ABSTRACT
The aim of this study was to (1) determine if the organochlorine artificial sweetener sucralose is metabolized in rat intestine with repeated dosing and (2) examine whether sucralose might bioaccumulate in rat adipose tissue. Sucralose was administered to 10 rats by gavage daily for 40 days at an average dosage of 80.4 mg/kg/day. The dosages were within the range utilized in historical toxicology studies submitted for regulatory approval in North America, Europe, and Asia. Feces and urine were collected individually from each animal for every 24-hr period during the 40-day dosing period. Analysis of the urine and fecal extracts by ultra-high performance liquid chromatography tandem mass spectrometry (UHPLC–MS/MS) revealed two new biotransformation products that have not previously been reported. These two metabolites are both acetylated forms of sucralose that are less polar and hence more lipophilic than sucralose itself. These metabolites were present in urine and feces throughout the sucralose dosing period and still detected at low levels in the urine 11 days after discontinuation of sucralose administration and 6 days after sucralose was no longer detected in the urine or feces. The finding of acetylated sucralose metabolites in urine and feces do not support early metabolism studies, on which regulatory approval was based, that claimed ingested sucralose is excreted unchanged (i.e. not metabolized). The historical metabolic studies apparently failed to detect these metabolites in part because investigators used a methanol fraction from feces for analysis along with thin layer chromatography and a low-resolution linear radioactivity analyzer. Further, sucralose was found in adipose tissue in rats two weeks after cessation of the 40-day feeding period even though this compound had disappeared from the urine and feces. Thus, depuration of sucralose which accumulated in fatty tissue requires an extended period of time after discontinuation of chemical ingestion. These new findings of metabolism of sucralose in the gastrointestinal tract (GIT) and its accumulation in adipose tissue were not part of the original regulatory decision process for this agent and indicate that it now may be time to revisit the safety and regulatory status of this organochlorine artificial sweetener
Introduction
The artificial sweetener sucralose is used globally as a sugar substitute to sweeten foods, beverages, and medications. Sucralose is a polar organochlorine compound with the chemical formula 1,6-dichloro-1,6-dideoxy-β-D-fructo-furanosyl-4-chloro-4-deoxy-α-D-galactopyranoside (). On a weight basis the sweetness potency of this chemical is approximately 600-fold greater than sucrose and thus contributes few or no calories to the products in which it is incorporated as an ingredient. Sucralose is currently used worldwide to sweeten thousands of low-calorie foods and beverages and has the dominant share of the United States market for artificial sweeteners and sugar substitutes (Lerner Citation2009).
Sucralose first gained regulatory approval in Canada in 1991 followed by approvals in Australia in 1993, New Zealand in 1996, the United States in 1998, and the European Union in Citation2004 (Schiffman and Rother Citation2013). Regulatory approval of sucralose was based upon early pharmacokinetic and metabolism studies that suggested the majority of orally ingested sucralose (65–95% depending upon the species tested) was not absorbed from the gastrointestinal tract (GIT) but rather excreted unchanged in the feces (U.S. Food and Drug Administration (US FDA) Citation1998). An additional conclusion was that the portion of ingested sucralose absorbed from the GIT was excreted unchanged in the urine or as a glucuronide conjugate with no marked dechlorination or degradative metabolism. These early metabolism investigations on which regulatory approval was based were ultimately published (Roberts et al. Citation2000; Sims et al. Citation2000).
The conclusions of the published data, from both rats (Sims et al. Citation2000) and humans (Roberts et al. Citation2000), that ingested sucralose is excreted unchanged (i.e. not metabolized) appear to be premature. First, the assertion that sucralose is not metabolized in the gut was based upon thin layer chromatography (TLC) and linear radioactivity scanner analysis of methanolic fecal extracts obtained after oral administration of 14C-sucralose to rats and humans. The TLC profiles of samples extracted from feces using methanol as the extracting solvent were reported to demonstrate that only sucralose and no metabolites were detected in fecal material (Roberts et al. Citation2000; Sims et al. Citation2000). However, metabolites of sucralose might potentially have occurred in the TLC profiles if solvents other than methanol were utilized for extraction. Some organochlorine compounds are only sparingly soluble or even insoluble in methanol at room temperature (Patel et al. Citation2012; US Pharmacopeia Citation2018; Brooks Citation2018). Thus, any methanol-insoluble metabolites of sucralose may not have been detected in fecal methanol extracts employed in the TLC analyses that were reported in previous investigations (Roberts et al. Citation2000; Sims et al. Citation2000). Secondly, the “major peak” in the TLC traces from methanolic fecal extracts that was noted to correspond solely to sucralose by Sims et al. (Citation2000) may actually have consisted of several poorly resolved peaks (Schiffman and Abou-Donia Citation2012; Schiffman and Rother Citation2013). At present it has not yet been determined if these multiple peaks represent compounds in addition to sucralose or are simply due to peak-splitting as a consequence of suboptimal experimental artifacts of the low-resolution linear radioactivity analyzers used in the historic studies. Examples of potential confounding artifacts include overlaying methanolic fecal extracts on the TLC plates resulting in differences in migration rate or limitations in accurately quantifying the radioactivity on TLC plates scanned with a linear radioactivity analyzer.
