Abstract
IL-15 has been actively investigated for its potential in tumor immunotherapy. To enhance the anti-tumor activity of IL-15, the novel PFC-1 construct was designed, which comprises the following 3 parts: (1) IL-15Rα fused with IL-15 to enhance IL-15 activity, (2) an Fc fragment to increase protein half-life, and (3) an integrin-targeting RGD peptide to enhance tumor targeting. PFC-1 showed tumor cell targeting without compromising IL-15 activity. PFC-1 also had potent anti-tumor activities in xenograft models, suggesting the potential application of this multi-functional fusion protein in tumor therapy.
Abbreviations
CFSE | = | Carboxyfluorescein N-succinimidyl ester |
Fc domain | = | fragment crystallization domain |
HUVEC | = | human umbilical vein endothelial cells |
IL-15 | = | interleukin-15 |
IL-15R | = | interleukin-15 receptor |
NK cell | = | natural killer cell |
PBMC | = | peripheral blood mononuclear cell. |
Introduction
Cytokines play important roles in the regulation of the immune system, including anti-tumor immune responses. A number of cytokines have been shown to have anti-tumor potential. Among these cytokines, interleukin (IL)-15 has been extensively studied as a promising anti-tumor candidate.Citation1,2 IL-15 belongs to the common receptor γ-chain cytokine family that also includes IL-2.Citation3 IL-15 and IL-2 share the β and common γ chains of their receptors (IL-2/15βγ), but bind to different α receptor chains (IL-2Rα/IL-15Rα). In a trans-presentation model, during which IL-15 binds to IL-15Rα expressed on antigen-presenting cells, the IL-15/IL-15 Rα complex then binds to the IL-15βγ complex expressed on nearby effector cells.Citation3,4 Similar to IL-2, IL-15 can stimulate the proliferation of T cells and natural killer (NK) cells, the expansion of cytotoxic T cells and the activation of NK cells. Unlike IL-2, IL-15 is not involved in the activation-induced cell death and maintenance of regulatory T cells,Citation5,6 which can block the therapeutic effects of IL-2. Thus, IL-15 is ranked at the top of the National Cancer Institute's list of agents with great potential for cancer immunotherapy.Citation7
Recent studies have suggested that to achieve biological anti-tumor responses in vivo, high dosages of IL-15 are indispensable.Citation8,9 The IL-15/IL-15Rα complex has been proposed to enhance the activity of IL-15 approximately 50-fold in vivo;Citation10-12 thus, an IL-15/IL-15Rα fusion may perform better than IL-15. Another limitation of IL-15 as a therapeutic agent is its short plasma half-life.Citation13 Diverse attempts have been undertaken to prolong the therapeutic activity of IL-15, such as gene therapy with viral vectors,Citation14 engineering cells to secrete IL-15,Citation15 or fusing IL-15 to another larger protein fragmentCitation16 such as the Fc domain of IgG, which has been widely used to increase the plasma half-life of many proteins.Citation17-19 One potential limitation of these efforts is that the function of IL-15 is systemic and not tumor specific. In response to a long IL-15 half-life, cytotoxic T cells or NK cells are expanded systemically, not only in tumors. As uncontrolled systemic activation of the immune system can be very toxic and lethal, a more desirable therapeutic agent will need to be able to limit its function to tumors and spare other tissues to reduce toxicity.
One approach to the development of tumor-targeting therapeutic agents, such as chemical compounds or large biological molecules, is to use RGD peptides.Citation20,21 RGD (Arg-Gly-Asp)-containing peptides are a group of peptides that display a strong binding affinity and selectivity to integrins, specifically the αvβ3 integrin.Citation22 Integrins are a family of heterodimeric glycoprotein receptors comprising an α and a β subunit, which mediate cell-to-cell and cell-to-matrix interactions. The αvβ3 integrin is frequently over-expressed on many tumor cell types and on endothelial cells involved in tumor-associated angiogenesis.Citation23 Thus, small or large molecules that are conjugated or fused to an RGD peptide can be targeted to tumor tissues. The increased local concentration of the therapeutic reagents can enhance tumor-killing activities and limit toxicity.
In this paper, we constructed PFC-1, a targeting protein that comprises the IL-15/IL-15Rα complex as an effector module, an Fc domain to enhance the protein half-life, and an RGD peptide to target the tumor. PFC-1 can be efficiently expressed and showed potent anti-tumor effects with increased cytotoxic T cells and NK cells in the tumor tissues. These data suggest that PFC-1 is a promising tumor immunotherapeutic agent.
