Abstract
Aquaporin 1 (AQP1), a member of water channel proteins, functions as a water-selective transporting protein in cell membranes. In recent years, AQP1 has been found to be overexpressed in various tumors. However, the molecular mechanism underlying the biological function of AQP1 in osteosarcoma is still unclear. This study was aimed at elucidating the roles of AQP1 in regulating the biological behavior of osteosarcoma cells. In this study, we found that AQP1 mRNA was elevated in osteosarcoma tissue. High level of AQP1 was associated with poor prognosis in osteosarcoma. Then, we found that knockdown of AQP1 in osteosarcoma cells, U2OS or MG63 cells inhibited cell proliferation and significantly increased cells population in G1 phase. Additionally, suppressing AQP1 expression in osteosarcoma cells dramatically induced cell apoptosis. We also found that down-regulation of AQP1 significantly inhibited cell adhesion and invasion. More importantly, AQP1 knockdown inhibited tumor growth in vivo and prolonged the survival time of nude mice. Gene set enrichment analysis (GSEA) showed that transforming growth factor-β (TGF-β) signaling pathway and focal adhesion genes was correlatively with AQP1 expression. In addition, real time PCR and western blot analysis revealed that expression of TGF-β1/TGF-β2, RhoA and laminin β 2 (LAMB2) was remarkably impaired by AQP1 silencing. In conclusion, AQP1 may be a useful diagnosis and prognosis marker for osteosarcoma. AQP1 knockdown can effectively inhibit cell proliferation, adhesion, invasion and tumorigenesis by targeting TGF-β signaling pathway and focal adhesion genes, which may serve a promising therapeutic strategy for osteosarcoma.
Abbreviations
AQP1 | = | Aquaporin 1 |
ECM | = | extracellular matrix |
EMT | = | mesenchymal–epithelial transition |
FITC | = | fluorescein isothiocyanate |
GSEA | = | Gene set enrichment analysis |
LAMB2 | = | laminin beta 2 |
TGF-β | = | transforming growth factor-β. |
Introduction
Osteosarcoma is a rare malignancy but the most common bone sarcoma in children and adolescents.Citation1 Osteosarcoma arises predominantly in the long bones and rarely in the soft tissues.Citation2 Along with the development of combination treatment (adjuvant chemotherapy, surgery and neoadjuvant chemotherapy), the prognosis of osteosarcoma has gradually improved over the past 30 y.Citation3-5 However, for patients who present with metastatic disease or whose tumor recurs, the survival was less than 30% and 20%, respectively.Citation6 This emphasizes the need for new therapeutic targets and approaches for osteosarcoma.
Aquaporin 1 (AQP1) is membrane protein that control the permeability of endothelial and epithelial barriers by facilitating water movement across cell membranes.Citation7 In recent years, AQP1 has been found to be overexpressed in various tumors.Citation8-15 Exploration of a possible role of AQP1 in tumor progression has revealed an unexpected function of AQPs in cell migration. It is reported that AQP1 accelerate the migration of cultured Chinese hamster ovary cells,Citation16 fisher rat thyroid cells,Citation16 cultured renal proximal tubule cells,Citation17 B16F10 melanoma,Citation18 and 4T1 breast cancer cells.Citation18 Cell migration was greatly impaired in aortic endothelia from AQP1-null mice, with abnormal vessel formation in vitro.Citation16 Recently, its promotion effects on the proliferation and anchorage-independent growth of NIH-3T3 cells was revealed by Hoque et al.. However, little is known about the expression pattern and biological functions of AQP1 in osteosarcoma.
Here we describe our study in helping understanding the role of AQP1 in osteosarcoma. Firstly, osteosarcoma dataset analysis (Gene Expression Omnibus database, Access ID: GSE42352) and real-time PCR analysis showed that AQP1 was highly expressed in osteosarcoma tissues. Moreover, high level of AQP1 expression was associated with poor prognosis in osteosarcoma. Then, we investigated the biological function of AQP1 in osteosarcoma cell lines. We found that AQP1 was involved in multiple cellular progress including cell proliferation, apoptosis, adhesion and invasion. And AQP1 might participate these progresses by regulating the expression of transforming growth factor-β1 (TGF-β1), TGF-β2, RhoA and laminin β 2 (LAMB2). At last, in vivo tumor formation experiment showed that knockdown of AQP1 remarkably inhibited the tumor growth. These data suggest that AQP1 is a potent oncogene and a potential target for treatment of osteosarcoma.
Results
Up-regulated AQP1 expression correlated with poor osteosarcoma patient survival
We first analyzed data of osteosarcoma patients from GEO data set (Access ID: GSE42352) and found that AQP1 expression significantly increased in osteosarcoma tumor tissues compared with the adjacent tissues of patients (, P < 0.01). We then compared the mRNA level of AQP1 between osteosarcoma tissues (n = 44) and bone cysts (n = 14) collected from patients admitted to Department of Orthopedics, Shanghai tenth People's Hospital by using real-time PCR. Our data also suggest AQP1 is significantly overexpressed in osteosarcoma tissues compared with that in bone cysts (, P < 0.001).
