ABSTRACT
Plastics- and rubber-derived chemicals are given increasing focus due to their migration into the environment and potential for causing detrimental effects. The current study demonstrates the use of a novel biomonitoring platform using caged fertilized eggs of lumpfish (Cyclopterus lumpus) in combination with gas chromatography tandem mass spectrometry analysis of a selection of target chemicals extracted from the lumpfish eggs after deployment. A monitoring campaign in the Trondheim harbor and off the coast of Trøndelag in Norway was executed using the described system. Here we found accumulation of UV stabilizers (benzophenone and benzothiazoles), plasticizers (n-butylbenzenesulfonamide), reagents, and polymer synthesis precursors (bisphenol A, acetophenone, phthalide, and phthalimide) in deployed eggs. Several of the compounds were detected in concentrations above previously quantified legacy contaminants in the same study areas.
Introduction
While plastic litter and microplastic accumulation effects on the environment have been a major research focus for several years, more recently focus has shifted toward plastics in the environment as a vector for associated chemicals. All plastics and rubber contain a variety of both intentionally (additives) and non-intentionally added substances, most of which are not chemically bound to the polymer matrix and thus available for leaching into the surrounding environment (Hahladakis et al. Citation2018; Hermabessiere et al. Citation2017).
With the emerging acknowledgment of problems associated with plastic litter pollution, tire wear particles (TWP) and granulates of car tire rubber (CTR) originating from re-purposing of end-of-life tires, for instance as playground and sports ground infill, has been recognized as one of the major constituents of microplastic particles reaching the marine environment (Baensch-Baltruschat et al. Citation2020; Hann et al. Citation2018; Sundt et al. Citation2020; Wagner et al. Citation2018). A recent report estimate that in Norway alone, microplastic discharge to the environment from terrestrial sources is 19,000 tons annually, with TWPs and rubber granulates from artificial soccer pitches contributing >70% of these estimated emissions (Sundt et al. Citation2020).
By now, a variety of CTR-associated chemicals have been found in most environmental compartments (Cao et al. Citation2022; Johannessen et al. Citation2022). The toxicity of chemicals leaching into seawater from CTR has been demonstrated in laboratory studies (Capolupo et al. Citation2020, Citation2021; Halsband et al. Citation2020). Recent efforts have been made to characterize the chemical content of such leachates (Capolupo et al. Citation2020; Halsband et al. Citation2020; Llompart et al. Citation2013), and to link specific rubber chemicals to accumulation and observed detrimental effects of urban run-off (Tian et al. Citation2021).
Another key source of microplastic in the Norwegian environment is synthetic textiles (Sundt et al. Citation2020), and recent studies have demonstrated the presence and potential leaching of chemicals from textile fibers released into aquatic environments (Sait et al. Citation2021; Sørensen et al. Citation2021).
While plastic and rubber associated chemicals are frequently detected in several environmental matrices, including wastewater, rivers, air, and precipitation, little knowledge on the uptake and presence of such compounds (beyond those frequently studied) in biota exist.
A recent study by our group demonstrated a novel approach using fertilized eggs of caged lumpfish (Cyclopterus lumpus) for biomonitoring of contamination in coastal areas (Hansen et al. Citation2022) While the study focused on petrogenic contaminants (polycyclic aromatic hydrocarbons, PAHs, and phenolic compounds as potential petrogenic metabolites), it also provided evidence for other sources of pollution. Bisphenol A (BPA) was detected above the limit of detection (LOD) in almost all samples close to the urban center of Trondheim, but not in the more remote sampling location. While no acute effects of contamination were observed in the exposed embryos, slightly higher indices of embryonic deformations were observed in two of the locations with the highest accumulation of PAHs and phenolics occurred. Here, we aim to expand on the previous work to document the accumulation of a suite of rubber and plastic associated chemicals in the same embryos, further validating the applicability of the biomonitoring methodology and providing evidence of these chemicals being present in the area.