Another unresolved issue regarding the fate of ingested sucralose is whether this organochlorine sweetener bioaccumulates over time in fatty tissue with repeated consumption. Organochlorine compounds are well-known to accumulate in adipose tissue (Botella et al. Citation2004; Çok and Şatiroğlu Citation2004; Covaci et al. Citation2002; Skaare, Tuveng, and Sande Citation1988). It is noteworthy that sucralose was found in human breast milk at 0.04 µg/ml (0.1mM) (Sylvetsky et al. Citation2015) which exceeds the human sweet taste threshold for sucralose of 0.013mM (Schiffman, Sattely-Miller, and Bishay Citation2008). Previous metabolic studies in rats and humans reported that a single dose of sucralose was eliminated from the body within 5 days (Roberts et al. Citation2000; Sims et al. Citation2000). In rats administered a single dose of radiolabeled sucralose, 3–15% was excreted in urine, predominantly within the first 24 hr after dosing. The remainder of the single dose was excreted in the feces within 5 days (Sims et al. Citation2000). In humans treated with a single dose of radiolabeled sucralose (1 mg/kg), 9–22% was excreted in urine, predominantly within the first 24 hr after dosing with the majority excreted in feces (Roberts et al. Citation2000). In humans, 92.7% of the radioactive dose was excreted in urine and feces within 5 days; however, 7.3% of radiolabeled sucralose dose on average was not recovered in urine or feces. No apparent sucralose was detected in adipose tissue of rats by whole-body autoradiography at 15 min following a single intravenous (iv) dose of 100 mg/kg radiolabeled sucralose (Sims et al. Citation2000). These single dose investigations in rats and humans, however, did not address the potential retention and accumulation of sucralose in adipose tissue after multiple doses or recurring intake. Sucralose is an amphiphilic molecule that possesses lipophilic as well as hydrophilic properties (Schiffman and Rother Citation2013); hence it has the potential for bioaccumulation with habitual consumption.
The purpose of the present study was 2-fold: to 1) revisit the issue of whether sucralose is metabolized in rat intestine during and after 40 days of consecutive dosing with this compound, and 2) determine if sucralose might bioaccumulate in rat adipose tissue. The rat was selected as the animal model in this study because the United States Food and Drug Administration (U.S. Food and Drug Administration (US FDA) Citation1998) concluded that this species was the appropriate animal model for establishing the Acceptable Daily Intake (ADI) for sucralose in humans. The same rat species was used as in previous historical studies (Sims et al. Citation2000).
Methods
Animals
Ten Sprague-Dawley rats (5 males and 5 females, CD Strain 001) were obtained from Charles River Laboratories, Inc. USA. The animals were housed in individual metabolism cages (Ancare, Bellmore, NY) for separate collection of urine and feces at the animal facility of North Carolina State University College of Veterinary Medicine, Raleigh, North Carolina, USA. The animals were allowed to acclimate for 9 days (control period) before the sucralose dosing period began. At the beginning of the 9-day control period, the males ranged in weight from 160 to 405g, and the females ranged in weight from 265 to 315g. At the beginning of the sucralose dosing period, the males ranged in weight from 306 to 446g, and the females ranged in weight from 286 to 350g. The animals had unrestricted access to water and a purified, customized, uncontaminated rodent diet (AIN-93G Growth Purified Diet, Purina, St. Louis, MO). Animals were provided with white chew bones (Nylabones, Neptune City, NJ) in their cages to reduce any malocclusions. The water, Nylabones and rodent diet were screened by ultra-high performance liquid chromatography tandem mass spectrometry (UHPLC/MS/MS) to ensure that no chlorinated pesticide residues or other chlorinated compounds were present that might confound identification of sucralose and its potential metabolites. The protocol was approved by the Institutional Animal Care and Use Committee (IACUC) at North Carolina State University and was compliant with all animal health and ethics standards.
