ABSTRACT
Capacitive-Resistive Electric Transfer (CRET) thermotherapies aim at tissue repair and regeneration through non-invasive application of RF currents. We have reported that the cellular response to subthermal CRET currents is non-linearly dependent on the signal frequency, and that in vitro exposure to a 448-kHz CRET signal promotes ADSC proliferation, as well as collagen and glycosaminoglycan synthesis in prechondrocytic cells. The present work investigates the response of neonatal fibroblasts to subthermal exposure (100 µA/mm2) to two CRET signals: a 448-kHz, non-modulated sinusoidal wave vs. a 20-kHz amplitude-modulation of the 448-kHz carrier. To that end, cell proliferation and expression of the biomarkers Hsp47, Hsp27 and decorin were assessed by cell count, PCNA and Western blotting. The results revealed that while both signals significantly and equivalently increased early (4 h) expression of Hsp47, the modulated signal was more efficient in inducing Hsp27 and decorin overexpression and promoting cell proliferation. These data indicate that the cellular response is dependent on the RF signal modulation and suggest that the therapeutic effects of CRET could be mediated by promotion of fibroblastic proliferation and overexpression of biomarkers that are essential in skin regeneration.
Introduction
Stimulation with radiofrequency (RF) currents or fields has been successfully applied in physical treatments for wound healing and tissue regeneration (Conner-Kerr and Isenberg Citation2012; Rawe and Vlahovic Citation2012). Capacitive-resistive electric transfer (CRET) therapies are based on non-invasive application of RF, sinewave electric currents within the 448–600 kHz range, aimed to induce hyperthermia in the treated tissues (Fousekis et al. Citation2020; Grimnes and Martinsen Citation2000; Kotnik and Miklavcic Citation2000; López-de-Celis et al. Citation2020). CRET therapies have been reported to be effective in treating bone (Kumaran and Watson Citation2019), muscle (Ganzit et al. Citation2000; Mondardini et al. Citation1999; Parolo and Honesta Citation1998; Takahashi et al. Citation1999; Yokota et al. Citation2018), or tendons and ligaments (Bito et al. Citation2019; Costantino et al. Citation2005; Stasinopoulos et al. Citation2020).
We have reported that in vitro exposure to 448 kHz, sinewave CRET current at a subthermal density induces proliferation of human adipose-derived stem cells (ADSC) (Hernández-Bule et al. Citation2014), as well as promotion of early chondrocyte differentiation through increased synthesis of cartilage-specific extracellular matrix molecules, such as collagen and glycosaminoglycans (Hernández-Bule et al. Citation2017). From this, we have proposed that such, electrically induced molecular and cellular effects could intervene in the tissue repairing properties attributed to CRET electrothermal therapies.
We have also reported that, as is the case for other RF electric and magnetic fields, the cellular response to CRET currents is non-linearly dependent on the frequency of the sinusoidal signal (Hernández-Bule et al. Citation2019). The modulation of the carrier signal is another parameter that has been shown relevant, both in the bioeffects induced by RF electromagnetic fields (Barbault et al. Citation2009; Blackman Citation2012; Juutilainen et al. Citation2011) as in those elicited by RF electric currents such as those used by the electric scalpel when in modes cutting vs. coagulation (Karaki et al. Citation2019). Thus, it is conceivable that modulation of the RF signal could also significantly influence the cellular response to CRET electric stimulation.
Based on the above, the present work investigates the response of human fibroblasts of cutaneous origin to in vitro exposure to 448-kHz CRET currents at a 100 µA/mm2 subthermal density, comparing the effects of the non-modulated sinewave with those of a signal in which the 448-kHz carrier is amplitude modulated at an envelope frequency of 20 kHz. The cellular responses investigated were related to the potential participation of fibroblasts in skin tissue repair: cell proliferation and expression of decorin (DCN) and heat shock proteins (HSP) Hsp47 and Hsp27, which are involved in skin regeneration (Atalay et al. Citation2009; Kovalchin et al. Citation2006; Millar and Murrell Citation2012; Reed and Iozzo Citation2002; Tukaj and Kaminski Citation2019). Fibroblastic Hsp47 is a specific chaperone for collagen synthesis and release (Nagata Citation1998) and is, among the constitutive and inducible HSPs, one of the most relevant for tissue repair (Ito and Nagata Citation2019; Masago et al. Citation2012). In subcutaneous tissues, Hsp47 induction parallels both cell proliferation and increased synthesis of type I collagen (Wang et al. Citation2002). As for Hsp27, it belongs to the family of small proteins that provide stability to actin microfilaments (Landry and Huot Citation1995; Lavoie et al. Citation1993, Citation1995) and inhibit stress-induced apoptosis (Concannon et al. Citation2003). Hsp27 phosphorylation increases at the wound edges during the healing phase (Hirano et al. Citation2002) and wound contraction is favoured by Hsp27 fibroblastic overexpression, while it is compromised when fibroblasts have low levels of the protein (Hirano et al. Citation2004). Regarding decorin, it constitutes 30% – 40% of all cutaneous proteoglycans (Li et al. Citation2013) and plays a prominent role in skin homeostasis, as revealed, for example, by the abnormal morphology of collagen fibrils and the skin fragility reported in mice having invalid DCN gene (Reed and Iozzo Citation2002).
