Abstract
Methionine enkephalin (MENK), an endogenous neuropeptide, plays an crucial role in both neuroendocrine and immune systems. CD4+Foxp3+ regulatory T cells (Tregs) are identified as a major subpopulation of T lymphocytes in suppressing immune system to keep balanced immunity. The aim of this research work was to elucidate the mechanisms via which MENK interacts with Tregs in cancer situation. The influence of MENK on transforming growth factor-β (TGF-β) mediated conversion from naïve CD4+CD25- T cells to CD4+CD25+ Tregs was determined and the data from flow cytometry (FCM) analysis indicated that MENK effectively inhibited the expression of Foxp3 during the process of TGF-βinduction. Furthermore, this inhibiting process was accompanied by diminishing phosphorylation and nuclear translocation of Smad2/3, confirmed by western blot (WB) analysis and immunofluorescence (IF) at molecular level. We established sarcoma mice model with S180 to investigate whether MENK could modulate Tregs in tumor circumstance. Our findings showed that MENK delayed the development of tumor in S180 tumor bearing mice and down-regulated level of Tregs. Together, these novel findings reached a conclusion that MENK could inhibit Tregs activity directly and retard tumor development through down-regulating Tregs in mice. This work advances the deepening understanding of the influence of MENK on Tregs in cancer situation, and relation of MENK with immune system, supporting the implication of MENK as a new strategy for cancer immunotherapy.
Abbreviations
MENK | = | methionine enkephalin |
Tregs | = | regulatory T cells |
IL-2 | = | interleukin-2 |
TGF-β, | = | transforming growth factor-β |
Foxp3 | = | forkhead box P3 transcription factor |
CTLA-4 | = | cytotoxic T Lymphocyte Antigen-4 |
GITR | = | glucocorticoid-induced TNFR |
FasL | = | Fas ligand |
CCR4 | = | CC chemokine receptor 4 |
MTS | = | 3-(4, 5-dimethylthiazol-2-yl)-5-(3-carboxymethoxyphenyl)-2-(4-sulfophenyl)-2H-tetrazolium, inner salt |
FCM | = | flow cytometry |
WB | = | protein gel blot |
real-time PCR | = | real-time polymerase chain reaction |
IF | = | immunofluorescence |
MFI | = | median fluorescence intensity |
Introduction
MENK, composed of 5 amino acid with the following sequence: Tyr-Gly-Gly-Phe-Met, an endogenous opioid peptide is generated in adrenal gland and derived from proenkephalin.Citation1 It is suggested to be an important mediator between the immune and neuroendocrine systems.Citation2 MENK enhanced proliferation of subpopulation in human peripheral blood.Citation3
MENK triggers immune responses through binding to the opioid receptors on the surface of immune cells. Recently opioid receptors on many types of tumor cells have been detected out and accumulated documents suggest that MENK participates in antitumor immunity.
Studies in cancer patients treated with MENK demonstrated regression in tumor incidence, retardation in tumor progression and prolonged survival.Citation4-6
Since 2010 our research team has published quite some of data elucidating the role of MENK in coordinating cells in immune system, as evidenced by: (1) dendritic cells (DCs) treated with MENK could up-regulate expressions of opioid receptors (delta and kappa), and positively regulate functions of CD8+T cells;Citation7(2) MENK could induce the influx of Ca2+ on CD8+T cells, leading to translocation of NFAT2 into the nucleus, consequently followed by expansion of CD8+T cells and the development of anti-tumor CTLs;Citation8 (3) MENK participates in innate immunity against tumors by reversing macrophages polarization from M2 to M1, which consequently enhances macrophages to kill tumor cells as well as produce Th1 cytokines;Citation9 (4) MENK could intensify the pathway of DC-CD4+T cells and (5) MENK could improve antitumor activity of DC loaded with tumor antigen. More importantly, our team finds that MENK shows strong inhibition to Treg in peripheral blood of normal donors and cancer patients.Citation10
Regulatory T cells (Tregs) exist in many forms with characteristic, being those that CD4+, CD25+, and Foxp3+ cells and are regarded as an important component of immune system, displaying suppressive activity to other cells, which are implicated in tumor-induced immune escape.
Tregs play an essential role in maintaining immunological self-tolerance and immune homeostasis.Citation11 The transcription factor Foxp3 (forkhead box P3 transcription factor) is specifically expressed in Tregs and is a critical regulator of Tregs development and function. Human and mice with inactivating Foxp3 mutations will result in autoimmune diseases.Citation12-14 Recently, evidence has been accumulating in mouse models and patients with cancer, showing that the number of Foxp3+Tregs is significantly increased in the peripheral blood, bone marrow, tumor draining lymph nodes and tumor tissues,Citation15-17 which have been interlinked with an unfavorable clinical prognosis within human tumors.Citation18,19 Tregs dampens immune responses by suppressing the function of the effector CD4+, CD8+, and natural killer (NK) cellsCitation20,21 and by inhibiting dendritic cell activation.Citation22,23 Thus, Tregs represent a critical barrier to immunotherapy of tumors.Citation24
However, the detailed mechanisms for this remain unclear. What is the relation of Tregs with other immune cells and cancer cells in cancer situation under effect of MENK? Whether could the exogenous MENK exert regulatory effects on Tregs directly and hinder tumor growth?
Therefore, we conducted the following exploration to reveal the detailed regulation of MENK on Tregs in tumor situation and interaction of MENK with Tregs while affecting tumor growth, so as to support clinical application of MENK as a new drug fighting cancers in the future.
Results
Isolation of Tregs
CD4+CD25+ Tregs were isolated from fresh mice splenocytes by the way of magnetic beads. First, negative selection using biotin-conjugated antibody cocktail led to depletion of non-CD4+ T cells. Second, CD4+T cells were labeled by CD25+ PE antibody and underwent positive selection for enriching CD4+CD25+ T cells. In addition, reapplication of the magnetically isolated cells to a second column for additional purification further increased the enrichment of CD25+ cells. The efficiency of purification was assessed by FCM and the purity of Tregs has reached>94% as shown in .
