Abstract
The ability of living cells to exert physical forces upon their surrounding is a necessary prerequisite for diverse biological processes, such as local cellular migrations in wound healing to metastatic-invasion of cancer. How forces are coopted in metastasis has remained unclear, however, because the mechanical interplay between cancer cells and the various stromal components has not been experimentally accessible. Current dogma implicates inflammation in these mechanical processes. Using Fourier transform traction microscopy, we measured the force-generating capacity of human breast cancer cells occupying a spectrum of invasiveness as well as basal and inducible COX-2 expression (MCF-7<SUM-149<MDA-MB-231). Compared with non-invasive MCF-7 and moderately-invasive SUM-149, poorly-differentiated MDA-MB-231 cells showed increased cellular dispersion on collagen matrix that was accompanied by emergent distribution of contractile stresses at the interface between the adherent cell and its substrate, defined herein as the traction field. In metastatic MDA-MB-231 cells, the local tractions were precisely tuned to the surrounding matrix rigidity in a physiologic range with the concomitant expression of mechanosensitive integrin β1. These discrete responses at the single-cell resolution were correlated with PGE2 secretion and were ablated by shRNA-mediated knockdown of COX-2. Both COX-2-silenced and COX-2-expressing cells expressed EP2 and EP4 receptors, but not EP1 and EP3. Exogenous addition of PGE2 increased cell tractions and stiffened the underlying cytoskeletal network. To our knowledge this is the first report linking the expression of COX-2 with mechanotransduction of human breast cancer cells, and the regulation of COX-2-PGE2-EP signaling with physical properties of the tumor microenvironment. Drug treatments aimed at reducing this mechanical interplay may have therapeutic potential in the treatment of breast cancer.
Abbreviations
COX-2 | = | cyclooxygenase-2; CSK, cytoskeleton; ECM, extracellular matrix; FACS, fluorescence-activated cell sorting; FTTM, Fourier transform traction microscopy; MTC, magnetic twisting cytometry; PGE2, prostaglandin E2; PLA2, phospholipase A2; RGD, arginine-glycine-aspartic acid |
Introduction
Breast cancer is the most common malignancy diagnosed in womenCitation1 and is the second leading cause of cancer-related death among women in the United States.Citation2 It is estimated that up to 10% of breast cancer in Western countries is due to genetic predisposition with many extrinsic factors contributing to the disease progression, presumably via genetic and/or epigenetic changes.Citation3 The prognosis of metastatic breast cancer remains dismal, however, and current surgical and medical treatments are entirely ineffective in eradicating metastatic spread of primary tumors–the major cause of morbidity and mortality in cancer patients. There is an unmet need for understanding the mechanisms governing metastasis and developing new effective treatments for breast cancer.
A classical view of tumor metastasis begins with the acquisition of traits that allow malignant cells to escape from the primary tumor, to invade the local parenchyma constituting the extracellular matrix (ECM), and to enter the circulation.Citation4,5 Metastasis then progresses with the transportation of cancer cells via blood circulation to distant target organs, whereupon individual cancer cells adhere, spread and migrate through the ECM in the surrounding tissue forming secondary tumors.Citation4,5 In this metastatic-invasion framework, the ability of an individual cancer cell to invade its local microenvironment, to evade shear stresses imposed by circulation, and to migrate through the surrounding ECM all comprise mechanical properties.Citation6 Current dogma implicates inflammation within the tumor microenvironment in these mechanical processes.Citation7,8 However, the mechanical interplay between cancer cells and their physical tissue microenvironment is poorly understood. Further, it remains unclear how an individual cancer cell senses, integrates and initiates its network of mechanical forces during cellular metastasis.
Cyclooxygenase-2 (COX-2), the inducible isoform of prostaglandin (PG) H2 synthase, is a key mediator of inflammation and converts PLA2-mobilized arachidonic acid into biologically active lipids.Citation9 Expression of COX-2 is elevated in various human malignancies,Citation10-13 and COX-2 catalyzed PGE2 has been implicated in angiogenesisCitation14 and metastasis of various cancers, including that of the breast.Citation15,16 We have previously shown that highly metastatic MDA-MB-231 human breast cancer cells stably expressing a COX-2-specific short hairpin RNA interference molecule exhibit marked reduction in mRNA expression of the classical invasion-related matrix metalloproteinases.Citation17 Corroborating these changes, cells lacking COX-2 showed decreases in their ability to degrade and infiltrate reconstituted ECM in vitro.Citation17,18 Further, the loss of COX-2 significantly delayed tumor onset as well as inhibited extrapulmonary metastasis in vivo.Citation18 To what extent this loss-of-function of metastatic-invasion in human breast cancer cells is attributable to the regulation of cellular mechanics remains unexplored, however. Here we report that COX-2 expression and activity are precisely tuned to the local rigidity of the collagen matrix and, in the absence of inflammatory milieu, regulate cell tractions in a feed-forward mechanism involving the COX-2-PGE2-EP signaling axis. To our knowledge this is the first report linking the expression of COX-2 with mechanotransduction of human breast cancer cells.
