ABSTACT
Human hepatocellular carcinoma (HCC) is known to have a poor prognosis. Sorafenib, a molecular targeted drug, is most commonly used for HCC treatment. However, its effect on HCC is limited in clinical use and therefore new strategies regarding sorafenib treatment are required. Iron overload is known to be associated with progression of chronic hepatitis and increased risk of HCC. We previously reported that iron depletion inhibited cancer cell proliferation and conversely induced angiogenesis. Indeed iron depletion therapy including iron chelator needs to be combined with anti-angiogenic drug for its anti-cancer effect. Since sorafenib has an anti-angiogenic effect by its inhibitory targeting VEGFR, we hypothesized that sorafenib could complement the anti-cancer effect of iron depletion. We retrospectively analyzed the relationship between the efficacy of sorafenib and serum iron-related markers in clinical HCC patients. In clinical cases, overall survival was prolonged in total iron binding capacity (TIBC) high- and ferritin low-patients. This result suggested that the low iron-pooled patients, who could have a potential of more angiogenic properties in/around HCC tumors, could be adequate for sorafenib treatment. We determined the effect of sorafenib (Nexavar®) and/or deferasirox (EXJADE®) on cancer cell viability, and on cell signaling of human hepatocarcinoma HepG2 and HLE cells. Both iron depletion by deferasirox and sorafenib revealed insufficient cytotoxic effect by each monotherapy, however, on the basis of increased angiogenesis by iron depletion, the addition of deferasirox enhanced anti-proliferative effect of sorafenib. Deferasirox was confirmed to increase vascular endothelial growth factor (VEGF) secretion into cellular supernatants by ELISA analysis. In in vivo study sorafenib combined with deferasirox also enhanced sorafenib-induced apoptosis. These results suggested that sorafenib combined with deferasirox could be a novel combination chemotherapy for HCC.
Keywords:
Introduction
Human hepatocellular carcinoma (HCC) is the fifth most common cancer, and is responsible for the third highest cancer mortality in the world.Citation1 HCC commonly appears in patients with chronic hepatitis and/or cirrhosis.Citation2 Sorafenib (Nexavar®), a molecular targeted drug, is most commonly used for HCC treatment.Citation3 Sorafenib is a multiple kinase inhibitor that directly suppresses tumor cell proliferation by blocking the activity of Raf kinases, leading to inhibition of the mitogen-activated protein kinase / extracellular signal-regulated kinase (MEK/ ERK) signaling pathway, and also inhibits angiogenesis by blocking several receptor tyrosine kinases such as vascular endothelial growth factor receptor (VEGFR) and platelet-derived growth factor receptor (PDGFR).Citation4,5 However its effect on HCC is limited in clinical use and therefore new strategies regarding sorafenib treatment are required.
Iron is an essential element for humans. Iron is taken orally and is mainly used in red blood cells to carry oxygen and is stored as ferritin in the liver.Citation6 Iron is also related with chronic inflammation including hepatitis. Phlebotomy has been performed to reduce iron levels, with the aim of the suppression of hepatitis virus activity. Such phlebotomy has indicated that reduction in iron suppressed to develop hepatitis. Iron plays important roles in normal cells such as in the creation of energy in mitochondria. Iron has a similar role in cancer cells. However, although both normal and cancer cells need iron to proliferate, cancer cells need more iron than normal cells because of their rapid proliferation. Iron overload is known to cause cancer in animal models Citation7,8 and conversely, iron depletion has been reported to suppress tumor growth.Citation9,10 However, monotherapy of iron reduction is not yet established as a cancer therapy for solid cancers including HCC. We have previously determined that perhaps one reason for this lack of effect of iron monotherapy for solid cancers is because iron depletion also results in induction of angiogenesis via HIF1-α and VEGF signaling.Citation11 Angiogenesis is thought to be a mechanism by which cancer cells escape from severe conditions. The fact that iron reduction inhibits tumor proliferation but enhances angiogenesis suggested that combination of iron reduction with an anti-angiogenic drug might result in increased anti-tumor effects. We thus hypothesized that iron depletion might enhance the anti-tumor effect of sorafenib. We therefore retrospectively analyzed clinical data of sorafenib and determined its potential anti-tumor capacity in combination with an iron chelator.
Materials and methods
Cell lines and cultures
The human hepatocarcinoma cell lines HepG2 (p53 wild type) and HLE (p53 mutant) were cultured in Dulbecco's modified Eagle's medium (DMEM, Sigma-Aldrich, St. Louis, MO) supplemented with 10% FBS (FCS, Hyclone, Logan, UT) and 100 U/mL penicillin/streptomycin (Sigma-Aldrich) in a humidified incubator at 37°C and 5% CO2.
Reagents
Sorafenib was purchased from LKT Laboratories (St. Paul, MN). The oral iron chelator, deferasirox (EXJADE®), was purchased from Novartis Pharma (Tokyo, Japan). Compounds were dissolved in 100% DMSO (Sigma-Aldrich) and diluted with DMEM to the desired concentration for in vitro studies.