Materials and methods
Husbandry and field deployment of lumpfish egg and milt acquisition and egg fertilisation
Unfertilized lumpfish eggs from wild-caught females were obtained from two different sources (Namdalen Rensefisk AS and Skjerneset AS, Norway). After strip-spawning, eggs were transported on ice, arriving at SINTEF Sealab in Trondheim within 4 h. Cryopreserved milt from one single male was obtained from Cryogenetics (Hamar, Norway). Eggs were fertilized in vitro as previously described (Hansen et al. Citation2022). Briefly, cryopreserved milt (1 mL) was gently mixed with eggs (50 mL) and added to filtered (1 µm, 50 mL) seawater. After 3 min, circular molds (2 cm diameter) were used to distribute fertilized eggs in circular egg monolayers (CEML), with approximately 80 eggs in each. CEMLs were kept submerged until the eggs hardened, after which they were placed in custom-built polycarbonate holding frames (16 CEML in each frame, seven frames total) (Hansen et al. Citation2022). CEMLs were sheltered from direct sunlight and kept under water during frame and deployment assembly and underwater throughout the whole in situ exposure period. The deployment rig is described in detail elsewhere (Hansen et al. Citation2022).
Rigs were deployed at six locations (Fig S1, Table S1), three locations in Trondheimsfjorden (November 2019, 17 days deployment) and three off the coast of Trøndelag (November 2019, 19 days deployment). Eggs from Namdalen Rensefisk AS were used for the Trondheimsfjorden deployment, and eggs from Skjerneset AS were used for coastal deployment. Conductivity, temperature and depth (CTD, Castaway®-CTD, SonTek) were monitored and temperature logged (HOBO Pendant® Temperature 64K Data Logger, Onset Computer Corporation) at 5 min intervals throughout deployment. Upon recovery of the rigs (after 17 days in Trondheimsfjorden and 19 days at the coastal deployment), they were kept in ambient seawater and transported to the onshore laboratory facility. Immediately upon arrival in the laboratory, 4 CEML replicates from each location were rinsed with filtered (1 µm) seawater and immediately frozen (−20°C) until extraction and chemical analyses as described below.
Extraction and contaminant target screening
Extractions of eggs were performed as described in (Hansen et al. Citation2022). Briefly, the samples were accurately weighed (sample mass range 0.35–0.66 g) and transferred to glass vials. After addition of n-hexane/DCM (1:1 v/v, 4 mL) and surrogate standards (25.08 ng naphthalene-d8, 5.00 ng phenanthrene-d10, 4.86 ng chrysene-d12, 5.08 ng perylene-d12, 2533.4 ng phenol-d6, 104.2 ng p-cresol-d8, 137.4 ng 4-n-propylphenol-d12), the samples were homogenized using a disperser (IKA 10 basic ULTRA-TURRAX®; IKA-Werke, Staufen, Germany), sodium sulfate was added followed by a brief vortex and centrifugation (720 g, 2 min). The supernatant was collected, and the extraction repeated two additional times. The combined extracts were volume reduced (40°C under a gentle flow of N2) to approximately 1 mL, and further subject to clean-up by gel permeation chromatography (GPC) where 0.5 mL was injected and on an Agilent 1200 LC system with a 1260 series fraction collector. Separation was achieved using an Envirogel column (19 × 300 mm, 15 μm; Waters Milford. MA, USA) and using DCM as a mobile phase (5 mL/min). Chromatograms were monitored at 210, 254, and 280 nm UV. Analyte fractions were collected from 10.1 to 14.5 min with pre-added n-hexane in the collection vials as a keeper. The sample volume was finally adjusted to 0.5 mL by solvent evaporation, and a recovery internal standard (100 ng fluorene-d10) was added prior to analysis.