Results
Force-generating capacity of individual human breast cancer cells with different COX-2 expression and invasiveness. We first interrogated the force-generating capacity of human breast cancer cell lines occupying a series of invasiveness, including the classical luminal-like, non-invasive MCF-7 and basal-like, moderately invasive SUM-149 and highly invasive MDA-MB-231. We focused on these cell lines because they exhibit low-to-high expression of basal and inducible COX-2 (MCF-7<SUM-149<MDA-MB-231).Citation18,19 This was undertaken using Fourier transform traction microscopy that provides for measuring individual cell mechanical properties in a precise manner, in cells growing on matrices with varying degrees of stiffness across physiological extremes.Citation20-22 For this study, we used collagen-coated, elastic gel substrate (∼8 kPa) that recapitulates the elastic modulus of human breast tissues ranging from fibroadenoma and low-grade invasive ductal carcinoma (IDC).Citation23
On this moderately stiff matrix in vitro, MCF-7 and SUM-149 cells showed a more rounded morphology in comparison to MDA-MB-231 cells: MDA-MB-231 cells were elongated and polarized, exhibiting a characteristic mesenchymal phenotype. As shown in , highly invasive MDA-MB-231 cells were bigger in size and exerted upon their surrounding greater tractions than moderately invasive SUM-149 and non-invasive MCF-7 cells. Strikingly, concordant with a ranked-order based on metastatic-invasion potential,Citation19,24 the net contractile moment, which is a scalar measure of the cell's contractile strength,Citation25 was ∼2.1-fold and ∼2.9-fold higher in MDA-MB-231 cells than the respective SUM-149 (P = 0 .0069, Wilcoxon Scores) and MCF-7 cells (P = 0 .0004, Wilcoxon Scores) (). Although SUM-149 cells trended toward increases in projected cell area and net contractile moment than MCF-7 cells, there were no significant differences between the 2 cell lines in terms of cell size (; P = 0 .0702, Wilcoxon Scores) and contractile strength (; P = 0 .2458, Wilcoxon Scores). These results suggest a close correlation between COX-2 expression, force generation, and the metastatic abilities of human breast cancer cells.
Figure 1. Association between invasiveness and COX-2 status on the generation of traction force in human breast cancer cells. (A) Representative phase contrast and traction map images of MCF-7, SUM-149 and MDA-MB-231 cells. White lines show the cell boundary, colors show the magnitude of the tractions in Pa (see color scale), and arrows show the direction and relative magnitude of the tractions. Scale bar, 50 μm. The projected cell area (B) and computed net contractile moments (C) of individual breast cancer cells (MCF-7, SUM-149, and MDA-MB-231). The line bars represent the median for each group (n = 12 -17 cells for each group).
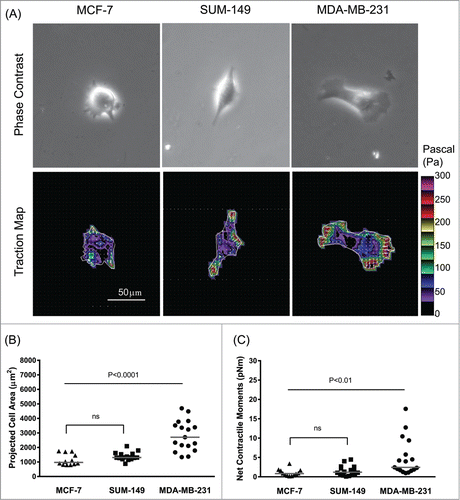
Role for COX-2 in cell tractions? COX-2 and its secondary lipid byproducts are critical determinants of breast cancer invasion and metastasis.Citation15,16,26 We have previously shown that poorly differentiated MDA-MB-231 cells stably expressing COX-2 shRNA showed marked attenuation in their abilities to secrete secondary lipid mediator products of the COX-2 reaction (i.e. PGE2) in response to the proinflammatory cytokine interleukin (IL)-1β. Citation17 We performed extensive quality control analyses of individual clones expressing a short hairpin RNA complementary to COX-2 mRNA (data not shown) and selected clone 2 which could not be induced by IL-1β to secrete PGE2 for detailed studies.Citation18 Cell ensembles of clone 2 were relatively more packed with their neighboring cells (Supplementary ) and showed restricted cellular motions than that of parental MDA-MB-231 counterpart (Supplementary Video 1). As denoted by colors in Supplementary Figure 1, cells lacking COX-2 exhibited ∼37% slower average speed (0.80 μm/min vs. 1.27 μm/min). Furthermore, as probed by spontaneous motions of microbeads functionalized to the cytoskeleton (CSK) through cell surface integrin receptors,Citation27,28 COX-2-silenced cells exhibited in turn marked decreases in the rate of cytoskeletal remodeling than COX-2-expressing MDA-MB-231 cells (Supplementary Fig. 2). Corroborating these changes in the mechanical properties, COX-2-silenced cells expressed appreciable decreases in the levels of transcripts involved in the cytoskeletal regulation pathways, including ras homolog gene family U and J (RhoU and RhoJ), Rho GTPase activating protein 24 (RhoGAP), and CDC42 effector protein 5 (CDC42EP5) (Supplementary Fig. 2). When we measured tractions within the cell monolayer using Monolayer Traction Microscopy,Citation29 root-mean-square (RMS) traction in cell ensemble of clone 2 trended toward decreases compared to that of parental MDA-MB-231 counterpart; however, the decrease was not statistically significant (data not shown). Herein, we performed the complementary experiments at the level of individually dispersed cells using Fourier transform traction microscopy.
At the single-cell level, MDA-MB-231 clone 2 stably expressing COX-2 shRNA exhibited marked reduction in the dispersion of cell size on collagen matrix than the COX-2-expressing parental counterpart (). Compared with parental MDA-MB-231 cells, COX-2-silenced cells showed ∼35% reduction in projected cell area (1364.47 ± 78.51 μm2 COX-2-silenced vs. 2903.37 ± 225.50 μm2 COX-2-expressing, Mean ± SE) while showing ∼60% reduction in net contractile moment (1.20 ± 0.19 pNm COX-2-silenced vs. 3.58 ± 0.13 pNm COX-2-expressing, Geometric Mean ± SE). These striking differences in cell size and contractile strength were persistent and long-lived across physiologic range of matrix rigidity–i.e., mimicking the stiffness of human breast tissues of normal fat to intermediate-grade IDC ().Citation23 Interestingly, with increasing matrix rigidity, MDA-MB-231 cells showed progressive increases in cell spreading () and net contractile moments (). In addition, MDA-MB-231 cells showed increased expression of mechanosensitive integrin β1, but not β3, with increasing matrix rigidity (; Supplementary Fig. 3). These discrete cellular responses to matrix rigidity were absent in COX-2-silenced MDA-MB-231 cells. These results, taken together, support the conclusion that expression and/or activity of COX-2 are critical for the mechanotransduction of human breast cancer cells and suggest that physical changes in the tumor microenvironment may affect metastatic-invasion of human breast cancer via mechanisms involving COX-2 and its lipid byproducts.