Cell viability assay
The proliferation of HepG2 and HLE cells was evaluated using the Cell Proliferation Kit II; XTT assay (Roche Diagnostics GmbH, Mannheim, Germany). Cells were plated at a density of 6000 cells per well in 96-well microplates and were incubated at 37°C in a humidified atmosphere of 5% CO2 for 24 hours. Compounds dissolved in DMEM with 0.02% FBS were added to the wells and the cells were then incubated for an additional 24 -72 hours. The absorbance of the samples was measured at 450 nm using a microplate reader after XTT solution was added to each well. The combination index was analyzed with the CalcuSyn software (BioSoft, Cambridge, UK).
Western blotting analysis
Cell lysates were extracted using cell lysis buffer (50 mmol/L Tris-HCl (pH 7.4), 30 mmol/L NaCl, and 1% Triton X-100) containing protease inhibitors (cOmplete Mini, Roche Diagnostics GmbH). Nuclear protein was extracted using NE-PER buffer (Thermo Fisher Scientific, Rockford, IL). Equal amounts of total cellular proteins were separated by sodium dodecyl sulfate-polyacrylamide gel electrophoresis and transferred electrophoretically to polyvinylidene difluoride filter membranes (GE Healthcare UK Ltd, Buckinghamshire, UK). The membranes were incubated with primary antibodies overnight at 4°C, followed by incubation with secondary antibodies. An ECL Prime Western Blotting Detection Reagent (GE Healthcare UK Ltd) was used to detect the peroxidase activity of secondary antibodies. Antibodies against the following proteins were used for Western blotting: cyclinE (Santa Cruz Biotechnology, Inc., Santa Cruz, CA), p53 (EMD Millipore, Inc., Billerica, MA), β-actin (Sigma-Aldrich), cyclinD1, CDK4, p21, p27, caspase3, cleaved caspase3, PARP, cleaved PARP, phospho-MEK1/2 (Ser217/221), MEK1/2, phospho-ERK1/2 (Thr202/Tyr204), ERK1/2 and HIF-1α (all from Cell Signaling Technology, Inc., Danvers, MA).
Flow cytometric analysis of the cell cycle
Cancer cells were treated with the indicated concentrations of agents for 24 hours and were then stained with 20 mg/mL propidium iodide. The effect of the agents on the cell cycle was analyzed using a fluorescence-activated cell sorter (FACScan, Becton Dickinson, Franklin Lakes, NJ) with FlowJo software (TREE STAR, Ashland, OR).
VEGF ELISA assay
HepG2 and HLE cells were seeded in 6-well plates at a density of 100,000 cells per well. After 24 hours, the cells were treated with different concentrations of deferasirox (0 - 1,000 μM) and were incubated for an additional 24 hours. VEGF secretion was evaluated by ELISA assay of VEGF in culture supernatants using the Human VEGF Quantikine ELISA Kit (R&D Systems, Minneapolis, MN), according to the manufacturer's instructions. Absorbance was determined at 450 nm using a microplate reader.
Tumor xenograft model and experiment
All animal studies were approved by the Ethics Review Committee for Animal Experimentation of Okayama University, Okayama, Japan. BALB/c athymic mice (nu/nu) were purchased from Clea (Tokyo, Japan). All animals received humane care according to the criteria outlined in the “Guide for the Care and Use of Laboratory Animals” (http://oacu.od.nih.gov/ac_cbt/guife3.htm.htm). All mice were allowed to eat a meal with water freely. Six week-old female mice were used to establish a tumor xenograft model. A total of 3×10 Citation7 HepG2 cells were suspended in a 50% mixture of Basement Membrane Matrix (BD Biosciences, Bedford, MA) and were injected subcutaneously into the right flank. One week after injection, the mice were randomly divided into control and 3 treatment groups (n = 3), and administration of sorafenib and deferasirox was started. Each agent was orally administered daily for 5 d per week. The tumor volume was measured in day 21.
Immunohistological staining
HepG2 tumors were harvested for histological analysis after sorafenib and deferasirox treatment. Resected tumors were fixed in 10% paraformaldehyde and embedded in paraffin. Paraffin sections were immunohistologically stained. Anti-cleaved PARP antibodies used for immunohistological staining were purchased from Cell Signaling Technology, Inc.
Patient studies
The patient study included 58 HCC patients who were treated with sorafenib in Okayama University Hospital and affiliated hospitals from February 2009 to November 2011. Clinical data including background information was reviewed retrospectively using medical records. All patients provided written informed consent and the study protocol was approved by the review committee of Okayama University, Okayama, Japan (#1452). Tumors were assessed by CT or MRI, where each tumor size was measured to evaluate the therapeutic efficacy of sorafenib was defined according to the Response Evaluation Criteria in Solid Tumors (RECIST v1.1). The patients were categorized into 2 groups according to blood serum levels of iron-related markers (cut off value; median). The survival time (Progression-Free Survival (PFS) and Overall Survival (OS)) was calculated from the date of first sorafenib treatment to disease progression or death.
