Abstract
In hepatocellular carcinoma (HCC), there exists a highly tumorigenic subset of cells defined by high expression of CD44 and CD133 that has been reported to contain cancer stem-like cells (CSCs). Krüppel-like factor 5 (KLF5) regulates many factors involved in cell cycle, migration, inflammation, angiogenesis and stemness and has cancer-promoting effects in some cancers. While some reports have indicated that KLF5 may have important roles in regulation of CSCs, what role, if any, KLF5 plays in regulation of CSCs in HCC remains to be elucidated. Flow cytometric analysis of CD44 and CD133 in HCC cell lines revealed subpopulations of CD44High/CD133High and CD44Low/CD133Low cells. We subsequently sorted these subpopulations and identified KLF5 as a gene that is significantly upregulated in CD44High/CD44High cells via RNA sequencing using next generation sequencing technology. Moreover, KLF5 overexpression enriched the CD44High/CD133High subpopulation and, consistent with the up-regulation of CD44High/CD133High cells, KLF5 overexpressing cells were more resistant to anti-cancer drugs and displayed enhanced colony-formation capacity. By contrast, knock-down of KLF5 by siRNA diminished the CD44High/CD133High subpopulation. When KLF5 was acetylated by TGF-β1, the KLF5-mediated CD44High/CD133High subpopulation enrichment was abrogated. Oppositely, ectopic expression of an acetylation-deficient KLF5 mutant further increased CD44High/CD133High subpopulations as compared to cell expressing wild-type KLF5. These findings provide novel mechanistic insight into a pivotal role for KLF5 in the regulation of CSCs in HCC.
Abbreviations
5FU | = | 5-fluorouracil |
CDDP | = | cisplatin |
CSCs | = | cancer stem-like cells |
FACS | = | fluorescence-activated cell sorting |
HCC | = | hepatocellular carcinoma |
KLF5 | = | Krüppel-like factor 5 |
NGS | = | next generation sequencing |
TGF-β | = | transforming growth factor-β. |
Introduction
Hepatocellular carcinoma (HCC) is the sixth most common cancer and the third most common cause of cancer death worldwide.Citation1 With the emergence of effective curative therapeutic options in HCC, including surgical resection, liver transplantation, radiofrequency ablation and trans-arterial chemoembolization, patient outcomes have dramatically improved. Unfortunately, patients with advanced HCC are often not eligible for such therapeutic interventions, resulting in maintained poor prognosis. This is particularly true of patients with extrahepatic metastases, a condition in which median survival time is estimated at 4.1 to 8.1 months.Citation2-4 Sorafenib is the only effective therapy for advanced HCC, however, the prognosis of advanced HCC patients treated with sorafenib is still not satisfactory.Citation5,6 Thus, further analysis of molecular mechanism of HCC is of critical importance to uncover novel therapeutic avenues against advanced HCC.
Cancer stem-like cells (CSCs) have been identified in many cancers including HCC.Citation7,8 CSCs are highly tumorigenic, metastatic and resistant to chemotherapeutic agents, resulting in dampened clinical response to therapy, tumor metastasis and recurrence, as well as deteriorated prognosis. In HCC, CD44, CD133, EpCAM, CD90 and CD24 are used to detect CSCs Citation9-13 and CSCs have been shown to significantly correlate with malignant biological behavior and worse prognosis.Citation14-16 Although novel CSC-targeting therapeutic strategies are highly sought after, pivotal molecules and pathways regulating CSCs still remain to be elucidated and further studies on CSCs in HCC are necessary.
Kruppel-like factor 5 (KLF5) is a transcription factor containing 3 zinc finger domains and one transactivation domain. The DNA binding site of KLF5 has an acetylation site and acetylation status of KLF5 is important for its transcriptional activity. KLF5 regulates many factors related to cell cycle, angiogenesis, migration, inflammation and stemness.Citation17 In cancers, KLF5 can act as either a tumor suppressor or an oncogene, depending on cancer cell type and context. KLF5 has been reported to have tumor-promoting effects in pancreatic cancer, breast cancer, lymphoblastic leukemia, bladder cancer and gastric cancer.Citation17-19 Furthermore, some reports have indicated critical roles of KLF5 in generation and maintenance of CSCs.Citation19-21 However, the function of KLF5 in HCC and CSCs in HCC has not been established.
In the present study, we performed RNA sequencing using next generation sequencing (NGS) technology to identify key molecules and pathways involved in CSC biology in HCC. We identify KLF5 as a pivotal factor regulating CSCs in HCC.
Results
CD44+/CD133+ cells have cancer stem-like characteristics in hepatocellular carcinoma
Yang et al. examined a relatively large number of patients with HCC and showed that CD44 and CD133 are statistically significant prognostic factors among CSC biomarkers.Citation14 Thus, we utilized CD44 and CD133 to detect HCC CSCs in the present study. Indeed, flow cytometric analysis revealed heterogeneous populations in Huh7 and HepG2 upon staining with anti-CD44 and anti-CD133 (). To characterize subpopulations of HCC cells, we then sorted cells with high expression of CD44 and CD133 (CD44+/CD133+ cells) and those with low expression of CD44 and CD133 (CD44−/CD133− cells) as shown in . As expected, CD44+/CD133+ cells exhibited increased anchorage-independent growth capability and formed significantly more colonies in soft-agar than CD44−/CD133− cells (). Furthermore, in both Huh7 and HepG2 cells, CD44+/CD133+ subpopulations displayed enhanced resistance to the anti-cancer drugs, CDDP and 5FU than their CD44−/CD133− counterparts (). These data indicate that CD44+/CD133+ cells possess traits consistent with cancer stem-like cells in hepatocellular carcinoma.