Figure 2. Effect of COX-2-silencing on the generation of traction force in invasive MDA-MB-231 cells. (A) Representative traction maps of MDA-MB-231 (COX-2-expressing vs. COX-2-silenced) cells. The projected cell area (B) and computed net contractile moments (C) of COX-2-expressing and COX-2-silenced cells (n = 31 cells for each group). Herein, projected cell area is presented as Mean + SE, and net contractile moment is presented as Geometric Mean ± SE.
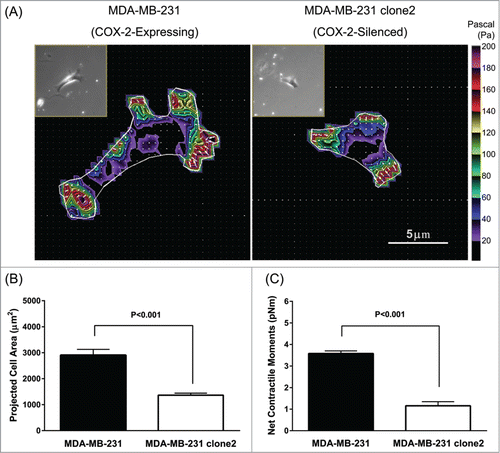
Figure 3. Effects of COX-2-silencing on the mechanobiology of invasive MDA-MB-231 cells. The projected cell area (A) and computed net contractile moments (B) of MDA-MB-231 cells (COX-2-expressing vs. COX-2-silenced) measured on elastic substrate with varying stiffness (1 kPa, n = 32 -37; 8 kPa, n = 31 ; 20 kPa, n = 18 -25 for each group). Expression levels of integrin β1 (C) and β3 (D) in MDA-MB-231 cells (COX-2-expressing vs. COX-2-silenced). Data are presented as Mean + SE (n = 3 experiments).
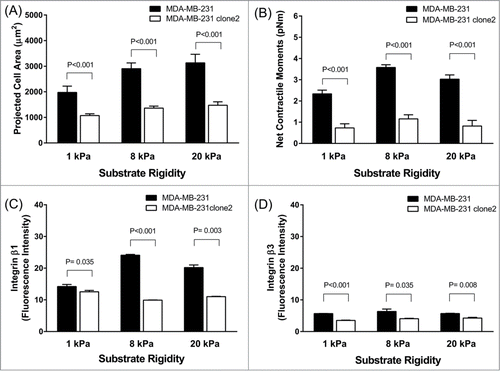
Propagation of cellular tractions is mediated by a feed-forward mechanism involving COX-2-PGE2 axis. The induction of COX-2 expression stimulates the biosynthesis and secretion of PGE2.Citation9 In COX-2-expressing, parental MDA-MB-231 cells, we found progressive and appreciable increases in the levels of PGE2 production with increasing matrix rigidity (). These cellular responses were entirely ablated in MDA-MB-231 clone 2 cells stably expressing COX-2 shRNA. PGE2 acts through G protein-coupled receptors (EP1–4) and initiates a complex downstream signaling that regulates, in turn, cell proliferation, migration and invasion.Citation16,30 Both parental and COX-2-silenced MDA-MB-231 cells showed transcript levels of EP2 and EP4, but not EP1 and EP3 (Supplementary Fig. 4). Whereas exogenous addition of PGE2 did not influence the dispersion of human breast cancer cells on collagen matrix (data not shown), it appreciably increased their ability to exert tractions (). In order to further validate this unique mechanical responsiveness to PGE2, we applied forced motions of ferrimagnetic microbeads functionalized to the CSK using Magnetic Twisting Cytometry ( and Supplementary Fig. 5).Citation31-33 As expected, PGE2 caused time- and dose-dependent decreases in the stiffness of isolated human airway smooth muscle cells (Supplementary Fig. 5)Citation34. In contrast, exogenous addition of PGE2, both acutely and chronically, stiffened the underlying cytoskeletal network of metastatic human breast cancer cells, including moderately-invasive SUM-149 cells ( and Supplementary Fig. 5). PGE2 had no effect on the stiffness of non-metastatic MCF-7 cells, however (). Taken together, these findings show that the physical nature of the tumor microenvironment, independent of immune inflammatory responses, may also impact COX-2 expression and/or activity in human breast cancer cells and that propagation of physical forces and cell metastasis may be mediated by COX-2-driven PGE2 in a feed forward manner.
Figure 4. A feed-forward mechanism involving COX-2-PGE2-EP signaling on the mechanotransduction of human breast cancer cells. (A) Effects of matrix rigidity on COX-2 activity. MDA-MB-231 (COX-2-expressing and COX-2-silenced) cells were cultured on elastic substrates with varying stiffness, and the secreted levels of PGE2 in the culture media were measured after 24 h. Data are presented as Mean + SE (n = 3 experiments); ANOVA test (P = parental ; C = clone2 ). (B) COX-2-expressing and COX-2-silenced cells were seeded onto an elastic substrate with Young's modulus of 1 kPa, and treated for 24 h with or without increasing concentrations of PGE2. On the one hand, cell spreading area increased progressively with increasing concentrations of PGE2 (50 nM < 100 nM < 500 nM < 1 μM). On the other hand, while cell traction force increased with PGE2 at 50 nM and 100 nM, PGE2 concentrations above 100 nM led to decreases in cell traction force (data not shown). Here we report the effects of 50nM PGE2 on cell traction force. Data are presented as Geometric Mean + SE (COX-2-expressing cells, n = 19–21; COX-2-silenced cells, n = 14–22). (C) Effects of PGE2 on cytoskeletal stiffness of human breast cancer cells. For this MTC study, cells were plated at 30,000 cells/cm2 on plastic wells previously coated with collagen type I for 24 h in media alone or media supplemented with 50–100nM PGE2. Data are presented as Geometric Mean + SE (MCF-7, n = 183 -200 cells; SUM-149, n = 296 -310 cells; MDA-MB-231, n = 171 -397 cells).
