ABSTRACT
Herd immunity is a form of indirect protection that is offered to the community when a large proportion of individuals contained in the community are immune to a certain infection. This immunity can be due to vaccination or to the recovery post-disease. Effective herd immunity in SARS-CoV-2 infection has several hurdles upon achievement. Herd immunity cannot be obtained concomitantly in many geographical areas because the areas have different population density and the societal measures to contain the spreading are different. A proportion of 50–66% of the population needs to be immunized naturally or artificially in this SARS-Cov2 pandemic and this percentage is not easily achievable. The duration of herd immunity is another issue while information on the long-term immune response against SARS-CoV2 is yet scarce. Epitope stability, another issue to be solved when achieving herd immunity, is important. Mutation in the viral structure will call upon other sets of neutralizing antibodies and hence for other herd immunity type installment. The societal tactics to achieve the much-needed herd immunity should be developed keeping in mind the welfare of the population. Without being exhaustive, throughout our paper we will elaborate on each of the hurdles encountered in developing herd immunity to SARS-Cov2 infection.
KEYWORDS:
Introduction
The history of the pandemic we are facing today begins 15 years ago, when SARS-CoV determined the major pandemic of the new millennium.[Citation1–3] The rapid economic growth of China increased the demand for food and implicitly, exotic animal consumption. Simultaneously, the lack of bio-security measures in markets favored the passage of this new virus to humans.[Citation4,Citation5] The viral infection spread quickly due to lack of intra-hospital infection control and international travel. Fifteen years ago, 8,000 persons were infected and the infection had a 10% mortality rate. In a few months SARS-CoV was detected in several countries and the beginning of 2003 was facing a pandemic that was equaled only by the last known plague. By the end of 2003, when markets in China were re-opening, the virus quickly reemerged. The studies that followed the SARS-CoV pandemic have shown that a very similar virus was identified in horseshoe bats (Rhinolophus ferrumequinum). This type of bat has an extended range, from the south of UK to China and Japan, and extended to North Africa. Moreover, researchers pointed out this virus can gain mutations favoring human infection.[Citation6–9] Therefore, a paper published in 2007 highlighted that the viral reservoir in horseshoe bats coupled with some culinary habits in East Asia represents a “biological bomb” that we have to prepare for in the future years.[Citation10] Nevertheless this clear warning did not resonate, so after 15 years from SARS-CoV epidemic we are facing now the largest pandemic after the Spanish flu in 1918, with over 21 millions of infected persons and over 750,000 deaths worldwide.[Citation11]
In this current pandemic, there are several issues that need to be clarified: how dangerous is this infection, what co-morbidities favor a clinical bad outcome, how long the immunological memory lasts, what are the best therapies and how the world will cope with a long-lasting pandemic. To the question of how dangerous this virus is, the medical world still has to answer. Thus, from the clinical cases that are reported worldwide, SARS-Cov2 virus is highly pathogenic along with other viruses such as SARS, MERS, Yellow fever, Polio virus, West Nile virus, rabies virus, hepatitis viruses B, C, D and E, HTLV1 and HTLV2 and last, but not the least HIV.[Citation12] As the virus is highly pathogenic, research is seeking the best way to protect the public from this infectious disease. Within community protection, acquiring herd immunity or community immunity is one of the modalities to stop spreading the disease.
Without being exhaustive we will revise some of the issues that are related to the herd immunity acquirement in the current pandemic.
Herd immunity outlines
Herd or community immunity is established when a large part of the population within an area becomes immune to a specific disease and the infectious agent will stop spreading. As not each single individual is immune to the infection, the population as a whole offers protection. This happens because, proportionally there will fewer high-risk individuals in the entire population. Therefore, the infection rates decrease, and the disease gradually fades out. Herd immunity would protect at-risk individuals, like very young, very old or individuals that have a low immune response due to co-morbidities. Herd immunity can be achieved naturally by recovering after the disease or artificially through vaccination. After infection and recovery, the immunological memory will be sustained by memory immune clones that, if subsequent exposure to the same antigen occurs, will expand and protect the individual from the same infection. For example, the Zika virus outbreak in Brazil stopped because after 2 years, 63% of the population was exposed to the virus and hence herd immunity was reached in the population.[Citation13] The artificial way to obtain herd immunity is vaccination and probably the most used example is the elimination of poliomyelitis virus spread due vaccination.[Citation14]
The percentage needed for a community to reach herd immunity depends on the basic reproduction number (R0). R0 describes the average number of people that a single-infected person can infect nonimmune people. The higher the R0, the more people need to be resistant to reach herd immunity.[Citation15] For example, for a R0 less than one, the infectious disease spread will die out on its own.[Citation16] The 1918 Spanish flu pandemic that spread to one third of world’s population and had a death toll of 50 million had an R0 of 1.8.[Citation17] For SARS-Cov2, it was calculated that R0 is in the range of 2–3.[Citation18] Therefore, for these numbers a range of 50–67% of the population must be resistant so that herd immunity acquirement would drop the infection rates.[Citation19] A graphical outline of how the herd immunity can stop an infectious disease spread is presented in . Vaccination is a way to stop infectious diseases and this endeavor to build herd immunity was verified in many other infectious diseases.[Citation20]
Figure 1. Herd immunity in infectious diseases. Disease spreads rapidly in non-immunized community. If there are few immunized individuals the disease will spread sparing the immunized ones. If the community contains around 64% immunized individuals the disease will not spread to the susceptible ones and finally the virus spread will reduce
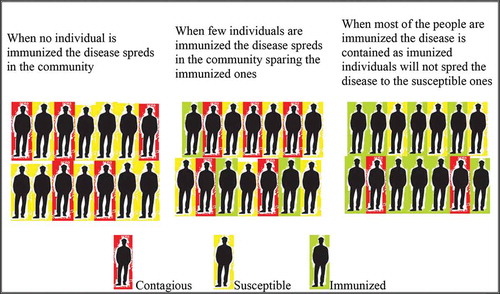
Deciphering populational immunity in different regions and countries for this type of infection, we must gather information on population heterogeneity, transmission and the accumulation of herd immunity.[Citation21] Nevertheless, there are still many hurdles in achieving a proper efficient vaccine against the COVID-19 disease.