Figure 1. CD4+CD25+Treg cells were freshly isolated from mouse splenocytes using magnetic beads. Mouse splenocytes went through negative selection for CD4+ T cells, followed by CD25+ positive selection. After the process above, the purity was up to >94% by FCM. Data was representative of at least 3 cell isolation experiments.
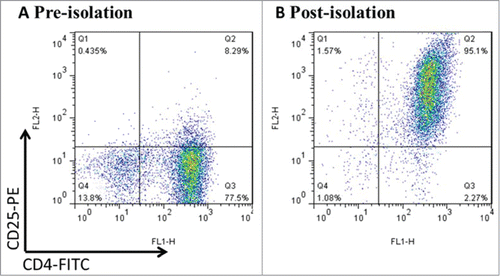
MENK reduced the viability of CD4+CD25+ Tregs
First, whether MENK could affect proliferation of existing Tregs was studies in vitro. After treatment with MENK for 24 h, MTS assay was performed to determine the viability of Tregs in vitro. Compared with control group, Tregs was significantly diminished in response to MENK at the concentration of 10−12 M (), suggesting that MENK inhibited the expansion of Tregs, which was consistent with our findings in lymphocytes of human peripheral blood previously.Citation3
Figure 2. MENK reduced the viability of CD4+CD25+ Treg cells and inhibited TGF-β mediated conversion in vitro. (A), The viability of CD4+CD25+Treg cells was assessed by MTS. (B), Isolated CD4+CD25-T cells underwent TGF-βconversion in the presence of plate-coated anti-CD3 plus soluble anti-CD28 and IL-2 for 48 h or 72 h. The cells receiving treatment of MENK at 10−12 M or RPMI 1640 alone were examined by FCM analysis. Gate was set on CD4+CD25+, and the expression of Foxp3 was shown. (C), Real-time PCR was conducted to quantify the mRNA level of Foxp3 of the cells receiving treatment for 72 h. (D), FCM analysis for the expression pattern of CTLA-4, CCR4, GITR, and CD69 in TGF-βinduced Treg cells for 72 h. Data was investigated by Flowjo software. The red curve was the isotype control staining; the blue curve indicated specific staining. Data was collected from at least 2 independent experiments with similar results and presented as the mean±SD (triplicates). *P < 0.05; **P < 0.01 versus that in RPMI 1640 group (CONT group) as determined by Student's t test or one-way ANOVA.
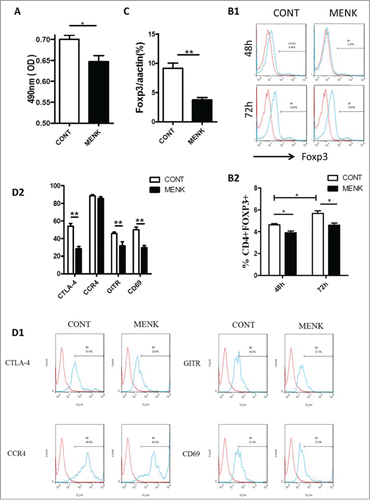
MENK inhibited TGF-βmediated conversion of CD4+CD25-T cells to CD4+Foxp3+Tregs in vitro
Next, we examined whether MENK could weaken the effect of TGF-β on conversion of naïve T cells to Tregs by induction of transcription factor Foxp3. Freshly isolated CD4 + CD25-T cells were cultured with TGF-βin the presence and absence of MENK for 48 h and 72 h, then we evaluated the proportions of CD4+Foxp3+Tregs by FCM. As expected, TGF-βconverted CD4+CD25-T cells to Tregs, and the effect of the conversion became stronger as longer exposure in control group (Foxp3 4.6 ± 0.11 % for 48 h and 5.7 ± 0.24 % for 72 h). In contrast, MENK hindered the conversion effect of TGF-β as evidenced by Foxp3 3.9 ± 0.16 % for 48 h and 4.7 ± 0.064% for 72 h (). Furthermore, this finding was reconfirmed by real-time PCR at mRNA level (). The results provided a clue that MENK displayed inhibiting effect on the viability of Tregs at early stage of conversion. Notably, MENK-treated cells for 72 h displayed an attenuated expression in intracellular CTLA-4, GITR and CD69, which are involved in the immunosuppressive action of Tregs, compared with the cells in control group; but no difference was observed in expression of CCR4 (). Therefore, treatment with MENK induced a significantly resistance of TGF-βconverting CD4+CD25+Foxp3+ Tregs.
MENK inhibited Tregs by affecting the phosphorylation and nuclear translocation of Smad2/3
TGF-β/Smad signaling pathway plays a critical role in the process of induction and maintenance of Foxp3 expression.Citation25 To establish a link between MENK treatment and the decreased Foxp3 expression, we used WB analysis to expose the influence of MENK on phosphorylation of Smad2/3, which are key mediators of TGF-βsignaling. It showed that MENK treatment rendered cells to show a decreased level of phosphorylated Smad2/3 (). We further investigated nuclear translocation of phosphorylated Smad3 at 30 min and 60 min using IF analysis. The results suggested that the cells in MENK group, not in control group, showed much lower fluorescence intensity of pSmad3 inside the nuclei at both 30 min and 60 min(15 ± 2.1 vs. 42 ± 3.4; and 21 ± vs. 40 ± 2.0, respectively) (), which was consistent with activity of Tregs. Thus, MENK suppressed the expression of Foxp3 induced by TGF-βvia weakening the phosphorylation and nuclear translocation of Smad2/3.