Results
PFC-1 fusion protein strongly stimulates IL-15-dependent cell proliferation in vitro
To generate an immunotherapeutic protein with tumor targeting features, a fusion protein, PFC-1, was constructed (). PFC-1 comprises an IL-15/IL-15Rα complex to stimulate an immune reaction, an Fc domain to enhance protein half-life, and an RGD peptide to specifically target tumors (). The fusion gene (PFC-1) was cloned into the pCDNA3.1(+) vector and then transiently transfected into 293 cells. The protein was purified from cell culture medium using a Protein-A immunoaffinity chromatography method with yields of 30 mg/L. A single band of approximately 60 kDa was observed under reducing conditions () in accordance with the predicted molecular weight of PFC-1, 53.3 kDa. Under non-reducing conditions, the majority of the protein is approximately 120 kDa () with a minor product at approximately 60 kDa and a lesser product at the very top of the gel, suggesting that the majority of the PFC-1 protein is in a dimeric form, likely due to Fc domain dimerization.
Figure 1. In vitro characterization of the PFC-1 fusion protein. (A) Schematic representation of PFC-1. SP, signal peptide; RGD, Arg-Gly-Asp peptide motif; Fc, CH2 and CH3 of human IgG1; IL-15Ra, IL-15Rα sushi domain including the subsequent 12 amino acids from exon 3; L1, SS; L2, G4S; L4, SG2SG4SG3SG4SLQ. (B) Coomassie blue staining of the fusion protein developed from 10% SDS-PAGE under non-reducing (NR) or reducing (R) conditions. (C) Mo7e proliferation stimulated by rhIL-15 and PFC-1. (D) CTLL-2 proliferation stimulated by rhIL-15 and PFC-1. The concentration was calculated according to the molecular weight of a PFC-1 monomer. The data are shown as the mean ± SD of triplicate samples and the lines represent the sigmoidal dose-response curve fit for EC50 determination. The results are representative of at least 3 experiments.
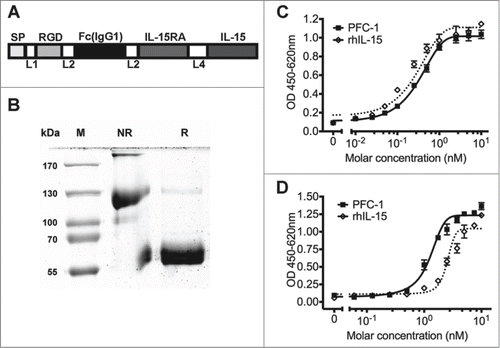
The cytokine activity of PFC-1 was analyzed using a cytokine-dependent cell proliferation assay in CTLL-2 and Mo7e cell lines. CTLL-2 is a murine cytotoxic T lymphocytic cell line with positive expression of both the IL-15Rα chain and the IL-15βγ complex, while Mo7e is a human megakaryocytic leukemic cell line that only expresses the IL-15βγ complex. Proliferation of both cell lines can be induced by the presence of IL-15.Citation6 Similar to IL-15, PFC-1 can stimulate the proliferation of both Mo7e and CTLL-2 cell lines, demonstrating the IL-15 cytokine activity of PFC-1 (). When the molar concentration of PFC-1 was calculated as a monomer in Mo7e cells, rhIL-15 showed a slightly higher cytokine activity than PFC-1 (). PFC-1 worked better than rhIL-15 in CTLL-2 cells, with approximately 2- to 4-fold stronger activity ().
We also constructed PFC-2, a mutant PFC-1 protein. Compared to PFC-1, PFC-2 comprises the IL-15Rα sushi domain, which is 12 amino acids shorter than the IL-15Rα in PFC-1, plus the 72nd amino acid in IL-15 is mutated from an N to a D (IL-15 N72D), which was previously reported to have higher activity.Citation24 PFC-2 was expressed and purified in the same way as PFC-1. PFC-2 activity is weaker with nearly half the potency of PFC-1 in both Mo7e and CTLL-2 cell lines (Fig. S1).
PFC-1 fusion protein stimulates primary immune cell proliferation
To measure the activity of PFC-1 on primary immune cells, PBMCs (Peripheral blood mononuclear cells) were prepared, stained with CFSE and incubated with rhIL-15 or PFC-1 for 6 d. Both rhIL-15 and PFC-1, at either 1 or 10 nM, significantly stimulated the proliferation of PBMCs compared to the control group (). However, different from the Mo7e and CTLL-2 cytokine-dependent proliferation assay, PFC-1 exhibited an approximately 10-fold stronger potency than rhIL-15 in PBMC proliferation, while 10 nM rhIL-15 led to a proliferation rate of 20.71% and 1 nM PFC-1 resulted in a mean value of 22.05% (). The enhanced activity could be due to the higher activity of IL-15/IL-15Rα than IL-15, the extended half-life by the Fc fragment, or both.