Materials
Pure sucralose (analytic grade standard) was purchased from Sigma-Aldrich (St. Louis, MO). Sucralose was screened for impurities by UHPLC/MS/MS, and none were present at or above the limit of detection (LOD). Methanol (Pharmco, Brookfield, CT), ethyl acetate (Alfa Aesar, Ward Hill, MA), acetonitrile (Pharmco, Brookfield, CT), acetone (Honeywell, Muskegon, MI), water (Pharmco, Brookfield, CT), formic acid (Honeywell, Muskegon, MI), glacial acetic acid (Amresco, Solon, OH), dimethyl sulfoxide, (Alfa Aesar, Ward Hill, MA), and dichloromethane (Honeywell, Muskegon, MI) were purchased from various US based vendors and were either reagent grade or LC/MS grade. All were tested by UHPLC/MS/MS for potentially interfering chlorinated ingredients, and none were detected.
Treatment protocol
The 63-day experiment consisted of three phases: 1) control period, 2) sucralose dosing period, and 3) depuration and recovery. During the 9-day control period (denoted as days −9 to −1), rats were allowed to adjust to their new environment in the individual metabolism cages and special lab diet. After the control period, sucralose was administered to each rat once daily by gavage for 40 consecutive days (denoted as days 0 to 39 where day 0 was first day of dosing with the last dose administered on day 39). Sucralose administration was withdrawn after 40 days for an additional 14 days during the depuration period (denoted as days 40 to 53). Feces and urine samples were collected individually from each animal daily for every 24-hr period throughout the three phases of the 63-day experiment. Feces were deposited in 50ml sterile centrifuge tubes and urine in 50ml sterile centrifuge tubes, and all samples were kept frozen after collection until analysis. Animal body weights were also recorded throughout the study. During the sucralose treatment phase, body weights were determined prior to each dosing. On the 63rd day, 4 animals (2 males and 2 females) were randomly selected and euthanized, and fat samples obtained from the ventral abdomen. The remaining 6 animals were retained by the College of Veterinary Medicine after completion of the depuration period.
Sucralose dosing solutions were prepared by Avazyme on a weekly basis, and the concentration verified by UHPLC/MS/MS. Storage stability of the dosing solutions for at least 1 month was demonstrated prior to the beginning of the study by UHPLC/MS/MS. The target concentration was 25mg sucralose/ml dosing solution for female rats and 36.5mg sucralose/ml dosing solution for male rats. These concentrations were selected on the basis of expected growth rates of male and female rats using normative growth tables. Sucralose in aqueous solution was administered by oral gavage daily at a constant dosing volume of 1ml/test animal, that is, at daily doses of 36.5mg sucralose/animal for 5 male rats and doses of 25mg/animal for 5 female rats. In all test animals body weights increased during the course of the study. This resulted in a steady decrease of the administered sucralose dose on a mg/kg body weight basis for each test animal during the course of the study. The mean daily dosage of sucralose for the 10 animals over the 40 days of sucralose administration was 80.4 mg/kg ().
Table 1. Mean dosages of sucralose administered per rat weight (mg/kg) during the 40 days the rats received the treatment.
Sample preparation, extraction, and UHPLC/MS/MS analysis
Urine was analyzed directly after dilution with water for UHPLC/MS/MS analysis. Feces (0.5 g) were extracted with 1 ml water, methanol, ethyl acetate, acetonitrile, acetone, dimethyl sulfoxide, or dichloromethane, in a Geno/Grinder (two 4mm stainless steel beads, 1,000 RPM, 2 min), centrifuged at 13,000 g for 10 min, 100 μl aliquot placed in an autosampler vial and diluted with 900 μl methanol for UHPLC/MS/MS analysis. Adipose fat tissue was weighed, extracted with a 1 X volume of ethyl acetate in a Geno/Grinder (two 4mm stainless steel beads, 1,000 RPM, 2 min), centrifuged at 13,000 g for 10 min, 100 μl aliquot placed in an autosampler vial and diluted with 900 μl methanol for UHPLC/MS/MS analysis.
Analysis of biological samples and materials was performed under Avazyme’s quality systems and Standard Operating Procedures. The mass detector was a Shimadzu 8050 triple quadrupole mass spectrometer with a Nexera UHPLC front end for the liquid chromatography. A robust analytical method for the detection of chlorine containing potential metabolites and chlorinated parent molecule sucralose was developed in the relevant matrices of urine, feces, and adipose tissue. The presence of biotransformation products of sucralose was assessed using the 3-chlorine isotope pattern in negative ion mode. For sucralose, this isotope pattern consists of three base peaks with masses of m/z 395, 397, and 399 and relative heights of 1:1:0.34 respectively. These three peaks are a consequence of the naturally occurring isotopes of chlorine 35Cl and 37Cl with relative natural abundance of 75.78% and 24.22% respectively. The base peak of 395 represents the sucralose molecule containing three 35Cl atoms with the loss of a proton in negative ion mode (M-H)−. The ions as at 397 and 399 represent the isotopes of sucralose containing one or two 37Cl atoms respectively.