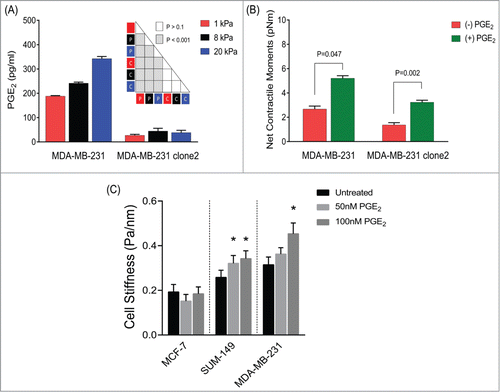
Discussion
Mechanotransduction is the most pervasive element of the metastatic cascade but is incompletely understood, especially within the context of the wound-like inflammatory environments that exist within tumors. Most solid tumors, including breast cancers, exhibit inflammatory properties characterized by increased levels of prostaglandins and other proinflammatory cytokines that are secreted by tumor cells, stromal cells, and specialized immune cells.Citation35 Eicosanoids formed by the action of COX-2 on arachidonic acid have been shown to impact cell motility, invasion, vascular characteristics and metastatic dissemination.Citation36-38 A major product of the COX-2-catalyzed reaction is prostaglandin E2 (PGE2), an inflammatory mediator that participates in various biological processes,Citation39 and plays multiple roles in cancer aggressiveness.Citation40,41 Here for the first time we have identified a role for COX-2 expression, signaling and function in the induction of physical forces in human breast cancer. We also identified, for the first time, the effect of matrix rigidity on the propagation of these forces, demonstrating the role of the physical microenvironment on the inflammatory response of cancer cells.
We observed a robust association between invasiveness and force-generating capacity of human breast cancer cells. The most invasive and metastatic MDA-MB-231 cells displayed the highest net contractile moment–a scalar measure of a cell's contractile strength (). The association between invasiveness and contractile strength is consistent with 2 recent studies that reported increased tractions in more invasive breast cancer cells.Citation42,43 Increased local cell tractions–the force exerted upon its surrounding area–may provide a biophysical marker of the cell's ability to adhere, spread and migrate through the ECM, as well as invade and metastasize through the endothelial cell barrier during intravasation and extravasation. The three cell lines used in this study also expressed low-to-high basal and inducible COX-2 (MCF-7<SUM-149<MDA-MB-231). Because of the different lineages of these cells we could identify an association between COX-2 and cell tractions but not a direct role of COX-2 in driving these parameters. Further validation was provided by the marked reduction in cell spreading and tractions observed in MDA-MB-231 clone 2 cells stably expressing COX-2 shRNA than COX-2 expressing parental counterpart cells (). MDA-MB-231 clones stably expressing COX-2 shRNA are poorly invasive and lack the ability to metastasize.Citation17,18
In order to show the loss-of-function, here we applied shRNA-mediated knockdown of COX-2 in the highly invasive and metastatic MDA-MB-231 cell line. This approach was chosen, rather than using pharmacological inhibitions, however, because COX-2 inhibitors are known to have several COX-2-independent functions and, like most drugs, have off target effects. For example, many of the celecoxib-associated effects observed in vitro and in vivo are not related to COX-2 inhibition, but to COX-2-independent actions of celecoxib.Citation44 MDA-MB-231 cells treated with celecoxib also exhibited a trend toward decreases in net contractile moment but, unlike the dramatic effect of COX-2-silencing on cell spreading, had no effect on the extent of cell spreading on the elastic substrate (Supplementary Fig. 6). These findings, taken together, suggest that COX-2-mediated induction of effector protein expression might be required to fully realize the propagation of mechanotransduction in human breast cancer cells. Toward this end, increased COX-2 can mediate the upregulation of several oncogenic pathways including proteolytic enzymes.Citation18 Interestingly, in pancreatic cancer, COX-2 has been recently identified as a novel target of the transcription regulator Yes-associated protein (YAP).Citation45 There is also increasing evidence to suggest that YAP is a mechanosensor in various cell types, suggesting that COX-2 can be induced mechanically.
Cancerous tissues typically exhibit increased ECM rigidity compared to normal tissues. Breast and other solid tumors are denser than surrounding stroma because of the increased deposition and differential remodeling of collagen fibers that surround the growing tumor.Citation46 A high density of collagen 1 fibers in the ECM has been identified as a predictor of increased metastasis.Citation47,48 High collagen density has also been observed to increase tractions by breast cancer cells.Citation43 Increased metastasis associated with high collagen density may be mediated in part by this increased tractions. Previous studies have identified the effects of ECM stiffness on migration and proliferation of glioma cells, and the effect of matrix stiffness and confinement on cell migration speed of glioma cells.Citation49-50 Here, with increasing matrix rigidity, MDA-MB-231 cells showed progressive increases of cell spreading that was accompanied by increased expression of mechanosensitive integrin β1 (). These mechanical responses to increasing matrix rigidity were absent in COX-2 silenced MDA-MB-231 cells. Our data are consistent with earlier observations that cancer cells on substrates of increasing stiffness exhibit increased tractions,Citation43 and demonstrate for the first time that expression and/or activity of COX-2 are critical in this mechanotransduction of human breast cancer cells. Further support for the role of COX-2 is evident from the increase of PGE2 secretion with increasing substrate rigidity in MDA-MB-231 cells, but not in COX-2 silenced cells (). Exogenous addition of PGE2 to these COX-2 silenced cells resulted in a partial recovery of tractions whereas further increased responses are noted in their parental counterpart, MDA-MB-231 cells (). The increase of PGE2 secretion with increasing substrate rigidity suggests mechanical induction of COX-2 in these cells.