The obtained results support previous indications that the cellular response to CRET exposure is dependent on electric signal parameters such as frequency and modulation. Indeed, although these results show that both of the tested signals significantly and similarly increase early (4 hours) Hsp47 expression, the early Hsp27 expression and later (20 h) DCN expression were significantly increased by the modulated signal, but not by the non-modulated one. Regarding fibroblastic proliferation, it was significantly increased by both signals, with the modulated one being more effective. The results are also suggestive that the effects of CRET therapies on tissue regeneration could be mediated in part by electrically-induced changes in the fibroblastic expression of HSP that are potentially capable of promoting cell survival and wound repair.
Material and methods
Cell culture
Primary cultures of human dermal fibroblast (HDF neonatal; C-004-5 C Gibco, MA, USA) were maintained in high-glucose D-MEM (Biowhittaker, Lonza, Verviers, Belgium) supplemented with 10% inactivated foetal bovine serum (FBS; Gibco BRL, Invitrogen, Paisley, Scotland, UK), 1% glutamine and 1% penicillin-streptomycin (Gibco, Invitrogen, Thermo Fisher Scientific, Waltham, MA USA). The cells were subcultured once a week and seeded in 60 mm Petri dishes (Nunc, Roskilde, Denmark) at a density of 5500 cells/cm2, either directly on the dish or on glass coverslips placed inside the dish. A total of 8 Petri dishes were used in each experimental run.
CRET exposure
The RF exposure procedure has been described in detail elsewhere (Hernández-Bule et al. Citation2014). Briefly, the RF currents were delivered through pairs of sterile stainless steel electrodes designed ad hoc for in vitro stimulation. The stimulation pattern consisted of 5-minute pulses of RF current delivered at a subthermal density of 100 µA/mm2, separated by 4-h interpulse lapses, for a total period of 4:10 h (i.e., two 5-minute pulses with a 4-hour interval), 24 h or 48 h, depending on the experiment. The stimulation parameters and the atmosphere inside the incubators (37 °C, 90% relative humidity and 5% CO2) were constantly monitored. The electromagnetic environment inside the incubators (Thermo Fisher Scientific, Waltham, MA, USA) was monitored using specific magnetometers for three frequency ranges of interest: static DC field (Bartington model Mag-03, GMV associates, San Carlos, CA, USA), 50 Hz AC (EFA-3 model BN 2245/90.20, Wandel & Goltermann, Eningen, Germany) and RF < 3 GHz (PMM 8053 portable field strength meter with external PMM EP-330 E-field probe, both from Narda Safety Test Solutions, Milan, Italy). The obtained values (means ± SD) were BDC = 23.5 ± 3.3 μT rms, BAC = 4.5 ± 2 μT rms and ERF< 0.03 V/m (detection threshold). Two different current signals were tested: a 448-kHz, sinusoidal, non-modulated signal that has been reported to regulate human stem cell proliferation and differentiation (Hernández-Bule et al. Citation2016, Citation2014, Citation2017) and a 20-kHz, 40% amplitude modulation of the 448-kHz sinewave signal. On day 4thpostplating, electrode pairs were fitted inside all 8 Petri dishes and connected in series to a signal generator (model Elite NS, IDC 0309, INDIBA®, Barcelona, Spain). Only electrodes inserted in the 4 dishes corresponding to the RF-exposed samples were energized, the samples in the remaining 4 dishes being considered sham-exposed controls.
Cell viability and proliferation
Cells were harvested at the end of 48 h of RF- or sham-exposure and their viability was quantified by Trypan blue exclusion method, using 0.4% Trypan blue (Sigma, Steinheim, Germany). Five experimental replicates were conducted per signal pattern. Cell proliferation at 24 or 48 hours of RF- or sham-treatment was tested through Western blotting quantification of proliferating cell nuclear antigen (PCNA) expression.
Immunofluorescence
The expression levels of proteins Hsp47 and Hsp27 and the proteoglycan decorin were immunoblot assessed. The procedure has been described in detail elsewhere (Hernández-Bule et al. Citation2010). Briefly, at the end of each experimental run, the cell samples were centrifuged and lysed, and the protein concentrations were determined by Pierce BCA Protein Assay (Thermo Fisher Scientific, Rockford, IL, USA). The protein samples (60 μg protein aliquots) were separated in 10% sodium dodecyl sulfate-polyacrylamide gel and electrophoretically transferred to nitrocellulose membranes (Amersham, Buckinghamshire, UK). For decorin determination, two buffer types: reducing and non-reducing, were used to discriminate between protein bands. This way, bands both of 50 and 100 kDa were obtained when in the presence of ß-mercaptoethanol, whereas only the 100 kDa band was observed when ß-mercaptoethanol was absent. The blots were incubated at 4 °C overnight with rabbit anti-PCNA antibody, anti-Hsp47 mouse monoclonal antibody (both diluted 1:1000; Santa Cruz Biotechnology), anti-Hsp27 rabbit polyclonal antibody (1:1000, Cell Signaling) or rabbit anti-decorin polyclonal antibody (1:1000, Thermo Fisher Scientific-Invitrogen). Anti-GAPDH rabbit polyclonal antibody (1:1000, Santa Cruz Biotechnology) was used as loading control. Immunoreactive bands were detected with ECL-anti-mouse IgG horseradish peroxidase-linked antibody or with ECL-anti-rabbit IgG horseradish peroxidase-linked antibody (GE Healthcare, Little Chalfont, Buckinghamshire, UK). ECL-chemiluminescence in the blots was detected by ChemiDoc Imager (Bio-Rad, Utrecht, Netherlands). The obtained bands were densitometry analysed (PDI Quantity One 4.5.2 software, Bio-Rad, Munich, Germany) and the values were normalized against the corresponding GAPDH band. Nineteen experimental replicates for PCNA protein, eleven replicates for Hsp47 and eight replicates for Hsp47 analysis were conducted. Eight samples (4 CRET- and 4 sham-exposed) were analysed per replicate and per signal pattern.
