ABSTRACT
The isoprenoid biosynthetic pathway (IBP) plays a critical role in providing substrates and enzymes necessary for the post-translational modification and thus activation of a number of proteins involved in prostate cancer metastasis. Previous work by our lab found novel compound disodium [(6Z,11E,15E)-9-[bis(sodiooxy)phosphoryl]−17-hydroxy-2,6,12,16-tetramethyheptadeca-2,6,11,15-tetraen-9-yl]phosphonate (GGOHBP), which inhibits the IBP enzyme geranylgeranyl diphosphate synthase (GGDPS), reduced protein geranylgeranylation without altering protein farnesylation. This activity significantly reduced adrenal gland tumor burden in a murine model of human prostate cancer metastasis which relied on treatment of established disease. The present study determined the ability of GGDPS inhibition to slow the development of prostate cancer metastasis in a preventative murine model. Using tail vein injection of human derived PC-3 prostate cancer cells 4 d after initiating daily GGOHBP or vehicle treatments, we found GGOHBP significantly reduced whole body tumor burden, significantly slowed the development of tumors, and prolonged overall survival as compared to vehicle treated animals. The observed reduction in soft tissue tumor burden corresponded to a biochemical reduction in Rap1A geranylgeranylation, which for prostate cancer is important in its own merit and which serves as a surrogate marker for Rho family, i.e. Rac, protein modification. This effect was present in all treated mice pointing to strong target engagement, which was not observed in non-tumor burdened tissues or control mice. Our findings reiterate a role for protein geranylgeranylation in the development of prostate cancer metastasis in vivo.
Introduction
There are over 30,000 deaths from PCa each year in the United States. Virtually all of these deaths result from tumor metastases that have spread beyond the initial site of tumor development. Prostate cancer is often diagnosed early when treatment with surgery and/or radiation has a good outcome. More advanced disease is usually treated with androgen ablation resulting in a castrate state.Citation1 While hormone therapy naïve cancers typically have high response rates to androgen ablation, the disease generally returns as castrate or hormone therapy resistant prostate cancer which is invasive and lethal, typically causing death within 1–3 y.Citation2,3
Many of the cellular processes required for prostate cancer metastasis rely on proteins of the isoprenoid biosynthetic pathway (IBP, ). The IBP is commonly known for its production of cholesterol but is also critical for the post-translational modification and thus activation of the Ras superfamily of small GTPases. Ras superfamily proteins involved in the malignant cellular processes of prostate cancer metastasis include: RhoA, Rac1, and Cdc42 in cell polarityCitation4 and cell motility;Citation5,6 RhoA and Rac1 in cell junctions;Citation7-10 RhoC in vascularization;Citation11 and the Rho family in intravasation.Citation12,13 Each of these proteins require the post-translational addition of a 20 carbon geranylgeranyl pyrophosphate (GGPP) moiety for proper spatio-temporal activity, a process known as geranylgeranylation.Citation14,15
Figure 1. Clinically relevant inhibitors of the isoprenoid biosynthetic pathway (IBP) (a) Statins inhibit HMG CoA reductase, consequently reducing the entire IBP. Nitrogenous bisphosphonates inhibit FPP synthase (FDPS), consequently reducing FPP, GGPP, farnesylation, and geranylgeranylation. Our novel isoprenoid bisphosphonate compounds (b) DGBP and (c) GGOHBP inhibit GGPP synthase (GGDPS), consequently reducing only GGPP and protein geranylgeranylation.