Using MTC, we further validated the effects of PGE2 across breast cancer cell lines: non-invasive and non-metastatic MCF-7, moderately invasive and metastatic SUM-149 cells, and most invasive and metastatic MDA-MB-231 cells ( and Supplementary Fig. 5). Consistent with the ranked order differences in the net contractile moments measured by Fourier transform traction microscopy (), we found appreciable ranked order differences in the basal cytoskeletal stiffness across breast cancer cells (MCF-7<SUM-149<MDA-MB-231). Compared with MCF-7 cells, both SUM-149 and MDA-MB-231 cells exhibited significantly higher cytoskeletal stiffness (). There were no statistical differences between SUM-149 and MDA-MB-231 cell stiffness, however. More strikingly, PGE2 treatment increased the stiffness of moderately invasive SUM-149 and highly invasive MDA-MB-231 cells whereas it had no effect on non-invasive MCF-7 cells. Taken together, our results using two independent single-cell analyses confirmed the unique physical properties of metastatic human breast cancer cells, and further validate the role for COX-2-generated PGE2 on the mechanotransduction and progression of cell metastasis. In conclusion, our findings provide a new mechanistic framework in which changes in the physical properties of the tumor microenvironment may affect metastatic-invasion of human breast cancer via mechanisms involving COX-2 and its lipid byproducts. As such, drug treatments aimed at reducing this mechanical interplay may have therapeutic potential in the treatment of breast cancer.
Materials and Methods
Cell lines and culture conditions. All human breast cancer cell lines, with the exception of SUM-149, were cultured with DMEM (Sigma-Aldrich, St. Louis, MO) supplemented with 10% fetal bovine serum (Gibco BRL, Grand Island, NY). SUM-149 cell line was maintained in RPMI 1640 media (Sigma-Aldrich) supplemented with 8.25% fetal bovine serum, 0.1mM HEPES (Sigma-Aldrich) and 10 mg/ml pancreatic insulin (Sigma-Aldrich). Cells were maintained at 37oC in humidified air containing 5% CO2.
Preparation of elastic matrix. As previously described,Citation50 a mixture of acrylamide (5–10%) and bis-acrylamide (0.03–0.3%) was used to vary the rigidity of elastic gel blocks in the physiological range.Citation23 For elastic gels used in the detection of COX-2 activity and mechanosensitive integrins, gel blocks were fashion onto individual 35 mm glass-bottom wells with 20 mm inner glass diameter (P35G-0–20-C; MatTek Inc., Ashland, MA). For gels used for gauging cell traction forces, fluorescent microbeads (0.2 μm in diameter, Molecular Probes, Eugene, OR) were added to the mixture of acrylamide and bis-acrylamide and were fashioned onto individual 35 mm glass-bottom wells with 14 mm inner glass diameter (P35G-0–14-C). All gel blocks were coated with collagen type I (0.2 mg/ml) using a photo-activating cross-linker sulfo-SANPAH (Pierce, Rockford, IL).
Fourier transform traction microscopy (FTTM). The contractile stress arising at the interface between each adherent cell and its substrate was measured with traction microscopy.Citation20-22,25 Cells were plated sparsely on elastic gel blocks, and allowed to adhere and stabilize for 24 h. For each adherent cell, images of fluorescent microbeads embedded near the gel apical surface were taken at different times; the fluorescent image of the same region of the gel after cell detachment with trypsin was used as the reference (traction-free) image. The displacement field between a pair of images was then obtained by identifying the coordinates of the peak of the cross-correlation function.Citation25 From the displacement field and the known elastic properties of the gel (Young's Modulus, 1 kPa to 20 kPa and Poisson's ratio 0.48), the traction field was computed using both constrained and unconstrained Fourier transform traction cytometry as described previously.Citation25 The computed traction field was used to obtain net contractile moment, which is a scalar measure of the cell's contractile strength expressed in units of pico-Newton meters (pNm).
Magnetic Twisting Cytometry (MTC). To quantify material properties of the adherent human breast cancer cells, we used MTC as described by us in detail elsewhere.Citation31-33 In brief, an RGD-coated ferrimagnetic microbead (4.5 μm in diameter) anchored to the CSK through cell surface integrin receptors was magnetized horizontally and then twisted in a vertically aligned homogenous magnetic field that varied sinusoidally in time; measurements were performed at a single frequency of 0.75 Hz or oscillatory frequencies between 10–1 and 103 Hz. The sinusoidal twisting field causes both a rotation and a pivoting displacement of the bead (Supplementary Fig. 6). As the bead moves, the cell develops internal stresses which in turn resist bead motions.Citation51 Lateral bead displacements in response to the resulting oscillatory torque were detected via a CCD camera (Orca II-ER, Hamamatsu, Japan), and with an accuracy of 5 nm using an intensity-weighted center-of-mass algorithm.Citation51 The ratio of specific applied torque to lateral bead displacements was computed and expressed as the cell stiffness in units of Pascals (Pa) per nanometer.
Detection of COX-2 activity. COX-2 activity in human breast cancer cells was assessed by measurement of secreted PGE2. For this study, we plated 1 × 106 cells per elastic gel block with varying rigidity (1 kPa to 20 kPa) for 24 h. The PGE2 concentration in the supernatants was determined using a commercial EIA kit (DetectX Prostaglandin E2 High Sensitivity Immunoassay Kit, Arbor Assays, Ann Arbor, MI) following the manufacturer's instructions. The absorbance in the samples was measured at 490 and 540 nm with a microplate reader (Molecular Devices, Sunnyvale, CA). The concentration of PGE2 was calculated from a standard curve derived using recombinant proteins. All samples were assayed in triplicate.