Figure 2. PBMC proliferation stimulation in vitro by PFC-1. CFSE-labeled PBMCs were incubated with various concentrations of rhIL-15 or PFC-1 for 6 d Proliferation of PBMCs was assessed by flow cytometry. (A) Representative FACS images of PBMC proliferation are shown. (B) Quantitative analysis of PBMC proliferation stimulation by rhIL-15 or PFC-1. The concentration was calculated according to the molecular weight of a PFC-1 monomer. The data are shown as the mean ± standard deviation of triplicate samples. The results are representative of 3 experiments. The t test was used for statistical analysis with p < 0.05.
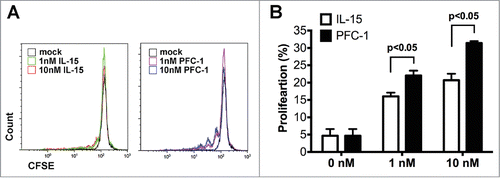
PFC-1 binding and co-localization with αVβ3 integrin
To confirm that PFC-1 can indeed bind to tumor cells or tumor endothelial cells through cell surface integrins, HUVEC endothelial cells or SKOV3 ovarian cancer cells were used to check PFC-1 binding as both of these cell lines have high expression of αVβ3 integrins.Citation21,25 Flow cytometry analysis suggested that both HUVEC (, left panel) and SKOV3 cells (, left panel) are indeed αVβ3-integrin positive. Though weaker than the anti-αVβ3 integrin mAb, PFC-1 can in fact bind to HUVEC ( right panel) and SKOV3 cells (, right panel). The binding is specific as no binding was observed for the colon cancer cell line LS174T, which is αVβ3-integrin negative (). Furthermore, imaging analysis showed that PFC-1 co-localized with the anti-αVβ3 integrin mAb on both HUVEC and SKOV3 cells (), demonstrating that the binding of PFC-1 to HUVEC and SKOV-3 was αVβ3-integrin specific, likely via the RGD motif.
Figure 3. PFC-1 binding to αVβ3 integrin (CD51/61) and co-localization with αVβ3 integrin (CD51/61). Flow cytometry analysis of PFC-1 binding to αVβ3 integrin on (A) HUVEC and (B) SKOV3 cells. Anti-αVβ3 integrin (CD51/61) is in the left panel and PFC-1 is in the right panel. (C). Confocal microcopy of PFC-1 co-localization with αVβ3 integrin (CD51/61) in HUVEC cells (upper panel) and SKOV3 cells (lower panel). The bar scale represents 20 μm.
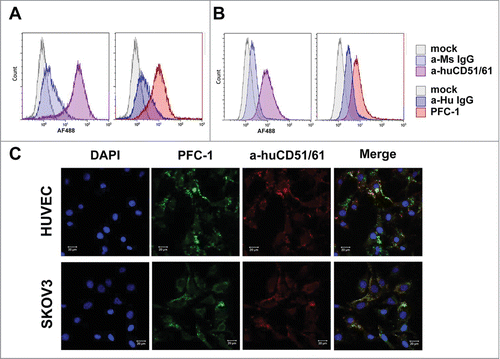
PFC-1 has potent anti-tumor effects in vivo
To further evaluate the in vivo anti-tumor efficacy of PFC-1, C57BL/6 mice transplanted with B16F10 melanoma cells were treated with 5 or 20 μg PFC-1 or vehicle. Five μg PFC-1 treatment was able to restrain tumor growth by 70%, while the 20 μg PFC-1 treatment was able to completely (100%) abrogate tumor growth (), demonstrating the potent anti-tumor effects of PFC-1.
Figure 4. In vivo anti-tumor activity of PFC-1. C57BL/6 mice transplanted with B16F10 melanoma cells received 5 or 20 μg PFC-1 or vehicle (200 μl per mouse, i.p.) on indicated days (arrow) every 3 d for 2 treatments in total. Then, tumor volumes were measured correspondingly. The data are shown as the mean ± SD; n = 5˜8; **, p < 0.01, t test.
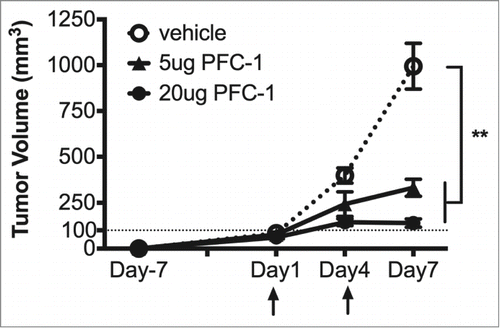
To analyze the effect of PFC-1 on immune cells, C57BL/6 mice were transplanted with B16F10 melanoma cells. After large tumor burdens were established, 10 μg PFC-1 () was injected intravenously for 2 consecutive days. Potent in vivo tumor growth blockage was observed (). On day 5, the tumor volume had shrunk by 25% after PFC-1 treatment () compared to the initial tumor volume. With an additional PFC-1 treatment on day 6, tumor volumes decreased to 54% of the initial tumor volume ().