Figure 1. Upregulated AQP1 expression correlated with poor osteosarcoma patient survival and knockdown of AQP1 suppressed the proliferation of osteosarcoma cells. (A) AQP1 expression was significantly increased in osteosarcoma tissues when compared with the adjacent tissues of patients from GEO dataset GSE42352 (*P < 0.05). (B) AQP1 mRNA level was significantly higher in osteosarcoma tissues (n = 64) than that in bone cysts (n = 14) from patients admitted to Shanghai tenth People's Hospital between 2009 and 2012. (C) the overall survival time of 64 patients with osteosarcoma. (D) AQP1 expression level in 5 osteosarcoma cell lines was analyzed by Western blot (upper panel) and real-time PCR (lower panel). Data were based on at least 3 independent experiments. (E, F) Expression of AQP1 in U2OS and MG63 cells was analyzed by Western blot (upper panel) and real-time PCR (lower panel). WT: wild type cells; NC: scrambled shRNA virus infected cells; Ri-1, Ri-2 and Ri-3: AQP1-shRNA-1, −2 and −3 virus infected cells. (G, H) Cell proliferation was detected 0, 24, 48 and 72 hours after viral infection in U2OS and MG63 cells. WT: wild type cells; NC: scrambled shRNA virus infected cells; AQP1-Ri-1: AQP1-shRNA-1 virus infected cells. Data were based on at least 3 independent experiments, and shown as mean ± SD (**P < 0.01 as compared with NC).
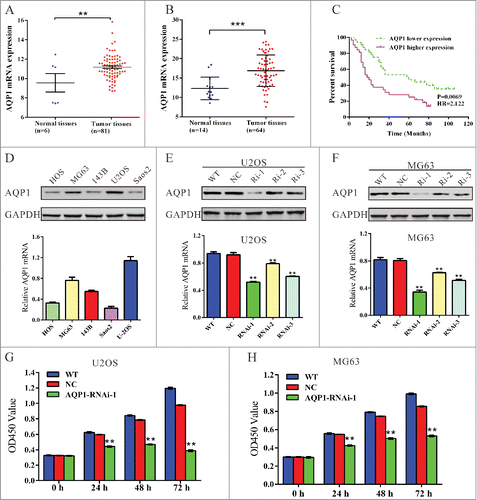
Then, we investigated the correlation between AQP1 expression and prognosis of the patients with osteosarcoma. Kaplan-Meier analysis showed that the overall survival time of lower-AQP1-expressing was notably higher than that of higher-AQP1-expressing patients (). Our data indicated that AQP1 expression was upregulated in osteosarcoma patients which correlates with poor osteosarcoma patient survival.
Knockdown of AQP1 suppressed the proliferation of osteosarcoma cells
We evaluated the expression level of AQP1 in 5 osteosarcoma cells HOS, MG63, 143B, U2OS and Saos2 by real-time PCR and western blot. Two cell lines, MG63 and U2OS, showed higher AQP1 mRNA and protein expression, while the other 3 cell lines, HOS, 143B and Saos2, showed lower mRNA and protein expression (). Therefore, MG63 and U2OS cells were chosen for the following assays.
To investigate the functions of AQP1 on osteosarcoma, we knockdown its expression in osteosarcoma cells by RNA interference (RNAi). Three pairs of shRNA (AQP1-Ri-1, AQP1-Ri-2 and AQP1-Ri-3) targeting human AQP1 and negative control (NC, a non-specific scramble shRNA) were cloned into a lentiviral plasmid. The recombinant lentivirus was then packaged in HEK293T cells and used to infect MG63 and U2OS cells. The silencing effect of the shRNA was evaluated by Western blotting and real-time PCR (). Our results indicated that AQP1-Ri-1 was the most efficient one, with a knockdown efficiency of about 50%. Thus, AQP1-Ri-1was chosen for the further assays.
Knockdown of AQP1 through transduction of AQP1-shRNA virus into U2OS or MG63 cells resulted in decreased cell growth rate compared with corresponding control (). Thus, these results showed that AQP1 had proliferation-promoting properties in osteosarcoma cells.
Knockdown of AQP1 repressed G1/S cell cycle transition and induced apoptosis in osteosarcoma cells
We then determined the possible effect of AQP1 knockdown on cell cycle progression. PI staining and flow cytometry analysis () revealed that the population of U2OS cells infected with AQP1-Ri-1 virus in G0/G1 phase was significantly increased by 30.9 % (P < 0.05), and the population of S phase cells was notably decreased, compared with cells infected with scramble shRNA virus (NC). Similar results were obtained in MG63 cells (). These results suggested that AQP1 promoted G1/S cell cycle transition in osteosarcoma cells.
Figure 2. Knockdown of AQP1 repressed cell proliferation by attenuating G1/S phase transition and induced cell apoptosis in osteosarcoma cells. U2OS and MG63 cells were infected with indicated virus and 48 hours later cells were collected. (A, B) Cell cycle profile was analyzed using flow cytometry. Data were based on at least 3 independent experiments, and shown as mean ± SD. (C, D) Cells were double-stained with Annexin V-FITC/PI and apoptosis rates was analyzed using flow cytometry. (E, F) Protein levels of cleaved caspase 3, Bax and Bcl2 were detected by Western blot. Represented figures were shown in the left panel, while quantitative densitometry of Western blot results were shown in the right panel. Data were based on at least 3 independent experiments, and shown as mean ± SD. WT: wild type cells; NC: scrambled shRNA virus infected cells; AQP1-Ri-1: AQP1-shRNA-1 virus infected cells (**P < 0.01 as compared with NC).