Figure 1. CD44High/CD133High cells in hepatoma cell lines. (A) Hepatoma cell lines, Huh7 and HepG2, were stained with anti-CD44 and anti-CD133 and analyzed by FACS. Cells expressing high CD44 and CD133 (CD44+/CD133+ cells) and those expressing low CD44 and CD133 (CD44−/CD133− cells) were sorted by FACS Aria III as gated. (B) Anchorage independent growth was examined by soft-agar colony formation assay with the sorted CD44+/CD133+ cells and CD44−/CD133− cells. (*P < 0.05 vs. CD44−/CD133− cells) (C) Sensitivity to anti-cancer drugs, CDDP and 5FU, was analyzed by MTS assay (* P < 0.05 vs. CD44−/CD133− cells).
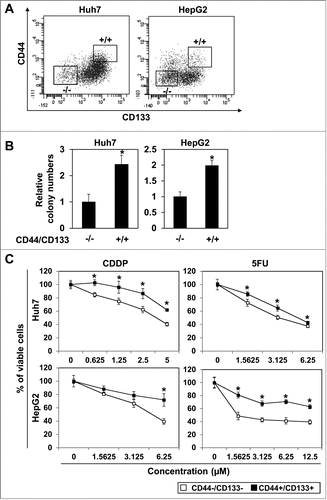
KLF5 is up-regulated in CD44+/CD133+ cells
To explore key molecules and pathways that may regulate CD44+/CD133+ subpopulations, we carried out RNA sequencing using NGS. The cut-off value of RPKM was set as 1.0. Interestingly, CD44+/CD133+ and CD44−/CD133− cells () displayed distinct gene expression profiles. 595 mRNAs were significantly upregulated over 1.5 fold and 465 mRNAs were down-regulated less than −1.5 fold in Huh7 cells (). Among those mRNAs, we focused on KLF5. To validate the result of RNA sequencing, we performed real-time PCR. Indeed, expression of CD44 and CD133 were increased in CD44+/CD133+ cells, and consistent with the result of RNA sequence, KLF5 was significantly up-regulated in CD44+/CD133+ cells in both Huh7 and HepG2 cells ().
Figure 2. KLF5 is significantly upregulated in CD44+/CD133+ cells. RNA sequence was performed using sorted Huh7 cells. Results of principal component analysis (A) and volcano plot (B). (C) Expression levels of CD44, CD133 and KLF5 were validated by real-time RT-PCR in Huh7 and HepG2 cells (* P < 0.05 vs. CD44−/CD133− cells).
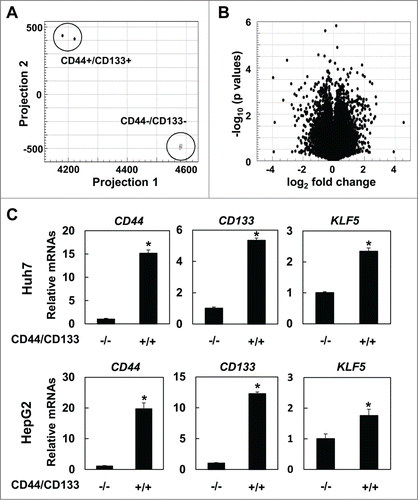
KLF5 is a pivotal transcription factor in maintenance of CD44+/CD133+ cells
To analyze the functional role of KLF5 in regulation of CD44+/CD133+ subpopulations, we knocked down KLF5 using siRNA in Huh7 and HepG2 cells. Indeed, 2 independent siRNA sequences against KLF5 significantly suppressed the expression of KLF5 and the expression of CD44 was also significantly decreased by KLF5 knockdown (). Consistent with the results of real-time PCR, the CD44+/CD133+ subpopulation was significantly suppressed by siRNA against KLF5 in both Huh7 and HepG2 cells (), and the cells knocked down KLF5 by siRNA were significantly more sensitive to 5FU than the control cells (). We next generated Huh7 cells ectopically expressing KLF5 to explore the consequences of KLF5 overexpression upon CD44+/CD133+ CSCs in HCC. Expression of KLF5 was confirmed by real-time RT-PCR () and western blot (). Overexpression of KLF5 enriched the CD44+/CD133+ subpopulation (). Furthermore, KLF5-overexpressing Huh7 cells displayed enhanced soft agar colony formation and resistance to CDDP and 5FU than the control cells (). These results suggest that KLF5 may play critical roles in maintenance of CD44+/CD133+ cancer stem-like cells in hepatocellular carcinoma.
Figure 3. Knockdown of KLF5 decreases CD44+/CD133+ population in hepatoma cell lines. (A) Two independent sequences of siRNA against KLF5 were used to knock-down KLF5 at the concentration of 5nM. Expression levels of KLF5 and CD44 were determined by real-time RT-PCR 48 hours after siRNA transfection. (* P <0.05 vs. scramble siRNA) (B) KLF5 was knocked-down by KLF5-A siRNA at a concentration of 5nM. 48 hours after siRNA transfection, the CD44+/CD133+ subpopulation was analyzed by FACS. A histogram shows relative fold change of CD44+/CD133+ cells in Huh7 and HepG2 cells. (* P<0.05 vs. scramble siRNA) (C) KLF5 was knocked-down by KLF5-A siRNA at a concentration of 5nM. 48 hours after siRNA transfection, sensitivity to 5FU was analyzed by MTS assay (* P<0.05 vs. siKLF5).