Fluorescence-activated cell-sorting (FACS) analysis. For the adherent cells on the respective elastic gel block (as above in COX-2 assay), we also quantified the cell surface expression levels of integrin β1 and β3 using FACS. In brief, cells were detached using cell dissociation buffer (Gibco Life Technologies, Grand Island, NY) and suspended in staining buffer (RPMI 1640 media, 2% FBS). Equal amount of cells in 100 μl volume was transferred into 12 × 75 mm polypropylene FACS tubes and incubated with 1 μg/ml of FcR blocking antibody (Santa Cruz Biotechnology, Santa Cruz, CA) for 10 min at room temperature. After blocking, cells were incubated with FITC-conjugated antibody against CD29 for integrin β1 (Millipore, San Diego, CA) and CD61 for integrin β3 (Millipore) for 30 min at room temperature in the dark. Cells were then washed twice with staining buffer and resuspended in 500 μl staining buffer for analysis. The antibody staining was quantified with a FACSCalibur flow cytometer (BD Biosciences Immunocytometry Systems, San Jose, CA) and data from 10,000 events were collected for further analysis. The three independent data from each group were analyzed using Cell Quest Pro (BD Biosciences Immunocytometry Systems) to determine the mean fluorescence intensity and the standard deviation.
Statistical analysis. Unless otherwise stated, we used Student's t-test and the Analysis of Variance (ANOVA) with adjusting for multiple comparisons by applying the Bonferroni's methods. All analyses were performed in SAS V.9.2 (SAS Institute Inc., Cary, NC), and the 2-sided P-values less than 0.05 were considered significant.
Disclosure of Potential Conflicts of Interest
No potential conflicts of interest were disclosed.
1003004_Supplementary_Materials.zip
Download Zip (3.4 MB)Supplemental Material
Supplemental data for this article can be accessed on the publisher's website.
Additional information
Funding
References
- DeSantis C, Ma J, Bryan L, Jemal A. Breast cancer statistics, 2013. CA Cancer J Clin 2014; 64:52-62; PMID:24114568.
- Jemal A, Ward E, Thun MJ. Recent trends in breast cancer incidence rates by age and tumor characteristics among US women. Breast Cancer Res 2007; 9:R28; PMID:17477859; http://dx.doi.org/10.1186/bcr1672.
- McPherson K, Steel CM, Dixon JM. ABC of breast diseases. Breast cancer-epidemiology, risk factors, and genetics. Bmj 2000; 321:624-8; PMID:10977847; http://dx.doi.org/10.1136/bmj.321.7261.624.
- Chambers AF, Groom AC, MacDonald IC. Dissemination and growth of cancer cells in metastatic sites. Nat Rev Cancer 2002; 2:563-72; PMID:12154349; http://dx.doi.org/10.1038/nrc865.
- Fidler IJ. The pathogenesis of cancer metastasis: the 'seed and soil' hypothesis revisited. Nat Rev Cancer 2003; 3:453-8; PMID:12778135; http://dx.doi.org/10.1038/nrc1098.
- Quail DF, Joyce JA. Microenvironmental regulation of tumor progression and metastasis. Nature Med 2013; 19:1423-37; PMID:24202395; http://dx.doi.org/10.1038/nm.3394.
- Balkwill F, Mantovani A. Inflammation and cancer: back to Virchow? Lancet 2001; 357:539-45; PMID:11229684; http://dx.doi.org/10.1016/S0140-6736(00)04046-0.
- Hagemann T, Balkwill F, Lawrence T. Inflammation and cancer: a double-edged sword. Cancer Cell 2007; 12:300-1; PMID:17936555; http://dx.doi.org/10.1016/j.ccr.2007.10.005.
- Garavito RM, DeWitt DL. The cyclooxygenase isoforms: structural insights into the conversion of arachidonic acid to prostaglandins. Biochim Biophys Acta 1999; 1441:278-87; PMID:10570255; http://dx.doi.org/10.1016/S1388-1981(99)00147-X.
- Achiwa H, Yatabe Y, Hida T, Kuroishi T, Kozaki K, Nakamura S, Ogawa M, Sugiura T, Mitsudomi T, Takahashi T. Prognostic significance of elevated cyclooxygenase 2 expression in primary, resected lung adenocarcinomas. Clin Cancer Res 1999; 5:1001-5; PMID:10353732.
- Buskens CJ, Van Rees BP, Sivula A, Reitsma JB, Haglund C, Bosma PJ, Offerhaus GJ, Van Lanschot JJ, Ristimaki A. Prognostic significance of elevated cyclooxygenase 2 expression in patients with adenocarcinoma of the esophagus. Gastroenterology 2002; 122:1800-7; PMID:12055587; http://dx.doi.org/10.1053/gast.2002.33580.
- Gupta RA, Dubois RN. Colorectal cancer prevention and treatment by inhibition of cyclooxygenase-2. Nat Rev Cancer 2001; 1:11-21; PMID:11900248; http://dx.doi.org/10.1038/35094017.
- Ristimaki A, Sivula A, Lundin J, Lundin M, Salminen T, Haglund C, Joensuu H, Isola J. Prognostic significance of elevated cyclooxygenase-2 expression in breast cancer. Cancer Res 2002; 62:632-5; PMID:11830510.
- Wang D, DuBois RN. Cyclooxygenase 2-derived prostaglandin E2 regulates the angiogenic switch. Proc Natl Acad Sci U S A 2004; 101:415-6; PMID:14707264; http://dx.doi.org/10.1073/pnas.0307640100.
- Wang D, Dubois RN. Eicosanoids and cancer. Nat Rev Cancer 2010; 10:181-93; PMID:20168319; http://dx.doi.org/10.1038/nrc2809.