Figure 5. PFC-1 in vivo anti-tumor efficacy on established large B16F10 tumor xenografts. (A) C57BL/6 mice with large established B16F10 tumor (1000 mm3) xenografts received 10 μg/mouse PFC-1 or vehicle (200 µl) i.v treatment son indicated days (arrow) and tumor volumes were measured correspondingly. (B) The CD8+T cell ratio (top) and NK cell ratio (bottom) were measured in the blood, spleen and tumor after mice were euthanized on day 7. (C) The change in the CD44 ratio in the CD8+T cells and NK cells was also measured in the blood, spleen and tumor by flow cytometry. The data are shown as the mean ± SD; n = 5˜8; **, p < 0.01; ***, p < 0.005, t test.
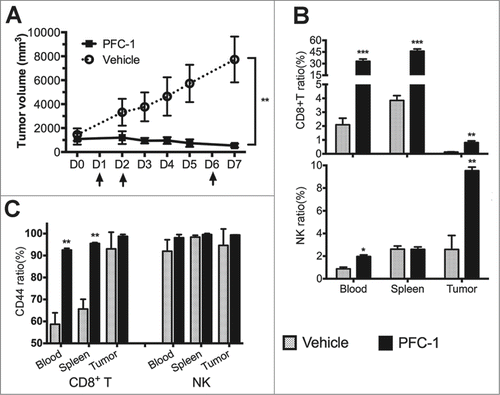
Flow cytometry was performed to analyze lymphocytes isolated from the peripheral blood, spleen and tumors of mice treated with PFC-1 or the vehicle groups. A steep increase in the number of CD8+T cells was observed in the peripheral blood, spleen and tumors of mice treated with PFC-1 (, top). A significant increase in CD44, a T cell activation marker, on CD8+T cells was also observed in peripheral blood, splenocytes, and tumor-infiltrating lymphocytes (), suggesting that PFC-1 not only increased the population but also activated CD8+T cells. PFC-1 was also able to mobilize more NK cells in tumors ( bottom). Similar observations were also observed in cells treated with PFC-2 with some slight differences (Fig. S3). All of the data suggest that PFC-1 and PFC-2 stimulate immune cells to kill tumor cells.
PFC-1 blocks tumor metastasis
IL-15 and IL-15/IL-15Rα have been demonstrated to block tumor metastasis. To evaluate the effect of PFC-1 on tumor metastasis, C57BL/6 mice were intravenously injected with B16F10 melanoma cells. Lung metastasis developed rapidly in these mice (). A single intraperitoneal administration of 10 μg PFC-1 effectively reduced the average lung metastasis node number from 61, the average in the vehicle group, to 11.4 in the treated group, a decrease of more than 80%, suggesting that PFC-1 can effectively block tumor metastasis.
Figure 6. Effect of PFC-1 on the B16F10 melanoma metastasis model. C57BL/6 mice were i.v. injected with 5*105 B16F10 melanoma cells on day 0 and a single dosage of 10 μg PFC-1 or equal volume of vehicle was administered. On day 21, the lungs were removed and metastasis nodes were counted. (Top) A representative figure of the lungs and (bottom) the lung metastasis number. The data are shown as the mean ± SD, n = 5 , t test.
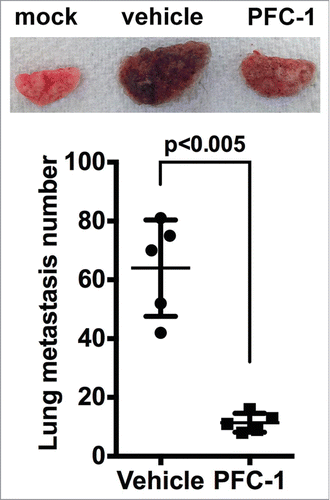
Discussion
In this study, we reported a cytokine fusion protein that combines a tumor-targeting RGD peptide, an IgG Fc fragment, and IL-15/IL-15Rα into a single molecule. In vitro and in vivo experiments suggest that each component of the fusion protein retains its specific function: the anti-tumor activity of IL-15/IL-15Rα, the tumor cell binding ability of the RGD peptide, and the ability of the Fc fragment to facilitate purification and likely enhance half-life in vivo. The combinatorial properties of this fusion protein provide a superior immunotherapeutic agent for anti-tumor therapy.
IL-15 is an ideal candidate for cancer immunotherapy,Citation7 as it stimulates the proliferation of T cells and NK cells, expands cytotoxic T cells and activates NK cells without affecting the activation-induced cell death activity of regulatory T cells.Citation5,6 However, as an anti-tumor agent, high dosageCitation8,9 and short plasma half-lifeCitation13 limit the potential of IL-15.