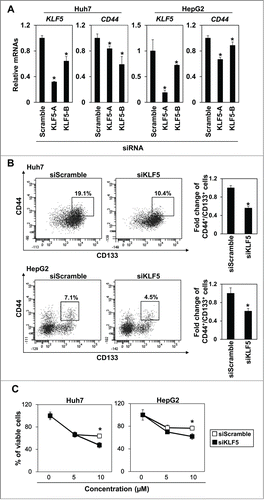
Figure 4. Overexpression of KLF5 increases the CD44+/CD133+ subpopulation and renders cells more resistant to anti-cancer drugs in hepatoma cell lines. KLF5 was transduced by retrovirus-mediated gene transfer into Huh7 cells. Expression level of KLF5 was examined by real-time PCR (A) and western blot (B). (* P < 0.05 vs. empty) (C) The CD44+/CD133+ subpopulation was determined by FACS. A histogram shows relative fold change of CD44+/CD133+ cells in Huh7 cells. (* P < 0.05 vs. empty) (D) KLF5 overexpressing cells and empty control cells were subjected to soft-agar colony formation assay. Chemotherapeutic reagents, CDDP (10 μM) and 5FU (5 μM), were added to the overlaid medium. Histogram shows relative colony numbers (* P < 0.05 vs. empty control).
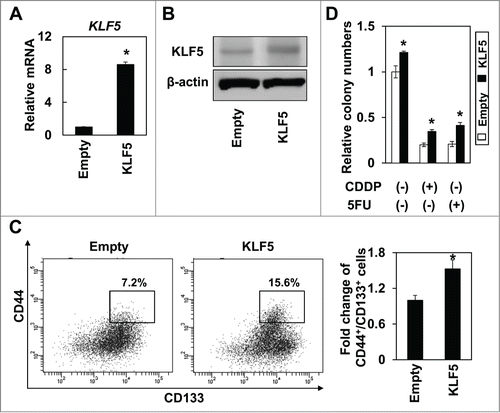
Acetylation status of KLF5 is critical for regulation of CD44+/CD133+ cells
As acetylation of KLF5 has been shown to alter the function of KLF5 in several cell lines,Citation22,23 we next examined the effect of acetylation of KLF5 on CD44+/CD133+ cancer stem-like cells. To induce acetylation of the DNA binding site of KLF5, cells were treated with TGF- β1.Citation23 Although TGF-β1 decreased endogenous KLF5 expression, ectopically expressed KLF5 was unaffected by TGF-β1 (). However, expression level of CD44 was significantly suppressed by TGF-β1 in both control and KLF5-overexpressing cells (). Consistent with decreased CD44 expression, the CD44+/CD133+ subpopulation was significantly suppressed by TGF-β1 in KLF5-overexpressing cells in which KLF5 expression was not affected by TGF-β1 (). These results suggest that acetylation status of the DNA binding site of KLF5 may be important for regulation of CD44+/CD133+ cells. To further explore the role of acetylation of KLF5 in regulation of CD44+/CD133+ cells, we generated Huh7 cells stably overexpressing an acetylation-deficient mutant of KLF5 (KLF5-K369R). Protein levels of the wild type and the mutant KLF5-K369R were similar as confirmed by protein gel blotting (). Induction of CD44 was robustly enhanced by the KLF5-K369R mutant (). The known target genes of KLF5,Citation17 Oct4 and Pim1 were further increased and p15 was further suppressed by the KLF5-K369R mutant than the wild type (). The CD44+/CD133+ subpopulation was also dramatically increased by the KLF5-K369R mutant (). Thus, acetylation status of KLF5 might be critical in the transcriptional activity of KLF5 and the regulation of CD44+/CD133+ cancer stem-like cells in HCC.
Figure 5. TGF-β1 decreases the CD44+/CD133+ subpopulation independent of altered KLF5 expression. Control (Empty) and KLF5 overexpressing (KLF5) Huh7 cells were treated with TGF-β1 (5ng/ml) for 48 hours. (A) Expression of KLF5 was determined by WB. (B) Expression of CD44 was determined by real-time PCR (* P < 0.05 vs. Empty/TGF-β1 (−), # P < 0.05 vs. Empty/TGF-β1 (−), ** P < 0.05 vs. KLF5/TGF-β1 (−)). (C) Expression of CD44/CD133 and percentage of CD44+/CD133+ cells were determined by FACS.
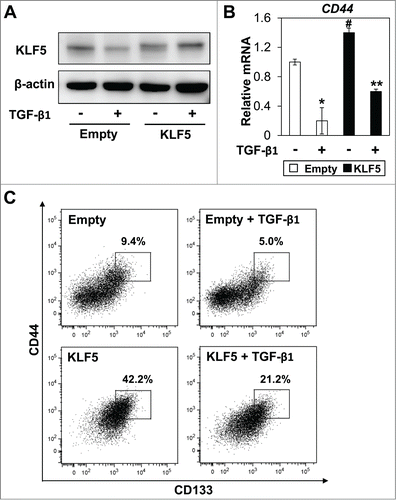
Figure 6. Acetylation status of KLF5 is critical for regulation of the CD44+/CD133+ subpopulation. Wild type (KLF5-WT) and acetylation-deficient (KLF5-K369R) KLF5 were transduced by retrovirus-mediated gene transfer into Huh7 cells. (A) Expression of KLF5 was determined by WB. (B) Expression of CD44 was determined by real-time PCR (* P < 0.05 vs. Empty, # P < 0.05 vs. KLF5-WT). (C) Expression levels of the known KLF5 target genes, Oct4, Pim1 and p15 were determined by real-time PCR (* P < 0.05 vs. Empty, # P < 0.05 vs. KLF5-WT). (D) Expression of CD44/CD133 and percentage of CD44+/CD133+ cells were determined by FACS.