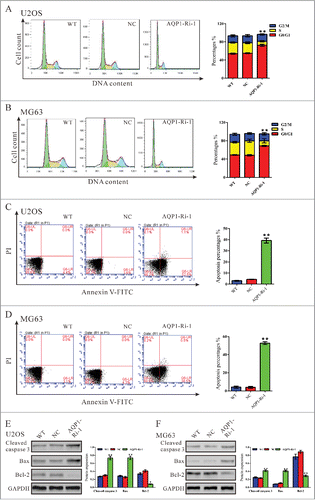
We also evaluated the apoptotic function of AQP1 in U2OS and MG63 cells by Annexin V-FITC/PI staining assay. As shown in , flow cytometry analysis revealed that knockdown of AQP1 in U2OS cells significantly induced cell apoptosis compared with corresponding scramble shRNA (NC, 39.17 ± 1.31% V.S. 4.30 ± 0.12%). Similar results were obtained in MG63 cells (). Western blot was then performed to detect apoptosis-related protein. As shown in , knockdown of AQP1 resulted in a significant reduction in the level of the antiapoptotic protein Bcl−2 with a notable increase in the level of proapoptotic protein Bax and cleaved caspase 3. These results indicated that the proliferation-promoting function of AQP1 was most likely mediated by promoting G1/S cell cycle transition and inhibiting apoptosis.
Silencing of AQP1 inhibited the metastasis of osteosarcoma cells
Metastasis begins with the invasion of tumor cells into the surrounding host tissue. The invasive tumor cells must first alter cell-to-cell adhesion and cell adhesion to the extracellular matrix (ECM).Citation19 We wondered whether AQP1 regulated metastasis of osteosarcoma cells and evaluated the effects of AQP1 on cell adherent () and cell invasive ability (). The adherent ability to fibronectin was significantly inhibited in osteosarcoma cells by AQP1 knockdown. The number of adherent AQP1-Ri-1 cells was 57% of that of NC cells when U2OS cells were used. Similar results were obtained in MG63 cells. These data suggested a role of AQP1 in osteosarcoma cell adhesion.
Figure 3. Silencing of AQP1 inhibited the metastasis of osteosarcoma cells and reduced tumor growth in vivo. U2OS and MG63 cells were infected with indicated virus. (A, B) Cell adhesion was analyzed by cell adhesion assay. Representative images were shown in (A). Scale bar, 100 μm. Quantitative results of cell adhesion assay were shown in (B). Data were based on at least 3 independent experiments, and shown as mean ± SD. (C, D) U2OS and MG63 cells were infected with indicated virus and cell invasion was analyzed in Matrigel-coated transwell chambers. Representative images were shown in (C). Scale bar, 100 μm. Quantitative results of cell adhesion assay were shown in (D). Data were based on at least 3 independent experiments, and shown as mean ± SD. WT: wild type cells; NC: scrambled shRNA virus infected cells; AQP1-Ri-1: AQP1-shRNA-1 virus infected cells (**P < 0.01, as compared with NC). (E) Protein levels of Twist, Snail and E-cadherin were detected by Western blot. Represented figures were shown in the left panel, while quantitative densitometry of Western blot results were shown in the right panel. (F–I) MG63 cells infected with shRNA control (siNC) or AQP1-Ri-1 were subcutaneously injected in athymic nude mice. (F) At day 45, mice were sacrificed and tumors were weighted. Tumor growth was significantly reduced in AQP1 knockdown tumors (**P < 0.01). (G) Photograph of tumors derived from NC and AQP1-Ri-1 cells in nude mice was shown on the upper panel. Weights of tumors was shown on the lower panel. (H) Tumor diameter was evaluated for 45 days; (I) Survival of nude mice bearing MG63 cell xenografts. The survival time of nude mice inoculated with AQP1-Ri-1 cells was significantly longer (P < 0.05) than that injected with NC cells (n = 15).
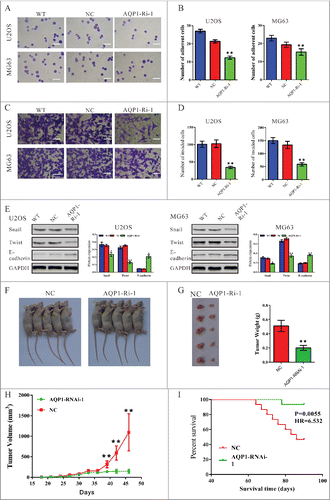
We also investigated whether AQP1 affected the invasive ability of osteosarcoma cells by transwell assay (). As shown in , transfection of AQP1-shRNA construct into U2OS or MG63 cells significantly reduced the cell invasion ability compared with scramble shRNA (NC). We then detected the expression of mesenchymal–epithelial transition (EMT) markers by western blot. Silencing of AQP1 expression led to a significant decrease in levels of Snail and Twist, and an obvious increase in E-cadherin expression (). These data suggested that AQP1 promoted osteosarcoma cell invasion.