Challenges in developing herd immunity to SARS-CoV2 infection
There are several hurdles in fighting SARS-Cov2 infection and moreover in establishing the intended herd immunity. First, it is an unknown or less known infection within the coronavirus family. Being new in humans, everyone is susceptible to infection now as there is no existing immunity to build on. Thus, as previously shown, the percentage of humans that are immunized one way or the other is important. Another issue is that even after recovering from infection, it is unknown how long the protection will be conferred or how long the immunological memory will last or more specifically how long the herd immunity is in action for that matter. The last, and as well important in establishing good and protective herd immunity, is the stability of the viral epitopes.[Citation22]
Immunological memory sustaining long-term herd immunity
T lymphocytes are involved in the early identification and further clearance of viral infections. Moreover, T-cells support the development of antibodies secreted by mature B lymphocytes. These are several reasons why recent studies in SARS-CoV-2 infection focus on T cell involvement in the immune response. For patients recovering from SARS-CoV and Middle East Respiratory Syndrome (MERS)-CoV it was reported that T-cell responses persist for more than ten years but with unknown protective efficacy.[Citation23] If we are facing a similar immunological pattern, long-term immunity in SARS-CoV-2, although IgG titers and memory B-cells may decline, memory T-cells could sustain a future immune response.[Citation24] A recent study published by a large group using two high-throughput immune profiling methods investigated T-cell response to SARS-CoV-2. It was shown that CD8 T-cell responses are initiated by a few immunodominant HLA-restricted epitopes. Specific T-cell response has a peak around 2 weeks post-infection and it is still detectable for several months. This data aids the route toward effective vaccine development.[Citation25]
More data will emerge in the post-pandemic period when the duration of effective immunity will be challenged. If the specific immunity is transient, the virus will lead to biennial/annual outbreaks entering a five years cycle. If the immunity is permanent this biannual/annual circulation will stop.[Citation26]
Seroconversion (seronegative to seropositive conversion) can be inefficient to neutralize viral particles. Wolfel et al. have published this year that seroconversion did not decline the viral load in sputum and antibody titers were not matching the clinical course of patients.[Citation27] Moreover, virus clearance is not entirely dependent on the antibody response, especially in severe cases.[Citation28] The neutralizing antibodies titers are significantly higher in middle-aged and elderly individuals compared to young individuals. The titer positively correlated with plasma CRP levels but negatively with the lymphocyte counts indicating an association between humoral response and cellular immune response.[Citation29] Therefore, it is still unknown if all recovered patients will have an adequate antibody response to the infection. The course of infection still lacks information. For example, patients that were discharged as cured were readmitted after 19 days,[Citation30] or after two consecutive negative reverse transcription-polymerase chain reaction test results, reemerged with positive results after 5 and 13 days later, suggesting viral shedding.[Citation31] These reports highlight that actual data regarding the course of infection in individuals are still to be gathered.
Stability of viral epitopes
An immune response established against certain viral epitopes will recognize the same epitopes upon re-infection. If the virus is subjected to mutations that are not cross-reactive with the ones prior generated, then the immune system will not have memory cells for them. The stability of viral epitopes is another concern in establishing herd immunity, as several reports show SARS-CoV-2 strains mutated.[Citation32] Interesting is that opposite to viruses with high fatality rate, infections with low fatality rates induce in the infected host an immune response that drives the virus to mutations in order toincrease its resistance to the immune attack. This effect would create individuals that have recovered and developed good immune response but overall the population immunity cannot be built as significant percentage as the virus continues to mutate and individual immunity is adapting to new viral epitopes. Thus, herd immunity would consist of several types of immunity against various mutated strains.[Citation33]
The SARS-CoV-2 strain that carries the Spike protein amino acid D614 is the most prevalent strain. But at multiple geographic levels a pattern with G614 mutation is continuously increasing. The shift suggests that the G614 mutated strain has some advantages in comparison to the initial D614 strain. G614 strain associates with lower RT-PCR cycle thresholds, meaning higher upper respiratory tract viral loads, nevertheless without an increased disease severity.[Citation34] The immune system tries to overcome the known genetic modification of the virus by developing multiple antibodies with various specificities. Recently it was shown that from convalescent plasma, 19 types of neutralizing antibodies could be isolated. These antibodies target multiple antigenic sites on the S protein of SARS-CoV-2 virus. The infection would trigger high-affinity and cross-reactive antibodies targeting the receptor-binding domain (RBD) and other sites on the S protein. From different sources, the potent neutralizing antibodies have VH segments identical to their germline origin, so that in case of vaccine development, extensive affinity maturation is not a mandatory requirement for neutralization capacity. The neutralization capacity of these antibodies is higher than of the most advanced HIV-1 and Ebola antibodies that are under evaluation.[Citation35] Zhou et al. has found similar results from convalescent plasma, namely a neutralizing antibody for SARS-CoV-2 cross-reacting with SARS-CoV-1. This antibody binds RBD and cryo electron microscopy has shown that the spike protein binds three Fab regions and two further multimeric forms containing the destabilized spike. This antibody has probably a major neutralizing function, important in COVID-19 therapy.[Citation36]
There are multiple antigenic RBD sites that are specific for SARS-CoV-2 and moreover there are additional non-RBD sites on the S protein of SARS-CoV and MERS-CoV,[Citation37] therefore guided vaccine can be effective in COVID-19 prevention and treatment.[Citation38]
The road to achieve herd immunity – countries’ experience
Probably the road to achieve herd immunity will not be identical in different geographical areas for a plentiful of reasons. The proportion of SARS-CoV-2-infected individuals within a population differs significantly between countries and between regions of the same country. In Italy, there was a notable gradient of infection from North to the South of Italy[Citation39] The same gradient is reported in USA from the North (Montana) to the South (California and New York State).[Citation40] This gradient of infection means that even if herd immunity is reached in one region the spread of the virus will continue in other regions. Countries which have a low infection rate will need longer time to reach herd immunity.[Citation24]
This topic was debated not only at research level but as well as government level with diverse implemented measures. While some of the countries followed the path to naturally occurring herd immunity, an option that worried the health care systems,[,Citation41,Citation42] other were more cautious in letting the virus spread freely.[Citation43] Brett and Rohani[Citation43] have shown that balancing between disease suppression and overwhelming hospitals, herd immunity is the primary objective. Thus, social distancing measures can be gradually relaxed and sustained for a longer period. Reaching herd immunity and counting the proportion of cases hospitalized,[Citation44] the fine-tuning of social distancing measures and their span in time will depend on the availability of health care system. The model designed by Verity et al.[Citation44] shows that the duration of achieving herd immunity depends on the health care availability, for example with 1.5 hospital beds per 1000 people, 6 months is necessary for herd immunity establishment. Another figure that needs to be considered is the number of hospitalization days. If a figure of 12.8 days[Citation45] or 22–24 days[Citation46] would induce a diminishing of R0 value below 1.3 to not exceed hospital capability and, in these conditions herd immunity would be achieved after 300 days of keeping normal restriction measures. Another epidemiological factor relates to age-related transmission. There are studies that show children are at similar risk of infection as adults[Citation47] while other show the 15–34 age group is driving SARS-CoV-2 transmission.[Citation48] Another epidemiologically related question refers to the seasonality of the infection. Other coronaviruses, HCoV-OC43 and HCoVHKU1, cause annual outbreaks in wintertime mainly in temperate regions,[Citation49] thus it is highly probable that seasonality will also characterize SARS-CoV-2 circulation.[Citation26] Knowledge of SARS-Cov2 seasonality is lacking, but if it will have the same pattern as the related coronaviruses, the outbreak will overlap with ongoing schools/universities attendance. Thus, social distancing and any other social measures will have low impact in controlling the spread. Therefore, additional variability is added that will block herd immunity achievement.[Citation26] Issues regarding the modeling proposed in published studies for achieving herd immunity, the duration and effectiveness of the specific immune response still remain unknown. If there are chances that individuals that had active infection and had built immunity can get re-infected, then there are reduced chances to achieve herd immunity via natural infection.[Citation26]