Extracts were analyzed by an Agilent 7890 gas chromatograph coupled with an Agilent 7010B triple quadrupole mass spectrometer fitted with an EI source and collision cell was used (Agilent Technologies, Santa Clara, CA, USA). Two Agilent J&W HP-5 MS UI GC-columns (30 m × 0.25 mm × 0.25 µm) were coupled in series through a purged ultimate union (PUU). The carrier gas was high-purity helium at constant flow (1.2 mL/min). Samples (1 µL) were injected at 250°C, the oven temperature was kept at 40°C for 1.5 min, then ramped to 110°C by 40°C/min, to 310°C by 5°C/min and held for 20 min. The temperature was finally held at 330°C for 5 min, while the first column was backflushed. The transfer line temperature was 300°C, the ion source temperature was 230°C and the quadrupole temperatures were 150°C. The EI source was operated at 70 eV. N2 was used as collision gas at a flow of 1.5 mL/min and helium was used as a quench gas at a flow of 2.25 mL/min. Target analytes were identified by two unique MRM transitions and quantified by the area of the most intense peak (Table S2). For quantification, the peak area was normalized to that of chrysene-d12 and a quadratic regression (0.1–1000 ng/mL) curve was used. Calibration standards were re-run for each 12 sample injections to monitor the system performance, and a variation of no more than 25% was accepted. Method limits of detection (LOD, based on laboratory blanks) is provided in Table S2.
Statistics
Data treatment and statistical analyses were conducted with GraphPad Prism V9.5.1 (GraphPad Software, Inc., CA, USA). Comparisons of contaminant body burdens between stations were performed with one-way ANOVA, followed by Tukey’s multiple comparisons test or Kruskal–Wallis test, followed by Dunn’s multiple comparison test for non-normal distributed data sets according to D′Agostino & Pearson omnibus normality test. Significance level was set to p < 0.05 unless otherwise stated.
Results and discussion
In addition to being proven applicable for target quantification of PAHs and alkyl phenols in fish eggs (Oppegård, Hansen, and Sørensen Citation2020; Sørensen et al. Citation2019), the applied extraction-purification protocol was recently validated for use in non-target and suspect screening of a range of legacy and chemicals of emerging concern in small biotic samples (Sørensen et al. Citation2023).
A selection of plastic and rubber relevant chemicals () was identified and quantified in deployed lumpfish eggs, at several of the deployment locations (). The highest concentrations of most compounds (BPA, acetophenone, benzothiazole, 2-(methylthio)benzothiazole, phthalide, and phthalimide) were detected at the two locations (Brattørkaia; BK, Nyhavna; NH) located closest to the city center of Trondheim (Fig. S1), particularly Brattørkaia (BK) where concentrations were significantly higher (p < 0.05) than all other stations for acetophenone, benzothiazole, benzophenone, 2-(methylthio)benzothiazole, phthalide, and phthalimide (). Only one of the compounds, the plastic softener n-butylbenzenesulfonamide, was detected in significantly higher concentrations at a coastal deployment site (ACE3) compared to the studied harbor areas (p < 0.05).
Figure 1. Concentrations of rubber and plastic associated chemicals accumulated in lumpfish eggs after exposure in the field at Brattørkaia (BK), Nyhavna (NH), Trondheimsfjorden (MH5: 5 m depth, MH15: 15 m depth), and in a transect from an aquaculture facility at the coast (ACE1 being closest). Significant differences (p < 0.05) between groups are denoted different letters (one-way ANOVA followed by Tukey’s multiple comparisons test).
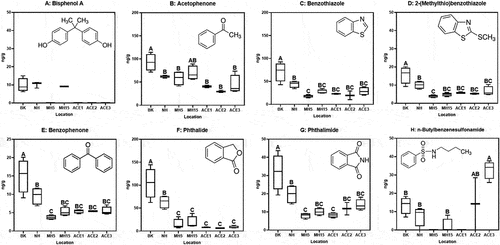
Table 1. An overview of quantified analytes and their properties. * Octanol-water partition coefficients (KOW) and theoretical bioconcentration factors (BCFs) based on regression are extracted from EpiSuite (U.S. EPA Citation2012). A(Corrales et al. Citation2015), B(Chai et al. Citation2018; Kühn et al. Citation2020), C(Liao, Kim, and Kannan Citation2018), D(Guo et al. Citation2020), E(Salazkin and Shaposhnikova Citation2013), F(Guo et al. Citation2009), G(Rider et al. Citation2020).