To enhance the activity of IL-15, we first fused IL-15Rα with IL-15. The fusion of IL-15 to IL-15Rα has been reported to maximize its stimulatory properties in vitroCitation26,27 and enhance the anti-tumor activities of IL-15 in various mouse tumor models.Citation9 However, the IL-15 activity in PFC-1 is complicated. Using CTLL-2 and Mo7e cells, PFC-1 stimulated cell proliferation similarly to IL-15 (). In PBMC proliferation assays, PFC-1 showed fold10- higher activity than IL-15 (). The topological orientation of the IL-15/IL-15Rα fusion in PFC-1 is different than in previous studies.Citation28 Due to the presence of the Fc fragment, the PFC-1 protein dimerized (). It is not clear how these structural changes affect IL-15 activity. However, the similar or higher activity of PFC-1 suggested that IL-15 activity is not compromised. The enhanced activity observed in the PBMC proliferation assays may be related to the enhanced PFC-1 half-life due to the presence of the Fc fragment, which also facilitates the easy purification of the fusion protein using protein A affinity purification.
To maximize the IL-15/IL-15Rα activity, we also made PFC-2, an IL-15 mutant (N72D), which has displayed increased IL-15 activity. However, with the PFC-2 construct, probably due to the deletion of the 12 exon 3 amino acids directly after the IL-15Rα sushi domain, lower cell proliferation activities were observed in Mo7e and CTLL-2 cells (Fig. S3), which is similar to previous reports.Citation26,27 Similar or slightly lower in vivo activities were also observed in PFC-2 compared to PFC-1 (Fig. S3).
Another potential issue with IL-15 is its non-specific systematic distribution, which can lead to toxicity in undesired tissues. To increase the local concentration of IL-15 in tumor tissues, an RGD peptide was fused to the N-terminal end of PFC-1. RGD (Arg-Gly-Asp) peptides display a strong binding affinity and selectivity for integrins, especially the αvβ3 integrin,Citation22 which is overexpressed on many tumor cells and on endothelial cells involved in tumor-associated angiogenesis.Citation23 PFC-1 demonstrated potent αvβ3-integrin binding to the αvβ3-integrin positive tumor cell line SKOV-3 and the endothelial HUVEC cells, while not binding to the αvβ3-integrin negative cell line LS174T (), suggesting that the RGD peptide will likely enhance tumor targeting. However, due to the low tolerated dosage of PFC-1 in mouse experiments, we were unable to identify PFC-1 enrichment in tumor samples, specifically at sites of tumor angiogenesis.
In conclusion, the combinatorial fusion protein PFC-1 retains the activity of IL-15 and shows strong anti-tumor effects. This fusion protein overcomes the limiting issues of IL-15 by increasing its half-life and tumor targeting, which can reduce systemic exposure to IL-15 and reduce toxicity. This molecule has the potential to be a powerful immunotherapeutic agent for malignant tumors.
Materials and Methods
Antibodies
Recombinant human IL-2 (AF-200-02) and granulocyte-macrophage colony-stimulating factor (300-03) were purchased from Peprotech. Recombinant human IL-15 (247-IL-105) was purchased from R&D Systems. Anti-MsCD3e (145-2c11)-PerCP, anti-MsCD8a(53-6.7)-FITC, anti-MsNK1.1(PK136)-FITC, anti-MsCD44(IM7)-PE and anti-MsCD122(TM-Bta1)-PE were purchased from BD PharMingen. Anti-human CD51/61 (αVβ3 integrin) purified mAb was purchased from eBioscience. Goat anti-human IgG(H+L)-AlexaFluor 488, goat anti-mouse IgG(H+L)-AlexaFluor 488 and goat anti-Mouse IgG(H+L)-AlexaFluor 647 were purchased from Invitrogen.
Cell lines and animals
SKOV-3, CTLL-2, and Mo7e cells were purchased from the Shanghai Cell Bank. HUVEC cells were kindly gifted by Dr. Gao Huile from Sichuan University.Citation21 CTLL-2 cells were cultured in RPMI 1640 supplemented with 20% fetal bovine serum (FBS), 30 ng/ml IL-2, and 1% non-essential amino acids. Mo7e cells were cultured in RPMI 1640 supplemented with 10% fetal bovine serum (FBS), 10 ng/ml GM-CSF, and 1% non-essential amino acids. SKOV-3 and HUVEC cells were cultured in DMEM supplemented with 10% FBS. PBMC were isolated from the buffy coat of healthy donors using Ficoll-Paque plus (GE health) and cultured in RPMI-1640 supplemented with 10% FBS. C57bl/6 mice were purchased from the Animal Experiment Facility of Sun Yat-sen University. Human blood collection, animal care and animal experiments were approved by Sun Yat-sen University.