With each individual that is recovered from the Covid-19 disease the percentage to achieve herd immunity increases. Although there are cases of re-infection or possible re-infection,[,Citation30,Citation31,Citation50] concerns remain that the majority of the recovered population will not be re-infected within 3 months. Although a person may be re-infected with the circulating virus it is more likely that a person will be infected with a mutated strain. SARS-CoV-2 recovered individuals can induce a selection of mutant viruses, and further spread it in the community.[Citation33] Future outbreaks and/or seasonal outbreaks will directly depend on the long-lasting immune memory and by the cross-reactivity with any mutant virus that may emerge. Populational kinetics of specific immunity need to be thoroughly investigated considering specific antibodies but as well T and B memory lymphocytes. Although mass longitudinal immunity testing is necessary, it is difficult to achieve in various regions and countries due to lack of funding, lack of standard tests and possibly, lack of willingness. These kinetics immunity tests would provide invaluable information on the immunity through time to this specific viral infection.[Citation51]
Countries like Italy, France and Spain were facing troubling numbers by end of March 2020. Germany, with a robust health care system, imposed measures that prevented the overload of the system. In contrast, Sweden has chosen to combine a government response with instilling a sense of individual responsibility. The economic impact of these measures would be less severe compared to other countries and the herd immunity goal would be quickly achieved. In Sweden, it was predicted that with such measures in May 2020 seroconversion would reach a 40% in Stockholm. The results were that IgG seroprevalence was around only 15%.[Citation52] Orlowski and Goldsmith have stated that the earliest conclusions on the best herd immunity national tactics could be done only after 1–2 years.[Citation52] Spain with its lockdown tactics reached just a 5% seroprevalence and moreover it raised the proposition that achieving herd immunity by natural infection would be unethical.[Citation53]
Investigating over 30,500 individuals in Iceland using six assays, that identify specific IgM, IgG, and IgA antibodies against the virus nucleoprotein it was shown that 0.9% of this population sample was infected with SARS-CoV-2.[Citation54] Iceland having a low population number (approximately 350,000 citizens) and low density,[Citation55] the percentage of naturally immunized individuals is low.
Therefore, there were different measures in different countries. Europe has opted for a strategy of mitigation, intending to gradually achieve herd immunity, but due to the high infectivity, an exponential increase of cases in just weeks, thousands of deaths that put a high pressure on the health care systems in several countries, and this strategy was cataloged as unethical.
In East Asia, a completely different strategy was adopted, namely a very rapid lockdown and further follow-up measures to halt the virus spread. Differences in East Asian and Southeast Asian countries were noticed as well. For example, there was a complete lockdown in China, while only a moderate one in Japan. The results were that in China, there were fewer than 100,000 cases per 1.4 billion people, while in Japan under 5,000 cases per 126 million. The strategy described as “hammer and dance” by Pueyo combines strong suppression with controlled release and this strategyis probably the most efficient.[Citation56] The “hammer and the dance” strategy is an implementation of health care measures keeping in mind also the economic burden that can put additional pressure on a society struck by pandemic. The strong lockdown initially developed by governments temporarily limited economic activity in order to control the actual spread of the infection; this stage was named as “the hammer”. In this phase, economic rescue packages were implemented to protect companies across all sectors. This stage was however different again from country to country in terms of measures types, intensities, and durations. The second step consists of a gradual lifting of lockdown measures and restarting formerly closed economic activities. This is the step that we are now facing and during this phase, the spread of infection must be kept under control while being not completely suppressed, and this stage being named “the dance”.[Citation57] In this stage we must keep in mind that there is a lack of a vaccine or a specific anti-viral therapy that could stop the spread. However optimistic one could be, it is highly unlikely that the end of this year will bring a large-scale vaccine and mass vaccination. From the epidemiological point of view, the R-value should be below 1 in order to stop the outbreak, but for “the dance” stage we are in, it still limits citizens’ civil liberties. “Dancing” between epidemiological measures and civil liberties in the XXI century is not an easy task, both medically and socially. In Japan, the relaxation stage brought quickly another surge of infections due to returning to the normal human behavior and/or due to increased testing. Learning from the East Asian experience, in the “dance” stage we have to test and quarantine infected patients and contacts, implement serosurveillance of the population to depict what is the level of built-up immunity, what are its dynamics and how far are we from establishing herd immunity. These measures should be doubled by already known hygiene measures to limit infection spread. Keeping the population at a reduced infection rate would give space for implementation of measures toward economical stability and day-to-day life.[Citation58]
In Italy, there are clear differences between regions in the healthcare system, differences that present a strong challenge in the COVID-19 outbreak due to different regional policies, including the policy around population testing.[Citation59] Due to these differences in some regions, more individuals were tested and hospitalized in different percentages. This resulted in the fact that databases were different, so that the necessity to develop common national databases is important to accurately follow the immunity development.[Citation59,Citation60]
Inducing herd immunity
In the current COVID-19 pandemic there are currently no targeted therapies and no vaccine. Thus, without an actual vaccine/therapy a good proportion of the population would have to be infected to build herd immunity. A critical review of potential treatments for COVID-19 shows that there are various therapies and procedures that are mostly nonimmune therapies () and that the immune – based therapies are limited.[Citation61]
Figure 2. Therapies outline in COVID-19. Nonimmune therapies that target various viral and infected cell mechanisms outrun the immune-related therapies that comprise only vaccination and immune modulation
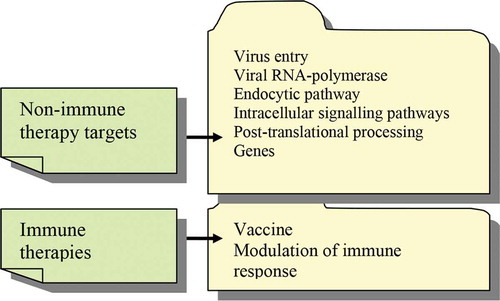
The best population protection could be achieved with vaccination, but although there are around 200 types of vaccines in the pipeline, we are lacking any confidence that we will have a vaccine implemented by the end of this year. There are vaccines that have around 10 years of testing before being applied and there are vaccines that never entered the clinical application stage due to stumbling in different testing stages. In the first phase, the safety of the vaccine and the appearance of secondary effects are tested on dozens of healthy volunteers. After the first phase completion, phase two tests are done on several hundreds of individuals and the efficacy of the vaccine is tested. In this phase individuals that are healthy or are diagnosed with various co-morbidities are tested in an epidemic area to analyze if the vaccine is efficient. In the third phase, the vaccine is tested on thousands of individuals and as previously stated, in each phase of testing new data can occur and so the actual clinical implementation can be done after ten years of tests or, as many other vaccines have gone through, never reaching clinical implementation.