Statistical analysis
All experimental procedures and analyses were conducted blindly for treatment. Data were normalized and expressed as means ± standard error (SEM) of at least four independent experimental replicates. The data corresponding to treated groups and their respective controls were compared by the Student’s t-test using GraphPad Prism software (San Diego, CA, USA). The threshold for statistical significance was set at p < 0.05.
Results
CRET effects on cell proliferation
Cell number
The 48-hour treatment with either of the two CRET signals induced statistically significant increases in cell number (). The non-modulated signal induced a significant, 10.5% average increase over its sham-exposed controls (p < 0.01), whereas modulation induced a 20.2% increase over the corresponding controls (p < 0.001), as well as a significant cell number increase over that obtained in response to the non-modulated signal (p < 0.05).
Figure 1. Proliferative response to CRET stimulation. Trypan blue quantification of cell number in samples intermittently exposed (5 min On/4 hours Off) or sham-exposed for 48 h. Both CRET signals, non-modulated (Non-Mod) and modulated (Mod) significantly increased cell proliferation. Each bar represents the mean ± SEM of 5 independent replicates, with 4 CRET-exposed dishes and 4 sham-exposed controls per replicate. The data are normalized over the respective controls (dotted line 100%). *: 0.01 ≤ p < 0.05; **: 0.001 ≤ p < 0.01; ***: p < 0.001; Student’s t-test
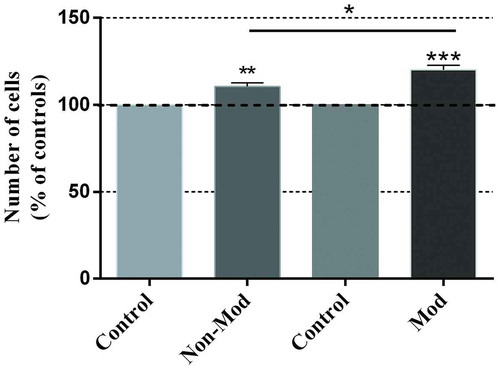
PCNA expression
Both CRET signals significantly promoted PCNA expression ( (a,b)), the response to the modulated signal occurring earlier (at 24 h) than that to the non-modulated signal (at 48 h), which is in accordance with the above described increases in cell number after 48 hours of CRET exposure.
Figure 2. Western blotting of PCNA expression. (a) Analysis of the response to 24 or 48 hours of CRET stimulation from day 4th after plating. Data are normalized over the corresponding sham-exposed controls and expressed as the means ± SEM of 9 independent replicates with the Non-Mod signal (N = 4 at 24 h and N = 5 at 48 h) and 10 replicates with the Mod signal (N = 5 at 24 h and N = 5 at 48 h). *: 0.01 ≤ p < 0.05, Student’s t-test. (b). Densitometry of PCNA protein: representative blots; 60 μg protein per lane. For quantitative analysis, the density of each band was normalized over that of the GAPDH protein
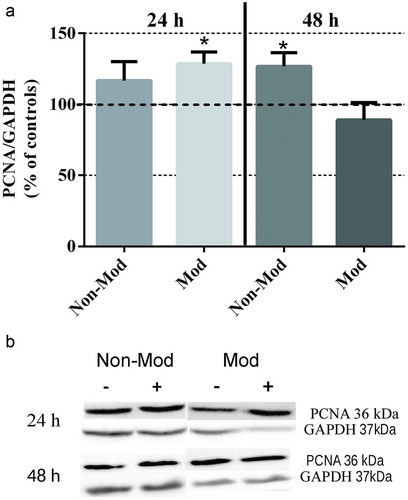
CRET effects on Hsp47 and Hsp27 expression
Although the immunoblot assay at 4:10 hours revealed that Hsp47 was constitutively expressed in both control and CRET-exposed cultures, the data analysis showed that, compared to their respective control groups, the expression levels of the protein increased significantly and equally in samples stimulated with either of the two CRET signals, ()). The analysis revealed that Hsp27 was also constitutively expressed in control cells ()) and this expression was increased in CRET-stimulated samples, although only under treatment with the modulated signal the difference over controls was statistically significant. These results received support from the immunofluorescence analysis ()), which revealed a general increase of Hsp47 immunolabeling present in the cytoplasm of CRET-stimulated samples, as well as a higher density of Hsp27 labeling in the samples stimulated with the modulated signal.