UHPLC analysis was performed with a Shimadzu Nexera system including LC-30AD pumps, a SIL30AC autosampler, and a CTO-20A column oven. A Phenomenex Luna Omega C18 (100 A, 50 mm x 1.2 mm, 1.6 µm) UHPLC column was used. Buffer A was 2% acetonitrile, 98% water, and 0.1% formic acid. Buffer B consisted of 2% water, 98% acetonitrile, and 0.1% formic acid; flow rate was 500 µl/min. The gradient elution from 2% Buffer B (held 0.5 min) reached 35% B at 4 min which produced separation of sucralose from the metabolites. The gradient continued to 100% B at 4.5 min (held 1 min) then dropped to 2% B (held 2 min). The mass detector was a Shimadzu 8050 triple quadrupole mass spectrometer operated in ESI+ APCI (DUIS) negative ion mode with a nebulizing gas flow of 3 L/min, heating gas flow of 10 L/min, and a drying gas flow of 10 L/min. All gas flows were nitrogen. Quantitative analysis was performed with MRM (multiple reaction monitoring) transitions optimized for the [M-H]− and [M+ formate-H]− ions of sucralose. The MRM transitions, voltages and collision energies are listed in .
Table 2. MRM transitions monitored: collision energies and voltages.
Results
Body weights and dosage
All animals gained weight during the three phases of the study. Growth curves for each of the 10 rats during the study are presented in . The initial body weights and subsequent growth curves of the females were more uniform than for males. The effect of sucralose treatment on growth rate was outside the scope of this study and was not calculated.
Figure 2. Growth curves of individual female and male rat weights in grams per day during study period. Sucralose was administered daily for all 10 rats starting at Day 0, with the last dose occurring at Day 39.
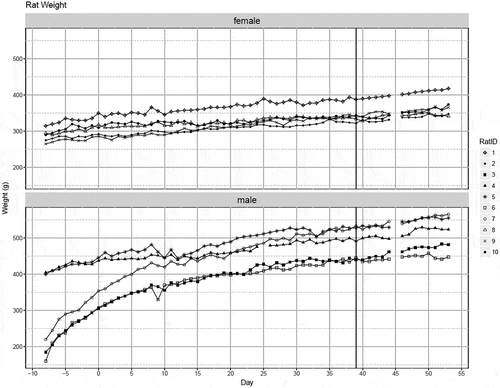
The dosages of sucralose administered per rat weight (mg/kg) during the 40-day sucralose treatment for each animal are presented in . Daily dosages varied because a constant concentration was administered daily for males (36.5mg sucralose daily) and females (25mg daily) throughout the treatment period but body weights increased from the beginning to the end of the sucralose treatment period. Overall, the dosages decreased from Day 0 to Day 39 as animals gained weight. The mean dosage for males over 40 days of sucralose administration was 84.3 mg/kg. The mean dosage for females over 40 days of sucralose administration was 76.5 mg/kg. The mean dosage for males was 101.2 mg/kg at the beginning of the sucralose treatment and 75.3 mg/kg at the end of treatment. The mean dosage for females was 80.7 mg/kg at the beginning of sucralose treatment and 72.6 mg/kg at the end of treatment. On the last day of the treatment period (Day 39), there was no statistical difference in the mean dosage between the males and females.
UHPLC/MS/MS analysis of feces and urine
The results of UHPLC/MS/MS analysis of a fecal sample extracted with ethyl acetate are provided in . The chromatogram in illustrates the presence of two metabolites that were extracted along with sucralose. The retention time between injection of fecal extract and detection of sucralose was 2.4 min. The retention time was 3.2 min for metabolite 1, the less abundant metabolite, and 3.5 min for metabolite 2. These two metabolites were also identified in chromatograms of fecal samples extracted with acetonitrile, dimethyl sulfoxide, methanol, acetone, and dichloromethane although the abundance was significantly less than extracts with ethyl acetate.