Of the selected compounds, BPA was detected in lowest concentrations in the eggs and only in harbor stations and one sample in the Trondheim fjord (no significant differences between station, p > 0.05, ). With the highest theoretical bioconcentration factor (BCF), this may further imply that aqueous concentrations of the other compounds are orders of magnitude higher than of BPA. Although it is not known to what extent lumpfish embryos are able to metabolize different xenobiotics, they can induce cyp1a expression early on in embryogenesis (84-h post fertilization) (Hansen et al., Citation2023); thus, metabolization of target compounds during deployment would potentially affect the measured concentrations. Some of the target compounds in our study, including benzothiazoles and BPA, are capable of inducing biotransformation enzymes like cyp1a (He, Zhao, and Denison Citation2011) and UDP-glucuronosyltransferases (Huang et al. Citation2018), respectively.
Quantified levels of BPA (by wet weight) accumulated in the lumpfish eggs over the length of deployment herein were at the lower end of the range of those detected in lipid-rich Arctic zooplankton species in a recent study by our group (Sørensen et al. Citation2023). BPA has also been detected in both Arctic seawater and organisms and hypothesized sources include long-range atmospheric transport as well as transport by plastic debris (Ademollo et al. Citation2018, Citation2021). BPA remain a high-production volume chemical with multiple applications, pinpointing the source of pollution in the test sites is currently not possible.
All compounds shown to accumulate in lumpfish eggs in the current study may have several sources. However, all the compounds are commonly found in various types of rubber or plastic products that may be used domestically or in outdoor applications. The sampling station BK is the main harbor for small maritime traffic in Trondheim, including passenger vessels. NH has been an important industrial area since the late 1800s and today the NH harbor area consists of a shipyard and a local industrial hub. Two municipal wastewater treatment plants exist in Trondheim and may contribute to observed pollution in both near-city locations. Further, urban run-off and transport from the river Nidelva, which lets out close to the BK station, may also explain the presence of these chemicals in this area (Tian et al. Citation2021). The ACE stations were in the vicinity of an aquaculture production facility for farmed salmon. Local leaching of n-butylbenzenesulfonamide from plastic components of the facility may explain its elevated presence at these locations, but this needs further investigation.
UV stabilizers benzothiazole, 2-(methylthio)benzothiazole, phthalide, and phthalimide have previously been detected in seawater leachates of CTR (Capolupo et al. Citation2020; Halsband et al. Citation2020). Benzophenone, benzothiazole, phthalide, phthalimide, n-butylbenzenesulfonamide, have also been found in textile microfibres and their corresponding aqueous leachates (Sait et al. Citation2021; Sørensen et al. Citation2021). Benzothiazole and 2-(methylthio)benzothiazole have also previously been detected in a variety of environmental matrices including wastewaters and receiving waters (Liao, Kim, and Kannan Citation2018), and have recently been quantified in megacity air samples from across the world (Johannessen et al. Citation2022). The plasticizer n-butylbenzenesulfonamide is commonly detected in environmental samples (Di Carro et al. Citation2018) and is currently under review due to its persistence and toxicity, both to aquatic organisms and humans (Blum et al. Citation2018; Rider et al. Citation2020). Acetophenone has previously been detected in polystyrene plastics and further observed to transfer from plastic particulates to lipid-rich biological fluids (Kühn et al. Citation2020).
When transported back to the laboratory for incubation in clean seawater until hatch, a battery of ecotoxicological endpoints were assessed both in the embryos and in the larvae after hatching. These have all been reported previously (Hansen et al. Citation2022). Briefly, a higher proportion of the embryos incubated in BK displayed morphological deformations and delayed development compared to the other stations. Toxic effects of benzothiazoles on fish, including effects of nutrient accumulation, retarded larvae growth, and histological impairment of gills, have been reported in the literature previously (Liao, Kim, and Kannan Citation2018). The eggs incubated in the harbor areas accumulated a mixture of different pollutants, including PAHs up to comparable levels with benzothiazoles (Figure S2). Thus, the observed embryotoxicity cannot be assigned exclusively to a specific group of pollutants, e.g., the plastic and rubber associated chemicals reported herein. Given the low BCFs reported in the literature for benzothiazoles (<400) (Liao, Kim, and Kannan Citation2018) compared to many of the other more lipophilic pollutants (e.g., PAHs and PCBs) found to accumulate in lumpfish eggs, it is, however, reasonable to assume the exposure concentrations for the plastic and rubber-associated chemicals were higher. After incubation in the laboratory until hatch, no gross morphological impacts on hatched larvae were observed, suggesting that the surviving larvae, at least to some extent, were able to withstand toxicity from the accumulated pollutants (Hansen et al. Citation2022).