AQP1 knockdown inhibited tumor growth in nude mice xenograft model
Next, we determined whether knockdown of AQP1 in osteosarcoma cells could reduce tumor growth in vivo. According to our previous experiences, although both MG63 and U2OS cells form tumors in nude mice, MG63 cells had stronger tumorigenesis ability than U2OS cells. Thus, MG63 cells were selected for in vivo tumorigenicity assay. MG63 cells infected with scramble shRNA control or AQP1-shRNA were subcutaneously injected in athymic nude mice respectively, and tumor volumes were measured for 45 d As shown in , the AQP1-Ri-1 treated tumors grew slower than the control tumors in mice and the volume as well as weight was less than half of control tumors. These data suggested that knockdown of AQP1 inhibited tumor growth in nude mice.
The survival of nude mice inoculated with MG63 cells was then assessed. A total of 2 × 106 NC (n = 15) or AQP1-Ri-1 treated cells (n = 15) were injected into nude mice to evaluate the effect of AQP1-Ri-1 on the mortality rate. As depicted in , the survival time of nude mice inoculated with AQP1-Ri-1 treated cells was significantly longer than that injected with NC cells (P < 0.01).
AQP1 was positively correlated with TGF-β signaling pathway and focal adhesion molecules
The exact pathway that AQP1 may regulate in osteosarcoma cancers remains unclear. To probe the AQP1-associated pathways on an unbiased basis, we performed GSEA using osteosarcoma data from the NCBI Gene Expression Omnibus database (Access ID: GSE42352). Among all the 188 predefined ‘KEGG pathways’ gene sets, TGF-β signaling pathway () and focal adhesion molecules () was identified to be closely correlated with AQP1 expression. Gene expression of TGF-β1, TGF-β2, RhoA and LAMB2 was determined both in mRNA and protein levels (). The expression of detected genes was remarkably decreased after the downregulation of AQP1.
Figure 4. TGF-β signaling pathway and focal adhesion molecules were positively correlated with AQP1 expression. (A, B) GSEA was performed using GSE42352 data set. TGF-β signaling pathway and focal adhesion molecules were identified with the strongest association with AQP1-higher expression. The expression of TGF-β pathway related genes and focal adhesion molecules was evaluated by Western blot (C, E) and real-time PCR (D, F) in U2OS and MG63 cells. WT: wild type cells; NC: scrambled shRNA virus infected cells; AQP1-Ri-1: AQP1-shRNA-1 virus infected cells (**P < 0.01, as compared with NC).
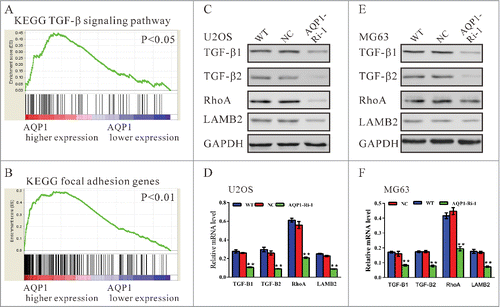
Discussion
In the present study, the clinical data showed that AQP1 was high-expressed in osteosarcoma tissues, which was associated with overall survival of osteosarcoma patients. The in vitro experiments showed that knockdown of AQP1 in osteosarcoma cancer cells strongly inhibited transforming ability and tumorigenicity of osteosarcoma cells in nude mice. These data indicated that AQP1 may serve as a useful prognosis marker and potential target for treatment of osteosarcoma.
Altered expression of AQP1 has been previously observed in several cancers. In consistent with these findings, our present data firstly showed that AQP1 was high-expressed in osteosarcoma tissues (). Previous studies have revealed that overexpression of AQP1 stimulates the proliferation and anchorage-independent growth of NIH-3T3 cells, but decreases the apoptosis of NIH-3T3 cellsCitation20 and Chinese hamster ovary (CHO) cells.Citation21 In the present study, we found that knockdown of AQP1 in 2 osteosarcoma cell lines, U2OS and MG63, significantly reduced cell growth rate (), inhibited G1/S cell cycle transition and promoted cell apoptosis (). The in vivo tumor formation study further indicated that knockdown of AQP1 in osteosarcoma cells reduced the progress of tumor formation and prolonged the survival time of nude mice bearing tumor xenografts ().
The exact pathway that AQP1 may regulate in osteosarcoma remains unclear. Our GSEA data indicated that AQP1 overexpression was positively correlated with the TGF-β signaling pathway and focal adhesion molecules (). The TGF-β signaling pathway has been considered as a promoter of tumor progression, EMT and invasion. In response to elevated TGF-β levels, the tumor cell becomes more migratory and invasive.Citation22 Here, we showed that AQP1 knockdown in osteosarcoma cells significantly decreased their invasion capability, which was associated with altered expression of EMT markers () and impaired expression of TGF-β1 and TGF-β2, thus suggesting that AQP1 may promote the metastasis of osteosarcoma cells through regulating TGF-β-induced EMT pathway. Moreover, RhoA, which is required for the formation and maintenance of focal adhesions, was reported up-regulated in several cancers. The expression level of RhoA may be positively correlated with the progress of these carcinomas, suggesting that RhoA may play an important role in tumorigenesis and tumor progression.Citation23-26 The malignant phenotype in gastric cancer cellsCitation27 and breast cancer cellsCitation28 can be reversed by the inhibition of RhoA expression. Additionally, LAMB2 has been implicated in a wide variety of biological processes including cell adhesion, differentiation, migration and metastasis.Citation29 In the present study, we observed that AQP1 knockdown decreased the expression of RhoA and LAMB2 (). We speculated that AQP1 may perform its biological function in osteosarcoma cells through regulating TGF-β signaling pathway and focal adhesion molecules.