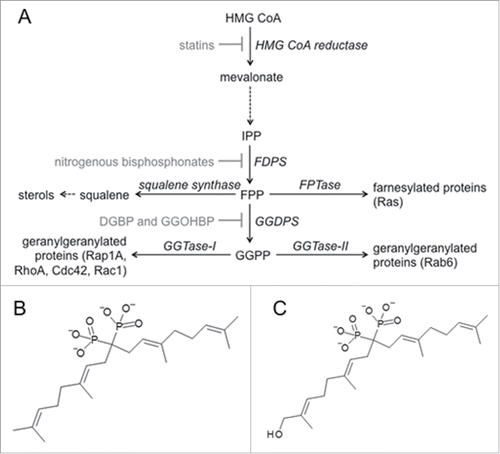
In particular Rho family member Rac1 is associated clinically with PCa progression and metastasis. Lyons et al. demonstrated Rac1 is central to androgen receptor activation under ligand-free conditions and important for in vitro conversion to a hormone resistant phenotype.Citation15 These same authors had previously demonstrated that Rac1 activity was greater in androgen independent cell lines,Citation16 and that this activity was instrumental in down regulating the tumor suppressor p21CIP1. Another study examined clinical prostatectomy specimens and found that increased levels of Rac proteins (Rac1, Rac2 and Rac3) were associated with a decrease in disease-free survival.Citation17 Additional studies of Rac associated signaling implicates Rac as a crucial information integration point where enhanced signal strength leads to advanced disease, metastatic spread, and poor prognosis.Citation18,19 Rac signaling is linked to progression to the hormone resistant state by controlling communication between genotropic and non-genotropic signaling in the proliferation of PCa cells.Citation20,21
A member of another family of signaling molecules, the Ras family of proteins, is Rap1. The parent family member Ras undergoes post-translational modification with a 15 carbon IBP intermediate (farnesylation), Rap1 however, is gernaylgeranylated. Rap1, specifically the isoform Rap1A, is involved in cellular signaling and cell adhesion.Citation22 Signaling through Rap1A has been implicated in cell proliferation of several cancers including disease originating in breast and pancreas as well as melanoma.Citation23-26 The study of Rap1A in tumor metastasis has demonstrated more complex behavior. In a pancreatic cancer model reduced Rap1A signaling reduced local invasion,Citation24 while in an osteosarcoma model reduction of Rap1A signaling promoted an invasive phenotype.Citation23 In prostate cancer it has been demonstrated that activation of Rap1A promotes metastatic behavior.Citation27 Recently, reduction in Rap1A signaling by mi-RNA-203 was shown to reduce proliferation, and invasive behavior in prostate cancer cells.Citation28
The IBP provides the substrates and enzymes critical for protein geranylgeranylation.Citation29-37 Clinically relevant inhibitors of the pathway, including statins and nitrogenous bisphosphonates (NBP), are some of the most prescribed drugs and have numerous effects. The statins are inhibitors of the early rate limiting step in the IBP catalyzed by 3-hydroxy-3-methylglutaryl coenzyme A reductase (HMGR), and are prescribed worldwide for treatment of hypercholesterolemia. The lipophilic statins inhibit HMGR predominantly in the liver and reduce farnesyl pyrophosphate flux through to cholesterol ultimately reducing plasma cholesterol. Pleotropic effects of the statins have been implicated through epidemiological studies for their ability to reduce the risk of prostate cancer progression.Citation35,36, 38-41
The NBP are used to treat osteoporosis and bone metastatic disease associated with cancers of the breast and prostate.Citation42 These compounds are hydrophilic and bind tightly to bone mineral with the bulk of the dose being removed from the systemic circulation very rapidly and eliminated via the kidneys.Citation43 The bone bound compound is released slowly as bone turnover occurs and has a pharmacodynamic effect predominantly in the bone milieu.Citation43 The NBP are competitive inhibitors of the IBP enzyme farnesyl pyrophosphate synthase directly reducing the amount of FPP available for conversion to GGPP, protein farnesylation, and other downstream metabolism.Citation44,45 Interestingly, even though most of the effects are thought to occur in the bone there is evidence of systemic effect on the cancer phenotype. A significant reduction in breast cancer recurrence was found in a large meta-analysis study of adjuvant NBP treatment early in the disease.Citation46-48 In these studies the NBP zoledronic acid reduced both bone and distant metastatic recurrence of breast cancer and improved disease free survival in post-menopausal woman.Citation49