Figure 3. a) UHPLC/MS Chromatogram of a fecal sample extracted with ethyl acetate showing the retention times (horizontal axis) and absorbance (vertical axis) for sucralose at 2.4 minutes and two metabolites. Metabolite 1 elutes at 3.2 minutes and metabolite 2 at 3.5 minutes. b) The negative ion mass spectrum of the molecular ion region for sucralose showing mass to charge (m/z) ratio (horizontal axis) and relative abundance (vertical axis). The 3-chlorine isotope pattern is observed for sucralose (m/z 395/397/399) and for the typical electrospray negative ion adducts of chloride (m/z 431/433/435) and formate (m/z 441/443/445). c) The negative ion mass spectrum of the molecular ion region for metabolite 2. The 3-chlorine isotope pattern is observed for metabolite 2 (m/z 437/439/441) and for the typical electrospray negative ion adducts of chloride (m/z 473/475/477) and formate (m/z 483/485/487). d) The negative ion mass spectrum of the molecular ion region for metabolite 1. The 3-chlorine isotope pattern is observed for metabolite 1 (m/z 437/439) and for the typical electrospray negative ion adducts of chloride (m/z 473/475/477) and formate (m/z 483/485/487).
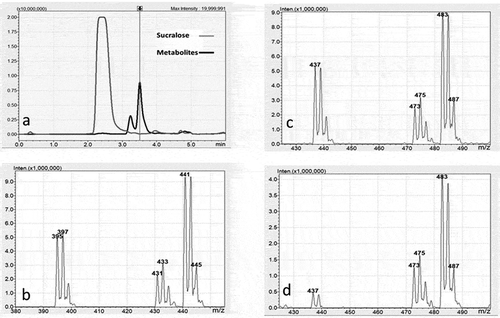
, , and demonstrate the three-chlorine isotope patterns in the mass spectrum formed by passing sucralose and the two metabolites respectively through the ionization chamber of the mass spectrometer. In , the characteristic three-chlorine pattern beginning at 395 represents the sucralose molecule with the loss of a proton in negative ion mode [M-H)]−. The chlorine isotope patterns at 431 and 441 represent adducts formed with chlorine in the fecal material as well as formic acid used in the UHPLC buffer. The relative abundance of the formic acid adduct was greater than for sucralose itself. and show the molecular ion regions for both metabolites display a 3-chlorine isotope pattern at m/z 437/439/441 in the mass spectrum using the negative ion mode. The isotope patterns for the two metabolites were similar but relative abundance differed between the two. The mass change of 42 amu relative to sucralose (437–395 = 42) indicates that the metabolites are two different acetylation metabolites. The chlorine isotope patterns at 473 and 483 represent adducts formed with chlorine in fecal material as well as formic acid employed in the UHPLC buffer.
shows the relative abundance of sucralose and metabolite 2 over the 3 phases of the study. No chlorinated compounds were found in any fecal or urine samples of any animal during the initial 9-day control period. However, sucralose and the metabolites were detected in urine and feces throughout the chemical dosing period. The variability in the abundance of sucralose and metabolites was due to biological variations in the consistency of the feces and volume of urine over the 40-day organochlorine agent administration period. The presence of sucralose metabolite 2, but not parent itself, was detected in urine 11 days after oral chemical delivery on day 50.
Figure 4. Mean sucralose and sucralose metabolite in female and male rat feces and urine during study period. The abscissa represents the day of the study period that the sample was obtained relative to the 1st dose, and the ordinate represents the relative abundance of sucralose or sucralose metabolite in each sample over the samples tested. a) Sucralose/mg in feces extracted with ethyl acetate; b) sucralose metabolite 2/mg in feces extracted with ethyl acetate; c) sucralose/ml in urine; d) sucralose metabolite 2/ml in urine.
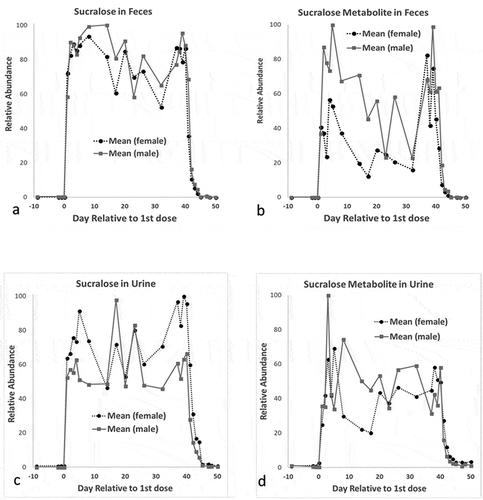
UHPLC/MS/MS analysis of adipose tissue
The samples of adipose tissue obtained 14 days after sucralose withdrawal were extracted with ethyl acetate. Sucralose was found in all 4 adipose samples as presented in . Sucralose was found in adipose tissue on the 14th day of depuration even though it could no longer be detected in the feces and urine. The metabolites were not detected in the adipose tissue.
Table 3. Sucralose Concentrations in Rat Adipose tissue.