Statistical analysis
Analysis of variance was performed using Student's t-test. Survival time was estimated by the Kaplan-Meier estimator and statistical differences were analyzed using the log-rank test. The data analysis was performed using IBM SPSS Statistics ver.22 (IBM, Armonk, NY).
Results
Low iron conditions prolonged overall survival in sorafenib treated patients
First, we retrospectively investigated HCC patients that were treated with sorafenib (n = 58) in Okayama University Hospital and affiliated hospitals. The patients were categorized into low and high groups according to the levels of serum Fe, TIBC and ferritin, and survival time was statistically analyzed (cut off value; median). The background of the patients was not significantly different between the 2 treatment groups (Supplementary Table 1). Mean survival time (MST) of the high Fe patients (mean Fe 109.7 ± 8 .3 μg/dl) was 6.8 months and that of the low Fe patients (mean Fe 47.1 ± 2 .7 μg/dl) was 8.4 months. Mean survival time (MST) of the high TIBC patients (mean TIBC 395.4 ± 10 .2 μg/dl) was 9.8 months and that of the low TIBC patients (mean TIBC 229.7 ± 11 .1 μg/dl) was 5.6 months. Mean survival time (MST) of the high ferritin patients (mean ferritin 373.5 ± 46 .2 μg/dl) was 5.7 months and that of the low ferritin patients (mean ferritin 55.7 ± 8 .3 μg/dl) was 8.7 months. OS was significantly prolonged in the high TIBC and low ferritin patients; i.e. in low iron storage patients ().
Figure 1. The relationship between overall survival and serum related markers in HCC patients treated with sorafenib HCC patients treated with sorafenib were divided into 2 groups according to serum iron related markers (cut off value; median), and overall survival was analyzed by Kaplan-Meier survival analysis. *, p < 0.05. Survival time of low iron patients (low Fe, high total iron-binding capacity (TIBC), low ferritin groups) is shown as continuous lines, and survival time of high iron patients (high Fe, low TIBC, high ferritin groups) is shown as dotted lines.
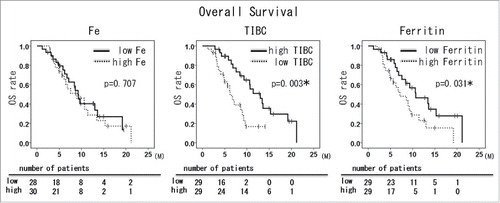
Sorafenib needed high concentration to induce apoptosis compared to cell cycle arrest
To determine the anti-cancer effect of sorafenib, the effect on the viability of 2 HCC cell lines were assayed using an XTT cell viability assay. This assay showed that sorafenib suppressed cell viability in a dose dependent manner (). Western blot analysis showed that sorafenib decreased the expression of the cell cycle regulators, cyclinD1 of 10 µM(). Sorafenib induced the expression of the apoptosis marker, Cleaved Caspase 3 of 100 µM. Sorafenib also induced the expression of another apoptosis marker, Cleaved PARP in HepG2 cells with its concentration of 100 µM. These data suggested that 10-fold high concentration of sorafenib was necessary for the induction of apoptosis compared to that of cell cycle arrest.
Figure 2. The inhibitory effect of sorafenib against hepatocellular carcinoma (HCC) cell lines in vitro (A) Cultured HepG2 and HLE cells were treated with different concentrations of sorafenib for 24 hours and cell viability was then evaluated using the XTT assay. Cell viability in the absence of treatment was set at 100%. Results are means + SD of 3 independent experiments. (B) Cultured HepG2 and HLE cells were treated with different concentrations of sorafenib for 72 hours and cell cycle and apoptotic effect was then evaluated using western blot analysis. Cells were then harvested and total protein in cell lysates was analyzed for expression of the indicated proteins.