Vaccination in COVID-19 disease has an accelerated pace. Therefore when we were writing a paper on this subject in March 2020 there were 44 types of vaccines, with two in the phase 1 of testing[Citation62,Citation63] and now after not more than 5 months, we have almost 200 vaccine types in the pipeline[Citation64] and probably until this paper will be published some additional ones will be added. Although the mentioned stages are mandatory the accelerated pace and the ethical concerns that arise on testing on healthy volunteers are still a matter of thorough debate.[Citation65]
The accelerated pace in achieving a proper vaccination can overlook cellular and molecular mechanisms within the understanding of COVID-19 disease. For example, if the vaccination would generate the immune process antibody-dependent enhancement (ADE) in COVID-19 vaccine, the scientific eye must be more vigilant. In the dengue virus infection, the symptoms are flu-like in most people with mild clinical evolution. In a small number of patients, a severe disease can develop, with fatal bleeding and organ damage. The risk of severe disease is increased in people who have been infected a second time. Dengvaxia, the first live-attenuated vaccine, protected previously infected children, but put non-infected individuals at risk of the disease.[,Citation66,Citation67] This phenomenon resulted in a lack of trust in public health[Citation68] and the lack of fundamental immunological information on ADE in this Dengvaxia.[Citation69] If the accelerated vaccine development in this pandemic will not fulfill the expectation for whatever reasons we will face public trust attrition in research and in public health overall decisions.[Citation69] Thus, ADE or any other immunological issues should have full consideration from the scientific community for any SARS-CoV-2 vaccine[Citation70–72] and the pace for vaccine development should follow the knowledge accumulation in this type of disease without cutting any research corners.
Although passive immunization is a good therapeutical option in all its facets[Citation73] mainly for difficult cases, it does not install herd immunity.
An interesting and pertinent opinion was recently published by Kostoff et al. stating that in the quest to find new therapies we must take into account intrinsically strengthening our immune system. This can be actually easily achieved by eliminating the factors that weaken the immune system.[Citation74] The factors that influence the immune system are extremely diverse, from lifestyle, diet to chronic inflammation and obesity, from neuroendocrine factors to environmental factors.[Citation75–80] Obesity is a factor that drives difficult cases of SARS-Cov2 infection and this is one of the first clinical observation in the current pandemic. A probable explanation is that the enlarged number of adipocytes would contribute to the increased inflammation pattern of the infection. Inflammatory cytokines would be produced first by the infected lung alveolar cells, the secreted inflammatory cytokines would call lymphocytes at the infection site generating more cytokines and adipocytes would secrete adipokines with pro-inflammatory functions. Thus, a normal inflammatory pattern will be rapidly transformed in the so-called “cytokine storm”.[Citation81] To characterize this cytokine storm, multiplex technology[Citation82] was used to evaluate in dynamics the level of various cytokines in Covid-19 patients, IL-6, TNF-α, IL-1β, as markers of inflammation and CXCL8/IL-8 as recruitment/activation cytokine for neutrophils.[Citation83] Results obtained on 1,400 patients have shown that these cytokines have a predictive power upon COVID-19 survival and mortality. IL-6 was found as a robust prognostic marker of survival, out-running CRP, D-dimer and ferritin. TNF-α, indicating organ damage, could predict poor outcome of the disease.[Citation84] IL-8 was found associated with survival time. These findings point out that the inflammatory status of the individual is yet again a measure of the disease outcome. The authors point out that COVID-19-related cytokine response is different from the cytokine storm associated with sepsis. Abolishing the cytokine storm, namely IL-6, was tried throughout several trails but large-scale results are still to be published.[Citation85,Citation86] Anti-inflammatory and immunosuppressant, dexamethasone, reduced also the level of IL-6.[Citation87] Early cytokine measurements could predict patient’s clinical evolution and hence could rapidly orient therapy.[Citation84] Another overlooked issue is that cytokine pattern is sex-dependent[Citation88] and that data gather upon differences in soluble IL-2 R, IL-6, ferritin, procalcitonin, LDH, and hsCRP in males versus female patients, and independently associated with disease severity in COVID-19 patients.[Citation89]
Conclusion
Effective herd immunity has complicated corollaries in SARS-CoV-2 infection. There are various factors and several hurdles to be solved in order to achieve it. Herd immunity in many geographical areas and locations is difficult to concomitantly reach because of population availability and societal differences. In Europe there are several differences between the countries, such as the health systems, specific interventions, country size, that may influence the overall rate of SARS-CoV-2 infection.
Mathematical epidemiology indicates that 50–66% of the population needs to be immunized naturally or artificially and this percentage is not easily achievable. Another issue that science has to establish is the duration of this herd immunity. It is still unknown for SARS-CoV2 infection how long is the immunity memory. An efficient immune response cannot be established in all individuals and cases of relapse have been reported. Epitope stability is yet another issue to be solved when achieving herd immunity because mutation in the viral structure will call upon other sets of neutralizing antibodies and hence for other molecular framework to herd immunity establishment. Effective vaccine is a possibility to control the current outbreak and induce herd immunity to prevent future outbreaks, but also strengthening of the immune system is a pathway to alleviate healthcare systems. Furthermore, societal tactics coping with the lockdown need to be thoroughly elaborated so that the economic impact should be minimum and the establishment of solid herd immunity in this infection would be optimal.
Acknowledgments
The author would like to thank Simina Neagu for paper proofreading.
Additional information
Funding
References
- Drosten, C.; Günther, S.; Preiser, W.; van der Werf, S.; Brodt, H.-R.; Becker, S.; Rabenau, H.; Panning, M.; Kolesnikova, L.; Fouchier, R. A. M.; et al. Identification of a Novel Coronavirus in Patients with Severe Acute Respiratory Syndrome. New England Journal of Medicine. 2003, 348(20), 1967–1976.
- Ksiazek, T. G.; Erdman, D.; Goldsmith, C. S.; Zaki, S. R.; Peret, T.; Emery, S.; Tong, S.; Urbani, C.; Comer, J. A.; Lim, W.; et al. A Novel Coronavirus Associated with Severe Acute Respiratory Syndrome. N. Engl. J. Med. 2003, 348(20), 1953–1966.
- Peiris, J. S.; Lai, S. T.; Poon, L. L.; Guan, Y.; Yam, L. Y.; Lim, W.; Nicholls, J.; Yee, W. K.; Yan, W. W.; Cheung, M. T.; et al. Coronavirus as a Possible Cause of Severe Acute Respiratory Syndrome. Lancet. 2003, 361, 1319–1325. DOI: 10.1016/S0140-6736(03)13077-2.
- Webster, R. G.;. Wet Markets—a Continuing Source of Severe Acute Respiratory Syndrome and Influenza? Lancet. 2004, 363, 234–236. DOI: 10.1016/S0140-6736(03)15329-9.