Figure 3. Western blot analysis and photomicrographs of Hsp47 and Hsp27 expression after 4:10-hour treatment. Means ± SEM of protein expression in samples exposed to Non-Mod or Mod CRET signals, normalized over the respective sham-exposed controls, and the corresponding representative blots (60 μg protein per lane) from the densitometric assays. For quantitative analysis, the density value of each band was normalized over that of the GAPDH protein. (a) Hsp47 expression; 5 or 6 repeats per experimental group. (b) Densitometry for Hsp47, representative blots. (c) Hsp27 expression; 6 repeats per experimental group. (d) Densitometry for Hsp27 protein, representative blots. NS. Not significant statistically. **: 0.001 ≤ p < 0.01, Student’s t-test. (e) Immunofluorescent staining for Hsp47 (red) and Hsp27 (green) intracellular location in CRET- and sham-exposed samples: representative micrographs in which the cell nuclei (blue) were Hoechst counterstained; original magnification: x400. While in sham-exposed samples, Hsp47 and Hsp27 formed small granular particles evenly distributed throughout the cytoplasm, increased and dense Hsp47 and Hsp27 immunostainings was found in the cytoplasm of CRET-exposed cells. Besides, although no obvious differences with respect to controls were detected in the Hsp47 immunostaining pattern of CRET-exposed samples, in many of these RF-stimulated cells, the Hsp47 labeling also concentrated along the distal end of their cytoplasmic prolongations
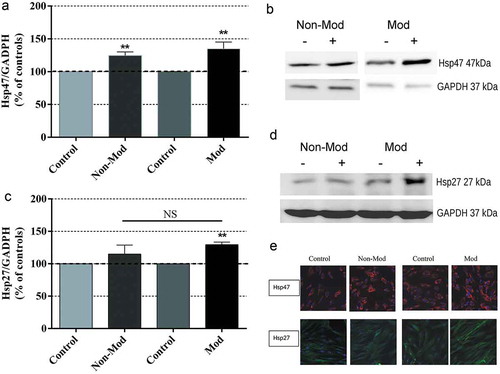
CRET effects on decorin expression
After 24 hours of treatment, the decorin central protein (DCN-core; 50 kDa) was significantly overexpressed by the modulated signal, the non-modulated one being ineffective ()). As for the mature protein (DCN-GAG) of 100 kDa, neither signal significantly changed its expression with respect to the corresponding sham-exposed controls ()).
Figure 4. Western blotting of decorin (DCN) expression after 24-hour exposure to Non-Mod or Mod CRET signals. (a) Expression of DCN core (DCN-core/GAPDH ratio) (b) Expression of mature decorin (DCN-GAG/GAPDH ratio). Data are means ± SEM of 6 repeats per experimental group, normalized over the respective sham-exposed controls. *: 0.01 ≤ p < 0.05, Student’s t-test. (c). Densitometry of the two DCN proteins (60 μg protein per lane): representative blots. For quantitative analysis, the density value of each band was normalized over that of the GAPDH protein
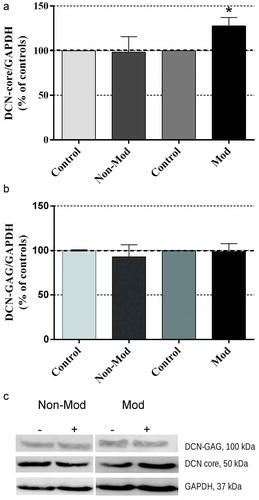
Discussion
The present results show that, as in ADSC from healthy donors (Hernández-Bule et al. Citation2014), sub-thermal stimulation with CRET currents significantly increases proliferation in neonatal skin fibroblast, in which a 48-hour exposure to the modulated signal elicited a cell number increase significantly greater than that induced by the unmodulated signal (). PCNA expression was also significantly increased in response to both CRET signals, but the effect of the modulated signal was 24 hours ahead of that of the unmodulated one (), indicating that modulation could boost the response to the signal. On the other hand, increased PCNA levels might be interpreted as suggestive of DNA damage due to CRET exposure. However, since such effect is accompanied by increased cell number, the proliferative response is more likely to be mediated by CRET-induced stimulation of cell cycle progression, as previously observed in ADSC exposed to the non-modulated electric signal (Hernández-Bule et al. Citation2014). Therefore, it is conceivable that the electrically induced promotion of stem cell and fibroblast proliferation could intervene in the beneficial effects on tissue repair and regeneration attributed to CRET therapies.
Regarding heat shock proteins as potential molecular markers of the response to CRET stimulation, we have focused on Hsp27 (also called HspB1 in humans or Hsp25 in mice) and Hsp47 because of their relevance in skin tissue regeneration. In fact, Hsp47, which belongs to the serpin gene family, is functionally associated with collagen maturation and, along with Hsp27, is a pivotal protein in skin homeostasis and functionality (Dams et al. Citation2010). In turn, Hsp27 controls cellular functions related to actin cytoskeleton remodelling in various cell types and, in fact, it colocalizes with actin filaments in cardiac (Lutsch et al. Citation1997), skeletal (Neufer and Benjamin Citation1996) and smooth muscle tissues (Bitar Citation2002). In fibroblasts, Hsp27 participates in the regulation of cell adhesion and motility, as well as in that of the contraction of the extracellular matrix (Hirano et al. Citation2004). Furthermore, there is strong evidence that actin microfilaments stabilization and accelerated recovery after their disruption are part of the main antioxidant and protective functions of Hsp27 against stress, whether thermal or otherwise (Huot et al. Citation1996; Lavoie et al. Citation1995; Vidyasagar et al. Citation2012).