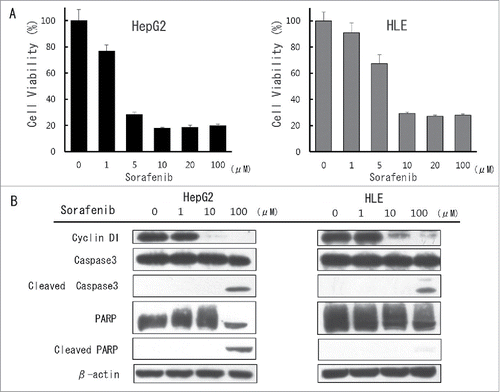
Iron depletion by deferasirox inhibited cancer cell proliferation
We previously reported that iron depletion by deferasirox suppressed cell proliferation in the lung cancer cell lines.Citation11 To determine the anti-cancer effect of iron depletion by deferasirox in the hepatocarcinoma cell lines, the effect on the viability of cell lines were assayed using an XTT cell viability assay. This assay showed that deferasirox suppressed cell viability in a dose dependent manner (). Western blot analysis showed that Deferasirox decreased the expression of cyclinD1 in HepG2 cells with its concentration of 100 µM and in HLE cells with its concentration of 10µM (). However, Deferasirox did not induce the expression of the apoptosis marker, Cleaved Caspase 3 of HepG2. Deferasirox also slightly induced Cleaved Caspase 3 and Cleved PARP in HLE cells with its concentration of 100 µM. FACS analysis showed that deferasirox increased the percentage of cells in the G0-G1 phase and decreased the percentage in the G2-M phase (Supplementary Fig. S1). These data suggested that major aspect of deferasirox on the suppression of cell proliferation would be cell cycle arrest.
Figure 3. The inhibitory and angiogenic effects of deferasirox against hepatocellular carcinoma (HCC) cell lines in vitro (A) Cultured HepG2 and HLE cells were treated with different concentrations of deferasirox for 72 hours and cell viability was then evaluated using the XTT assay. Cell viability in the absence of treatment was set at 100%. Results are means + SD of 3 independent experiments. (B) Cultured HepG2 and HLE cells were treated with different concentrations of deferasirox for 72 hours and cell cycle and apoptotic effect was then evaluated using protein gel blot analysis. Cells were then harvested and total protein in cell lysates was analyzed for expression of the indicated proteins. (C) Cultured HepG2 and HLE cells were treated with different concentrations of deferasirox for 72 hours and the supernatant was then harvested and the amount of VEGF secreted by the cells was assessed using an ELISA assay. The level of VEGF secreted by non-treated cells was set at 100%. *, p < 0.05; **, p < 0.01. (D) Cultured HepG2 and HLE cells were treated with different concentrations of deferasirox for 72 hours. Cells were then harvested and nuclear proteins were analyzed by Western blotting to examine the expression of HIF-1α. The gels were run under the same experimental conditions.
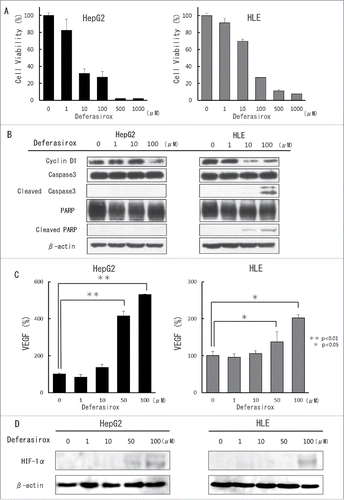
Iron depletion by deferasirox induced angiogenesis via HIF-1α and VEGF signaling
As mentioned above, iron depletion only is not enough to cancer progression. One of the reasons may be that iron depletion by deferasirox induced angiogenesis via HIF-1α and VEGF signaling in compensation as we reported previously. To investigate the potential effect of deferasirox on angiogenesis in the hepatocarcinoma cell lines, we assayed the level of VEGF secreted by cells into the supernatant using an ELISA assay. Deferasirox treatment increased VEGF secretion in a dose dependent manner (). Western blot analysis also indicated that deferasirox induced nuclear HIF-1α expression (). These results suggested that iron depletion by deferasirox also induced angiogenesis via HIF-1α and VEGF signaling in compensation.
Deferasirox enhanced the inhibitory effect of sorafenib on cell viability
Next question is whether increased angiogenic status by iron depletion can be a preferable condition for sorafenib treatment. Cell viability assay revealed that the addition of deferasirox to sorafenib treatment strongly suppressed cell proliferation, compared to single administration of them (). To better assess the synergistic effect of iron depletion combined with sorafenib, we calculated the combination index. The calculated combination index showed that deferasirox displayed significant synergy with sorafenib at a number of concentrations ().
Figure 4. Synergistic inhibitory effect of sorafenib and deferasirox against HCC cells (A) HepG2 and HLE cells were treated with the indicated concentrations of sorafenib and deferasirox for 48 hours, following which cell viability was assessed using the XTT assay. (B) The combination index was defined as interaction indices, and was calculated from the data in (A) using CalcuSyn software. An index of less than 1 indicates synergistic interaction and an index greater than 1 indicates antagonistic interaction.