- Woo, P. C.; Kp Lau, S.; Yuen, K.-Y. Infectious Diseases Emerging from Chinese Wet-markets: Zoonotic Origins of Severe Respiratory Viral Infections. Curr. Opin. Infect. Dis. 2006, 19, 401–407. DOI: 10.1097/01.qco.0000244043.08264.fc.
- Che, X. Y.; Di, B.; Zhao, G.-P.; Wang, Y.-D.; Li-W., Q.; Hao, W.; Wang, M.; Qin, P.-Z.; Liu, Y.-F.; Chan, K.-H.; et al. A Patient with Asymptomatic Severe Acute Respiratory Syndrome (SARS) and Antigenemia from the 2003–2004 Community Outbreak of SARS in Guangzhou, China. Clin. Infect. Dis. 2006, 43, e1–e5. DOI: 10.1086/504943.
- Lau, S. K.; Woo, P. C.; Li, K. S.; Huang, Y.; Tsoi, H. W.; Wong, B. H.; Wong, S. S.; Leung, S. Y.; Chan, K. H.; Yuen, K. Y. Severe Acute Respiratory Syndrome Coronavirus-like Virus in Chinese Horseshoe Bats. Proc. Natl. Acad. Sci. USA. 2005, 102, 14040–14045. DOI: 10.1073/pnas.0506735102.
- Li, W.; Shi, Z.; Yu, M.; Ren, W.; Smith, C.; Epstein, J. H.; Wang, H.; Crameri, G.; Hu, Z.; Zhang, H.; et al. Bats are Natural Reservoirs of SARS-like Coronaviruses. Science. 2005, 310, 676–679. DOI: 10.1126/science.1118391.
- Wang, M.; Yan, M.; Xu, H.; Liang, W.; Kan, B.; Zheng, B.; Chen, H.; Zheng, H.; Xu, Y.; Zhang, E.; et al. SARS-CoV Infection in a Restaurant from Palm Civet. Emerg. Infect. Dis. 2005, 11, 1860–1865. DOI: 10.3201/eid1112.041293.
- Cheng, V. C.; Lau, S. K.; Woo, P. C.; Yuen, K. Y. Severe Acute Respiratory Syndrome Coronavirus as an Agent of Emerging and Reemerging Infection. Clin. Microbiol. Rew. 2007, 20(4), 660–694.
- https://www.worldometers.info/coronavirus/ ( accessed Aug 14).
- Osborn, M.; Lucas, S.; Stewart, R.; Swift, B.; Youd, E. Briefing on COVID-19. Autopsy Practice Relating to Possible Cases of COVID-19. The Royal College of Pathologists, https://www.rcpath.org/uploads/assets/d5e28baf-5789-4b0f-acecfe370eee6223/fe8fa85a-f004-4a0c-81ee4b2b9cd12cbf/Briefing-on-COVID-19-autopsy-Feb-2020.pdf.
- Carvalho, M. S.; Freitas, L. P.; Cruz, O. G.; Brasil, P.; Bastos, L. S. Association of past Dengue Fever Epidemics with the Risk of Zika Microcephaly at the Population Level in Brazil. Sci. Rep. 2020, 10, 1752. DOI: 10.1038/s41598-020-58407-7.
- Bandyopadhyay, A. S.; Macklin, G. R. Final Frontiers of the Polio Eradication Endgame. Curr. Opin. Infect. Dis. 2020, 33(5), 404–410. DOI: 10.1097/QCO.0000000000000667.
- Kirtimaan, S.;. COVID-19: Herd Immunity and Convalescent Plasma Transfer Therapy. J. Med. Virol. 2020. DOI: 10.1002/jmv.25870.
- Heffernan, J. M.; Smith, R. J.; Wahl, L. M. Perspectives on the Basic Reproductive Ratio. J. R. Soc. Interface. 2005, 2(4), 281–293. DOI: 10.1098/rsif.2005.0042.
- Biggerstaff, M.; Cauchemez, S.; Reed, C.; Gambhir, M.; Finelli, L. Estimates of the Reproduction Number for Seasonal, Pandemic, and Zoonotic Influenza: A Systematic Review of the Literature. BMC Infect. Dis. 2014, 14, 480. DOI: 10.1186/1471-2334-14-480.
- Liu, Y.; Gayle, A. A.; Wilder-Smith, A.; Rocklov, J. The Reproductive Number of COVID-19 Is Higher Compared to SARS Coronavirus. J. Travel Med. 2020, 27(2), taaa021. DOI: 10.1093/jtm/taaa021.
- Zhao, S.; Cao, P.; Gao, D.; Zhuang, Z.; Cai, Y.; Ran, J.; Chong, M. K. C.; Wang, K.; Lou, Y.; Wang, W.; et al. Serial Interval in Determining the Estimation of Reproduction Number of the Novel Coronavirus Disease (COVID-19) during the Early Outbreak. J. Travel Med. 2020, 27(3), taaa033. DOI: 10.1093/jtm/taaa033.
- Garnett, G. P.;. Role of Herd Immunity in Determining the Effect of Vaccines against Sexually Transmitted Disease. J. Infect. Dis. 2005, 191(Suppl 1), S97–106. PMID 15627236. DOI: 10.1086/425271.
- Thompson, R. N.; Hollingsworth, T. D.; Isham, V.; Arribas-Bel, D.; Ashby, B.; Britton, T.; Challenor, P.; Chappel, L. H. K.; Clapham, H.; Cunniffe, N. J.; et al. Key Questions for Modelling COVID-19 Exit Strategies, Key Questions for Modelling COVID-19 Exit Strategies. Proc. R. Soc. B 2020, 287, 20201405. DOI: 10.1098/rspb.2020.1405.
- Mallory, M. L.; Lindesmith, L. C.; Baric, R. S. Vaccination-induced Herd Immunity: Successes and Challenges. J. Allergy Clin. Immunol. 2018, 142, 64–66. DOI: 10.1016/j.jaci.2018.05.007.
- Rokni, M.; Ghasemi, V.; Tavakoli, Z. Immune Responses and Pathogenesis of SARS-CoV-2 during an Outbreak in Iran: Comparison with SARS and MERS. Rev. Med. Virol. 2020, 30, e2107. DOI: 10.1002/rmv.2107.
- Papachristodoulou, E., .; Kakoullis, L.; Parperis, K., .; Panos, G. Long-term and Herd Immunity against SARS-CoV-2: Implications from Current and past Knowledge. Pathog. Dis. 2020, 78, ftaa025. DOI: 10.1093/femspd/ftaa025.
- Snyder, T. M.; Gittelman, R. M.; Klinger, M.; May, D. H.; Osborne, E. J.; Taniguchi, R.; Zahid, H. J.; Kaplan, I. M.; Dines, J. N.; Noakes, M. N.; et al. Magnitude and Dynamics of the T-Cell Response to SARS-CoV-2 Infection at Both Individual and Population Levels. medRxiv. 2020, 4. DOI: 10.1101/2020.07.31.20165647.