Figure 3. MENK inhibited Treg cells by affecting the phosphorylation and nuclear translocation of Smad2/3. (A),WB for analysis of phosphorylated Smad2/3. Band intensities were quantified using Quantity One version4.6.2 software. (B), The slides of cells were incubated with anti-phospho-Smad3 (1:200), and examined by Fluorescence Microscope with Digital CCD Imaging System. Analysis was done with ImageJ software. The data were representative of 3 separate experiments with similar results. The concentration for MENK treatment was 10−12 M. Data was presented as the mean±SD. *P < 0.05; **P < 0.01 vs. the RPMI 1640 group
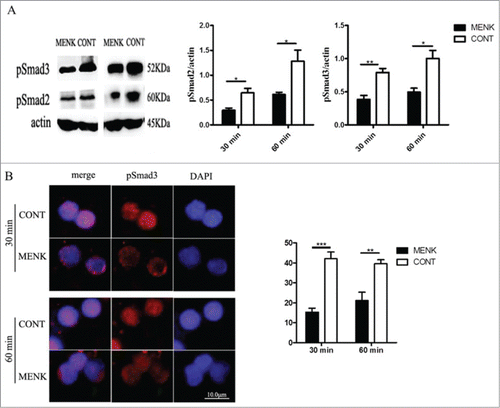
MENK decreased the tumor growth and suppressed the splenic CD4+Foxp3+ Tregs
Extending the studies in vivo, we established a S180 sarcoma model in their respective C57BL/6 mice by subcutaneous injection of 2 × 106/animal. Subsequently, the treatment with MENK was administrated i.p. daily for successively 14 days. First, the sizes and weights of tumor tissues were measured. Strikingly, the weight and size were decreased significantly in MENK treated group compared with those in the normal saline group, which was consistent with the previous results,Citation7-9 (), reconfirming the antitumor effect of MENK in vivo. Then we focus on Tregs in spleen of tumor bearing mice. As shown in , the percentage of Tregs of MENK-treated group were markedly lowered than those in the control group by 44.3%. The similar result was obtained by measuring the mRNA expression level of Foxp3 using real-time PCR (). Also, we determined the expression of intracellular CTLA-4, GITR, and FasL of these Tregs. The following results were available. Although the proportion of CLTA-4 in MENK treatment was slightly lower than control, absolute number of these cells was significantly decreased in MENK group. Moreover, GITR was diminished not only on percentage but also on absolute number. No remarkable difference in FasL expression was found between 2 groups ().
Figure 4. MENK suppressed the tumor growth. (A), C57BL/6 mice were injected with 2 × 106 S180 sarcoma cells s.c. and randomized prior to treatment (8 mice for each treatment), as indicated. MENK (20 mg/kg) was administrated i.p. daily for 14 days after tumor inoculation. Photographs of tumors were the sizes measured on day15(Original magnification × 1). (B and C), The weights and sizes of tumors. Data was presented as the mean±SD. *P < 0.05, ***P < 0.0001 versus that in control group (CONT group) as determined by Student's t test.
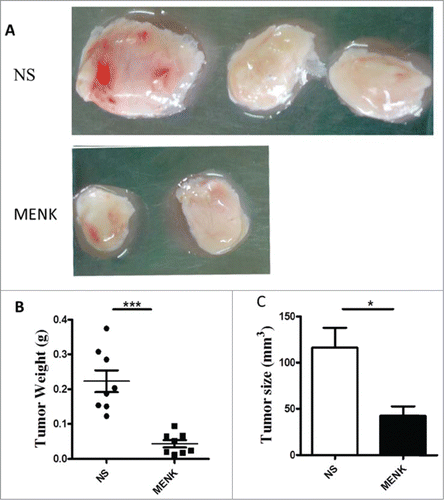
Figure 5. MENK suppressed splenic CD4+Foxp3+ Treg cells in S180 model mice. (A1 and A2), Isolated splenocytes were stained with anti-CD4 (FITC) and anti-Foxp3 (APC) and submitted to FCM analysis. (B), The mRNA level of Foxp3 in splenocytes of both groups. (C), The cells were gated on CD4+Foxp+ and analyzed for CTLA-4, GITR and FasL- positive cells, respectively. Data was analysis by FCS Express and Flowjo software. The red line was the isotype control staining; the blue line indicated specific staining mentioned above. The data were representative of at least 3 independent experiments with similar results and were presented as the mean±SD. *P < 0.05; **P < 0.01 vs. that in control group (NS group) as determined by Student's t test.
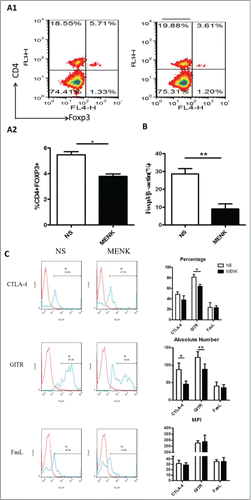
Next we investigated whether MENK could modulate Tregs within tumor-draining lymph nodes(TDLN), which are related to tumor-induced immune evasion.Citation26 Interestingly, in contrast to the control group, CD4+Foxp3+Tregs were more frequent in TDLN in MENK-treatment group (), different from the findings in splenocytes; nevertheless, the proportion of CTLA-4, GITR, CCR4 and CD69 resembled those in control group when these cells were analysis based on CD4+Foxp3+ gate. In particular, of the mice receiving MENK treatment, CTLA-4+ Tregs expanded in absolute number and GITR+ Tregs displayed a slightly higher MFI than their rivals of mice receiving normal saline (). Hence, MENK dampened the development of S180 tumor, and led to a modification of Tregs in spleen and in TDLN.
Figure 6. (A), Collected lymphocytes from tumor draining lymph nodes (TDLN) were stained with anti-CD4 (FITC) and anti-Foxp3 (APC) and submitted to FCM. (B), CD4+Foxp3+ cells were gated on and analyzed forCTLA-4, GITR, CCR4 and CD69- positive cells, respectively. The red line was the isotype control staining; the blue line indicated specific staining. The data are one representative of 3 separate experiments with similar results. Data were presented as the mean±SD. *P < 0.05 versus that in control group (CONT group) as determined by Student's t test.