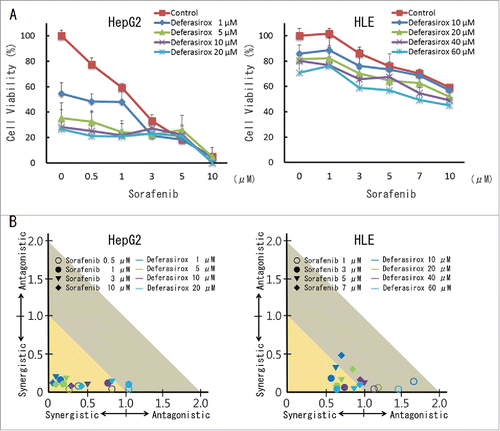
Sorafenib combined with deferasirox synergistically inhibited the cell cycle and induced apoptosis
To analyze the mechanism of synergy between sorafenib and deferasirox in greater detail, changes in the cell cycle, apoptosis and in MEK-ERK (a signaling pathway blocked by sorafenib) signaling cascades were investigated by Western blot analysis. The combination of sorafenib and deferasirox resulted in a greater decrease in the expression of cell cycle regulatory proteins (cyclinD1, cyclinE), cyclin-dependent kinase (CDK4) and cyclin-dependent kinase inhibitors (p21, p27) and a greater increase in cleaved PARP compared to the effect of either agent alone (). Monotherapy with deferasirox induced no significant changes in MEK-ERK signaling. FACS analysis showed that sorafenib combined with deferasirox also inhibited the cell cycle (Fig. S3). These data suggested that sorafenib combined with deferasirox synergistically inhibited the cell cycle and could facilitate sorafenib-induced apoptosis.
Figure 5. Changes in signaling cascades induced by sorafenib and/or deferasirox HepG2 (top) and HLE (bottom) cells were treated with the indicated concentrations of sorafenib and/or deferasirox for 48 hours. Cells were then harvested and total protein in cell lysates was analyzed for expression of the indicated proteins involved in cell cycle regulation (left), apoptosis (middle) and MAPKinase cascades (right) by Western blot analysis. The gels were run under the same experimental conditions. Protein bands were quantified by densitometry using Image J software.
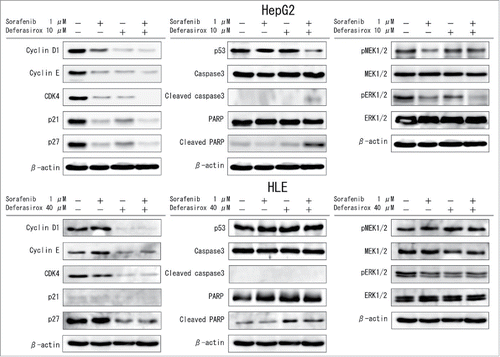
Sorafenib combined with deferasirox enhanced apoptosis in vivo
To confirm the enhancement of sorafenib-induced apoptosis by deferasirox in vivo, a subcutaneous tumor model with HepG2 cells was used. Oral administration of sorafenib and/or deferasirox was started one week after the injection of HepG2 cells. The tumor volume of sorafenib combined with deferasirox was smaller than the single and control treatment groups (). Immunostaining of the tumors indicated that the expression of cleaved PARP, an indicator of apoptosis, was increased in the combination treatment group compared with the single and control treatment groups (). These data suggested that the enhancement of anti-tumor effect of sorafenib by adding deferasirox could represent in in vivo setting.
Figure 6. Synergistic inhibitory effect of sorafenib and deferasirox against HCC in vivo (A) HepG2 cells (3×107 per animal) were implanted subcutaneously into the right flank of mice. Sorafenib and/or deferasirox administration was initiated one week after injection. Each agent was orally administered daily for 5 d per week. Tumor measurement was started 3 weeks after injection. (B) Resected tumors were analyzed for cleaved PARP by immunohistological staining. Cleaved PARP staining showed apoptotic cells as positive spot areas.
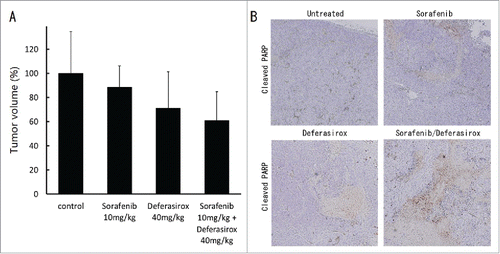
Discussion
Iron levels are related to carcinogenesis and cancer progression. An excess of some iron compounds causes cancer.Citation7,8 Liver is an organ that metabolizes and stores iron, and liver diseases are often associated with iron metabolic disorders. In many hepatic diseases such as hemochromatosis, chronic hepatitis B/C and non-alcoholic steatohepatitis (NASH) iron homeostasis cannot be maintained because of the dysregulation of iron regulatory proteins.Citation12-15 An excess of iron in hepatocytes has recently been suggested to be closely associated with the development of HCC.Citation16,17 The adverse effect of iron excess is the reason why iron depletion therapy is performed to improve many hepatic diseases. Indeed, phlebotomy is performed for hepatitis C patients to lower the risk of developing HCC.Citation18 Therefore, iron depletion is a rational approach for the therapy of hepatic malignant disease. Iron levels in the body can be reduced by phlebotomy, an iron depleted diet, or with an iron chelator. Phlebotomy has been performed to improve inflammation of the liver in hepatitis patients. However low Hb patients could not be treated with phlebotomy in spite of their high storage of iron. Although an iron depleted diet is comparatively safe, dietary restriction over a long time is required. The use of an iron chelator is the easiest way to reduce iron and is expected to be applied to treatment of liver disease.