The results reported here show that both CRET signals significantly increase Hsp47 protein expression (). Among the endoplasmic reticulum-resident stress proteins, only Hsp47 is heat shock-induced (Ito and Nagata Citation2019) and its transcription from the corresponding gene is regulated by a heat shock element (HSE) located in the promoter region. However, the constitutive, tissue-specific Hsp47 expression, which correlates with that of collagen, is regulated via enhancer elements located in the promoter and intron regions of the DNA (Ishida and Nagata Citation2011). In the present experiments Hsp47 overexpression is induced by a sub-thermal stimulus, thus, it is likely that such effect is not regulated by the HSE, but by said enhancer regulatory elements, which are key players in cell and tissue homeostatic responses to external stimuli or disease (Andersson and Sandelin Citation2020). This would be consistent with the reported collagenogenic effects induced by CRET in human stem cells (Hernández-Bule et al. Citation2017).
Regarding protein Hsp27, both its phosphorylation (32.9% ± 12.8% over controls; p < 0.05; data not shown) and its expression were significantly increased by short-term exposure to the modulated CRET signal (). The samples exposed to the non-modulated signal showed Hsp27 overexpression too, but the differences with respect to their sham-exposed controls were not significant statistically. This points to the possibility that, like Hsp47, Hsp27 is sensitive to both CRET signals, the modulated one being more effective in inducing early overexpression of this protein.
The results show that the signal modulation parameter is also relevant to the CRET-induced expression of the small leucine rich proteoglycan (SLRP) decorin. After being synthesized in the fibroblast endoplasmic reticulum (Fransson et al. Citation2000), the decorin central protein, DCN-core, is transported to the Golgi apparatus, where it binds to a GAG chain to form the mature decorin molecule (Tiedemann et al. Citation2001). The present results reveal that a 24-hour stimulation with the modulated signal induced significant overexpression of DCN-core, but not of mature decorin, which could be due to the fact that the stimulus was applied at an early stage, prior to formation of the mature protein. On the other hand, it has been reported that the Hsp47 protein residing in the endoplasmic reticulum binds decorin with an affinity comparable to that of the interaction between Hsp47 and type I collagen, and that the lack of Hsp47 inhibits both the secretion of collagen and that of the SLRPs lumican and decorin (Ishikawa et al. Citation2018). This inkling that besides playing a role in collagen folding and secretion (Ishida et al. Citation2006; Ishikawa et al. Citation2016; Nagai et al. Citation2000) Hsp47 can intervene in SLRP secretion, contributes to better understanding the coordinated molecular interactions that control the secretion and organization of the functional collagen matrix. We do not know whether the decorin overexpression induced after 24 hours of exposure to the modulated signal is mediated by the prior induction (at 4:10 hours) of Hsp47 overexpression. However, we do know that both markers are closely related to collagenogenesis and fibrillogenesis. Indeed, decorin exerts functions of alignment, stabilization and regulation of the diameter of collagen fibres, and acts as a bridge between various collagen types. Since it also performs as a signaling mediator of membrane receptors such as the epidermic growth factor receptor, decorin is considered a relevant biomarker in processes involved in skin aging or wound healing and regeneration (Hill et al. Citation2018; Li et al. Citation2007; Ma et al. Citation2014; Pang et al. Citation2020). In this context, the present results are suggestive that Hsp47, Hsp27 and decorin may take part of a pool of biomarkers whose electrically induced overexpression in fibroblasts could intervene in processes of cell migration, extracellular matrix contraction, collagenogenesis and fibrillogenesis, which are essential in tissue repair by CRET therapies.
In sum, the results of this study, which are consistent with previously reported collagenogenic and cytoproliferative effects of electric stimulation with the non-modulated CRET signal (Hernández-Bule et al. Citation2019, Citation2014, Citation2017), reveal that subthermal CRET stimulation is also effective in promoting fibroblast proliferation, the modulated signal being more effective than the non-modulated one in eliciting such response. Furthermore, although in the short term (4 h:10 min) both signals induce significant overexpression of the collagen-specific chaperone Hsp47, only the modulated signal induces significant activation and overexpression of Hsp27 in the same early phase, as well as decorin overexpression 20 hours later (24 hours after the exposure onset). Thus, modulation at 20 kHz would increase the efficiency of the 448-kHz sinusoidal signal at early inducing the studied responses. Work is in progress to elucidate whether or not acceleration of processes involved in tissue regeneration is the primary effect of modulation. Deepening knowledge on long-term CRET effects on factors involved in the regulation of fibrillogenesis and connective tissue regeneration is essential to better understand the phenomena underlying the electrically induced cellular responses. Such knowledge might significantly contribute to optimize and expand the medical applications of these RF therapies.
Declaration of interest
The authors declare that they have no competing interests.
References
- Andersson, R., and A. Sandelin. 2020. Determinants of enhancer and promoter activities of regulatory elements. Nat. Rev. Genet. 21:71–87. doi:10.1038/s41576-019-0173-8.
- Atalay, M., N. Oksala, J. Lappalainen, D. Laaksonen, C. Sen, S. Roy, et al. 2009. Heat shock proteins in diabetes and wound healing. Curr. Protein Pept. Sci. 10:85–95. doi:10.2174/138920309787315202.
- Barbault, A., F. P. Costa, B. Bottger, R. F. Munden, F. Bomholt, N. Kuster, B. Pasche, et al. 2009. Amplitude-modulated electromagnetic fields for the treatment of cancer: Discovery of tumor-specific frequencies and assessment of a novel therapeutic approach. J. Exp. Clin. Cancer Res. 28:51. doi:10.1186/1756-9966-28-51.