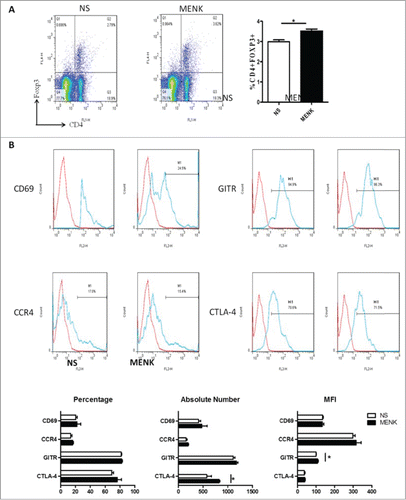
Discussion
Tumor-induced immune suppression continues to impede the effectiveness of immune-based therapies against cancer. Solid tumors utilize numerous mechanisms designed to evade host antitumor immune responses.Citation27 Tregs play an important role in the control of cancer immunity and Tregs accumulate in tumor tissues to appear to hinder the induction of effective antitumor immunity.Citation28 Thus, optimal strategies need to be established for reducing Tregs or attenuating their suppressive function in tumor tissues, together with activating and expanding tumor-specific effector T cells.Citation29
MENK, the endogenous neuropeptide, appears to be useful in immune responses against tumors by up-regulation of CD8+T cells’ activity; down-regulation macrophage polarization from M2 to M1 type; induction dendritic cell maturation to initiate T cells responds and to intensify CD4+T cells functions as well as the secretion of various cytokines.Citation7-9
In current study we provide evidence that MENK attenuates TGF-βinduced conversion of CD4+CD25-T cells to CD4+CD25+Tregs in vitro and that MENK affects Tregs in spleen and TDLN in S180 mice model. Several important conclusions can be drawn from the current work. First in vitro experiments, MENK treatment impaired proliferation of freshly isolated Tregs in despite of stimulation with anti-CD3 as well as high dose of exogenous IL-2 in the first 24 h, which was consistence with our previous observation.Citation3 Importantly, MENK rendered CD4+CD25-T cells resistant to TGF-β induction of transcription factor Foxp3, which could be attributed to MENK in diminishing phosphorylation of Smad2/3 and consequent nuclear translocation. Furthermore, phenotypic analysis of these Tregs post treatment with MENK revealed a lower expression pattern of GITR, intracellular CTLA-4, and CD69 than those in control. No significant difference was observed for the expression of CCR4 between 2 groups.
Second in S180 mice model, MENK treatment resulted in restrained the development of S180 solid tumor associated with a lower frequency of CD4+Foxp3+Tregs. Data from real-time PCR reconfirmed downregulation of Foxp3 at mRNA level as well. Of these Tregs, the expression of GITR was reduced not only in frequency but also in absolute number; yet, intracellular CTLA-4 is impaired in absolute number in MENK treatment group. However, the expression of FasL and CCR4 were not significantly altered compared with that in normal control.
Foxp3 is a transcription factor necessary and sufficient for induction and maintenance of the immunosuppressive functions in Tregs.Citation30,31 Because MENK treatment inhibits the expression of Foxp3 in vitro and in vivo, it is plausible that MENK could not only decrease the number of Tregs, but also attenuate its suppressive functions. CTLA-4 is a potent negative regulator of T cell-mediated immune responses through its action in both effector T cells and Tregs. On one hand, CTLA-4 is necessary to mold a TGF-β/CTLA-4/Foxp3/CTLA-4 positive loop for the generation and maintenance of induced Tregs;Citation32 on the other hand, CTLA-4 is involved in contact-dependent suppression of Tregs.Citation33 MENK repression of CTLA-4 expression on Tregs may account for additional mechanism of how MENK restrains TGF-βinducing Tregs to express Foxp3 both at protein level and at mRNA level from a new perspective. GITR is constitutively expressed on CD4+Foxp3+Tregs, which modulates the suppression of Tregs.Citation34 Declined GITR expression on Tregs with MENK treatment both in vitro and in vivo may contribute to lower frequencies of CD4+Foxp3+Tregs by make them susceptible to TCR-mediated apoptotic signals. Additionally, CD69 is identified as negative regulator by promoting the synthesis of TGF-β.Citation35,36 In this regard, it is feasible that MENK facilitates CD4+CD25-T cells resistant to TGF-βby impairing the expression of CD69 in vitro. These findings support the notion that MENK regulates Tregs on total number and its suppressive activities via impacting the expression of Foxp3, CTLA-4, GITR and CD69.
Surprisingly, our data indicates that mice receiving MENK treatment harbor higher frequency of CD4+Foxp3+Tregs with other important molecules depleting their advantages of enhancing antitumor activities in TDLN; even, the absolute number of CTLA-4+Tregs is higher than normal saline treatment. The results seems a great contradiction to our observation of obvious shrinkage of tumor volumes in vivo and to the findings of MENK regulation Tregs in vitro. This apparent inconsistency could be, at least in part, attributed to different compositions of Foxp3+T cells subpopulations in tumor tissues.Citation29
Numerous studies has suggested that certain Tregs could undergo reprogramming without loss of the transcription factor Foxp3.Citation37 The reprogrammed Tregs provides virtually all of the helper activity to support the initial cross-presentation to CD8+ cells.Citation38 Indoleamine 2,3-dioxygenase (IDO), which is expressed by a subset of murine plasmacytoid dendritic cells (pDCs) in TDLN, functions as a critical molecular “switch” in Tregs reprogramming.Citation24
It should be further noted that our team has demonstrated that MENK could up-regulate TLR-9 effectively; MENK could induce DCs to develop mainly from bone marrow progenitors to myeloid DCs (mDCs) rather than pDCs.Citation7
These findings suggest the possibility that MENK might be involved in reprogramming Tregs by decreasing the expression of IDO and impairing its effect on sustaining the suppressive activity. TLR9 may also be related as the upstream of MyD88 and IL-6.Citation39
In spite of fruitful results we obtained above, there are still quite a few issues to be solved in more depth. For example, the concrete signal pathway via which MENK modulates Tregs to undergo reprogramming.