Discussion
Analysis of fecal and urine extracts from rats dosed daily with sucralose for 40 days revealed new biotransformation products previously not reported (Roberts et al. Citation2000; Sims et al. Citation2000) or in data submitted to regulatory agencies (U.S. Food and Drug Administration (US FDA) Citation1998; Union Citation2004). An extract of the feces with the polar aprotic solvent ethyl acetate identified two new molecules with a 3-chlorine isotope pattern of a molecular ion in the mass spectrum using negative ion mode at m/z 437/439/441 at retention times significantly longer than the retention time of sucralose. Data indicate that these new biotransformation products are less polar and likely more lipophilic than sucralose. With a mass change of 42 amu, this would be consistent with two different acetylation metabolites of sucralose, where hydrogen on a hydroxyl group of sucralose was replaced with an acetyl group (CH3CO). Most likely, two different hydroxyl groups were acetylated to form two different isomeric sucralose metabolites. These acetylated compounds were also extracted with a non-polar solvent (dichloromethane) and three additional polar aprotic solvents (acetone, acetonitrile, and dimethyl sulfoxide). Extraction with dichloromethane (CH2Cl2) and acetonitrile (CH3CN) was a confirmation and verification that these are biotransformation products and that they are not artifacts formed during the UHPLC/MS/MS analysis because neither solvent contains reagents or molecules associated with acetylation. Further, acetylated sucralose was not present in the highly purified sucralose that was administered to the rats. These putative acetylated metabolites were also found in the urine without the need for extraction.
The historical rat and human toxicity studies (Roberts et al. Citation2000; Sims et al. Citation2000) failed to detect the presence of these acetylated metabolites of sucralose in urine or feces for several possible reasons. In the early rat and human experiments, the polar protic solvent methanol was used for the fecal extractions. Upon extraction into methanol, the acetylated metabolites may have undergone transesterification to produce sucralose and methyl acetate. Although the mobile phases employed for separation in the early TLC studies did contain several polar aprotic solvents including ethyl acetate, the sucralose metabolites may not have been detected if the methanolic fecal extracts applied to the TLC plates did not contain the metabolites as a result of transesterification. The acetylated metabolites were also not described in the historical analyses of urine of either humans or rats (Roberts et al. Citation2000; Sims et al. Citation2000). In human urine studies, the residue applied to the TLC plates was obtained from a methanol eluate obtained after urine was passed through an XAD-2 column. Similar to fecal studies, the metabolites may have undergone transesterification in the presence of methanol. Unlike the human studies, rat urine samples were not extracted with methanol. The TLC trace from rat urine samples reported by Sims et al. (Citation2000) exhibited a large broad “peak” attributed to sucralose and several smaller peaks that represent compounds more polar than sucralose and considered glucuronide adducts of intact sucralose. However, the large “peak” in the TLC trace was truncated in the Figure by Sims et al. (Citation2000) so it is not known if there were multiple peaks in the broad TLC trace that may have included the acetylated metabolites. Other possible explanations for absence of acetylated metabolites in early toxicity studies include low solubility of the metabolites in methanol as well as sensitivity and resolution limitations of the linear analyzers used in the historical studies, especially when compared to UHPLC/MS/MS used in the present study.
The mechanism by which sucralose is acetylated is not known. Sucralose may be acetylated by the host (rat) by transfer of the acetyl group of acetyl coenzyme A (acetyl CoA) to a hydroxyl group. Alternatively, this organochlorine agent may be acetylated by commensal bacteria in the GIT. Intestinal bacteria are known to acetylate xenobiotic compounds (Deloménie et al. Citation2001; Dull, Salata, and Goldman Citation1987), and studies of sewage and different soils noted that sucralose is metabolized by microorganisms including bacteria (Labare and Alexander Citation1994). Further, a newly isolated bacteria from the phylum Firmicutes, Bacillus amyloliquefaciens WZS01, was recently shown to be capable of both acetylating as well as deacetylating sucralose (Sun et al. Citation2017). Sucralose was previously found to alter bacterial composition in the rat intestine as well as interact with components of first pass metabolism (Abou-Donia et al. Citation2008).
Another major finding of this study was that sucralose persisted in adipose tissue two weeks after cessation of use even though it had mostly cleared from urine and feces by 5 days. The retention of sucralose in adipose tissue during or after cessation of repeated chemical administration was not examined previously. Roberts et al. (Citation2000) claimed that sucralose had a “lack of bioaccumulative potential” primarily due to its “hydrophilic nature”. However, this reasoning was incorrect because sucralose is an amphiphilic compound (Schiffman and Rother Citation2013) with both lipid and water solubility and consequently partitions into adipose tissue as reported here. The fact that sucralose is an amphiphilic compound was confirmed in this study because it was extracted with both polar protic and aprotic solvents as well as a non-polar solvent. The finding that the sucralose metabolites, which are more lipophilic than sucralose, were not found in adipose tissue after a two-week withdrawal of sucralose administration may be due in part to their uptake in cell membranes of the intestines and other tissues prior to reaching abdominal fat. Cell membranes act as a sink or repository for many chemicals including organochlorine compounds (Vauquelin Citation2016).