- Bitar, K. N. 2002. HSP27 phosphorylation and interaction with actin-myosin in smooth muscle contraction. Am. J. Physiol. Gastrointest. Liver Physiol. 282:G894–903. doi:10.1152/ajpgi.00141.2001.
- Bito, T., Y. Tashiro, Y. Suzuki, Y. Kajiwara, H. Zeidan, M. Kawagoe, T. Sonoda, Y. Nakayama, Y. Yokota, K. Shimoura, et al. 2019. Acute effects of capacitive and resistive electric transfer (CRet) on the Achilles tendon. Electromagn. Biol. Med. 38:48–54. doi:10.1080/15368378.2019.1567525.
- Blackman, C. F. 2012. Treating cancer with amplitude-modulated electromagnetic fields: A potential paradigm shift, again? Br. J. Cancer 106:241–42. doi:10.1038/bjc.2011.576.
- Concannon, C. G., A. M. Gorman, and A. Samali. 2003. On the role of Hsp27 in regulating apoptosis. Apoptosis 8:61–70. doi:10.1023/A:1021601103096.
- Conner-Kerr, T., and R. A. Isenberg. 2012. Retrospective analysis of pulsed radiofrequency energy therapy use in the treatment of chronic pressure ulcers. Adv. Skin Wound Care 25:253–60. doi:10.1097/01.ASW.0000415342.37554.ed.
- Costantino, C., F. Pogliacomi, and E. Vaienti. 2005. Cryoultrasound therapy and tendonitis in athletes: A comparative evaluation versus laser CO2 and t.e.ca.r. therapy. Acta Biomed. 76:37–41.
- Dams, S. D., M. de Liefde-van Beest, A. Nuijs, et al. 2010. Pulsed heat shocks enhance procollagen type I and procollagen type III expression in human dermal fibroblasts. Skin Res. Technol. 16:354–64. doi:10.1111/j.1600-0846.2010.00441.x.
- Fousekis, K., G. Chrysanthopoulos, M. Tsekoura, D. Mandalidis, K. Mylonas, P. Angelopoulos, D. Koumoundourou, V. Billis, E. Tsepis, et al. 2020. Posterior thigh thermal skin adaptations to radiofrequency treatment at 448 kHz applied with or without Indiba® fascia treatment tools. J. Phys. Ther. Sci. 32:292–96. doi:10.1589/jpts.32.292.
- Fransson, L. A., M. Belting, M. Jönsson, K. Mani, J. Moses, Å. Oldberg, et al. 2000. Biosynthesis of decorin and glypican. Matrix Biol. 19:367–76. doi:10.1016/s0945-053x(00)00083-4.
- Ganzit, G. P., L. Stefanini, and G. Stesina. 2000. Nuove methodice nei trattamento della patología muscolo-articolare dell´atleta. La tecarterapia. Med. Sport 53:361–67.
- Grimnes, S., and Ø. G. Martinsen. 2000. Joule effect and temperature rise. Bioimpedance and bioelectricity basics, 71–73. London: Academic Press: Harcourt and Technology Co.
- Hernández-Bule, M. L., C. L. Paíno, M. A. Trillo, and A. Úbeda. 2014. Electric stimulation at 448 kHz promotes proliferation of human mesenchymal stem cells. Cell. Physiol. Biochem. 34:1741–55. doi:10.1159/000366375.
- Hernández-Bule, M. L., E. Medel, C. Colastra, R. Roldán, and A. Úbeda. 2019. Response of neuroblastoma cells to RF currents as a function of the signal frequency. BMC Cancer 19:889. doi:10.1186/s12885-019-6090-6.
- Hernández-Bule, M. L., J. Martínez-Botas, M. A. Trillo, C. L. Paíno, and A. Úbeda. 2016. Antiadipogenic effects of subthermal electric stimulation at 448 kHz on differentiating human mesenchymal stem cells. Mol. Med. Rep. 13:3895–903. doi:10.3892/mmr.2016.5032.
- Hernández-Bule, M. L., M. A. Cid, M. A. Trillo, J. Leal, and A. Ubeda. 2010. Cytostatic response of HepG2 to 0.57 MHz electric currents mediated by changes in cell cycle control proteins. Int. J. Oncol. 37:1399–405.
- Hernández-Bule, M. L., M. A. Trillo, M. A. Martínez, C. Abilahoud, and A. Úbeda. 2017. Chondrogenic differentiation of adipose-derived stem cells by radiofrequency electric stimulation. J. Stem Cell. Res. Ther. 7:12. doi:10.4172/2157-7633.1000407.
- Hill, L. J., R. J. A. Moakes, C. Vareechon, G. Butt, A. Ng, K. Brock, G. Chouhan, R. C. Vincent, S. Abbondante, R. L. Williams, et al. 2018. Sustained release of decorin to the surface of the eye enables scarless corneal regeneration. NPJ Regen. Med. 3:23. doi:10.1038/s41536-018-0061-4.
- Hirano, S., E. A. Shelden, and R. R. Gilmont. 2004. HSP27 regulates fibroblast adhesion, motility, and matrix contraction. Cell Stress & Chaperones 9:29–37. doi:10.1379/1466-1268(2004)009<0029:HRFAMA>2.0.CO;2.