The marked inhibition of the expansion of Tregs by MENK reveals a new mechanism by which MENK exerts positive regulation to the cells of immune system or anticancer and therefore this is unique discovery by us would guide explanation to application of MENK as a drug in cancer treatment.
Materials and Methods
Ethic statement
All animal experiments were conducted in accordance with the guide for Animal Use and Care Committee of China Medical University. The protocol was approved by the Committee on the ethics of Animal Experiments of China National Institutes of Health (permit number GB 14923-2010).
Cell line
Sarcoma 180 (S180) cell line was brought from cell resource center of Shanghai institute of life science, Chinese Academy of Science and the cell line had been reconfirmed by multi-loci DNA fingerprinting and multiplex PCR DNA profiling analysis. The cells were grown in vivo in syngeneic C57BL/6 mice in ascites by intra-peritoneal administration.
Key reagents
MENK was a gift from Penta Biotech. Inc.. USA (≥98% purity). The McAbs used in current work were purchased from BD PharMingen, Biolegend, Invitrogen Life Technology and Cell Signaling Technology. RMPI 1640 was supplemented with 10% fetal calf serum, 2 mM L-glutamine, 1.2% sodium bicarbonate, 100 units/ml penicillin and 100 ug/ml streptomycin. Other chemicals frequently used in our laboratory were products made in Sigma-Aldrich.
Mice
Female C57BL/6 mice (aged 6-8 weeks, 18-21 g) were obtained from Slac Laboratory Animals Co. Ltd. All animals were treated kindly per the Guide for the Care and Use of Laboratory Animals of China medical university and all the surgical procedures were approved by The Committee of Experimental Animals of China Medical University. All mice were anesthetized by intraperitoneal injection of Inactin (thiobutabarbital,100 mg/kg; RBI, Natick, MA) and ventilated with oxygen (35%) and air mixture.
CD4+CD25+ Tregs and CD4+CD25- T cells isolation
Tregs and CD4+CD25-T cells were isolated from C57BL/6 mice spleens using magnetic bead separation (CD4+CD25+ Treg cell kit; MiltenyiBiotec). Briefly, non-CD4+ T cells were depleted using biotinylated Ab mixture and anti-biotin microbeads. CD4+CD25+Tregs were positively selected from CD4+T cells using PE-labeled anti-CD25 mAb and anti-PE microbeads. The purity of CD4+CD25+Tregs was checked by FCM and has reached >94%.
MTS assay
After isolation 2 × 105/well in a total volume of 100 ul of the purified Tregs were plated into a 96-well plate (Corning-Costar) pre-coated with 5 ug/ml anti-CD3 Ab. The culture media contained 1.2 ug/ml anti-CD28 Ab and100 ng/ml rIL- 2 with or without 10−12 M MENK.Citation7,9 All cells were grown in a humidified atmosphere of 5% CO2 and 95% air at 37°C After 24 h, finally, the viability of Tregs was determined at 490 nm (A490) by 3-(4,5-dimethylthiazol-2-yl)-5-(3-carboxymethoxyphenyl)-2-(4-sulfophenyl)-2H-tetrazolium, inner salt (MTS, Promega)Citation40 on bichromaticmicroplate reader.
MENK affected conversion of CD4+Foxp3- T cells to CD4+Foxp3+ Tregs in vitro
Isolated CD4+CD25- T cells were stimulated with plate-bound anti- CD3 (2.5 ug/ml) and soluble anti-CD28 (2 ug/ml) in the presence of TGF-β(2.5 ng/ml; Protech) and rIL-2 (200 U/ml)Citation41 and incubated with MENK (10−12 M) or RPMI1640 alone at 37°C for indicated time. Then the following analyses were used: FCM analysis for expression of Foxp3; WB for phosphorylated Smad2/3; IF for reconfirmation of nuclear translocation of phosphorylated Smad3 and real-time PCR for Foxp3 expression at mRNA level.
MENK affected proliferation of Tregs in mouse tumor Model in vivo
S180 cells (2.0 × 106/0.1 ml) were injected subcutaneously (s.c) into C57BL/6 mice. At 24 h after tumor cell injection, the mice were divided into 2 groups (8 mice/ group). The mice in testing group were treated with MENK (20 mg/kg) i.p. daily for 14 successive days and the mice in control group were treated with normal saline. Then the mice were sacrificed and spleens and tumor draining lymph nodes were collected for analysis of Foxp3 expression with FCM analysis and real-time PCR. Also tumor weights were measured to evaluate the efficacy of anticancer by MENK.
FCM analysis
For experiment in vivo, the spleens and tumor draining lymph nodes obtained from 2 groups of the mice were prepared as a single-cell suspension in FCM buffer (PBS with 2% FBS and 0.1% NaN3). The staining was performed per the manufacturer's instructions and following Abs: anti-CD4 (FITC), anti-Foxp3 (APC), anti-CD69 (PE), anti-GITR (PE), anti- FasL (PE), anti-CD152 (PE) and anti-CCR4 (PE) were used.
For experiment in vitro, the purified CD4+CD25-T cells were stained in the same way described above. The samples were acquired with a FACSCalibur (BDBiosciences) and analyzed with Flowjo7.6.1 software and FCS Express Version 4 software.