Conclusions
The current study demonstrates the presence of a small suite of rubber and plastic chemicals in the marine waters outside Trondheim in Norway. The city harbor locations demonstrated significantly higher levels of most of the chemicals, apart from the softener n-butylbenzenesulfonamide, which was primarily detected at two coastal locations. This study supports the applicability of the lumpfish egg biomonitoring tool, which was previously demonstrated for petroleum hydrocarbons, also to assess environmental contamination of more polar and mobile chemicals. Further, the study shows the potential for these compounds to accumulate in marine biota, several of which are known to be toxic. Future investigations should aim at elucidating the toxicity of these chemicals to native species in these habitats.
Acknowledgments
The authors are grateful to Marianne Molid for assistance with chemical analysis.
Disclosure statement
No potential conflict of interest was reported by the authors.
Data availability statement
The authors confirm that the data supporting the findings of this study are available within the article and its supplementary materials.
Additional information
Funding
References
- Ademollo, N., L. Patrolecco, J. Rauseo, J. Nielsen, and S. Corsolini. 2018. Bioaccumulation of nonylphenols and bisphenol a in the Greenland shark somniosus microcephalus from the Greenland seawaters. Microchem. J Pharmacol. Res. Anal Approaches 136:106–12. doi:10.1016/j.microc.2016.11.009.
- Ademollo, N., F. Spataro, J. Rauseo, T. Pescatore, N. Fattorini, S. Valsecchi, S. Polesello, and L. Patrolecco. 2021. Occurrence, distribution and pollution pattern of legacy and emerging organic pollutants in surface water of the Kongsfjorden (Svalbard, Norway): Environmental contamination, seasonal trend and climate change. Mar. Pollut. Bull. 163:111900. doi:10.1016/j.marpolbul.2020.111900.
- Baensch-Baltruschat, B., B. Kocher, F. Stock, and G. Reifferscheid. 2020. Tyre and road wear particles (TRWP) - a review of generation, properties, emissions, human health risk, ecotoxicity, and fate in the environment. Sci. Total Environ. 733:137823. doi:10.1016/j.scitotenv.2020.137823.
- Blum, K. M., P. L. Andersson, L. Ahrens, K. Wiberg, and P. Haglund. 2018. Persistence, mobility and bioavailability of emerging organic contaminants discharged from sewage treatment plants. Sci. Total Environ. 612:1532–42. doi:10.1016/j.scitotenv.2017.09.006.
- Cao, G., W. Wang, J. Zhang, P. Wu, X. Zhao, Z. Yang, D. Hu, and Z. Cai. 2022. New evidence of rubber-derived quinones in water, air, and soil. Environ Sci Technol 56 (7):4142–50. doi:10.1021/acs.est.1c07376.
- Capolupo, M., K. Gunaalan, A. M. Booth, L. Sørensen, P. Valbonesi, and E. Fabbri. 2021. The sub-lethal impact of plastic and tire rubber leachates on the Mediterranean mussel Mytilus galloprovincialis. Environ. Pollut. 283:117081. doi:10.1016/j.envpol.2021.117081.
- Capolupo, M., L. Sørensen, K. D. R. Jayasena, A. M. Booth, and E. Fabbri. 2020. Chemical composition and ecotoxicity of plastic and car tire rubber leachates to aquatic organisms. Water Res. 169:115270. doi:10.1016/j.watres.2019.115270.
- Chai, K., K. Lu, Z. Xu, Z. Tong, and H. Ji. 2018. Rapid and selective recovery of acetophenone from petrochemical effluents by crosslinked starch polymer. J. Hazard. Mater. 348:20–28. doi:10.1016/j.jhazmat.2018.01.034.
- Corrales, J., L. A. Kristofco, W. B. Steele, B. S. Yates, C. S. Breed, E. S. Williams, and B. W. Brooks. 2015. Global assessment of bisphenol a in the environment: Review and analysis of its occurrence and bioaccumulation. Dose-Response 13 (3):1559325815598308. doi:https://doi.org/10.1177/1559325815598308.