- Chang SH, Liu CH, Conway R, Han DK, Nithipatikom K, Trifan OC, Lane TF, Hla T. Role of prostaglandin E2-dependent angiogenic switch in cyclooxygenase 2-induced breast cancer progression. Proc Natl Acad Sci U S A 2004; 101:591-6; PMID:14688410; http://dx.doi.org/10.1073/pnas.2535911100.
- Shah T, Stasinopoulos I, Wildes F, Kakkad S, Artemov D, Bhujwalla ZM. Noninvasive imaging identifies new roles for cyclooxygenase-2 in choline and lipid metabolism of human breast cancer cells. NMR Biomed 2012; 25:746-54; PMID:21953546; http://dx.doi.org/10.1002/nbm.1789.
- Stasinopoulos I, O'Brien DR, Wildes F, Glunde K, Bhujwalla ZM. Silencing of cyclooxygenase-2 inhibits metastasis and delays tumor onset of poorly differentiated metastatic breast cancer cells. Mol Cancer Res 2007; 5:435-42; PMID:17510310; http://dx.doi.org/10.1158/1541-7786.MCR-07-0010.
- Keller PJ, Lin AF, Arendt LM, Klebba I, Jones AD, Rudnick JA, DiMeo TA, Gilmore H, Jefferson DM, Graham RA, et al. Mapping the cellular and molecular heterogeneity of normal and malignant breast tissues and cultured cell lines. Breast Cancer Res 2010; 12:R87; PMID:20964822; http://dx.doi.org/10.1186/bcr2755.
- An SS, Kim J, Ahn K, Trepat X, Drake KJ, Kumar S, Ling G, Purington C, Rangasamy T, Kensler TW, et al. Cell stiffness, contractile stress and the role of extracellular matrix. Biochem Biophys Res Commun 2009; 382:697-703; PMID:19327344; http://dx.doi.org/10.1016/j.bbrc.2009.03.118.
- Garzon-Muvdi T, Schiapparelli P, ap Rhys C, Guerrero-Cazares H, Smith C, Kim DH, Kone L, Farber H, Lee DY, An SS, et al. Regulation of brain tumor dispersal by NKCC1 through a novel role in focal adhesion regulation. PLoS Biol 2012; 10:e1001320; PMID:22570591; http://dx.doi.org/10.1371/journal.pbio.1001320.
- Wang N, Tolic-Norrelykke IM, Chen J, Mijailovich SM, Butler JP, Fredberg JJ, Stamenovic D. Cell prestress. I. Stiffness and prestress are closely associated in adherent contractile cells. Am J Physiol Cell Physiol 2002; 282:C606-16; PMID:11832346; http://dx.doi.org/10.1152/ajpcell.00269.2001.
- Samani A, Zubovits J, Plewes D. Elastic moduli of normal and pathological human breast tissues: an inversion-technique-based investigation of 169 samples. Phys Med Biol 2007; 52:1565-76; PMID:17327649; http://dx.doi.org/10.1088/0031-9155/52/6/002.
- Hoffmeyer MR, Wall KM, Dharmawardhane SF. In vitro analysis of the invasive phenotype of SUM 149, an inflammatory breast cancer cell line. Cancer Cell Int 2005; 5:11; PMID:15857504; http://dx.doi.org/10.1186/1475-2867-5-11.
- Butler JP, Tolic-Norrelykke IM, Fabry B, Fredberg JJ. Traction fields, moments, and strain energy that cells exert on their surroundings. Am J Physiol Cell Physiol 2002; 282:C595-605; PMID:11832345; http://dx.doi.org/10.1152/ajpcell.00270.2001.
- Singh B, Berry JA, Shoher A, Ramakrishnan V, Lucci A. COX-2 overexpression increases motility and invasion of breast cancer cells. Int J Oncol 2005; 26:1393-9; PMID:15809733.
- Bursac P, Fabry B, Trepat X, Lenormand G, Butler JP, Wang N, Fredberg JJ, An SS. Cytoskeleton dynamics: fluctuations within the network. Biochem Biophys Res Commun 2007; 355:324-30; PMID:17303084; http://dx.doi.org/10.1016/j.bbrc.2007.01.191.
- Bursac P, Lenormand G, Fabry B, Oliver M, Weitz DA, Viasnoff V, Butler JP, Fredberg JJ. Cytoskeletal remodelling and slow dynamics in the living cell. Nat Mater 2005; 4:557-61; PMID:15937489; http://dx.doi.org/10.1038/nmat1404.
- Angelini TE, Hannezo E, Trepat X, Fredberg JJ, Weitz DA. Cell migration driven by cooperative substrate deformation patterns. Phys Rev Lett 2010; 104:168104; PMID:20482085; http://dx.doi.org/10.1103/PhysRevLett.104.168104.
- Abrahao AC, Castilho RM, Squarize CH, Molinolo AA, dos Santos-Pinto D, Jr., Gutkind JS. A role for COX2-derived PGE2 and PGE2-receptor subtypes in head and neck squamous carcinoma cell proliferation. Oral Oncol 2010; 46:880-7; PMID:20951077; http://dx.doi.org/10.1016/j.oraloncology.2010.09.005.
- Trepat X, Deng L, An SS, Navajas D, Tschumperlin DJ, Gerthoffer WT, Butler JP, Fredberg JJ. Universal physical responses to stretch in the living cell. Nature 2007; 447:592-595; PMID:17538621; http://dx.doi.org/10.1038/nature05824.
- Deshpande DA, Wang WCH, McIlmoyle EL, Robinett KS, Schillinger RM, An SS, Sham JSK, Liggett SB. Bitter taste receptors on airway smooth muscle bronchodilate by localized calcium signaling and reverse obstruction. Nat Med 2010; 16:1299-1304; PMID:20972434; http://dx.doi.org/10.1038/nm.2237.