- Kissler, S. M.; Tedijanto, C.; Goldstein, E., .; Grad, Y. H.; Lipsitch, M. Projecting the Transmission Dynamics of SARS-CoV-2 through the Postpandemic Period. Science. 2020, 368, 860–868. DOI: 10.1126/science.abb5793.
- Wolfel, R.; Corman, V. M.; Guggemos, W.; Seilmaier, M.; Zange, S.; Müller, M. A.; Niemeyer, D.; Jones, T. C.; Vollmar, P.; Rothe, C.; et al. Virological Assessment of Hospitalized Patients with COVID-2019. Nature 2020, 581, 465–469. DOI: 10.1038/s41586-020-2196-x.
- Zhao, J.; Yuan, Q.; Wang, H.; Liu, W.; Liao, X.; Su, Y.; Wang, X.; Yuan, J.; Li, T.; Li, J.; et al. Antibody Responses to SARS-CoV-2 in Patients of Novel Coronavirus Disease 2019. Clin. Infect. Dis. 2020, ciaa344. DOI: 10.1093/cid/ciaa344.
- Wu, F.; Wang, A.; Liu, M.; Wang, Q.; Chen, J.; Xia, S.; Ling, Y.; Yuling Zhang, Y.; Xun, J.; Lu, L.; et al. Neutralizing Antibody Responses to SARS-CoV-2 in a COVID-19 Recovered Patient Cohort and Their Implications. medRxiv. 2020. DOI: 10.1101/2020.03.30.20047365.
- Li, X.; Zhang, Z.; Zong, Z. A Case of A Readmitted Patient Who Recovered from COVID-19 in Chengdu. Crit. Care. 2020, 24, 8–10. DOI: 10.1186/s13054-020-02877-8.
- Lan, L.; Xu, D.; Ye, G.; Xia, C.; Wang, S.; Li, Y.; Xu, H. Positive RT-PCR Test Results in Patients Recovered from COVID-19. JAMA. 2020, 323, 1502–1503. DOI: 10.1001/jama.2020.2783.
- Wang, M.; Li, M.; Ren, R.; Li, L.; Chen, E.-Q.; Weimin Li, W.; Binwu Ying, B. International Expansion of a Novel SARS-CoV-2 Mutant. J. Virol. 2020, 94, e00567–20. DOI: 10.1128/JVI.00567-20.
- Biswas, A.; Bhattacharjee, U.; Chakrabarti, A. K.; Tewari, D. N.; Banu, H.; Dutta, S. Emergence of Novel Coronavirus and COVID-19: Whether to Stay or Die Out? Crit. Rev. Microbiol. 2020, 1–12.
- Korber, B.; Fischer, W. M.; Gnanakaran, W. M.; Yoon,H., S.; Theiler, J.; Abfalterer, W.; Hengartner, N.; Giorgi, E. E.; Bhattacharya, T.; Foley, B.; et al. Tracking Changes in SARS-CoV-2 Spike: Evidence that D614G Increases Infectivity of the COVID-19 Virus. Cell. 2020, 182(4), 812–827.e19. DOI: 10.1016/j.cell.2020.06.043.
- Pascal, K. E.; Dudgeon, D.; Trefry, J. C.; Anantpadma, M.; Sakurai, Y.; Murin, C. D.; Turner, H. L.; Fairhurst, J.; Torres, M.; Rafique, A.; et al. Development of Clinical-Stage Human Monoclonal Antibodies that Treat Advanced Ebola Virus Disease in Nonhuman Primates. J. Infect. Dis. 2018, 218(suppl_5), S612–S626.
- Zhou, D.; Duyvesteyn, H. M. E.; Chen, C.-P.; Huang, C.-G.; Chen, T.-H.; Shih, S.-R.; Lin, Y.-C.; Cheng, C.-Y.; Cheng, S.-H.; Huang, Y.-C.; et al. Structural Basis for the Neutralization of SARS-CoV-2 by an Antibody from a Convalescent Patient. Nat. Struct. Mol. Biol. 2020 Jul 31. Online ahead of print. DOI: 10.1038/s41594-020-0480-y.
- Shanmugaraj, B.; Siriwattananon, K.; Wangkanont, K.; Phoolcharoen, W. Review Perspectives on Monoclonal Antibody Therapy as Potential Therapeutic Intervention for Coronavirus Disease-19 (COVID-19). Asian Pac J. Allergy Immunol. 2020, 38(1), 10–18.
- Brouwer, P. J. M.; Caniels, T. G.; van der Straten, K.; Snitselaar, J. L.; Aldon, Y.; Bangaru, S.; Torres, J. L.; Okba, N. M. A.; Claireaux, M.; Kerster, G.; et al. Potent Neutralizing Antibodies from COVID-19 Patients Define Multiple Targets of Vulnerability. Science. 2020, 369(6504), 643–650. Epub 2020 Jun 15. DOI: 10.1126/science.abc5902.
- La Maestra, S.; Abbondandolo, A.; De Flora, S. Epidemiological Trends of COVID-19 Epidemic in Italy during March 2020. From 1,000 to 100,000 Cases. J. Med. Virol. 2020, 92(10), 1956–1961. DOI: 10.1002/jmv.25908.
- Wissel, B. D.; Van Camp, P. J.; Kouril, M.; Weis, C.; Glauser, T. A.; White, P. S.; Kohane, I. S.; Judith, W.; Dexheimer, J. W. An Interactive Online Dashboard for Tracking COVID-19 in U.S. Counties, Cities, and States in Real Time. J. Am. Med. Inform. Assoc. 2020, 27(7), 1121–1125. DOI: 10.1093/jamia/ocaa071.
- Hunter, D. J.;. Covid-19 and the Stiff Upper Lip—The Pandemic Response in the United Kingdom. N. Engl. J. Med. 2020, 382, e31. DOI: 10.1056/NEJMp2005755.
- Horton, R.;. Offline: COVID-19—a Reckoning. Lancet (London, England). 2020, 395(10228), 935. DOI: 10.1016/S0140-6736(20)30669-3.
- Brett, T. S.; Rohani, P. COVID-19 Herd Immunity Strategies: Walking an Elusive and Dangerous Tightrope. medRxiv. 2020. DOI: 10.1101/2020.04.29.20082065.
- Verity, R.; Okell, L. C.; Dorigatti, I.; Winskill, P.; Whittaker, C.; Imai, N.; Cuomo-Dannenburg, G.; Thompson, H.; Walker, P. G. T.; Fu, H.; et al. Estimates of the Severity of Coronavirus Disease 2019: A Model-based Analysis. Lancet Infect Dis. 2020, 20(6), 669–677. DOI: 10.1016/S1473-3099(20)30243-7.
- Guan, W. J.; Ni, Z. Y.; Hu, Y.; Liang, W. H.; Ou, C. Q.; He, J. X.; Liu, L.; Shan, H.; Lei, C.-L.; Hui, D. S. C.; et al. Clinical Characteristics of Coronavirus Disease 2019 in China. N. Engl. J. Med. 2020, 382(18), 1708–1720. DOI: 10.1056/NEJMoa2002032.