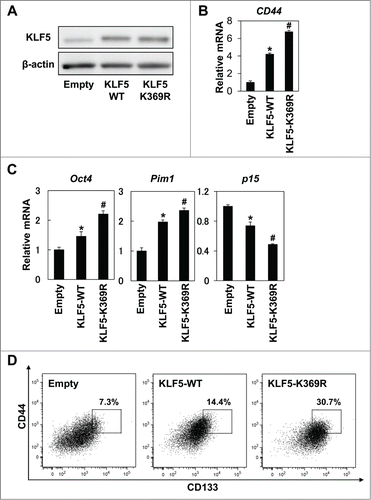
Discussion
In the present study, we have shown that KLF5 is a pivotal transcription factor involved in the regulation of CSCs in HCC. Recent genome-wide sequencing studies have revealed that 10 to 30% of HCC cases have mutations in exon 3 of CTNNB1, a key mediator of Wnt/β-catenin signaling.Citation24 CD44 and Myc are targets of Wnt/β-catenin signaling and aberrant activation of Wnt/β-catenin signaling leads to expansion of CSCs in HCC.Citation11,25,26 Activation of Wnt/β-catenin has been reported to increase expression of EpCAM, one of cancer stem cell markers,Citation27 and Wnt/β-catenin and EpCAM form multiprotein complexes to regulate shared target genes.Citation28 It is obvious that Wnt/β-catenin signaling is important for generation and maintenance of CSCs in HCC. Since it has been reported that deletion of KLF5 from the intestinal mucosa results in attenuation of Wnt/β-catenin signaling,Citation29 KLF5 and Wnt/β-catenin signaling may intersect in the regulation of CSCs in HCC. Inflammation also plays important roles in regulation of CSCs in HCC. IL-6 secreted by tumor-associated macrophages promotes expansion of CD44 positive CSCs via activation of STAT3,Citation30 and IL-8 has been shown to enhance self-renewal and tumor initiation of CSCs through activation of the mitogen-activated protein kinase (MAPK) pathway in HCC.Citation31 Most HCCs arise from chronic inflammation such as viral hepatitis, non-alcoholic steatohepatitis and alcoholic hepatitis. KLF5 might have some roles in hepatitis and further study is necessary to clarify the role of KLF5 in inflammation in regulation of CSCs in HCC.
A role of KLF5 in the regulation of CSCs has not been well established so far. In Helicobacter pylori infection, KLF5 is induced by CDX1 and KLF5 promotes trans-differentiation of gastric epithelial cells into intestinal epithelial cells, leading to carcinogenesis and enrichment of CSCs in gastric cancer.Citation19,20 Nakaya et al. have reported that KLF5 acts as a key regulator of intestinal carcinogenesis at the stem-cell level in their mouse model.Citation32 These reports indicate that KLF5 might play important roles in the regulation of CSCs; however, detailed mechanisms through which KLF5 regulates CSCs have yet to be elucidated. In the present study, we have shown that KLF5 regulates expression of CD44, one of the most important stem cell markers. Although Pagliuca et al. have shown that both CD44 and KLF5 are regulated by miR-143 and miR-145,Citation33 regulation of CD44 by KLF5 has not been reported thus far. Our findings indicate that expression of CD44 is controlled by expression level of KLF5 in overexpression and knockdown experiments (). CD44 interacts with xCT, a light-chain subunit of the cystine-glutamate transporter xc(−), and reduces reactive oxygen species (ROS). CD44 protects CSCs from excessive ROS under stressful conditions, such as rapid tumor growth and metastasis, and maintain CSCs.Citation34,35 Thus, KLF5 may enrich and maintain CSCs via induction of CD44, one of important functional molecules in CSC biology. In the present study, we have not determined if KLF5 directly upregulates expression of CD44. Smith et al. have shown that NF-κB directly up-regulates CD44 expression.Citation36 KLF5 may indirectly regulate CD44 expression through NF-κB, a known KLF5 target gene.Citation37 Further analysis is required to identify the detailed regulatory mechanism of CD44 by KLF5.
Previous reports have shown that post-translational modification of KLF5 dramatically alters transcriptional activity and function of KLF5.Citation17 Phosphorylation of KLF5 increases its transactivation activities,Citation38 ubiquitination of KLF5 by WWP1 or FBW7 regulates its stability,Citation39,40 and sumoylation regulates the nuclear localization of KLF5 by inactivating nuclear export signal.Citation41 Interestingly, acetylation of KLF5 by TGF-β signaling converts the function of KLF5 from tumor suppressive to oncogenic by changing target gene expression profile of KLF5.Citation22,23 Therefore, we hypothesized that acetylation status of KLF5 in addition to expression level might play an essential role in maintaining the malignant properties of CSCs in HCC. The present study indicates that TGF-β1 decreased expression of CD44 at both the mRNA and protein level although the protein expression level of KLF5 was not changed (). In addition, expression of a point mutation in KLF5 at amino acid 369 (KLF5-K369R), resulting in an acetylation-deficient mutant of KLF5, significantly increased CD44 expression compared with wild-type KLF5, where protein expression levels of KLF5-K369R and wild-type KLF5 were equal (). This suggests that KLF5, especially deacetylated KLF5, is important for upregulation of CD44 in HCC. We have shown for the first time that acetylation status of KLF5 is critical for regulation of CSCs in HCC.