- Hirano, S., R. S. Rees, and R. R. Gilmont. 2002. MAP kinase pathways involving hsp27 regulate fibroblast-mediated wound contraction. J. Surg. Res. 102:77–84. doi:10.1006/jsre.2001.6315.
- Huot, J., F. Houle, D. R. Spitz, and J. Landry. 1996. HSP27 phosphorylation-mediated resistance against actin fragmentation and cell death induced by oxidative stress. Cancer Res. 56:273–79.
- Ishida, Y., H. Kubota, A. Yamamoto, et al. 2006. Type I collagen in Hsp47-null cells is aggregated in endoplasmic reticulum and deficient in N-propeptide processing and fibrillogenesis. Mol. Biol. Cell 17:2346–55. doi:10.1091/mbc.e05-11-1065.
- Ishida, Y., and K. Nagata. 2011. Hsp47 as a collagen-specific molecular chaperone. Meth. Enzymol. 499:167–82. doi:10.1016/B978-0-12-386471-0.00009-2.
- Ishikawa, Y., K. Rubin, H. P. Bächinger, and S. Kalamajski. 2018. The endoplasmic reticulum-resident collagen chaperone Hsp47 interacts with and promotes the secretion of decorin, fibromodulin, and lumican. J. Biol. Chem. 293:13707–16. doi:10.1074/jbc.RA117.000758.
- Ishikawa, Y., S. Ito, K. Nagata, et al. 2016. Intracellular mechanisms of molecular recognition and sorting for transport of large extracellular matrix molecules. Proc. Natl. Acad. Sci. U S A 113:E6036–E6044. doi:10.1073/pnas.1609571113.
- Ito, S., and K. Nagata. 2019. Roles of the endoplasmic reticulum-resident, collagen-specific molecular chaperone Hsp47 in vertebrate cells and human disease. J. Biol. Chem. 294:2133–41. doi:10.1074/jbc.TM118.002812.
- Juutilainen, J., A. Höytö, T. Kumlin, and J. Naarala. 2011. Review of possible modulation-dependent biological effects of radiofrequency fields. Bioelectromagnetics 32:511–34. doi:10.1002/bem.20652.
- Karaki, W., C. Lopez, F. Rahul, D. D. Borca-Tasciuc, and S. De. 2019. Waveform-dependent electrosurgical effects on soft hydrated tissues. J. Biomech. Eng. 141:0510031–05100314. doi:10.1115/1.4042898.
- Kotnik, T., and D. Miklavcic. 2000. Theoretical evaluation of the distributed power dissipation in biological cells exposed to electric fields. Bioelectromagnetics 21:385–94. doi:10.1002/1521-186X(200007)21:5<385::AID-BEM7>3.0.CO;2-F.
- Kovalchin, J. T., R. Wang, M. S. Wagh, J. Azoulay, BS, M. Sanders, MD, R. Y. Chandawarkar, et al. 2006. In vivo delivery of heat shock protein 70 accelerates wound healing by up-regulating macrophage-mediated phagocytosis. Wound Repair Regen. 14:129–37. doi:10.1111/j.1743-6109.2006.00102.x.
- Kumaran, B., and T. Watson. 2019. Treatment using 448kHz capacitive resistive monopolar radiofrequency improves pain and function in patients with osteoarthritis of the knee joint: A randomised controlled trial. Physiotherapy 105:98–107. doi:10.1016/j.physio.2018.07.004.
- Landry, J., and J. Huot. 1995. Modulation of actin dynamics during stress and physiological stimulation by a signaling pathway involving p38 MAP kinase and heat-shock protein 27. Biochem. Cell. Biol. 73:703–07. doi:10.1139/o95-078.
- Lavoie, J. N., G. Gingras-Breton, R. M. Tanguay, and J. Landry. 1993. Induction of chinese hamster HSP27 gene expression in mouse cells confers resistance to heat shock. HSP27 stabilization of the microfilament organization. J. Biol. Chem. 268:3420–29.
- Lavoie, J. N., H. Lambert, E. Hickey, et al. 1995. Modulation of cellular thermoresistance and actin filament stability accompanies phosphorylation-induced changes in the oligomeric structure of heat shock protein 27. Mol. Cell. Biol. 15:505–16. doi:10.1128/mcb.15.1.505.
- Li, Y., J. Li, J. Zhu, B. Sun, M. Branca, Y. Tang, W. Foster, X. Xiao, J. Huard, et al. 2007. Decorin gene transfer promotes muscle cell differentiation and muscle regeneration. Mol. Ther. 15:1616–22. doi:10.1038/sj.mt.6300250.
- Li, Y., Y. Liu, W. Xia, D. Lei, J. J. Voorhees, G. J. Fisher, et al. 2013. Age-dependent alterations of decorin glycosaminoglycans in human skin. Sci. Rep. 3:2422. doi:10.1038/srep02422.
- López-de-Celis, C., C. Hidalgo-García, A. Pérez-Bellmunt, P. Fanlo-Mazas, V. González-Rueda, J. M. Tricás-Moreno, S. Ortiz, J. Rodríguez-Sanz, et al. 2020. Thermal and non-thermal effects of capacitive-resistive electric transfer application on the Achilles tendon and musculotendinous junction of the gastrocnemius muscle: A cadaveric study. BMC Musculoskelet. Disord. 21:46. doi:10.1186/s12891-020-3072-4.