Immunofluorescence analysis
Immuno-staining was performed to detect translocation of phosphor- Smad3 inside Tregs.Citation42 Briefly, the slides of cells were blocked with 5% BSA in PBS, and incubated with anti-phospho-Smad3 (1:200, Cell Signaling Technology) overnight at 4°C. After slides were rinsed with PBS the immune-reactivity was visualized by incubating the slides with a donkey-anti-rabbit IgG antibody conjugated with Alexa Fluor 546 (Invitrogen; 1:400) for 2 h at room temperature. The slides were then counterstained with DAPI (5 ug/ml) to visualize the nuclei under a fluorescence microscope (Fluorescence Microscope with Digital CCD Imaging System; Olympus). Finally the fluorescent intensity was measured by ImageJ software for at least 6 regions per section.
Western blot assay
The purified cells treated with MENK and untreated cells were lysed in cell disruption buffer (mirVana™ PARIS™ RNA and Native Protein Purification Kit; Invitrogen Life Technology, CA). Protein samples (40 μg) were subjected to electrophoretic analysis through 10% SDS-PAGECitation43 and subsequently transferred to PVDF membranes (Millipore, Bedford, MA). Following blocking in 5% fat-free milk in Tris-buffered saline tween-20 buffer (TBST), membranes were immune-labeled with primary antibodies: rabbit-anti-phospho-Smad2 (1:1000, Cell Signaling Technology), rabbit-anti-phospho-Smad3 (1:1000, Cell Signaling Technology) and rabbit –anti-β actin (1:5000, Cell Signaling Technologies) overnight at 4°C. Blots were exposed to the relevant horseradish peroxidase (HRP)-labeled secondary antibodies (1:2000, Cell Signaling Technology). Image detection was conducted using an Image Reader camera (BioRad). Band intensities were quantified using Quantity One version 4.6.2 software.
Real-time PCR
Total RNA was extracted from both in vivo and in vitro cells using mirVana™ PARIS™ RNA and Native Protein Purification Kit (Invitrogen Life Technologies) in accordance with the manufacturer's instructions, after which the concentration of total RNA was quantified by determining the OD at 260 nm (OD 260). The reaction mixtures were then subjected to 40 cycles of denaturation at 95°C for 15s and annealing at 60°C for 1 min. Amplification was conducted using the following gene-specific primers: Foxp3-F, 5′-GGC CCT TCT CCA GGA CAG A-3′; Foxp3-R, 5′-GCT GAT CAT GGC TGG GTT GT-3′; β-actin-F, 5′-TTC CAG CGT TCC TTC TTG GGT AT-3′; β-actin-R, 5′- GTT GGC ATA GAG GTG TTT ACG G-3′. Real-time PCR was conducted on a 7500 Real-Time PCR System (Applied Biosystems) using SYBR Green I as the dsDNA-specific binding dye continuously fluorescence monitoring.
Statistical analysis
Data was collected from at least 2 independent experiments. Statistical analysis was conducted using Prism 5.01 software (GraphPad Software). All values were presented as the means ± SD. Differences between the means in testing group and control group were determined by one-way ANOVA or Student's t test. A p value <0.05 was considered to be statistically significant.
Disclosure of Potential Conflicts of Interest
No potential conflicts of interest were disclosed.
Acknowledgments
We thank the additional researchers who have also contributed significantly to this study.
Funding
This work was supported by project for construction of discipline platform in universities of Liaoning province, China. This work received funding from Liaoning science foundation (2009225008-7, 2012225016).
References
- Tischler AS, DeLellis RA, Slayton VW, Blount MW, Wolfe HJ. Enkephalin-like immunoreactivity in human adrenal medullary cultures. Lab Invest 1983; 48:13-8; PMID:6337295
- Piva M, Moreno JI, Jenkins FS, Smith JKA, Thomas JL, Montgomery C, Wilson CB, Sizemore RC. In vitro modulation of cytokine expression by enkephalin-derived peptides. Neuroimmunomodulation 2005; 12:339-47; PMID:16557034; http://dx.doi.org/10.1159/000091127
- Hua H, Lu C, Li W, Meng J, Wang D, Plotnikoff NP, Wang E, Shan F. Comparison of stimulating effect on subpopulations of lymphocytes in human peripheral blood by methionine enkephalin with IL-2 and IFN-gamma. Hum Vaccin Immunother 2012; 8:1082-9; PMID:22854663; http://dx.doi.org/10.4161/hv.20759
- McLaughlin PJ, Stack BC, Braine KM, Ruda JD, Zagon IS. Opioid growth factor inhibition of a human squamous cell carcinoma of the head and neck in nude mice: dependency on the route of administration. Int J Oncol 2004; 24:227-32; PMID:14654962
- Hatzoglou A, Kampa M, Castanas E. Opioid-somatostatin interactions in regulating cancer cell growth. Front Biosci 2005; 10:244-56; PMID:15574365; http://dx.doi.org/10.2741/1524
