink salmon Oncorhynchus gorbuscha after exposure to crude oil during embryonic development. Mar. Ecol. Prog. Ser. 208: 205–216.
- Tansel, B., Fuentes, C., Sanchez, M., Predoi, K., and Acevedo, M. 2011. Persistence profile of polyaromatic hydrocarbons in shallow and deep Gulf waters and sediments: Effect of water temperature and sediment-water partitioning characteristics. Mar. Pollut. Bull. 62: 2659–2665.
- Turner, R. E., Overton, E. B., Meyer, B. M., Miles, M. S., McClenachan, G., Hooper-Bui, L., Engel, A. S., Swenson, E. M., Lee, J. M., Milan, C. S., and Gao, H. 2014. Distribution and recovery trajectory of Macondo (Mississippi Canyon 252) oil in Louisiana coastal wetlands. Mar. Pollut. Bull. 87: 57–67.
- van der Oost, R., Beyer, J., and Vermeulen, N. P. E. 2003. Fish bioaccumulation and biomarkers in environmental risk assessment: A review. Environ. Toxicol. Pharmacol. 13: 57–149.
- White, H. K., Hsing, P. Y., Cho, W., Shank, T. M., Cordes, E. E., Quattrini, A. M., Nelson, R. K., Camilli, R., Demopoulos, A. W. J., German, C. R., Brooks, J. M., Roberts, H. H., Shedd, W., Reddy, C. M., and Fisher, C. R. 2012. Impact of the Deepwater Horizon oil spill on a deep-water coral community in the Gulf of Mexico. Proc. Nat. Acad. Sci. USA 109: 20303–20308.
- Whitehead, A., Dubansky, B., Bodinier, C., Garcia, T. I., Miles, S., Pilley, C., Raghunathan, V., L., R. J., Walker, N., Walter, R. B., Rice, C. D., and Galvez, F. 2011. Genomic and physiological footprint of the Deepwater Horizon oil spill on resident marsh fishes. Proc. Nat. Acad. Sci. USA 108: 6193–6198.
- Wu, D., Wang, Z., Hollebone, B., McIntosh, S., King, T., and Hodson, P. V. 2012. Comparative toxicity of four chemically dispersed and undispersed crude oils to rainbow trout embryos. Environ. Toxicol. Chem. 31: 754–765.