In summary, we found that AQP1 was overexpressed in osteosarcoma and AQP1 expression level was associated with patients' survival rate. Thus, inhibition of AQP1 in tumor tissues might provide a therapeutic strategy. Our study provides for the first time that AQP1 played a key role in the proliferation, apoptosis and metastasis of osteosarcoma cells, and AQP1 might regulate these biological progress through TGF-β and focal adhesion signal pathways, thus may provide useful information for targeted therapy.
Materials and Methods
Patients and tissue samples
From 2009 to 2012, 14 patients with bone cysts and 64 conventional osteosarcoma patients admitted to Department of Orthopedics, Shanghai tenth People's Hospital were enrolled in this study. All patients have complete clinical and pathological follow-up data. Normal bone tissues were also collected as negative controls. These normal bone tissues were resected within at least 5 cm of the tumor margin when the patients underwent definitive surgery. Ethical approval for the study was provided by the independent ethics committee, the Shanghai tenth People's Hospital. Informed and written consent was obtained from all patients or their advisers according to the ethics committee guidelines.
Cell culture
MG63, HOS, 143B, Saos2, U2OS and HEK293T cells were purchased from American Type Culture Collection (Rockville, MD, USA). MG63, HOS, Saos2, 143B and HEK293T cells were grown in DMEM Medium (life technology, Carlsbad, CA, USA) with 10% fetal bovine serum (FBS, life technology) and 1% antibiotic (penicillin/streptomycin, life technology). U2OS cells was grown in RPMI 1640 medium with 10% FBS (life technology) and 1% antibiotic (penicillin/streptomycin).
Reverse transcription and real-time PCR
Total RNA was extracted using TRIzol Reagent (Invitrogen) according to the manufacturer's instructions. One μg total RNA was reverse transcribed using with cDNA synthesis kit (Thermo Fisher Scientific, Rockford, IL, USA) according to the manufacturer's instructions. Real-time PCR was performed using a standard SYBR Green PCR kit (Thermo, Rockford, IL, USA) protocol on ABI 7300 (Applied Biosystem, Foster City, CA USA) thermal cycler. GAPDH was served as an internal control. The primers used were list as follows: AQP1 (NM_001185060.1, F: 5′- GCCATTTAGAGGGTGAAG-3′; R: 5′-TGACAAGAGGGAGTAGAG-3′), TGF-β1 (NM_000660.4, F: 5′-GACTACTACGCCAAGGAGGTC-3′; R: 5′- GAGAGCAACACGGGTTCAG-3′), TGF-β2 (NM_001135599.2, F: 5′- AGAGCAGAAGGCGAATGG −3′; R: 5′- AAAGTGCAGCAGGGACAG −3′), RhoA (NM_001664.2, F: 5′- GAGTGTTCAGCAAAGACCAAAG-3′; R: 5′-TTGCAGCAAGGTTTCACAAG-3′), LAMB2 (NM_002292.3, F: 5′- CCGAAGGCTATGGACTATGAC-3′; R: 5′- CTCAAGGCAGACAGGATTAGG 3′) and GAPDH (NM_001256799.1, F: 5′-CACCCACTCCTCCACCTTTG-3′; R: 5′-CCACCACCCTGTTGCTGTAG-3′). All reactions were conducted using the following cycling parameters, 95°C for 10 min, followed by 40 cycles of 95°C for 15 s, 60°C for 45 s. The gene expression was calculated using the ΔΔ Ct method. All data represent the average of 3 replicates.
Western blotting
Treated and untreated MG63 and U2OS cells were harvest and washed twice with PBS and lysed in ice-cold radio immunoprecipitation assay buffer (RIPA, JRDUN Biotechnology, Shanghai, China) with freshly added 0.01% protease inhibitor cocktail (Sigma, St. Louis, MO USA) and incubated on ice for 30 minutes. Cell lysis was centrifuged at 13,000 rpm for 10 minutes at 4°C and the supernatant (20-30μg of protein) was run on SDS-PAGE gel and transferred electrophoretically to a nitrocellulose membrane (Millipore, Bredford, USA). The blots were blocked with 5% skim milk, followed by incubation with antibodies against AQP1 (Abcam, Cambridge, MA USA, Cat# Ab168387), LAMB2 (Abcam, Cat# ab 151735), TGF-β1 (Abcam, Ab92486), TGF-β2 (Abcam, Ab66045), RhoA (Abcam, Ab68826), Bax (Santa Cruz Biotech., Santa Cruz, CA, USA, Sc-493), Bcl2 (Santa Cruz Biotech., Sc-492), cleaved caspase 3 (CST Biotech., Danvers, MA, USA, Cat#9661), Twist (Abcam, ab175430), E-cadherin (Santa Cruz Biotech., Sc-9989), Snail(CST Biotech., Cat#3895) or GAPDH (CST Biotech. Cat#5174). Blots were then incubated with horseradish peroxidase-conjugated goat anti-mouse secondary antibody (Beyotime, Cat#A0208) or goat anti-rabbit secondary antibody (Beyotime, Cat#A0216) and visualized using enhanced chemiluminescence (ECL, Millipore).