- Lutsch, G., R. Vetter, U. Offhauss, M. Wieske, H.-J. Gröne, R. Klemenz, I. Schimke, J. Stahl, R. Benndorf, et al. 1997. Abundance and location of the small heat shock proteins HSP25 and αB-Crystallin in rat and human heart. Circulation 96:3466–76. doi:10.1161/01.cir.96.10.3466.
- Ma, R., J. Chen, Z. Li, et al. 2014. Decorin accelerates the liver regeneration after partial hepatectomy in fibrotic mice. Chin. Med. 127:2679–85.
- Masago, Y., A. Hosoya, K. Kawasaki, S. Kawano, A. Nasu, J. Toguchida, K. Fujita, H. Nakamura, G. Kondoh, K. Nagata, et al. 2012. The molecular chaperone Hsp47 is essential for cartilage and endochondral bone formation. J. Cell. Sci. 125:1118–28. doi:10.1242/jcs.089748.
- Millar, N. L., and G. A. Murrell. 2012. Heat shock proteins in tendinopathy: Novel molecular regulators. Mediators Inflamm. 2012:436203. doi:10.1155/2012/436203.
- Mondardini, P., R. Tanzi, L. Verardi, et al. 1999. New methods for the treatment of traumatic muscle pathology in athletes: C.R.E.T therapy. Medicina dello Sport 52:201–13.
- Nagai, N., M. Hosokawa, S. Itohara, E. Adachi, T. Matsushita, N. Hosokawa, K. Nagata, et al. 2000. Embryonic lethality of molecular chaperone hsp47 knockout mice is associated with defects in collagen biosynthesis. J. Cell. Biol. 150:1499–506. doi:10.1083/jcb.150.6.1499.
- Nagata, K. 1998. Expression and function of heat shock protein 47: A collagen-specific molecular chaperone in the endoplasmic reticulum. Matrix Biol. 16:379–86. doi:10.1016/s0945-053x(98)90011-7.
- Neufer, P. D., and I. J. Benjamin. 1996. Differential expression of B-crystallin and Hsp27 in skeletal muscle during continuous contractile activity. Relationship to myogenic regulatory factors. J. Biol. Chem. 271:24089–95. doi:10.1074/jbc.271.39.24089.
- Pang, X., N. Dong, and Z. Zheng. 2020. Small leucine-rich proteoglycans in skin wound healing. Front Pharmacol. 10:1649. doi:10.3389/fphar.2019.01649.
- Parolo, E., and M. P. Honesta. 1998. HCR 900. Hyperthermia by capacitive and resistive energy transfer in the treatment of acute and chronic muscular-skeletal injuries. La Riabilitazione 31:81–83.
- Rawe, I. M., and T. C. Vlahovic. 2012. The use of a portable, wearable form of pulsed radio frequency electromagnetic energy device for the healing of recalcitrant ulcers: A case report. Int. Wound J. 9:253–58. doi:10.1111/j.1742-481X.2011.00853.x.
- Reed, C. C., and R. V. Iozzo. 2002. The role of decorin in collagen fibrillogenesis and skin homeostasis. Glycoconj. J. 19:249–55. doi:10.1023/A:1025383913444.
- Stasinopoulos, D., A. Constantinou, and D. Lamnisos. 2020. 448 khz Capacitive resistive monopolar radiofrequency in patients with rotator cuff tendinopathy. A pilot study. Acta Scientific Orthopaedics 3:16–20. doi:10.31080/ASOR.2020.03.0156.
- Takahashi, K. S. T., S. Hirabayashi, S. Hirabayashi, S. Hirabayashi, N. Tsuzuki, L. Zhong-Shi, et al. 1999. Clinical effects of capacitive electric transfer hyperthermia therapy for lumbago. J. Phys. Ther. Sci. 11:45–51. doi:10.1589/jpts.11.45.
- Tiedemann, K., T. Larsson, D. Heinegård, and A. Malmström. 2001. The glucuronyl C5-epimerase activity is the limiting factor in the dermatan sulfate biosynthesis. Arch. Biochem. Biophys. 391:65–71. doi:10.1006/abbi.2001.2376.
- Tukaj, S., and M. Kaminski. 2019. Heat shock proteins in the therapy of autoimmune diseases: Too simple to be true? Cell. Stress Chaperones 24:475–79. doi:10.1007/s12192-019-01000-3.
- Vidyasagar, A., N. A. Wilson, and A. Djamali. 2012. Heat shock protein 27 (HSP27): Biomarker of disease and therapeutic target. Fibrogenesis Tissue Repair 5:7. doi:10.1186/1755-1536-5-7.
- Wang, Z. L., T. Inokuchi, H. Ikeda, et al. 2002. Collagen-binding heat shock protein HSP47 expression during healing of fetal skin wounds. Int. J. Oral Maxillofac. Surg. 31:179–84. doi:10.1054/ijom.2001.0191.
- Yokota, Y., T. Sonoda, Y. Tashiro, Y. Suzuki, Y. Kajiwara, H. Zeidan, Y. Nakayama, M. Kawagoe, K. Shimoura, M. Tatsumi, et al. 2018. Effect of capacitive and resistive electric transfer on changes in muscle flexibility and lumbopelvic alignment after fatiguing exercise. J. Phys. Ther. Sci. 30:719–25. doi:10.1589/jpts.30.719.