The finding that sucralose remains in the body for an extended period of time after termination of administration is consistent with exposure to other organochlorine compounds that exhibit long-term storage in adipose tissue (Jackson et al. Citation2017). Adipose tissue is involved in a large number of metabolic and regulatory processes including endocrine functions (Greenberg and Obin Citation2006; Jansen et al. Citation2017). Organochlorine compounds were found to modulate adipose tissue metabolism including increasing preadipocyte proliferation (Chapados et al. Citation2012). The chemical safety and health impacts of sucralose retention in fat tissue have yet to be evaluated in controlled human studies. Further studies are needed to determine if there are long-lasting effects of sucralose retention in adipose tissue and to low level, long-term release.
The mean sucralose dosage in this study was 80.4 mg/kg/day which is 16-fold greater than the acceptable daily intake (ADI) of 5 mg/kg/day set by the U.S. Food and Drug Administration (US FDA) (Citation1998) and 5.3 times higher than the ADI of 15 mg/kg/day approved in the European Union (Citation2004). The establishment of ADI for sucralose by regulatory agencies was based on the metabolic profile in the rat which was considered the appropriate model for humans. A 100-fold safety factor was applied to historical sucralose toxicity data to establish the ADI. If one were to apply a 100-fold safety factor to the biological effects reported in the present study, that is, metabolism and bioaccumulation at 80.4 mg/kg/day, this would lower the ADI for sucralose to less than 1 mg/kg/day.
Conclusions
In conclusion, data demonstrated that sucralose is metabolized in rats to compounds that are less polar and thus more lipophilic than the parent compound. Further, sucralose was retained in adipose tissue two weeks after cessation of intake. The findings of this study do not support the claims previously submitted to regulatory agencies that sucralose is a stable compound that (1) is not metabolized in vivo, (2) excreted unchanged in the feces, and (3) clears the body within a few days. These new observations of metabolism of sucralose in the GIT and accumulation of the compound in adipose tissue were not part of the original regulatory decision process for this organochlorine artificial sweetener. Data indicate that it may now be time to revisit the regulatory status of sucralose.
Acknowledgments
This research was supported by the Engineering Foundation at North Carolina State University.
References
- Abou-Donia, M. B., E. M. El-Masry, A. A. Abdel-Rahman, R. E. McLendon, and S. S. Schiffman. 2008. Splenda alters gut microflora and increases intestinal P-glycoprotein and cytochrome P-450 in male rats. Journal of Toxicology and Environmental Health, Part A 71:1415–29. doi:10.1080/15287390802328630.
- Botella, B., J. Crespo, A. Rivas, I. Cerrillo, M. F. Olea-Serrano, and N. Olea. 2004. Exposure of women to organochlorine pesticides in Southern Spain. Environmental Research 96:34–40. doi:10.1016/j.envres.2003.10.001.
- Brooks, G. T. 2018. Chlorinated insecticides. In Technology and Application, vol 1, 166. CRC Press. Taylor and Francis Group.
- Chapados, N. A., C. Casimiro, M. A. Robidoux, F. Haman, M. Batal, J. M. Blais, and P. Imbeault. 2012. Increased proliferative effect of organochlorine compounds on human preadipocytes. Molecular and Cellular Biochemistry 365:275–78. doi:10.1007/s11010-012-1268-0.
- Çok, I., and M. H. Şatiroğlu. 2004. Polychlorinated biphenyl levels in adipose tissue of primiparous women in Turkey. Environment International 30:7–10. doi:10.1016/S0160-4120(03)00140-5.
- Covaci, A., J. De Boer, J. J. Ryan, S. Voorspoels, and P. Schepens. 2002. Distribution of organobrominated and organochlorinated contaminants in Belgian human adipose tissue. Environmental Research 88:210–18. doi:10.1006/enrs.2002.4334.
- Deloménie, C., S. Fouix, S. Longuemaux, N. Brahimi, C. Bizet, B. Picard, E. Denamur, and J. M. Dupret. 2001. Identification and functional characterization of arylamine N-acetyltransferases in eubacteria: Evidence for highly selective acetylation of 5-aminosalicylic acid. Journal of Bacteriology 183:3417–27. doi:10.1128/JB.183.11.3417-3427.2001.