Deferasirox enhanced the inhibitory effect of sorafenib in in vitro study. Cancer cells require a large amount of iron because of their rapid proliferation. Indeed, iron depletion has been reported to have anti-cancer effects.Citation19-21 We examined cell cycle arrest and apoptosis by Western blotting and FACS analysis. Iron depletion by deferasirox inhibited cancer cell proliferation via cell cycle arrest rather than apoptosis. Single agent of sorafenib and deferasirox did not induce apoptosis strongly. However, the combination therapy of sorafenib and deferasirox synergistically inhibited cancer cell proliferation via cell cycle arrest and apoptosis. The synergistic effect of deferasirox and sorafenib on apoptosis in HepG2 cells might be associated with the notable change in cyclin-dependent kinase inhibitor p21 signals. During chemotherapy or radiation treatment cancer cells are known to repair themselves by upregulation of p21.Citation22,23 On the other hand, inhibition of p21 can possibly suppress cancer cell proliferation by preventing this self-repair mechanism.Citation24-26 Both sorafenib and deferasirox have the ability to inhibit cyclin-dependent kinase, and the combination of sorafenib and deferasirox have been shown to synergistically induce apoptosis by inhibition of p21.Citation27,28 These mechanisms are the reason for the synergistic effect of sorafenib in combination with deferasirox. However, we could not identify the mechanisms in the liver completely. The mechanisms including microenvironment should be examined by using orthotopic animal model or carcinogenic animal model in the next step.Citation29,30 Recently the imaging technology for cells and animals has been progressed.Citation31-34 It may be also helpful to reveal the synergistic effect of sorafenib in combination with deferasirox more detail.
Our results showed that OS was prolonged in high TIBC and low ferritin groups, which reflects low iron patients. This result is consistent with in vitro study, which iron depletion by deferasirox enhanced sorafenib via the induction of cell cycle arrest and apoptosis. It was also shown that iron depletion by deferasirox suppressed cancer cell proliferation and induced angiogenesis via HIF-1α and VEGF signaling. These results suggest that sorafenib still exerts its anti-angiogenic effect in combination with iron depletion treatment. In clinical cases, Serum iron (Fe) levels did not significantly affect OS. In general, blood serum iron levels (Fe) can change rapidly because of diurnal variation, which might be a reason why serum iron levels are not significantly correlated with OS.Citation35 In addition, although low iron patients appear to have reduced inflammation, it is unclear by how much inflammation is reduced. A prospective clinical study is expected to start soon.
In conclusion, low iron conditions prolonged overall survival in sorafenib treated patients. Iron depletion by deferasirox enhanced the inhibitory effect of sorafenib via induction of cell cycle arrest and apoptosis. Sorafenib is compatible with deferasirox because of HIF1-α and VEGF induction signaling by deferasirox. These results suggest that iron depletion by deferasirox has the potential to be a novel combination chemotherapy with sorafenib for HCC.
Disclosure of potential conflicts of interest
No potential conflicts of interest were disclosed.
Supplementary_Data.zip
Download Zip (488.2 KB)Funding
This work was supported by the Princess Takamatsu Cancer Research Fund and by a Grant-in-Aid for Scientific Research from the Japan Society for the Promotion of Science (Grant Number 26861048).