- Zhou, F.; Yu, T.; Du, R.; Fan, G.; Liu, Y.; Liu, Z.; Xiang, J.; Wang, Y.; Song, B.; et al. Clinical Course and Risk Factors for Mortality of Adult Inpatients with COVID-19 in Wuhan, China: A Retrospective Cohort Study. Lancet. 2020, 395(10229), 1054–1062.
- Bi, Q.; Wu, Y.; Mei, S.; Ye, C.; Zou, X.; Zhang, Z.; Liu, X.; Wei, L.; Truelove, S. A.; Zhang, T.; et al. Epidemiology and Transmission of COVID-19 in 391 Cases and 1286 of Their Close Contacts in Shenzhen, China: A Retrospective Cohort Study. Lancet 2020, 20, 911–919. DOI: 10.1016/S1473-3099(20)30287-5.
- Goldstein, E.; Lipsitch, M. Temporal Rise in the Proportion of Both Younger Adults and Older Adolescents among COVID-19 Cases in Germany: Evidence of Lesser Adherence to Social Distancing Practices? medRxiv. 2020. DOI: 10.1101/2020.04.08.20058719.
- Killerby, M. E.; Biggs, H. M.; Haynes, A.; Dahl, R. M.; Mustaquim, D.; Gerber, S. I.; Watson, J. T. Human Coronavirus Circulation in the United States 2014–2017. J. Clin. Virol. 2018, 101, 52–56. DOI: 10.1016/j.jcv.2018.01.019.
- Duggan, N. M.; Ludy, S. M.; Shannon, B. C.; Reisner, A. R.; Wilcox, S. R. Is Novel Coronavirus 2019 Reinfection Possible? Interpreting Dynamic SARS-CoV-2 Test Results through a Case Report. Am. J. Emerg. Med. 2020, [Epub ahead of print]. DOI: 10.1016/j.ajem.2020.06.079.
- Antia, A.; Ahmed, H.; Handel, A.; Carlson, N. E.; Amanna, I. J.; Antia, R.; Slifka, M. Heterogeneity and Longevity of Antibody Memory to Viruses and Vaccines. PLoS Biol. 2018, 16(8), e2006601. DOI: 10.1371/journal.pbio.2006601.
- Orlowski, E. J. W.; Goldsmith, D. J. A. Four Months into the COVID-19 Pandemic, Sweden’s Prized Herd Immunity Is Nowhere in Sight. J. R. Soc. Med. 2020, 113(8), 292–298. DOI: 10.1177/0141076820945282.
- Griffin, S.;. Covid-19: Herd Immunity Is “Unethical and Unachievable,” Say Experts after Report of 5% Seroprevalence in Spain. BMJ. 2020, 370, m2728. DOI: 10.1136/bmj.m2728.
- Gudbjartsson, D. F.; Norddahl, G. L.; Melsted, P.; Gunnarsdottir, K.; Holm, H.; Eythorsson, E.; Arnthorsson, A. O.; Helgason, D.; Bjarnadottir, K.; Ingvarsson, R. F.; et al. Humoral Immune Response to SARS-CoV-2 in Iceland. NEJM.org. 2020. DOI: 10.1056/NEJMoa2026116.
- World Health Organisation. WHO Director-General’s Opening Remarks at the Media Briefing on COVID-19-11. March 2020 [Internet]. https://www.who.int/dg/speeches/detail/who-director-general-s-opening-remarks-at-the-media-briefing-on-covid-19-11-march-2020.
- Pueyo, T.; Coronavirus: The Hammer and the Dance. https://medium.com/@tomaspueyo/coronavirus-the-hammer-andthe-dance-be9337092b56 2020.
- Assenza, T.; Collard, F.; Dupaigne, M.; Fève, P.; Hellwig, C.; Kankanamge, S.; Werquin, N. The Hammer and the Dance: Equilibrium and Optimal Policy during a Pandemic Crisis. CEPR Discussion Paper 14731, 2020.
- Jung, F.; Krieger, V.; Hufert, F. T.; Kupper, J.-H. How We Should Respond to the Coronavirus SARS-CoV-2 Outbreak: A German Perspective. Clin. Hemorheol. Microcirc. 2020, 7, 4363–4372. DOI: 10.3233/CH-209004.
- Sartor, G.; Del Riccio, M.; Dal Poz, I.; Bonanni, P.; Bonaccorsi, G. COVID-19 in Italy: Considerations on Official Data. Int. J. Infect. Dis. 2020, 9, 8188–8190.
- Rodriguez-Morales, A. J.; Cardona-Ospina, J. A.; Gutiérrez-Ocampo, E.; Villamizar-Peña, R.; Holguin-Rivera, Y.; Escalera-Antezana, J. P.; Alvarado-Arnez, L. E.; Bonilla-Aldana, D. K.; Franco-Paredes, C.; Henao-Martinez, A. F.; et al. Clinical, Laboratory and Imaging Features of COVID-19: A Systematic Review and Meta-analysis. Travel Med. Infect. Dis. 2020, 34, 101623. DOI: 10.1016/j.tmaid.2020.101623.
- Nitulescu, G. M.; Paunescu, H.; Moschos, S. A.; Petrakis, D.; Nitulescu, G.; Ion, G. N. D.; Spandidos, D. A.; Nikolouzakis, T. K.; Nikolaos Drakoulis, N.; Tsatsakis, A. Comprehensive Analysis of Drugs to Treat SARS-CoV-2 Infection: Mechanistic Insights into Current COVID-19 Therapies (Review). Int. J. Mol. Med. 2020, 46(2), 467–488. DOI: 10.3892/ijmm.2020.4608.
- https://www.gavi.org/vaccineswork/covid-19-vaccine-race
- Neagu, M.; Baicus, C.; Zurac, S. Pandemia Actuală – Back to the Future? Politici De Sanatate. 2020. https://www.politicidesanatate.ro/pandemia-actuala-back-to-the-future/
- https://www.webmd.com/lung/news/20200610/covid-19-latest-updates
- Daniela, C.; Hartung, T.; Docea, A. O.; Spandidos, D. A.; Egorov, A. M.; Shtilman, M. I.; Carvalho, F.; Tsatsakis, A. COVID-19 Vaccines: Ethical Framework Concerning Human Challenge Studies. DARU J. Pharm. Sci. DOI: 10.1007/s40199-020-00371-8.
- Halstead, S. B.;. Dengvaxia Sensitizes Seronegatives to Vaccine Enhanced Disease Regardless of Age. Vaccine. 2017, 35, 6355–6358. DOI: 10.1016/j.vaccine.2017.09.089.
- Santos, T. G.; Pharma Firm Issues Caution on Use of Anti-dengue Vaccine. 2017. https://technology.inquirer.net/69907/pharma-firm-issues-caution-anti-dengue-vaccine-sanofi-dengvaxia-vaccine-health-dengue.