- Di Carro, M., E. Magi, F. Massa, M. Castellano, C. Mirasole, S. Tanwar, E. Olivari, and P. Povero. 2018. Untargeted approach for the evaluation of anthropic impact on the sheltered marine area of Portofino (Italy). Mar. Pollut. Bull. 131:87–94. doi:10.1016/j.marpolbul.2018.03.059.
- Guo, X., F. S. Kim, S. A. Jenekhe, and M. D. Watson. 2009. Phthalimide-based polymers for high performance organic thin-film transistors. J. Am. Chem. Soc. 131 (21):7206–07. doi:10.1021/ja810050y.
- Guo, Q., D. Wei, H. Zhao, and Y. Du. 2020. Predicted no-effect concentrations determination and ecological risk assessment for benzophenone-type UV filters in aquatic environment. Environ. Pollut. 256:113460. doi:10.1016/j.envpol.2019.113460.
- Hahladakis, J. N., C. A. Velis, R. Weber, E. Iacovidou, and P. Purnell. 2018. An overview of chemical additives present in plastics: Migration, release, fate and environmental impact during their use, disposal and recycling. J. Hazard. Mater. 344:179–99. doi:10.1016/j.jhazmat.2017.10.014.
- Halsband, C., L. Sørensen, A. M. Booth, and D. Herzke. 2020. Car tire crumb rubber: Does leaching produce a toxic chemical cocktail in coastal marine systems? Front. Environ. Sci. 8. doi:10.3389/fenvs.2020.00125.
- Hann, S., C. Darrah, C. Sherrington, K. Blacklaws, I. Horton, and A. Thompson, 2018. Reducing household contributions to marine plastic pollution, report for friends of the earth. Eunomia.
- Hansen, B., A. Arukwe, H. Knutsen, K. Skarpnord, J. Farkas, L. Veylit, R. Nepstad, E. Khan, T. Nordtug, and L. Sørensen. 2023. Effects of exposure timing on cyp1a expression, PAH elimination and lipid utilization in lumpfish embryos exposed to produced water. Environ. Sci. Technol. In press.
- Hansen, B. H., T. Nordtug, I. B. Øverjordet, L. Sørensen, B. Kvæstad, E. J. Davies, S. Meier, T. Gomes, S. Brooks, and J. Farkas. 2022. Monitoring ocean water quality by deployment of lumpfish (Cyclopterus lumpus) eggs: In situ bioaccumulation and toxicity in embryos. Ecotoxicology and Environmental Safety 245:114074. doi:10.1016/j.ecoenv.2022.114074.
- Hermabessiere, L., A. Dehaut, I. Paul-Pont, C. Lacroix, R. Jezequel, P. Soudant, and G. Duflos. 2017. Occurrence and effects of plastic additives on marine environments and organisms: A review. Chemosphere 182:781–93. doi:10.1016/j.chemosphere.2017.05.096.
- He, G., B. Zhao, and M. S. Denison. 2011. Identification of benzothiazole derivatives and polycyclic aromatic hydrocarbons as aryl hydrocarbon receptor agonists present in tire extracts. Environ. Toxicol. Chem. 30 (8):1915–25. doi:https://doi.org/10.1002/etc.581.
- Huang, Q., Y. Liu, Y. Chen, C. Fang, Y. Chi, H. Zhu, Y. Lin, G. Ye, and S. Dong. 2018. New insights into the metabolism and toxicity of bisphenol a on marine fish under long-term exposure. Environ. Pollut. 242:914–21. doi:10.1016/j.envpol.2018.07.048.
- Johannessen, C., A. Saini, X. Zhang, and T. Harner. 2022. Air monitoring of tire-derived chemicals in global megacities using passive samplers. Environ. Pollut. 314:120206. doi:10.1016/j.envpol.2022.120206.
- Kühn, S., A. M. Booth, L. Sørensen, A. van Oyen, and J. A. van Franeker. 2020. Transfer of additive chemicals from marine plastic debris to the stomach oil of Northern Fulmars. Front. Environ. Sci. 8. doi:10.3389/fenvs.2020.00138.