Vector construction
pLKO.1, psPAX2 and pMD2.G were purchased from Addgene. Three shRNAs targeting human AQP1 mRNA (AQP1-Ri-1: AAGGAGAAATACCCATGTTAC; AQP1-Ri-2: GAGGCTGATTCCTCTCATTTC; AQP1-Ri-3: CAGCTTTGAAGGCTGGATTCT) were cloned into a lentiviral vector (PLKO.1). A non-specific scramble shRNA sequence (CCTAAGGTTAAGTCGCCCTCG) was used as negative control. The constructs were then transfected into HEK293T cells with lentiviral packaging vectors (psPAX2 and pMD2.G) by using lipofectamine 2000 (Invitrogen) according to the manufacture's instruction. Viruses were collected 48 h after transfection and used to infect U2OS cells and MG63 cells. After 48 hours, the cells were processed for real-time PCR and Western blot.
Cell proliferation assay
MG63 and U2OS2 cells (1˜5 × 103) were seeded in 96-well plates and cell proliferation were measured with CCK-8 Assay Kit (Dojindo Lab, Kumamoto, Japan) according to manufacturer's protocol. Briefly, at indicated time point, CCK8 solution (10 µl in 100µl DMEM medium) was added to each well and incubated for 1 h. Optical density values (OD) at wavelength 450 nm was measured by a microplate reader (Bio-Rad Laboratories Inc., Hercules, CA USA). All experiments were run in triplicates and repeated at least 3 times.
Cell cycle distribution assay
Propidium iodide (PI) staining was used to analyze DNA content. Cells were harvested 48 hours with or without infection and labeled with PI using previously described methods.Citation30 Briefly, cells were resuspended in PBS and fixed with 70% ethanol. After treatment with ribonuclease (Sigma, St. Louis, MO USA) for 15 min at 37°C, PI (0.05 mg/ml, Sigma) was added to the cells, followed by incubation at room temperature in the dark for 30 min. DNA content was then analyzed using a FACScan instrument equipped with FACStation running Cell Quest software (Becton Dickinson, San Jose, CA USA).
Cell apoptosis assay
The percentage of cells actively undergoing apoptosis was determined by double stained with Annexin V-fluorescein isothiocyanate (FITC) and PI. With or without viral infection for 48 hours, cells were harvested and then double-labeled with Annexin V-FITC and PI (Becton Dickinson) as described by the manufacturer. Cells were analyzed using a FACScan instrument equipped with FACStation running Cell Quest software (Becton Dickinson).
Cell adhesion assay
The adhesion assay was performed in 12-well plates. The plates were pre-coated with 1 ml of fibronectin (5 μg/ml) for 2 h at room temperature. Cells were infected with indicated viral 48 h before the assay was performed. Cell were seeded into the coated plates at a density of 105 cells per well and allowed to adhere at 37°C for 1 h. Nonadherent cells were washed off with phosphate-buffered saline (PBS) and fixed in 4% paraformaldehyde and stained with 0.2% crystal violet. The number of adherent cells determined as described previously.Citation31
In vitro invasion assay
The upper well of the transwell (Corning, NY USA) was coated with Matrigel (BD Biosciences, San Jose, CA USA) at 37°C in a 5% CO2 incubator for 1 h. Indicated cells were serum starved for 24 h, and then 500 μl of cell suspension containing 105 cells/ml were placed in the upper compartment of the chamber. Culture medium supplemented with 10% FBS (750 μl) was added into the lower well of the chamber. The plates were incubated for 48 hr. At the end of the incubation, the cells on the upper surface of the filter were completely removed by wiping with a cotton swab. Cells that migrated into the lower well were washed with PBS, fixed in 4% paraformaldehyde and stained by 0.2% crystal violet. The invading cells were observed under the microscope at 200 × magnification and counted in the 3 fields per experiments.
In vivo tumorigenicity assay
BALB/c nude mice aged 4-5 weeks old were purchased from Shanghai Laboratory Animal Company (SLAC, Shanghai). For each cell line, 2 × 106 cells were resuspended in DMEM medium and inoculated subcutaneously into the left flank of each of the 5 nude mice. Tumor formation was monitored every 3 or 4 d by measuring the largest and the smallest diameter of the formed tumors, and the volume of the tumors was calculated using the following formula: volume = 1/2 × (largest diameter) × (smallest diameter)2. After the mice were killed, the tumors were recovered and the wet weights of each tumor were examined. The experiment was performed under the Institute's guidelines for animal experiments.
Gene set enrichment analysis (GSEA)
Osteosarcoma dataset was downloaded from the NCBI Gene Expression Omnibus database (www.ncbi.nlm.nih.gov/geo/, Access ID: GSE42352). To further investigate the biological pathways involved in osteosarcoma pathogenesis through AQP1 pathway, we performed a gene set enrichment analysis (GSEA). GSEA is a method of analyzing and interpreting microarray and such data using biological knowledge.Citation32 GSEA was performed by using GSEA version 2.0 from the Broad Institute at MIT. The data set GSE42352 was analyzed by GSEA. In this study, GSEA firstly generated an ordered list of all genes according to their correlation with AQP1 expression, and then a predefined gene set (signature of gene expression upon perturbation of certain cancer-related gene) receives an enrichment score (ES), which is a measure of statistical evidence rejecting the null hypothesis that its members are randomly distributed in the ordered list. The expression level of AQP1 was used as phenotype label, and “Metric for ranking genes” was set to Pearson Correlation. All other basic and advanced fields were set to default. The KEGG gene sets biological process database (c2.KEGG.v4.0) from the Molecular Signatures Database–Msig DB (http://www.broad.mit.edu/gsea/msigdb/index.jsp) were used for enrichment analysis.