- Zagon IS, Jaglowski JR, Verderame MF, Smith JP, Leure-duPree AE, McLaughlin PJ. Combination chemotherapy with gemcitabine and biotherapy with opioid growth factor (OGF) enhances the growth inhibition of pancreatic adenocarcinoma. Cancer Chemother Pharmacol 2005; 56:510-20; PMID:15947928; http://dx.doi.org/10.1007/s00280-005-1028-x
- Liu J, Chen W, Meng J, Lu C, Wang E, Shan F. Induction on differentiation and modulation of bone marrow progenitor of dendritic cell by methionine enkephalin (MENK). Cancer Immunol Immunother 2012; 61:1699-711; PMID:22392190; http://dx.doi.org/10.1007/s00262-012-1221-9
- Li W, Chen W, Herberman RB, Plotnikoff NP, Youkilis G, Griffin N, Wang E, Lu C, Shan F. Immunotherapy of cancer via mediation of cytotoxic T lymphocytes by methionine enkephalin (MENK). Cancer Lett 2013
- Chen W, Liu J, Meng J, Lu C, Li X, Wang E, Shan F. Macrophage polarization induced by neuropeptide methionine enkephalin (MENK) promotes tumoricidal responses. Cancer Immunol Immunother 2012; 61:1755-68; PMID:22419372; http://dx.doi.org/10.1007/s00262-012-1240-6
- Wang Q, Gao X, Yuan Z, Wang Z, Meng Y, Cao Y, Plotnikoff NP, Griffin N, Shan F. Methionine enkephalin (MENK) improves lymphocyte subpopulations in human peripheral blood of 50 cancer patients by inhibiting regulatory T cells (Tregs). Hum Vaccin Immunother 2014; 10:1836-40; PMID:25424790; http://dx.doi.org/10.4161/hv.28804
- Nishikawa H, Sakaguchi S. Regulatory T cells in tumor immunity. Int J Cancer 2010; 127:759-67; PMID:20518016
- Fontenot JD, Gavin MA, Rudensky AY. Foxp3 programs the development and function of CD4+CD25+ regulatory T cells. Nat Immunol 2003; 4:330-6; PMID:12612578; http://dx.doi.org/10.1038/ni904
- Hori S, Nomura T, Sakaguchi S. Control of regulatory T cell development by the transcription factor Foxp3. Science 2003; 299:1057-61; PMID:12522256; http://dx.doi.org/10.1126/science.1079490
- Lahl K, Loddenkemper C, Drouin C, Freyer J, Arnason J, Eberl G, Hamann A, Wagner H, Huehn J, Sparwasser T. Selective depletion of Foxp3+ regulatory T cells induces a scurfy-like disease. J Exp Med 2007; 204:57-63; PMID:17200412; http://dx.doi.org/10.1084/jem.20061852
- Salama P, Phillips M, Grieu F, Morris M, Zeps N, Joseph D, Platell C, Iacopetta B. Tumor-infiltrating FOXP3(+) T regulatory cells show strong prognostic significance in colorectal cancer. J Clin Oncol 2009; 27:186-92; PMID:19064967; http://dx.doi.org/10.1200/JCO.2008.18.7229
- Ge YZ, Domschke C, Stoiber N, Schott S, Heil J, Rom J, Blumenstein M, Thum J, Sohn C, Schneeweiss A, et al. Metronomic cyclophosphamide treatment in metastasized breast cancer patients: immunological effects and clinical outcome. Cancer Immunol Immunother 2012; 61:353-62; PMID:21915801; http://dx.doi.org/10.1007/s00262-011-1106-3
- Miller AM, Lundberg K, Ozenci V, Banham AH, Hellstrom M, Egevad L, Pisa P. CD4(+)CD25(high) T cells are enriched in the tumor and peripheral blood of prostate cancer patients. J Immunol 2006; 177:7398-405; PMID:17082659; http://dx.doi.org/10.4049/jimmunol.177.10.7398
- Gallimore AM, Simon AK. Positive and negative influences of regulatory T cells on tumour immunity. Oncogene 2008; 27:5886-93; PMID:18836469; http://dx.doi.org/10.1038/onc.2008.269
- Griffiths RW, Elkord E, Gilham DE, Ramani V, Clarke N, Stern PL, Hawkins RE. Frequency of regulatory T cells in renal cell carcinoma patients and investigation of correlation with survival. Cancer Immunol Immunother 2007; 56:1743-53; PMID:17487490; http://dx.doi.org/10.1007/s00262-007-0318-z
- Antony PA, Piccirillo CA, Akpinarli A, Finkelstein SE, Speiss PJ, Surman DR, Palmer DC, Chan CC, Klebanoff CA, Overwijk WW, et al. CD8+ T cell immunity against a tumor/self-antigen is augmented by CD4+ T helper cells and hindered by naturally occurring T regulatory cells. J Immunol 2005; 174:2591-601; PMID:15728465; http://dx.doi.org/10.4049/jimmunol.174.5.2591
- Nishikawa H, Jager E, Ritter G, Old LJ, Gnjatic S. CD4+ CD25+ regulatory T cells control the induction of antigen-specific CD4+ helper T cell responses in cancer patients. Blood 2005; 106:1008-11; PMID:15840697; http://dx.doi.org/10.1182/blood-2005-02-0607
- Misra N, Bayry J, Lacroix-Desmazes S, Kazatchkine MD, Kaveri SV. Cutting edge: human CD4+CD25+ T cells restrain the maturation and antigen-presenting function of dendritic cells. J Immunol 2004; 172:4676-80; PMID:15067041; http://dx.doi.org/10.4049/jimmunol.172.8.4676
- Larmonier N, Marron M, Zeng Y, Cantrell J, Romanoski A, Sepassi M, Thompson S, Chen X, Andreansky S, Katsanis E. Tumor-derived CD4(+)CD25(+) regulatory T cell suppression of dendritic cell function involves TGF-beta and IL-10. Cancer Immunol Immunother 2007; 56:48-59; PMID:16612596; http://dx.doi.org/10.1007/s00262-006-0160-8
- Sharma MD, Hou DY, Liu YJ, Koni PA, Metz R, Chandler P, Mellor AL, He Y, Munn DH. Indoleamine 2,3-dioxygenase controls conversion of Foxp3(+) Tregs to TH17-like cells in tumor-draining lymph nodes. Blood 2009; 113:6102-11; PMID:19366986; http://dx.doi.org/10.1182/blood-2008-12-195354