In conclusion, we have identified KLF5 as one of the pivotal transcription factors regulating CSCs in HCC. KLF5 upregulates CD44 expression and expands CSCs. Furthermore, acetylation status of KLF5 is critical for regulation of CSCs and deacetylated KLF5 robustly increases subpopulation of CSCs in HCC. Thus, modulation of KLF5 expression and acetylation status may be investigated as potential novel therapeutic targets against CSCs in HCC.
Materials and methods
Cell lines and reagents
Hepatoma cell lines, Huh7 and HepG2 cells were grown in DMEM supplemented with 10% fetal bovine serum, 100 units/ml of penicillin G and 100 µg/ml of streptomycin. Anticancer drugs, cisplatin (CDDP) and 5-fluorouracil (5FU) were purchased from Sigma Aldrich (Saint Louis, MI). Recombinant human TGF-β1 (PHG9214) was purchased from Life Technologies (Carlsbad, CA).
DNA constructs
KLF5 was amplified by PCR using ADV-KLF5 Citation42 as a template with primers 5′- ACCGGATCCGCCACCATGGCTACAAGGGTGCTGAGCATGAG -33′ and 5′- ACCGTCGACTCAGTTCTGGTGCCTCTTCAT-33′. Amplified KLF5 was ligated at the BamHI and SalI sites of pBABE puro and designated as pBABE-KLF5. A point mutation was introduced into KLF5 by PCR using pBABE-KLF5 as a template with primers 5′-AGACGACGCATCCACTACTGCGAT-33′ and 5′-CTCCAAATCGGGGTTACTCCTTCT-33′. Mutated KLF5 was ligated at the BamHI and SalI sites of pBABE puro and designated as pBABE-KLF5-K369R. The DNA binding site of KLF5-K369R is acetylation deficient.
Retrovirus-mediated gene transfer
Replication-incompetent retrovirus expressing KLF5 and KLF5-K369R were produced and transduced as described previously.Citation43,44 Stable cell lines were established by drug selection with 2 μg/ml puromycin for 7 days.
Real-time PCR
RNA was extracted by RNeasy mini kit (QIAGEN, Valencia, CA USA) and cDNA was synthesized using Super Script II (Life Technologies) according to the manufacturer3′s instruction. Real-time PCR was performed with SYBR® Green (QIAGEN) and ABI 7300 real time PCR system (Life Technologies). β-actin was used as an internal control. Primers used for real-time PCR are listed in .
Table 1. Primers used for real-time RT-PCR
Western blotting
Western blotting was carried out as described previously Citation43 using the following primary antibodies at the indicated titers; anti-KLF5 (ab24331, Abcam) (1:1000) and anti-β-actin (AC74; Sigma-Aldrich) (1:5000).
Drug sensitivity assay
Five thousands cells were seeded per well in a 96 well plate. Twenty-four hours after seeding, drugs were added at indicated concentrations. After 72 hours incubation, MTS assay was carried out using CellTiter 96® AQueous One Solution Cell Proliferation Assay (Promega, Fitchburg, Wisconsin) according to the manufacturer3′s instruction. Absorbance (A490 - A630) was measured by Sunrise™ plate reader (F039302, TECAN, Durham, NC) and survival rate was determined.
Soft-agar colony formation assay
Soft agar colony formation assays were performed as described previously.Citation45 In brief, 1 × 103 cells were suspended in 0.67% agarose in growth media and then overlaid on top of 1% agarose in growth media per well. (24 well plate). 500 µl of medium with indicated reagents was added twice a week in each well and grown for 3 weeks. Colonies over 100 µm were counted. The experiments were performed in hexaduplicate.
Flow cytometry and Fluorescence Activated Cell Sorting (FACS)
FACSCantoII (BD Biosciences, San Jose, CA) and FlowJo (Tree Star, Ashland, OR) were used for flow cytometry. Cells were suspended in Hank's balanced salt solution (Invitrogen) containing 1% BSA (Sigma-Aldrich) and stained with APC-anti-CD44 (BD Biosciences) at 1:20 and PE-anti-CD133 (Miltenyi Biotec, Auburn, CA) at 1:20 on ice for 30 min. Dead cells were eliminated by 7-AAD (BD Biosciences) according to the manufacturer3′s instruction. To purify CD44High / CD133High CSCs and CD44Low / CD133Low non-CSCs, cells were sorted using a FACS Aria III (BD Bioscience).
siRNA transfection
Small interfering RNA (siRNA) sequences directed against KLF5 (S104161381 and S204281074) and a non-silencing control sequence (AllStars Negative Control siRNA) (QIAGEN) were purchased from QIAGEN. siRNAs were transfected transiently with Lipofectamine™ RNAi Max reagent (Life Technologies), following the manufacturer's instructions.
RNA sequence
RNA was extracted using RNeasy mini kit (QIAGEN) from sorted CD44+/CD133+ and CD44−/CD133− Huh7 cells. Intact polyadenylated (poly A) mRNAs were enriched by poly A selection using Dynbeads® mRNA DIRECT ™ Micro Kit (Life Technologies). Libraries for RNA sequence were constructed by Ion Total RNA-Seq Kit version 2 (Life Technologies) according to the manufacturer3′s instruction. Quality of the library was checked using Agilent Bioanalyzer 2100 (Agilent Technologies, Waldbronn, Germany). Emulsion PCR was carried out using Ion One Touch™ Duo and Ion One Touch™ ES (Life Technologies) and sequencing was performed using the Ion PGM™ system (Life Technologies), following the manufacturer3′s instruction. Acquired sequences were mapped to homo sapiens Hg19 and analyzed by CLC Genomics Workbench (CLC bio, Aarhus, Denmark). We set a cut-off value of RPKM (reads per kilobase of exon per million mapped sequence reads) Citation46 as 1.0 in the present study.