Generation of the recombinant protein
To generate the recombinant protein PFC-1, PFC-1 was cloned into the pcDNA3.1 vector with a mouse kappa chain signal peptide. The plasmid was transiently transfected to 293 cells. One hundred ml of media were collected after 3 d of culture. The PFC-1 protein was purified with a Protein-A-agarose affinity purification protocol.
Cytokine-dependent cell proliferation assay
To measure cytokine-dependent cell proliferation, CTLL-2 and Mo7e cells were harvested in their logarithmic growth phase, washed twice with PBS and incubated for 4 h in assay medium (RPMI 1640 supplemented with 10% FBS and 1%NEAA) for cytokine starvation at 37 °C and 5% CO2. During the incubation, IL-15 and PFC-1 were diluted to an initial concentration of 10 nM in the assay medium, followed by serial dilutions. After a 4-h incubation, cells were collected and a cell suspension (2 × 104 cells/well) was seeded immediately into corresponding wells and incubated at 37 °C and 5% CO2 for 48 or 72 h with CTLL-2 or Mo7e cells, respectively. After a 48-h or 72-h incubation period, CCK-8 assay (Dojindo) was performed to measure the amount of live cells.
CFSE labeling of PBMC and proliferation assay
To measure PBMC proliferation, PBMCs were freshly prepared by Ficoll centrifugation, adjusted to 2 × 106 cells/ml, and then stained with 5 μM CFSE (eBioscience) according to the manufacturer's instructions. Stained PBMCs (5 × 105 cell/ml) were incubated with 1 nM or 10 nM of rhIL-15 and PFC-1 for 6 d PBMC proliferation was assessed by flow cytometry using a Cytomic FC500 (Beckman Coulter) and analyzed using the Kaluza software (Beckman Coulter).
Integrin binding assay
To test the binding of PFC-1 to integrins, HUVEC and SKOV3 cells were cultured in DMEM supplemented with 10% FBS to 80% confluence. Cells were trypsinized, adjusted to 4 × 105 cells/ml and incubated in complete DMEM medium for 2 hr at 37 °C. Cells were then washed with PBS and aliquoted to a concentration of 2 × 105 cell/500 µl. Then, the cells were stained with 2 μg of anti-human CD51/61 (αVβ3 integrin) or PFC-1, followed by incubation with a fluorophore-conjugated secondary antibody before being subjected to flow cytometry analysis.
Confocal microscopy
SKOV3 and HUVEC cells were cultured on 30-mm glass-bottom dishes (In Vitro Scientific) to 70% confluence. The cells were then washed with cold PBS and fixed with 4% paraformaldehyde. The fixed cells were incubated with 2 μg of purified anti-human CD51/61 (αVβ3 integrin) or PFC-1 at room temperature for 1 hr, followed a second incubation at room temperature for 1 hour with either goat anti-mouse IgG-AlexaFluor 647 or goat anti-human IgG-AlexaFluor 488, respectively. The nuclei were counterstained with DAPI. Zeiss LSM710 confocal microscopy was used to observe the cells.
In vivo efficacy studies
For the anti-tumor studies, 4-6 week old female C57bl/6 mice were injected with 5 × 105 B16F10 mouse melanoma tumor cells in 200 µl PBS at the right flank. Ten to 12 d later, once tumors reached 5–8 mm in diameter (day 0), mice were treated with a tail-vein injection of 10 μg PFC-1/-2 protein in 200 µl PBS on day 1, day 2, and day 6. Tumor diameters were measured on a daily basis with an electro caliber. Tumor volumes were calculated using the following equation: tumor volume = length × width × width / 2.
To study the effect of PFC-1 on tumor metastasis, 4-6 week old female C57BL/6 mice were inoculated intravenously with 5 × 105 B16F10 cells on day 0. On day 1, a single dosage of 10 μg PFC-1 or an equivalent volume (100 µl) of PBS was administered by intra-peritoneal injection. On day 21, mice were euthanized. The mouse lungs were removed, washed with PBS, and preserved in 10% formaldehyde. Lung metastatic tumors were counted under a binocular microscope (Leica M125).
Phenotypic characterization by flow cytometry
To characterize mouse lymphocytes, peripheral blood was collected from the orbital vein. Spleens were removed and splenocytes were processed into single cell suspensions and filtered through a 70-µm nylon mesh (BD). Tumor tissues were also removed and gently disrupted with forceps followed by enzymatic digestion with 0.2 mg/ml collagenase IV (Sigma) and 0.1 mg/ml DNase I (Sigma) in RPMI-1640 at 37°C for 15 min. The released cells were collected and the remaining tumor tissue was subjected to further processing by incubation in fresh digestion medium for an additional 25 min at 37°C. Single-cell suspensions were filtered with a 70-um nylon mesh. Cell samples from the blood, spleen and tumor tissues were then stained with the corresponding antibodies at room temperature for 30 min, protected from light. Samples were then washed with PBS twice and adjusted to an appropriate volume for flow cytometry analysis.