Statistical analysis
All data are presented as means ± SD. Statistical significance was determined by 2-sided Student's t test. Overall survival time of patients was analyzed by the Kaplan-Meier survival curves and log-rank nonparametric test. P < 0.05 (*) was considered significant.
Disclosure of Potential Conflicts of Interest
No potential conflicts of interest were disclosed.
Reference
- Damron TA, Ward WG, Stewart A. Osteosarcoma, chondrosarcoma, and Ewing's sarcoma: National cancer data base report. Clin Orthop Relat Res 2007; 459:40-7; PMID:17414166; http://dx.doi.org/10.1097/BLO.0b013e318059b8c9
- Ottaviani G, Jaffe N. The epidemiology of osteosarcoma. Cancer Treat Res 2009; 152:3-13; PMID:20213383; http://dx.doi.org/10.1007/978-1-4419-0284-9_1
- Rytting M, Pearson P, Raymond AK, Ayala A, Murray J, Yasko AW, Johnson M, Jaffe N. Osteosarcoma in preadolescent patients. Clin Orthop Relat Res 2000:39-50; PMID:10810461; http://dx.doi.org/10.1097/00003086-200004000-00007
- Bacci G, Ferrari S, Bertoni F, Ruggieri P, Picci P, Longhi A, Casadei R, Fabbri N, Forni C, Versari M, et al. Long-term outcome for patients with nonmetastatic osteosarcoma of the extremity treated at the istituto ortopedico rizzoli according to the istituto ortopedico rizzoli/osteosarcoma-2 protocol: an updated report. J Clin Oncol 2000; 18:4016-27; PMID:11118462
- Provisor AJ, Ettinger LJ, Nachman JB, Krailo MD, Makley JT, Yunis EJ, Huvos AG, Betcher DL, Baum ES, Kisker CT, et al. Treatment of nonmetastatic osteosarcoma of the extremity with preoperative and postoperative chemotherapy: a report from the children's cancer group. J Clin Oncol 1997; 15:76-84; PMID:8996127
- Ferguson WS, Goorin AM. Current treatment of osteosarcoma. Cancer Invest 2001; 19:292-315; PMID:11338887; http://dx.doi.org/10.1081/CNV-100102557
- Verkman AS. More than just water channels: unexpected cellular roles of aquaporins. J Cell Sci 2005; 118:3225-32; PMID:16079275; http://dx.doi.org/10.1242/jcs.02519
- Chen Y, Tachibana O, Oda M, Xu R, Hamada J, Yamashita J, Hashimoto N, Takahashi JA. Increased expression of aquaporin 1 in human hemangioblastomas and its correlation with cyst formation. J Neuro-Oncol 2006; 80:219-25; PMID:17077939; http://dx.doi.org/10.1007/s11060-005-9057-1
- Endo M, Jain RK, Witwer B, Brown D. Water channel (aquaporin 1) expression and distribution in mammary carcinomas and glioblastomas. Microvasc Res 1999; 58:89-98; PMID:10458924; http://dx.doi.org/10.1006/mvre.1999.2158
- Longatti P, Basaldella L, Orvieto E, Dei Tos A, Martinuzzi A. Aquaporin(s) expression in choroid plexus tumours. Pediatric Neurosurg 2006; 42:228-33; PMID:16714863; http://dx.doi.org/10.1159/000092359
- Mazal PR, Susani M, Wrba F, Haitel A. Diagnostic significance of aquaporin-1 in liver tumors. Hum Pathol 2005; 36:1226-31; PMID:16260277; http://dx.doi.org/10.1016/j.humpath.2005.09.002
- Moon C, Soria JC, Jang SJ, Lee J, Obaidul Hoque M, Sibony M, Trink B, Chang YS, Sidransky D, Mao L. Involvement of aquaporins in colorectal carcinogenesis. Oncogene 2003; 22:6699-703; PMID:14555983; http://dx.doi.org/10.1038/sj.onc.1206762
- Saadoun S, Papadopoulos MC, Davies DC, Bell BA, Krishna S. Increased aquaporin 1 water channel expression in human brain tumours. Br J Cancer 2002; 87:621-3; PMID:12237771; http://dx.doi.org/10.1038/sj.bjc.6600512
- Li Q, Zhang B. Expression of aquaporin-1 in nasopharyngeal cancer tissues. J Otolaryngol Head Neck Surg 2010; 39:511-5; PMID:20828513
- Oshio K, Binder DK, Liang Y, Bollen A, Feuerstein B, Berger MS, Manley GT. Expression of the aquaporin-1 water channel in human glial tumors. Neurosurgery 2005; 56:375-81; discussion −81; PMID:15670385; http://dx.doi.org/10.1227/01.NEU.0000148904.57841.6B
- Saadoun S, Papadopoulos MC, Hara-Chikuma M, Verkman AS. Impairment of angiogenesis and cell migration by targeted aquaporin-1 gene disruption. Nature 2005; 434:786-92; PMID:15815633; http://dx.doi.org/10.1038/nature03460