References
- Llovet JM, Burroughs A, Bruix J. Hepatocellular carcinoma. Lancet 2003; 362:1907-17; PMID:14667750; http://dx.doi.org/10.1016/S0140-6736(03)14964-1
- Montalto G, Cervello M, Giannitrapani L, Dantona F, Terranova A, Castagnetta LA. Epidemiology, risk factors, and natural history of hepatocellular carcinoma. Ann N Y Acad Sci 2002; 963:13-20; PMID:12095924; http://dx.doi.org/10.1111/j.1749-6632.2002.tb04090.x
- Llovet JM, Ricci S, Mazzaferro V, Hilgard P, Gane E, Blanc JF, de Oliveira AC, Santoro A, Raoul JL, Forner A, et al. Sorafenib in advanced hepatocellular carcinoma. N Engl J Med 2008; 359:378-90; PMID:18650514; http://dx.doi.org/10.1056/NEJMoa0708857
- Wilhelm SM, Carter C, Tang L, Wilkie D, McNabola A, Rong H, Chen C, Zhang X, Vincent P, McHugh M, et al. BAY 43-9006 exhibits broad spectrum oral antitumor activity and targets the RAF/MEK/ERK pathway and receptor tyrosine kinases involved in tumor progression and angiogenesis. Cancer Res 2004; 64:7099-109; PMID:15466206; http://dx.doi.org/10.1158/0008-5472.CAN-04-1443
- Liu L, Cao Y, Chen C, Zhang X, McNabola A, Wilkie D, Wilhelm S, Lynch M, Carter C. Sorafenib blocks the RAF/MEK/ERK pathway, inhibits tumor angiogenesis, and induces tumor cell apoptosis in hepatocellular carcinoma model PLC/PRF/5. Cancer Res 2006; 66:11851-8; PMID:17178882; http://dx.doi.org/10.1158/0008-5472.CAN-06-1377
- Toyokuni S. Role of iron in carcinogenesis: cancer as a ferrotoxic disease. Cancer Sci 2009; 100:9-16; PMID:19018762; http://dx.doi.org/10.1111/j.1349-7006.2008.01001.x
- Okada S, Hamazaki S, Toyokuni S, Midorikawa O. Induction of mesothelioma by intraperitoneal injections of ferric saccharate in male Wistar rats. British J Cancer 1989; 60:708-11; PMID:2803947; http://dx.doi.org/10.1038/bjc.1989.344
- Jiang L, Akatsuka S, Nagai H, Chew SH, Ohara H, Okazaki Y, Yamashita Y, Yoshikawa Y, Yasui H, Ikuta K, et al. Iron overload signature in chrysotile-induced malignant mesothelioma. J Pathol 2012; 228:366-77; PMID:22864872; http://dx.doi.org/10.1002/path.4075
- Hann HW, Stahlhut MW, Blumberg BS. Iron nutrition and tumor growth: decreased tumor growth in iron-deficient mice. Cancer Res 1988; 48:4168-70; PMID:3390810
- Yu Y, Suryo Rahmanto Y, Richardson DR. Bp44mT: an orally active iron chelator of the thiosemicarbazone class with potent anti-tumour efficacy. British J Pharmacol 2012; 165:148-66; PMID:21658021; http://dx.doi.org/10.1111/j.1476-5381.2011.01526.x
- Ohara T, Noma K, Urano S, Watanabe S, Nishitani S, Tomono Y, Kimura F, Kagawa S, Shirakawa Y, Fujiwara T. A novel synergistic effect of iron depletion on antiangiogenic cancer therapy. Int J Cancer J Int du Cancer 2013; 132:2705-13; PMID:23161652; http://dx.doi.org/10.1002/ijc.27943
- Kew MC. Hepatic iron overload and hepatocellular carcinoma. Liver Cancer 2014; 3:31-40; PMID:24804175; http://dx.doi.org/10.1159/000343856
- Tirnitz-Parker JE, Glanfield A, Olynyk JK, Ramm GA. Iron and hepatic carcinogenesis. Crit Rev Oncog 2013; 18:391-407; PMID:23879586; http://dx.doi.org/10.1615/CritRevOncog.2013007759
- Horl WH, Schmidt A. Low hepcidin triggers hepatic iron accumulation in patients with hepatitis C. Nephrol Dial Transplant 2014; 29:1141-4; PMID:24286977; http://dx.doi.org/10.1093/ndt/gft467
- Beckman LE, Hagerstrand I, Stenling R, Van Landeghem GF, Beckman L. Interaction between haemochromatosis and transferrin receptor genes in hepatocellular carcinoma. Oncology 2000; 59:317-22; PMID:11096344; http://dx.doi.org/10.1159/000012189
- Chen J, Chloupkova M. Abnormal iron uptake and liver cancer. Cancer Biol Ther 2009; 8:1699-708; PMID:19571663; http://dx.doi.org/10.4161/cbt.8.18.9146
- Tseng HH, Chang JG, Hwang YH, Yeh KT, Chen YL, Yu HS. Expression of hepcidin and other iron-regulatory genes in human hepatocellular carcinoma and its clinical implications. J Cancer Res Clin Oncol 2009; 135:1413-20; PMID:19387685; http://dx.doi.org/10.1007/s00432-009-0585-5
- Kato J, Miyanishi K, Kobune M, Nakamura T, Takada K, Takimoto R, Kawano Y, Takahashi S, Takahashi M, Sato Y, et al. Long-term phlebotomy with low-iron diet therapy lowers risk of development of hepatocellular carcinoma from chronic hepatitis C. J Gastroenterol 2007; 42:830-6; PMID:17940836; http://dx.doi.org/10.1007/s00535-007-2095-z