- Fatima, K.; Syed, N. I. Dengvaxia Controversy: Impact on Vaccine Hesitancy. J. Glob. Health. 2018, 8. DOI: 10.7189/jogh.08.020312.
- Jiang, S.;. Don’t Rush to Deploy COVID-19 Vaccines and Drugs without Sufficient Safety Guarantees. Nature. 2020, 579, 321. DOI: 10.1038/d41586-020-00751-9.
- Iwasaki, A.; Yang, Y. The Potential Danger of Suboptimal Antibody Responses in COVID-19. Nat. Rev. Immunol. 2020, 20, 1–3. DOI: 10.1038/s41577-020-0321-6.
- Negro, F.;. Is Antibody-dependent Enhancement Playing a Role in COVID-19 Pathogenesis? Swiss Med. Wkly. 2020, 150, w20249.
- Coish, J. M.; MacNeil, A. J. Out of the Frying Pan and into the Fire? Due Diligence Warranted for ADE in COVID-19. Microbes Infect. 2020 Jun 24. [Epub ahead of print]. DOI: 10.1016/j.micinf.2020.06.006.
- Constantin, C.; Neagu, M.; Supeanu, T.; Chiurciu, V.; Spandidos, D. A. IgY - Turning the Page toward Passive Immunization in COVID-19 Infection. Exp. Ther. Med. 2020, 20(1), 151–158. https://www.spandidos-publications.com/10.3892/etm.2020.8704.
- Kostoff, R. N.; Briggs, M. B.; Porter, A. L.; Michael Aschner, M.; Spandidos, D. A.; Tsatsakis, A. COVID-19: Post-lockdown Guidelines. Int. J. Mol. Med. 2020, 46(2), 463–466. DOI: 10.3892/ijmm.2020.4640.
- Neagu, M.; Constantin, C.; Popescu, I. D.; Zipeto, D.; Tzanakakis, G.; Nikitovic, D.; Fenga, C.; Stratakis, C. A.; Spandidos, D. A.; Tsatsakis, A. M. Inflammation and Metabolism in Cancer Cell – Mitochondria Key Player. Front. Oncol. 2019, 9, 348. DOI: 10.3389/fonc.2019.00348.
- Neagu, M.;. Metabolic Traits in Cutaneous Melanoma. Front. Oncol. 2020, 10, 851. DOI: 10.3389/fonc.2020.00851.
- Neagu, M.; Constantin, C.; Caruntu, C.; Dumitru, C.; Surcel, M.; Zurac, S. Inflammation – Key Process in Skin Tumorigenesis. Oncol. Lett. 2019, 17, 4068–4084. DOI: 10.3892/ol.2018.9735.
- Neagu, M.; Constantin, C.; Longo, C. Chemokines in the Melanoma Metastasis Biomarkers Portrait. J Immunoassay Immunochem, 2015. 36(6):559-66. https://doi.org/10.3892/ol.2018.9735.
- Solomon, I.; Voiculescu, V. M.; Caruntu, C.; Lupu, M.; Popa, A.; Ilie, M. A.; Albulescu, R.; Caruntu, A.; Tanase, C.; Constantin, C.; et al. Neuroendocrine Factors and Head and Neck Squamous Cell Carcinoma – An Affair to Remember. Dis. Markers. 2018, 2018, Article ID 9787831. DOI: 10.1155/2018/9787831.
- Tzanakakis, G.; Giatagana, E.-M.; Kuskov, A.; Berdiaki, A.; Tsatsakis, A. M.; Neagu, M.; Nikitovic, D. Proteoglycans in the Pathogenesis of Hormone-dependent Cancers: Mediators and Effectors. Cancers. 2020, 12(9), E2401. DOI: 10.3390/cancers12092401.
- Alberto, B.;. Obesity, Inflammation and COVID-19. Swiss Med. Wkly. 2020, 150, w20349. DOI: 10.4414/smw.2020.20349.
- Popa, M.-L.; Albulescu, R.; Neagu, M.; Hinescu, M. E.; Tanase, C. Multiplex Assay for Multiomics Advances in Personalized-precision Medicine. J. Immunoassay Immunochem. 2019, 40, 3–25. Published online: 11 Jan 2019. DOI: 10.1080/15321819.2018.156294.
- Liu, J.; Li, S.; Liu, J.; Liang, B.; Wang, X.; Wang, H.; Li, W.; Tong, Q.; Yi, J.; Zhao, L.; et al. Longitudinal Characteristics of Lymphocyte Responses and Cytokine Profiles in the Peripheral Blood of SARS-CoV-2 Infected Patients. EBioMedicine. 2020, 55, 102763. DOI: 10.1016/j.ebiom.2020.102763.
- Del Valle, D. M.; Kim-Schulze, S.; Huang, -H.-H.; Beckmann, N. D.; Nirenberg, S.; Wang, B.; Lavin, Y.; Swartz, T. H.; Madduri, D.; Stock, A.; et al. An Inflammatory Cytokine Signature Predicts COVID-19 Severity and Survival. Nat. Med. 2020. DOI: 10.1038/s41591-020-1051-9.
- Xu, X.; Han, M.; Li, T.; Sun, W.; Wang, D.; Fu, B.; Zhou, Y.; Zheng, X.; Yang, Y.; Li, X.; et al. Effective Treatment of Severe COVID-19 Patients with Tocilizumab. Proc. Natl Acad. Sci. USA. 2020, 117, 10970–10975. DOI: 10.1073/pnas.2005615117.
- Gritti, G.; Raimondi, F.; Ripamonti, D.; Riva, I.; Landi, F.; Alborghetti, L.; Frigeni, M.; Damiani, M.; Micò, C.; Fagiuoli, S.; et al. IL-6 Signalling Pathway Inactivation with Siltuximab in Patients with COVID-19 Respiratory Failure: An Observational Cohort Study. medRxiv. 2020. DOI: 10.1101/2020.04.01.20048561.
- Ledford, H.;. Coronavirus Breakthrough: Dexamethasone Is First Drug Shown to Save Lives. Nature. 2020, 582, 469. DOI: 10.1038/d41586-020-01824-5.
- Surcel, M.; Constantin, C.; Caruntu, C.; Zurac, S.; Neagu, M. Inflammatory Cytokine Pattern Is Sex-dependent in Mouse Cutaneous Melanoma Experimental Model. J. Immunol. Res. 2017, 2017, Article ID 9212134, 1–10. DOI: 10.1155/2017/9212134.
- Zeng, Z.; Yu, H.; Chen, H.; Qi, W.; Chen, L.; Chen, G.; Yan, W.; Chen, T.; Ning, Q.; Han, M.; et al. Longitudinal Changes of Inflammatory Parameters and Their Correlation with Disease Severity and Outcomes in Patients with COVID-19 from Wuhan, China. Crit. Care. 2020, 24(1), 525. DOI: 10.1186/s13054-020-03255-0.