Disclose of Potential Conflicts of Interest
No potential conflicts of interest were disclosed.
Supplementary Figures
Download MS Word (695.8 KB)Acknowledgments
We are grateful to Dr. Mengfeng Li and Dr. Jiang Li at the Sun Yat-Sen University School of Medicine, and Dr. Wei Xie at the Sun Yat-Sen University School of Life Sciences for their technical support and valuable discussions.
Supplemental Material
Supplemental data for this article can be accessed on the publisher's website.
Funding
The financial support for this work was from the Introduced Innovative R&D Team Leadership of Guangdong Province (PR China) (2011Y038).
References
- Smyth MJ, Cretney E, Kershaw MH, Hayakawa Y. Cytokines in cancer immunity and immunotherapy. Immunol Rev 2004; 202:275-93; PMID:15546400; http://dx.doi.org/10.1111/j.0105-2896.2004.00199.x
- Fehniger TA, Cooper MA, Caligiuri MA. Interleukin-2 and interleukin-15: immunotherapy for cancer. Cytokine Growth Factor Rev 2002; 13:169-83; PMID:11900992; http://dx.doi.org/10.1016/S1359-6101(01)00021-1
- Dafne M. Targeted cancer immunotherapy: mimicking physiological trans-presentation of IL-15. Oncoimmunology 2012; 1:1213-4; PMID:23170284; http://dx.doi.org/10.4161/onci.20824
- Dubois S, Mariner J, Waldmann TA, Tagaya Y. IL-15Ralpha recycles and presents IL-15 In trans to neighboring cells. Immunity 2002; 17:537-47; PMID:12433361; http://dx.doi.org/10.1016/S1074-7613(02)00429-6
- Rochman Y, Spolski R, Leonard WJ. New insights into the regulation of T cells by gamma(c) family cytokines. Nat Rev Immunol 2009; 9:480-90; PMID:19543225; http://dx.doi.org/10.1038/nri2580
- Kermer V, Baum V, Hornig N, Kontermann RE, Muller D. An antibody fusion protein for cancer immunotherapy mimicking IL-15 trans-presentation at the tumor site. Mol Cancer Ther 2012; 11:1279-88; PMID:22491823; http://dx.doi.org/10.1158/1535-7163.MCT-12-0019
- Cheever MA. Twelve immunotherapy drugs that could cure cancers. Immunol Rev 2008; 222:357-68; PMID:18364014; http://dx.doi.org/10.1111/j.1600-065X.2008.00604.x
- Kobayashi H, Carrasquillo JA, Paik CH, Waldmann TA, Tagaya Y. Differences of biodistribution, pharmacokinetics, and tumor targeting between interleukins 2 and 15. Cancer Res 2000; 60:3577-83; PMID:10910071
- Bessard A, Sole V, Bouchaud G, Quemener A, Jacques Y. High antitumor activity of RLI, an interleukin-15 (IL-15)-IL-15 receptor α fusion protein, in metastatic melanoma and colorectal cancer. Mol Cancer Ther 2009; 8:2736-45; PMID:19723883; http://dx.doi.org/10.1158/1535-7163.MCT-09-0275
- Rubinstein MP, Kovar M, Purton JF, Cho JH, Boyman O, Surh CD, Sprent J. Converting IL-15 to a superagonist by binding to soluble IL-15R{α}. Proc Natl Acad Sci USA 2006; 103:9166-71; PMID:16757567; http://dx.doi.org/10.1073/pnas.0600240103
- Stoklasek TA, Schluns KS, Lefrançois L. Combined IL-15/IL-15Ralpha immunotherapy maximizes IL-15 activity in vivo. J Immunol 2006; 177:6072-80; PMID:17056533; http://dx.doi.org/10.4049/jimmunol.177.9.6072
- Dubois S, Patel HJ, Zhang M, Waldmann TA, Muller JR. Preassociation of IL-15 with IL-15R α-IgG1-Fc enhances its activity on proliferation of NK and CD8+/CD44high T cells and its antitumor action. J Immunol 2008; 180:2099-106; PMID:18250415; http://dx.doi.org/10.4049/jimmunol.180.4.2099
- Kukita T, Arima N, Matsushita K, Arimura K, Ohtsubo H, Sakaki Y, Fujiwara H, Ozaki A, Matsumoto T, Tei C. Autocrine and/or paracrine growth of adult T-cell leukaemia tumour cells by interleukin 15. Br J Haematol 2002; 119:467-74; PMID:12406087; http://dx.doi.org/10.1046/j.1365-2141.2002.03813.x