- Dull, B. J., K. Salata, and P. Goldman. 1987. Role of the intestinal flora in the acetylation of sulfasalazine metabolites. Biochemical Pharmacology 36:3772–74.
- European Union 2004. Directive 2003/115/EC of the European Parliament and of the Council of 22 December 2003 amending Directive 94/35/EC on sweeteners for use in foodstuffs. Office Journal European Union 47 (L24):65–71.
- Greenberg, A. S., and M. S. Obin. 2006. Obesity and the role of adipose tissue in inflammation and metabolism. American Journal of Clinical Nutrition 83( Suppl):461S–465S. doi:10.1093/ajcn/83.2.461S.
- Jackson, E., R. Shoemaker, N. Larian, and L. Cassis. 2017. Adipose tissue as a site of toxin accumulation. Comprehensive Physiology 7:1085–135. doi:10.1002/cphy.c160038.
- Jansen, A., J. L. Lyche, A. Polder, J. Aaseth, and M. A. Skaug. 2017. Increased blood levels of persistent organic pollutants (POP) in obese individuals after weight loss—A review. Journal of Toxicology and Environmental Health, Part B 20:22–37. doi:10.1080/10937404.2016.1246391.
- Labare, M. P., and M. Alexander. 1994. Microbial cometabolism of sucralose, a chlorinated disaccharide, in environmental samples. Applied Microbiology and Biotechnology 42:173–78.
- Lerner, I. 2009. Artificial sweeteners market to change. ICIS Chemical Business 275:28−29.
- Patel, A., J. P. Rai, D. K. Jain, and J. I. Banweer. 2012. Formulation, development and evaluation of cefaclor extended release matrix tablet. International Journal of Pharmacy and Pharmaceutical Sciences 4:355–57.
- Pharmacopeia, U. S. 2018. http://pharmacopeia.cn/
- Roberts, A., A. G. Renwick, J. Sims, and D. J. Snodin. 2000. Sucralose metabolism and pharmacokinetics in man. Food and Chemical Toxicology 38 (Suppl.2):S31–S41.
- Schiffman, S. S., and M. B. Abou-Donia. 2012. Sucralose revisited: Rebuttal of two papers about Splenda safety. Regulatory Toxicology and Pharmacology : RTP 63:505–08. doi:10.1016/j.yrtph.2012.05.002.
- Schiffman, S. S., and K. I. Rother. 2013. Sucralose, a synthetic organochlorine sweetener: Overview of biological issues. Journal of Toxicology and Environmental Health, Part B 16:399–451. doi:10.1080/10937404.2013.842523.
- Schiffman, S. S., E. A. Sattely-Miller, and I. E. Bishay. 2008. Sensory properties of neotame: Comparison with other sweeteners. Sweetness and sweeteners: Biology, chemistry and psychophysics. ACS Symposium Series 979. eds. D. K Weerasinghe and G. E. Dubois, 511–529. Oxford University Press.
- Sims, J., A. Roberts, J. W. Daniel, and A. G. Renwick. 2000. The metabolic fate of sucralose in rats. Food and Chemical Toxicology 38 (Suppl.2):S115–S121.
- Skaare, J. U., J. M. Tuveng, and H. A. Sande. 1988. Organochlorine pesticides and polychlorinated biphenyls in maternal adipose tissue, blood, milk, and cord blood from mothers and their infants living in Norway. Archives of Environmental Contamination and Toxicology 17:55–63.
- Sun, J., L. Chen, B. Lou, Y. Bai, X. Yu, M. Zhao, and Z. Wang. 2017. Acetylation and deacetylation for sucralose preparation by a newly isolated Bacillus amyloliquefaciens WZS01. Journal of Bioscience and Bioengineering 123:576–80. doi:10.1016/j.jbiosc.2016.12.013.
- Sylvetsky, A. C., A. L. Gardner, V. Bauman, J. E. Blau, H. M. Garraffo, P. J. Walter, and K. I. Rother. 2015. Nonnutritive sweeteners in breast milk. Journal of Toxicology and Environmental Health, Part A 78:1029–32. doi:10.1080/15287394.2015.1053646.
- U.S. Food and Drug Administration (US FDA). 1998. Food additives permitted for direct addition to food for human consumption; sucralose. 21CFR Part 172 [Docket No. 87F-0086]. Federal Reg. 63 (64):16417–33. http://www.gpo.gov/fdsys/pkg/FR-1998-04-03/pdf/98-8750.pdf accessed February 12, 2018
- Vauquelin, G. 2016. Cell membranes and how long drugs may exert beneficial pharmacological activity in vivo. British Journal Clinical Pharmacology 82:673–82. doi:10.1111/bcp.12996.