- Hara-Chikuma M, Verkman AS. Aquaporin-1 facilitates epithelial cell migration in kidney proximal tubule. J Am Soc Nephrol 2006; 17:39-45; PMID:16319186; http://dx.doi.org/10.1681/ASN.2005080846
- Hu J, Verkman AS. Increased migration and metastatic potential of tumor cells expressing aquaporin water channels. FASEB J 2006; 20:1892-4; PMID:16818469; http://dx.doi.org/10.1096/fj.06-5930fje
- Mareel M, Oliveira MJ, Madani I. Cancer invasion and metastasis: interacting ecosystems. Virchows Arch 2009; 454:599-622; PMID:19471961; http://dx.doi.org/10.1007/s00428-009-0784-0
- Hoque MO, Soria JC, Woo J, Lee T, Lee J, Jang SJ, Upadhyay S, Trink B, Monitto C, Desmaze C, et al. Aquaporin 1 is overexpressed in lung cancer and stimulates NIH-3T3 cell proliferation and anchorage-independent growth. Am J Pathol 2006; 168:1345-53; PMID:16565507; http://dx.doi.org/10.2353/ajpath.2006.050596
- Jablonski EM, Webb AN, McConnell NA, Riley MC, Hughes FM, Jr. Plasma membrane aquaporin activity can affect the rate of apoptosis but is inhibited after apoptotic volume decrease. Am J Physiol Cell Physiol 2004; 286:C975-85; PMID:14644770; http://dx.doi.org/10.1152/ajpcell.00180.2003
- Wakefield LM, Roberts AB. TGF-beta signaling: positive and negative effects on tumorigenesis. Curr Opin Genet Dev 2002; 12:22-9; PMID:11790550; http://dx.doi.org/10.1016/S0959-437X(01)00259-3
- Abraham MT, Kuriakose MA, Sacks PG, Yee H, Chiriboga L, Bearer EL, Delacure MD. Motility-related proteins as markers for head and neck squamous cell cancer. Laryngoscope 2001; 111:1285-9; PMID:11568556; http://dx.doi.org/10.1097/00005537-200107000-00027
- Horiuchi A, Imai T, Wang C, Ohira S, Feng Y, Nikaido T, Konishi I. Up-regulation of small GTPases, RhoA and RhoC, is associated with tumor progression in ovarian carcinoma. Laborat Invest 2003; 83:861-70; PMID:12808121; http://dx.doi.org/10.1097/01.LAB.0000073128.16098.31
- Kamai T, Arai K, Tsujii T, Honda M, Yoshida K. Overexpression of RhoA mRNA is associated with advanced stage in testicular germ cell tumour. BJU Int 2001; 87:227-31; PMID:11167647; http://dx.doi.org/10.1046/j.1464-410x.2001.02030.x
- Kamai T, Tsujii T, Arai K, Takagi K, Asami H, Ito Y, Oshima H. Significant association of Rho/ROCK pathway with invasion and metastasis of bladder cancer. Clin Cancer Res 2003; 9:2632-41; PMID:12855641
- Liu N, Bi F, Pan Y, Sun L, Xue Y, Shi Y, Yao X, Zheng Y, Fan D. Reversal of the malignant phenotype of gastric cancer cells by inhibition of RhoA expression and activity. Clin Cancer Res 2004; 10:6239-47; PMID:15448013; http://dx.doi.org/10.1158/1078-0432.CCR-04-0242
- Pille JY, Denoyelle C, Varet J, Bertrand JR, Soria J, Opolon P, Lu H, Pritchard LL, Vannier JP, Malvy C, et al. Anti-RhoA and anti-RhoC siRNAs inhibit the proliferation and invasiveness of MDA-MB-231 breast cancer cells in vitro and in vivo. Mol Ther 2005; 11:267-74; PMID:15668138; http://dx.doi.org/10.1016/j.ymthe.2004.08.029
- Scheele S, Nystrom A, Durbeej M, Talts JF, Ekblom M, Ekblom P. Laminin isoforms in development and disease. J Mol Med 2007; 85:825-36; PMID:17426950; http://dx.doi.org/10.1007/s00109-007-0182-5
- Henry MK, Lynch JT, Eapen AK, Quelle FW. DNA damage-induced cell-cycle arrest of hematopoietic cells is overridden by activation of the PI-3 kinase/Akt signaling pathway. Blood 2001; 98:834-41; PMID:11468186; http://dx.doi.org/10.1182/blood.V98.3.834
- Silletti S, Paku S, Raz A. Autocrine motility factor and the extracellular matrix. I. Coordinate regulation of melanoma cell adhesion, spreading and migration involves focal contact reorganization. Int J Cancer 1998; 76:120-8; PMID:9533771; http://dx.doi.org/10.1002/(SICI)1097-0215(19980330)76:1%3c120::AID-IJC19%3e3.0.CO;2-G
- Subramanian A, Tamayo P, Mootha VK, Mukherjee S, Ebert BL, Gillette MA, Paulovich A, Pomeroy SL, Golub TR, Lander ES, et al. Gene set enrichment analysis: a knowledge-based approach for interpreting genome-wide expression profiles. Proc Natl Acad Sci U S A 2005; 102:15545-50; PMID:16199517; http://dx.doi.org/10.1073/pnas.0506580102