- Yu YL, Wei CW, Chen YL, Chen MH, Yiang GT. Immunotherapy of breast cancer by single delivery with rAAV2-mediated interleukin-15 expression. Int J Oncol 2010; 36:365-70; PMID:20043070; http://dx.doi.org/10.3892/ijo_00000603
- Vera M, Razquin N, Prieto J, Melero I, Fortes P, Gonzalez-Aseguinolaza G. Intratumoral injection of dendritic cells transduced by an SV40-based vector expressing interleukin-15 induces curative immunity mediated by CD8+ T lymphocytes and NK cells. Mol Ther 2005; 12:950-9; PMID:15921960; http://dx.doi.org/10.1016/j.ymthe.2005.03.030
- Kaspar M, Trachsel E, Neri D. The antibody-mediated targeted delivery of interleukin-15 and GM-CSF to the tumor neovasculature inhibits tumor growth and metastasis. Cancer Res 2007; 67:4940-8; PMID:17510424; http://dx.doi.org/10.1158/0008-5472.CAN-07-0283
- Roopenian DC, Akilesh S. FcRn: the neonatal Fc receptor comes of age. Nat Rev Immunol 2007; 7:715-25; PMID:17703228; http://dx.doi.org/10.1038/nri2155
- Czajkowsky DM, Hu J, Shao Z, Pleass RJ. Fc-fusion proteins: new developments and future perspectives. EMBO Mol Med 2012; 4:1015-28; PMID:22837174; http://dx.doi.org/10.1002/emmm.201201379
- Kontermann RE. Antibody-cytokine fusion proteins. Arch Biochem Biophys 2012; 526:194-205; PMID:22445675; http://dx.doi.org/10.1016/j.abb.2012.03.001
- Aguzzi MS, Fortugno P, Giampietri C, Ragone G, Capogrossi MC, Facchiano A. Intracellular targets of RGDS peptide in melanoma cells. Mol Cancer 2010; 9:84; PMID:20412563; http://dx.doi.org/10.1186/1476-4598-9-84
- Gao H, Xiong Y, Zhang S, Yang Z, Cao S, Jiang X. RGD and interleukin-13 peptide functionalized nanoparticles for enhanced glioblastoma cells and neovasculature dual targeting delivery and elevated tumor penetration. Mol Pharm 2014; 11:1042-52; PMID:24521297; http://dx.doi.org/10.1021/mp400751g
- Chen K, Chen X. Integrin targeted delivery of chemotherapeutics. Theranostics 2011; 1:189-200; PMID:21547159; http://dx.doi.org/10.7150/thno/v01p0189
- Danhier F, Le Breton A, Preat V. RGD-based strategies to target α(v) β(3) integrin in cancer therapy and diagnosis. Mol Pharm 2012; 9:2961-73; PMID:22967287; http://dx.doi.org/10.1021/mp3002733
- Zhu X, Marcus WD, Xu W, Lee HI, Han K, Egan JO, Yovandich JL, Rhode PR, Wong HC. Novel human interleukin-15 agonists. J Immunol 2009; 183:3598-607; PMID:19710453; http://dx.doi.org/10.4049/jimmunol.0901244
- Xiao K, Li Y, Lee JS, Gonik AM, Dong T, Fung G, Sanchez E, Xing L, Cheng HR, Luo J, et al. “OA02” peptide facilitates the precise targeting of paclitaxel-loaded micellar nanoparticles to ovarian cancer in vivo. Cancer Res 2012; 72:2100-10; PMID:22396491; http://dx.doi.org/10.1158/0008-5472.CAN-11-3883
- Mortier E, Woo T, Advincula R, Gozalo S, Ma A. IL-15Ralpha chaperones IL-15 to stable dendritic cell membrane complexes that activate NK cells via trans presentation. J Exp Med 2008; 205:1213-25; PMID:18458113; http://dx.doi.org/10.1084/jem.20071913
- Bouchaud G, Garrigue-Antar L, Sole V, Quemener A, Boublik Y, Mortier E, Perdreau H, Jacques Y, Plet A. The exon-3-encoded domain of IL-15ralpha contributes to IL-15 high-affinity binding and is crucial for the IL-15 antagonistic effect of soluble IL-15Ralpha. J Mol Biol 2008; 382:1-12; PMID:18656487; http://dx.doi.org/10.1016/j.jmb.2008.07.019
- Wu Z, Xu Y. IL-15R α-IgG1-Fc enhances IL-2 and IL-15 anti-tumor action through NK and CD8+ T cells proliferation and activation. J Mol Cell Biol 2010; 2:217-22; PMID:20671116; http://dx.doi.org/10.1093/jmcb/mjq012