- Gaboriau F, Leray AM, Ropert M, Gouffier L, Cannie I, Troadec MB, Loreal O, Brissot P, Lescoat G. Effects of deferasirox and deferiprone on cellular iron load in the human hepatoma cell line HepaRG. Biometals 2010; 23:231-45; PMID:19997770; http://dx.doi.org/10.1007/s10534-009-9281-9
- Ba Q, Hao M, Huang H, Hou J, Ge S, Zhang Z, Yin J, Chu R, Jiang H, Wang F, et al. Iron deprivation suppresses hepatocellular carcinoma growth in experimental studies. Clin Cancer Res 2011; 17:7625-33; PMID:22052937; http://dx.doi.org/10.1158/1078-0432.CCR-10-3099
- Lui GY, Obeidy P, Ford SJ, Tselepis C, Sharp DM, Jansson PJ, Kalinowski DS, Kovacevic Z, Lovejoy DB, Richardson DR. The iron chelator, deferasirox, as a novel strategy for cancer treatment: oral activity against human lung tumor xenografts and molecular mechanism of action. Mol Pharmacol 2013; 83:179-90; PMID:23074173; http://dx.doi.org/10.1124/mol.112.081893
- Lazzarini R, Moretti S, Orecchia S, Betta PG, Procopio A, Catalano A. Enhanced antitumor therapy by inhibition of p21waf1 in human malignant mesothelioma. Clin Cancer Res 2008; 14:5099-107; PMID:18698027; http://dx.doi.org/10.1158/1078-0432.CCR-08-0255
- Waldman T, Kinzler KW, Vogelstein B. p21 is necessary for the p53-mediated G1 arrest in human cancer cells. Cancer Res 1995; 55:5187-90; PMID:7585571
- Wettersten HI, Hee Hwang S, Li C, Shiu EY, Wecksler AT, Hammock BD, Weiss RH. A novel p21 attenuator which is structurally related to sorafenib. Cancer Biol Ther 2013; 14:278-85; PMID:23298903; http://dx.doi.org/10.4161/cbt.23374
- Giovannini C, Baglioni M, Baron Toaldo M, Ventrucci C, D'Adamo S, Cipone M, Chieco P, Gramantieri L, Bolondi L. Notch3 inhibition enhances sorafenib cytotoxic efficacy by promoting GSK3b phosphorylation and p21 down-regulation in hepatocellular carcinoma. Oncotarget 2013; 4:1618-31; PMID:24113128; http://dx.doi.org/10.18632/oncotarget.1221
- Inoue H, Hwang SH, Wecksler AT, Hammock BD, Weiss RH. Sorafenib attenuates p21 in kidney cancer cells and augments cell death in combination with DNA-damaging chemotherapy. Cancer Biol Ther 2011; 12:827-36; PMID:21878748; http://dx.doi.org/10.4161/cbt.12.9.17680
- Fu D, Richardson DR. Iron chelation and regulation of the cell cycle: 2 mechanisms of posttranscriptional regulation of the universal cyclin-dependent kinase inhibitor p21CIP1/WAF1 by iron depletion. Blood 2007; 110:752-61; PMID:17429006; http://dx.doi.org/10.1182/blood-2007-03-076737
- Alkhateeb AA, Connor JR. The significance of ferritin in cancer: anti-oxidation, inflammation and tumorigenesis. Biochimica et biophysica acta 2013; 1836:245-54; PMID:23891969; http://dx.doi.org/10.1016/j.bbcan.2013.07.002
- Hoffman RM. Orthotopic metastatic mouse models for anticancer drug discovery and evaluation: a bridge to the clinic. Investigational new drugs 1999; 17:343-59; PMID:10759402; http://dx.doi.org/10.1023/A:1006326203858
- Hoffman RM. Patient-derived orthotopic xenografts: better mimic of metastasis than subcutaneous xenografts. Nat Rev Cancer 2015; 15:451-2; PMID:26422835; http://dx.doi.org/10.1038/nrc3972
- Yamamoto N, Jiang P, Yang M, Xu M, Yamauchi K, Tsuchiya H, Tomita K, Wahl GM, Moossa AR, Hoffman RM. Cellular dynamics visualized in live cells in vitro and in vivo by differential dual-color nuclear-cytoplasmic fluorescent-protein expression. Cancer Res 2004; 64:4251-6; PMID:15205338; http://dx.doi.org/10.1158/0008-5472.CAN-04-0643
- Hoffman RM, Yang M. Subcellular imaging in the live mouse. Nature protocols 2006; 1:775-82; PMID:17406307; http://dx.doi.org/10.1038/nprot.2006.109
- Hoffman RM. The multiple uses of fluorescent proteins to visualize cancer in vivo. Nat Rev Cancer 2005; 5:796-806; PMID:16195751; http://dx.doi.org/10.1038/nrc1717
- Yang M, Jiang P, Hoffman RM. Early Reporting of Apoptosis by Real-time Imaging of Cancer Cells Labeled with Green Fluorescent Protein in the Nucleus and Red Fluorescent Protein in the Cytoplasm. Anticancer Res 2015; 35:2539-43; PMID:25964527
- Uchida T, Akitsuki T, Kimura H, Tanaka T, Matsuda S, Kariyone S. Relationship among plasma iron, plasma iron turnover, and reticuloendothelial iron release. Blood 1983; 61:799-802; PMID:6403079