Statistical analysis
Data from triplicate and hexaduplicate experiments are presented as mean ± SD and were analyzed by 2-tailed Student3′s t test. P < 0.05 was considered significant.
Disclosure of Potential Conflicts of Interest
No potential conflicts of interest were disclosed.
Funding
This study was supported in part by the Japanese Ministry of Education, Culture, Sports, Science and Technology (MEXT) KAKENHI Grant Numbers 25860512, 26460933, and 15K08943, and Japanese Health Labor Sciences Research Grant.
References
- Ferlay J, Shin HR, Bray F, Forman D, Mathers C, Parkin DM. Estimates of worldwide burden of cancer in 2008: GLOBOCAN 2008. Int JCancer J Int du Cancer 2010; 127:2893-917; PMID:21351269; http://dx.doi.org/10.1002/ijc.25516
- Natsuizaka M, Omura T, Akaike T, Kuwata Y, Yamazaki K, Sato T, Karino Y, Toyota J, Suga T, Asaka M. Clinical features of hepatocellular carcinoma with extrahepatic metastases. J Gastroenterol Hepatol 2005; 20:1781-7; PMID:16246200; http://dx.doi.org/10.1111/j.1440-1746.2005.03919.x
- Uchino K, Tateishi R, Shiina S, Kanda M, Masuzaki R, Kondo Y, Goto T, Omata M, Yoshida H, Koike K. Hepatocellular carcinoma with extrahepatic metastasis: clinical features and prognostic factors. Cancer 2011; 117:4475-83; PMID:21437884; http://dx.doi.org/10.1002/cncr.25960
- Yoo DJ, Kim KM, Jin YJ, Shim JH, Ko GY, Yoon HK, Sung KB, Lee JL, Kang YK, Lim YS, et al. Clinical outcome of 251 patients with extrahepatic metastasis at initial diagnosis of hepatocellular carcinoma: does transarterial chemoembolization improve survival in these patients? J Gastroenterol Hepatol 2011; 26:145-54; PMID:21175808; http://dx.doi.org/10.1111/j.1440-1746.2010.06341.x
- Cheng AL, Kang YK, Chen Z, Tsao CJ, Qin S, Kim JS, Luo R, Feng J, Ye S, Yang TS, et al. Efficacy and safety of sorafenib in patients in the Asia-Pacific region with advanced hepatocellular carcinoma: a phase III randomised, double-blind, placebo-controlled trial. Lancet Oncol 2009; 10:25-34; PMID:19095497; http://dx.doi.org/10.1016/S1470-2045(08)70285-7
- Llovet JM, Ricci S, Mazzaferro V, Hilgard P, Gane E, Blanc JF, de Oliveira AC, Santoro A, Raoul JL, Forner A, et al. Sorafenib in advanced hepatocellular carcinoma. N Engl J Med 2008; 359:378-90; PMID:18650514; http://dx.doi.org/10.1056/NEJMoa0708857
- Clevers H. The cancer stem cell: premises, promises and challenges. Nat Med 2011; 17:313-9; PMID:21386835; http://dx.doi.org/10.1038/nm.2304
- Visvader JE, Lindeman GJ. Cancer stem cells: current status and evolving complexities. Cell Stem Cell 2012; 10:717-28; PMID:22704512; http://dx.doi.org/10.1016/j.stem.2012.05.007
- Ma S, Chan KW, Hu L, Lee TK, Wo JY, Ng IO, Zheng BJ, Guan XY. Identification and characterization of tumorigenic liver cancer stem/progenitor cells. Gastroenterology 2007; 132:2542-56; PMID:17570225; http://dx.doi.org/10.1053/j.gastro.2007.04.025
- Yang ZF, Ngai P, Ho DW, Yu WC, Ng MN, Lau CK, Li ML, Tam KH, Lam CT, Poon RT, et al. Identification of local and circulating cancer stem cells in human liver cancer. Hepatology 2008; 47:919-28; PMID:18275073; http://dx.doi.org/10.1002/hep.22082
- Yamashita T, Ji J, Budhu A, Forgues M, Yang W, Wang HY, Jia H, Ye Q, Qin LX, Wauthier E, et al. EpCAM-positive hepatocellular carcinoma cells are tumor-initiating cells with stem/progenitor cell features. Gastroenterology 2009; 136:1012-24; PMID:19150350; http://dx.doi.org/10.1053/j.gastro.2008.12.004
- Zhu Z, Hao X, Yan M, Yao M, Ge C, Gu J, Li J. Cancer stem/progenitor cells are highly enriched in CD133+CD44+ population in hepatocellular carcinoma. Int JCancer J Int du Cancer 2010; 126:2067-78; PMID:19711346; http://dx.doi.org/10.1002/ijc.24728
- Lee TK, Castilho A, Cheung VC, Tang KH, Ma S, Ng IO. CD24(+) liver tumor-initiating cells drive self-renewal and tumor initiation through STAT3-mediated NANOG regulation. Cell Stem Cell 2011; 9:50-63; PMID:21726833; http://dx.doi.org/10.1016/j.stem.2011.06.005
- Yang XR, Xu Y, Yu B, Zhou J, Qiu SJ, Shi GM, Zhang BH, Wu WZ, Shi YH, Wu B, et al. High expression levels of putative hepatic stem/progenitor cell biomarkers related to tumour angiogenesis and poor prognosis of hepatocellular carcinoma. Gut 2010; 59:953-62; PMID:20442200; http://dx.doi.org/10.1136/gut.2008.176271