- Tone Y, Furuuchi K, Kojima Y, Tykocinski ML, Greene MI, Tone M. Smad3 and NFAT cooperate to induce Foxp3 expression through its enhancer. Nat Immunol 2008; 9:194-202; PMID:18157133; http://dx.doi.org/10.1038/ni1549
- Jacobs JF, Nierkens S, Figdor CG, de Vries IJ, Adema GJ. Regulatory T cells in melanoma: the final hurdle towards effective immunotherapy? Lancet Oncol 2012; 13:e32-42; PMID:22225723; http://dx.doi.org/10.1016/S1470-2045(11)70155-3
- Mailloux AW, Young MR. NK-dependent increases in CCL22 secretion selectively recruits regulatory T cells to the tumor microenvironment. J Immunol 2009; 182:2753-65; PMID:19234170; http://dx.doi.org/10.4049/jimmunol.0801124
- Sugiyama D, Nishikawa H, Maeda Y, Nishioka M, Tanemura A, Katayama I, Ezoe S, Kanakura Y, Sato E, Fukumori Y, et al. Anti-CCR4 mAb selectively depletes effector-type FoxP3+CD4+ regulatory T cells, evoking antitumor immune responses in humans. Proc Natl Acad Sci U S A 2013; 110:17945-50; PMID:24127572; http://dx.doi.org/10.1073/pnas.1316796110
- Nishikawa H, Sakaguchi S. Regulatory T cells in cancer immunotherapy. Current Opin Immunol 2014; 27C:1-7; PMID:24413387; http://dx.doi.org/10.1016/j.coi.2013.12.005
- Martin F, Ladoire S, Mignot G, Apetoh L, Ghiringhelli F. Human FOXP3 and cancer. Oncogene 2010; 29:4121-9; PMID:20498631; http://dx.doi.org/10.1038/onc.2010.174
- Khattri R, Cox T, Yasayko SA, Ramsdell F. An essential role for Scurfin in CD4+CD25+ T regulatory cells. Nat Immunol 2003; 4:337-42; PMID:12612581; http://dx.doi.org/10.1038/ni909
- Zheng SG, Wang JH, Stohl W, Kim KS, Gray JD, Horwitz DA. TGF-beta requires CTLA-4 early after T cell activation to induce FoxP3 and generate adaptive CD4(+) CD25(+) regulatory cells. J Immunol 2006; 176:3321-9; PMID:16517699; http://dx.doi.org/10.4049/jimmunol.176.6.3321
- Kong KF, Fu G, Zhang Y, Yokosuka T, Casas J, Canonigo-Balancio AJ, Becart S, Kim G, Yates JR 3rd, Kronenberg M, et al. Protein kinase C-eta controls CTLA-4-mediated regulatory T cell function. Nat Immunol 2014; 15:465-72; PMID:24705298; http://dx.doi.org/10.1038/ni.2866
- Shimizu J, Yamazaki S, Takahashi T, Ishida Y, Sakaguchi S. Stimulation of CD25(+)CD4(+) regulatory T cells through GITR breaks immunological self-tolerance. Nat Immunol 2002; 3:135-42; PMID:11812990; http://dx.doi.org/10.1038/ni759
- Esplugues E, Sancho D, Vega-Ramos J, Martinez C, Syrbe U, Hamann A, Engel P, Sánchez-Madrid F, Lauzurica P. Enhanced antitumor immunity in mice deficient in CD69. J Exp Med 2003; 197:1093-106; PMID:12732655; http://dx.doi.org/10.1084/jem.20021337
- Sancho D, Gomez M, Viedma F, Esplugues E, Gordon-Alonso M, Garcia-Lopez MA, de la Fuente H, Martínez-A C, Lauzurica P, Sánchez-Madrid F. CD69 downregulates autoimmune reactivity through active transforming growth factor-beta production in collagen-induced arthritis. J Clin Invest 2003; 112:872-82; PMID:12975472; http://dx.doi.org/10.1172/JCI200319112
- Sharma MD, Huang L, Choi JH, Lee EJ, Wilson JM, Lemos H, Pan F, Blazar BR, Pardoll DM, Mellor AL, et al. An inherently bifunctional subset of Foxp3(+) T helper cells is controlled by the transcription factor eos. Immunity 2013; 38:998-1012; PMID:23684987; http://dx.doi.org/10.1016/j.immuni.2013.01.013
- Sharma MD, Hou DY, Baban B, Koni PA, He YK, Chandler PR, Blazar BR, Mellor AL, Munn DH. Reprogrammed foxp3(+) regulatory T cells provide essential help to support cross-presentation and CD8(+) T cell priming in naive mice. Immunity 2010; 33:942-54; PMID:21145762; http://dx.doi.org/10.1016/j.immuni.2010.11.022
- Pasare C, Medzhitov R. Toll pathway-dependent blockade of CD4+CD25+ T cell-mediated suppression by dendritic cells. Science 2003; 299:1033-6; PMID:12532024; http://dx.doi.org/10.1126/science.1078231
- McCauley J, Zivanovic A, Skropeta D. Bioassays for anticancer activities. Methods Mol Biol 2013; 1055:191-205; PMID:23963912; http://dx.doi.org/10.1007/978-1-62703-577-4_14
- Chen W, Jin W, Hardegen N, Lei KJ, Li L, Marinos N, McGrady G, Wahl SM. Conversion of peripheral CD4+CD25- naive T cells to CD4+CD25+ regulatory T cells by TGF-beta induction of transcription factor Foxp3. J Exp Med 2003; 198:1875-86; PMID:14676299; http://dx.doi.org/10.1084/jem.20030152
- Haiqi H, Yong Z, Yi L. Transcriptional regulation of Foxp3 in regulatory T cells. Immunobiology 2011; 216:678-85; PMID:21122941; http://dx.doi.org/10.1016/j.imbio.2010.11.002
- Choi SI, Kim BY, Dadakhujaev S, Jester JV, Ryu H, Kim TI, Kim EK. Inhibition of TGFBIp expression by lithium: implications for TGFBI-linked corneal dystrophy therapy. Invest Ophthalmol Vis Sci 2011; 52:3293-300; PMID:21310903; http://dx.doi.org/10.1167/iovs.10-6405