- Kim JJ, Yin B, Christudass CS, Terada N, Rajagopalan K, Fabry B, Lee DY, Shiraishi T, Getzenberg RH, Veltri RW, et al. Acquisition of paclitaxel resistance is associated with a more aggressive and invasive phenotype in prostate cancer. J Cell Biochem 2013; 114:1286-1293; PMID:23192682; http://dx.doi.org/10.1002/jcb.24464.
- Lawrence RA, Jones RL. Investigation of the prostaglandin E (EP-) receptor subtype mediating relaxation of the rabbit jugular vein. Br J Pharmacol 1992; 105:817-824; PMID:1324050; http://dx.doi.org/10.1111/j.1476-5381.1992.tb09063.x.
- Hu M, Peluffo G, Chen H, Gelman R, Schnitt S, Polyak K. Role of COX-2 in epithelial-stromal cell interactions and progression of ductal carcinoma in situ of the breast. Proc Natl Acad Sci U S A 2009; 106:3372-7; PMID:19218449; http://dx.doi.org/10.1073/pnas.0813306106.
- Fulton AM. The role of eicosanoids in tumor metastasis. Prostaglandins Leukot Essent Fatty Acids 1988; 34:229-37; PMID:3065787.
- Liu JF, Fong YC, Chang CS, Huang CY, Chen HT, Yang WH, Hsu CJ, Jeng LB, Chen CY, Tang CH. Cyclooxygenase-2 enhances alpha2beta1 integrin expression and cell migration via EP1 dependent signaling pathway in human chondrosarcoma cells. Mol Cancer; 9:43; PMID:20178602; http://dx.doi.org/10.1186/1476-4598-9-43.
- Menter DG, Schilsky RL, DuBois RN. Cyclooxygenase-2 and cancer treatment: understanding the risk should be worth the reward. Clin Cancer Res; 16:1384-90; PMID:20179228; http://dx.doi.org/10.1158/1078-0432.CCR-09-0788.
- Smith WL, DeWitt DL, Garavito RM. Cyclooxygenases: structural, cellular, and molecular biology. Annu Rev Biochem 2000; 69:145-82; PMID:10966456; http://dx.doi.org/10.1146/annurev.biochem.69.1.145.
- Howe LR. Inflammation and breast cancer. Cyclooxygenase/prostaglandin signaling and breast cancer. Breast Cancer Res 2007; 9:210; PMID:17640394; http://dx.doi.org/10.1186/bcr1678.
- Singh-Ranger G, Salhab M, Mokbel K. The role of cyclooxygenase-2 in breast cancer: review. Breast Cancer Res Treat 2008; 109:189-98; PMID:17624587; http://dx.doi.org/10.1007/s10549-007-9641-5.
- Koch TM, Munster S, Bonakdar N, Butler JP, Fabry B. 3D Traction forces in cancer cell invasion. PloS one 2012; 7:e33476; PMID:22479403; http://dx.doi.org/10.1371/journal.pone.0033476.
- Kraning-Rush CM, Califano JP, Reinhart-King CA. Cellular traction stresses increase with increasing metastatic potential. PloS one 2012; 7:e32572; PMID:22389710; http://dx.doi.org/10.1371/journal.pone.0032572.
- Grosch S, Maier TJ, Schiffmann S, Geisslinger G. Cyclooxygenase-2 (COX-2)-independent anticarcinogenic effects of selective COX-2 inhibitors. J Natl Cancer Inst. 2006; 98:736-747; PMID:16757698; http://dx.doi.org/10.1093/jnci/djj206.
- Zhang W, Nandakumar N, Shi Y, Manzano M, Smith A, Graham G, Gupta S, Vietsch EE, Laughlin SZ, Wadhwa M, et al. Downstream of mutant KRAS, the transcription regulator YAP is essential for neoplastic progression to pancreatic ductal adenocarcinoma. Sci Signal 2014; 7:ra42; PMID:24803537.
- Provenzano PP, Inman DR, Eliceiri KW, Knittel JG, Yan L, Rueden CT, White JG, Keely PJ. Collagen density promotes mammary tumor initiation and progression. BMC Med 2008; 6:11; PMID:18442412; http://dx.doi.org/10.1186/1741-7015-6-11.
- Kakkad SM, Solaiyappan M, Argani P, Sukumar S, Jacobs LK, Leibfritz D, Bhujwalla ZM, Glunde K. Collagen I fiber density increases in lymph node positive breast cancers: pilot study. J Biomed Optics 2012; 17:116017; PMID:23117811; http://dx.doi.org/10.1117/1.JBO.17.11.116017.
- Ulrich TA, de Juan Pardo EM, Kumar S. The mechanical rigidity of the extracellular matrix regulates the structure, motility, and proliferation of glioma cells. Cancer Res 2009; 69:4167-74; PMID:19435897; http://dx.doi.org/10.1158/0008-5472.CAN-08-4859.
- Pathak A, Kumar S. Independent regulation of tumor cell migration by matrix stiffness and confinement. Proc Natl Acad Sci U S A 2012; 109:10334-9; PMID:22689955; http://dx.doi.org/10.1073/pnas.1118073109.
- Wang YL, Pelham RJ, Jr. Preparation of a flexible, porous polyacrylamide substrate for mechanical studies of cultured cells. Methods Enzymol 1998; 298:489-96; PMID:9751904; http://dx.doi.org/10.1016/S0076-6879(98)98041-7.
- Fabry B, Maksym GN, Butler JP, Glogauer M, Navajas D, Fredberg JJ. Scaling the microrhelolgy of living cells. Phys Rev Lett 2011; 87:148102; http://dx.doi.org/10.1103/PhysRevLett.87.148102.
- Dai ZJ, Ma XB, Kang HF, Gao J, Min WL, Guan HT, Diao Y, Lu WF, Wang XJ. Antitumor activity of the selective cyclooxygenase-2 inhibitor, celecoxib, on breast cancer in vitro and in vivo. Cancer Cell Int 2012; 12:53-61; PMID:23249419; http://dx.doi.org/10.1186/1475-2867-12-53.