- Ma YC, Yang JY, Yan LN. Relevant markers of cancer stem cells indicate a poor prognosis in hepatocellular carcinoma patients: a meta-analysis. Eur J Gastroenterol Hepatol 2013; 25:1007-16; PMID:23478672; http://dx.doi.org/10.1097/MEG.0b013e32836019d8
- Schulze K, Gasch C, Staufer K, Nashan B, Lohse AW, Pantel K, Riethdorf S, Wege H. Presence of EpCAM-positive circulating tumor cells as biomarker for systemic disease strongly correlates to survival in patients with hepatocellular carcinoma. Int JCancer J Int du Cancer 2013; 133:2165-71; PMID:23616258; http://dx.doi.org/10.1002/ijc.28230
- Dong JT, Chen C. Essential role of KLF5 transcription factor in cell proliferation and differentiation and its implications for human diseases. Cell Mol Life Sci 2009; 66:2691-706; PMID:19448973; http://dx.doi.org/10.1007/s00018-009-0045-z
- Mori A, Moser C, Lang SA, Hackl C, Gottfried E, Kreutz M, Schlitt HJ, Geissler EK, Stoeltzing O. Up-regulation of Kruppel-like factor 5 in pancreatic cancer is promoted by interleukin-1beta signaling and hypoxia-inducible factor-1alpha. Mol Cancer Res 2009; 7:1390-8; PMID:19671674; http://dx.doi.org/10.1158/1541-7786.MCR-08-0525
- Noto JM, Khizanishvili T, Chaturvedi R, Piazuelo MB, Romero-Gallo J, Delgado AG, Khurana SS, Sierra JC, Krishna US, Suarez G, et al. Helicobacter pylori promotes the expression of Kruppel-like factor 5, a mediator of carcinogenesis, in vitro and in vivo. PloS One 2013; 8:e54344
- Fujii Y, Yoshihashi K, Suzuki H, Tsutsumi S, Mutoh H, Maeda S, Yamagata Y, Seto Y, Aburatani H, Hatakeyama M. CDX1 confers intestinal phenotype on gastric epithelial cells via induction of stemness-associated reprogramming factors SALL4 and KLF5. Proc Natl Acad Sci U S A 2012; 109:20584-9; PMID:23112162; http://dx.doi.org/10.1073/pnas.1208651109
- Dong Z, Yang L, Lai D. KLF5 strengthens drug resistance of ovarian cancer stem-like cells by regulating survivin expression. Cell Prolif 2013; 46:425-35; PMID:23869764; http://dx.doi.org/10.1111/cpr.12043
- Guo P, Zhao KW, Dong XY, Sun X, Dong JT. Acetylation of KLF5 alters the assembly of p15 transcription factors in transforming growth factor-beta-mediated induction in epithelial cells. J Biol Chem 2009; 284:18184-93; PMID:19419955; http://dx.doi.org/10.1074/jbc.M109.007096
- Li X, Zhang B, Wu Q, Ci X, Zhao R, Zhang Z, Xia S, Su D, Chen J, Ma G, et al. Interruption of KLF5 acetylation converts its function from tumor suppressor to tumor promoter in prostate cancer cells. Int JCancer J Int du Cancer 2015; 136:536-46; PMID:24931571
- Shibata T, Aburatani H. Exploration of liver cancer genomes. Nat Rev Gastroenterol Hepatol 2014; 11:340-9; PMID:24473361; http://dx.doi.org/10.1038/nrgastro.2014.6
- Nejak-Bowen KN, Thompson MD, Singh S, Bowen WC, Jr., Dar MJ, Khillan J, Dai C, Monga SP. Accelerated liver regeneration and hepatocarcinogenesis in mice overexpressing serine-45 mutant beta-catenin. Hepatology 2010; 51:1603-13; PMID:20432254; http://dx.doi.org/10.1002/hep.23538
- Akita H, Marquardt JU, Durkin ME, Kitade M, Seo D, Conner EA, Andersen JB, Factor VM, Thorgeirsson SS. MYC activates stem-like cell potential in hepatocarcinoma by a p53-dependent mechanism. Cancer Res 2014; 74:5903-13; PMID:25189530; http://dx.doi.org/10.1158/0008-5472.CAN-14-0527
- Yamashita T, Budhu A, Forgues M, Wang XW. Activation of hepatic stem cell marker EpCAM by Wnt-beta-catenin signaling in hepatocellular carcinoma. Cancer Res 2007; 67:10831-9; PMID:18006828; http://dx.doi.org/10.1158/0008-5472.CAN-07-0908
- Oishi N, Yamashita T, Kaneko S. Molecular biology of liver cancer stem cells. Liver Cancer 2014; 3:71-84; PMID:24944998; http://dx.doi.org/10.1159/000343863
- McConnell BB, Kim SS, Yu K, Ghaleb AM, Takeda N, Manabe I, Nusrat A, Nagai R, Yang VW. Kruppel-like factor 5 is important for maintenance of crypt architecture and barrier function in mouse intestine. Gastroenterology 2011; 141:1302-13, 13 e1-6
- Wan S, Zhao E, Kryczek I, Vatan L, Sadovskaya A, Ludema G, Simeone DM, Zou W, Welling TH. Tumor-associated macrophages produce interleukin 6 and signal via STAT3 to promote expansion of human hepatocellular carcinoma stem cells. Gastroenterology 2014; 147:1393-404; PMID:25181692; http://dx.doi.org/10.1053/j.gastro.2014.08.039