- Liao, C., U. -J. Kim, and K. Kannan. 2018. A review of environmental occurrence, fate, exposure, and toxicity of benzothiazoles. Environ Sci Technol 52 (9):5007–26. doi:10.1021/acs.est.7b05493.
- Llompart, M., L. Sanchez-Prado, J. Pablo Lamas, C. Garcia-Jares, E. Roca, and T. Dagnac. 2013. Hazardous organic chemicals in rubber recycled tire playgrounds and pavers. Chemosphere 90 (2):423–31. doi:https://doi.org/10.1016/j.chemosphere.2012.07.053.
- Oppegård, M., B. H. Hansen, and L. Sørensen. 2020. Determination of C0–C9 alkyl phenols in produced-water-exposed fish eggs using gas chromatography/tandem mass spectrometry. Rap Comm Mass Spectrom 34 (24):e8950. doi:https://doi.org/10.1002/rcm.8950.
- Rider, C. V., M. Vallant, C. Blystone, S. Waidyanatha, N. L. South, G. Xie, and K. Turner. 2020. Short-term perinatal toxicity study in Sprague Dawley rats with the plasticizer and emerging contaminant N-Butylbenzenesulfonamide. Toxicol. Lett. 330:159–66. doi:10.1016/j.toxlet.2020.05.005.
- Sait, S. T. L., L. Sørensen, S. Kubowicz, K. Vike-Jonas, S. V. Gonzalez, A. G. Asimakopoulos, and A. M. Booth. 2021. Microplastic fibres from synthetic textiles: Environmental degradation and additive chemical content. Environ. Pollut. 268:115745. doi:10.1016/j.envpol.2020.115745.
- Salazkin, S. N., and V. V. Shaposhnikova. 2013. Synthesis and behavior of phthalide-containing polymers, polymers for advanced technologies. Apple Academic Press. doi:10.1201/b14949-19.
- Sørensen, L., A. S. Groven, I. A. Hovsbakken, O. Del Puerto, D. F. Krause, A. Sarno, and A. M. Booth. 2021. UV degradation of natural and synthetic microfibers causes fragmentation and release of polymer degradation products and chemical additives. Sci. Total Environ. 755:143170. doi:10.1016/j.scitotenv.2020.143170.
- Sørensen, L., B. H. Hansen, J. Farkas, C. E. Donald, W. J. Robson, A. Tonkin, S. Meier, and S. J. Rowland. 2019. Accumulation and toxicity of monoaromatic petroleum hydrocarbons in early life stages of cod and haddock. Environ. Pollut. 251:212–20. doi:10.1016/j.envpol.2019.04.126.
- Sørensen, L., S. Schaufelberger, A. Igartua, T. R. Størseth, and I. B. Øverjordet. 2023. Non-target and suspect screening reveal complex pattern of contamination in Arctic marine zooplankton. Sci. Total Environ. 864:161056. doi:10.1016/j.scitotenv.2022.161056.
- Sundt, R. C., S. Rønnekleiv Haugedal, T. Rem, and P. -E. Schulze. 2020. Norske landbaserte kilder til mikroplast - Miljødirektoratet. Oslo, Norway: Miljødirektoratet/Norwegian Environment Agency.
- Tian, Z., H. Zhao, K. T. Peter, M. Gonzalez, J. Wetzel, C. Wu, X. Hu, J. Prat, E. Mudrock, R. Hettinger, et al. 2021. A ubiquitous tire rubber–derived chemical induces acute mortality in coho salmon. Science 371 (6525):185–89. doi:10.1126/science.abd6951.
- U.S. EPA. 2012. Estimation Programs Interface SuiteTM for Microsoft® Windows v 4.11. Washington, DC, USA: United States Environmental Protection Agency.
- Wagner, S., T. Hüffer, P. Klöckner, M. Wehrhahn, T. Hofmann, and T. Reemtsma. 2018. Tire wear particles in the aquatic environment - a review on generation, analysis, occurrence, fate and effects. Water Res. 139:83–100. doi:10.1016/j.watres.2018.03.051.