- Tang KH, Ma S, Lee TK, Chan YP, Kwan PS, Tong CM, Ng IO, Man K, To KF, Lai PB, et al. CD133(+) liver tumor-initiating cells promote tumor angiogenesis, growth, and self-renewal through neurotensin/interleukin-8/CXCL1 signaling. Hepatology 2012; 55:807-20; PMID:21994122; http://dx.doi.org/10.1002/hep.24739
- Nakaya T, Ogawa S, Manabe I, Tanaka M, Sanada M, Sato T, Taketo MM, Nakao K, Clevers H, Fukayama M, et al. KLF5 regulates the integrity and oncogenicity of intestinal stem cells. Cancer Res 2014; 74:2882-91; PMID:24626089; http://dx.doi.org/10.1158/0008-5472.CAN-13-2574
- Pagliuca A, Valvo C, Fabrizi E, di Martino S, Biffoni M, Runci D, Forte S, De Maria R, Ricci-Vitiani L. Analysis of the combined action of miR-143 and miR-145 on oncogenic pathways in colorectal cancer cells reveals a coordinate program of gene repression. Oncogene 2013; 32:4806-13; PMID:23128394; http://dx.doi.org/10.1038/onc.2012.495
- Ishimoto T, Nagano O, Yae T, Tamada M, Motohara T, Oshima H, Oshima M, Ikeda T, Asaba R, Yagi H, et al. CD44 variant regulates redox status in cancer cells by stabilizing the xCT subunit of system xc(−) and thereby promotes tumor growth. Cancer Cell 2011; 19:387-400; PMID:21397861; http://dx.doi.org/10.1016/j.ccr.2011.01.038
- Nagano O, Okazaki S, Saya H. Redox regulation in stem-like cancer cells by CD44 variant isoforms. Oncogene 2013; 32:5191-8; PMID:23334333; http://dx.doi.org/10.1038/onc.2012.638
- Smith SM, Lyu YL, Cai L. NF-kappaB affects proliferation and invasiveness of breast cancer cells by regulating CD44 expression. PloS one 2014; 9:e106966; PMID:25184276; http://dx.doi.org/10.1371/journal.pone.0106966
- Chanchevalap S, Nandan MO, McConnell BB, Charrier L, Merlin D, Katz JP, Yang VW. Kruppel-like factor 5 is an important mediator for lipopolysaccharide-induced proinflammatory response in intestinal epithelial cells. Nucleic Acids Res 2006; 34:1216-23; PMID:16500892; http://dx.doi.org/10.1093/nar/gkl014
- Zhang Z, Teng CT. Phosphorylation of Kruppel-like factor 5 (KLF5/IKLF) at the CBP interaction region enhances its transactivation function. Nucleic Acids Res 2003; 31:2196-208; PMID:12682370; http://dx.doi.org/10.1093/nar/gkg310
- Chen C, Sun X, Guo P, Dong XY, Sethi P, Cheng X, Zhou J, Ling J, Simons JW, Lingrel JB, et al. Human Kruppel-like factor 5 is a target of the E3 ubiquitin ligase WWP1 for proteolysis in epithelial cells. J Biol Chem 2005; 280:41553-61; PMID:16223724; http://dx.doi.org/10.1074/jbc.M506183200
- Luan Y, Wang P. FBW7-mediated ubiquitination and degradation of KLF5. World J Biol Chem 2014; 5:216-23; PMID:24921010
- Du JX, Bialkowska AB, McConnell BB, Yang VW. SUMOylation regulates nuclear localization of Kruppel-like factor 5. J Biol Chem 2008; 283:31991-2002; PMID:18782761; http://dx.doi.org/10.1074/jbc.M803612200
- Ohnishi S, Ohnami S, Laub F, Aoki K, Suzuki K, Kanai Y, Haga K, Asaka M, Ramirez F, Yoshida T. Downregulation and growth inhibitory effect of epithelial-type Kruppel-like transcription factor KLF4, but not KLF5, in bladder cancer. Biochem Biophy Res Commun 2003; 308:251-6; PMID:12901861; http://dx.doi.org/10.1016/S0006-291X(03)01356-1
- Natsuizaka M, Ohashi S, Wong GS, Ahmadi A, Kalman RA, Budo D, Klein-Szanto AJ, Herlyn M, Diehl JA, Nakagawa H. Insulin-like growth factor-binding protein-3 promotes transforming growth factor-{beta}1-mediated epithelial-to-mesenchymal transition and motility in transformed human esophageal cells. Carcinogenesis 2010; 31:1344-53; PMID:20513670; http://dx.doi.org/10.1093/carcin/bgq108
- Ohashi S, Natsuizaka M, Yashiro-Ohtani Y, Kalman RA, Nakagawa M, Wu L, Klein-Szanto AJ, Herlyn M, Diehl JA, Katz JP, et al. NOTCH1 and NOTCH3 coordinate esophageal squamous differentiation through a CSL-dependent transcriptional network. Gastroenterology 2010; 139:2113-23; PMID:20801121; http://dx.doi.org/10.1053/j.gastro.2010.08.040
- Ohashi S, Natsuizaka M, Naganuma S, Kagawa S, Kimura S, Itoh H, Kalman RA, Nakagawa M, Darling DS, Basu D, et al. A NOTCH3-mediated squamous cell differentiation program limits expansion of EMT-competent cells that express the ZEB transcription factors. Cancer Res 2011; 71:6836-47; PMID:21890822; http://dx.doi.org/10.1158/0008-5472.CAN-11-0846
- Mortazavi A, Williams BA, McCue K, Schaeffer L, Wold B. Mapping and quantifying mammalian transcriptomes by RNA-Seq. Nat Methods 2008; 5:621-8; PMID:18516045; http://dx.doi.org/10.1038/nmeth.1226