Previously, our lab reported the synthesis of several inhibitors of the IBP enzyme geranylgeranyl diphosphate synthase (GGDPS). This enzyme directly converts FPP to GGPP and is thus the most relevant IBP enzyme target for the reduction of protein geranylgeranylation. The library of GGDPS inhibitors include digeranyl bisphosphonate (DGBP, ) and disodium [(6Z,11E,15E)-9-[bis(sodiooxy)phosphoryl]-17-hydroxy-2,6,12,16-tetramethyheptadeca-2,6,11,15-tetraen-9-yl]phosphonate (GGOHBP, ). In vitro work in the human breast cancer cell line MDA-MB-231 found DGBP to inhibit cell migration.Citation50 The compound DGBP was also shown to induce autophagy in the PC-3 human prostate cancer cell line.Citation51 More recently, GGOHBP was used in a treatment model of human prostate cancer metastasis. In this mouse model the 22Rv1-luc prostate derived cell line was injected via the intercardiac route and established metastatic tumors in adrenal gland, bone and additional sites. In this experiment GGOHBP significantly reduced established adrenal gland tumor weights while no effect on bone metastatic sites was observed.Citation52
Because our novel bisphosphonates lack parts of the bone targeting portion of the clinical NBP we hypothesized that they may be more available for action at extra-skeletal sites. Herein we report activity of GGDPS inhibition in preventing the development of metastatic lesions in a model of prostate cancer metastasis using tail vein injection of the PC-3-luciferase tagged cell line. This cell line and route of tumor inoculation has been adapted to give soft tissue tumor metastasis preferentially.Citation53 Our previous work has shown that compounds like GGOHBP are not very toxic to cancer cells but that lower doses will reduce protein geranylgeranylation of Rho family proteins. For this reason we decided to test GGOHBP in a preventative model setting where drug treatment is begun prophylactically affording us the best chance of observing an effect on Rho protein involvement in metastatic colonization.
Results
GGOHBP reduced Rap1A and Rab6 geranylgeranylation without altering Ras farnesylation
In isolated GGDPS enzyme assays, DGBP and GGOHBP reduced GGDPS activity with IC50s of 0.2 μM and 0.8 μM respectively (). Analysis of compound cytotoxicity by MTT activity found DGBP and GGOHBP caused comparable reductions in the luciferase-expressing PC-3 cell line (). Following 48 hrs of treatment, DGBP reduced MTT activity below 80% at 1 μM as compared to 10 μM GGOHBP, while neither reduced activity below 50% until 100 μM ().
Figure 2. Novel compound GGOHBP inhibits Rap1A and Rab6 geranylgeranylation without altering Ras farnesylation (a) GGDPS enzyme inhibition and (b) MTT activity of the luciferase-expressing PC-3 cell line following 48 hr treatment with the indicated concentrations of each compound. Representative Western blot analysis of (c) Ras, (d) Rap1A, and (e) Rab6 following 48 hr treatment with the indicated concentrations of each compound in the luciferase-expressing PC-3 cell line. The anti-Ras and anti-Rab6 antibodies detect the unprenylated (aqueous) and prenylated (detergent) forms of Ras and Rab6, requiring separation by TX-114 detergent. The anti-Rap1A antibody detects the unprenylated form of Rap1A. Experiments were performed in triplicate. Error bars indicate standard deviation.
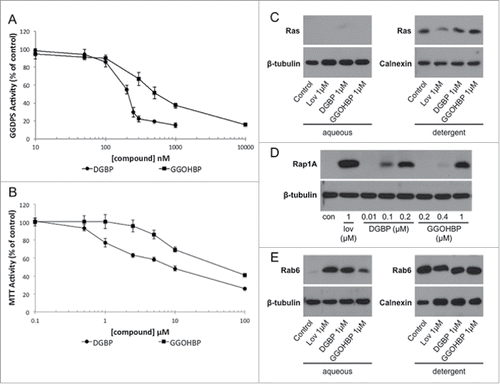
Prior to GGOHBP use in a preventative animal study, its effects on farnesylation and geranylgeranylation in the luciferase-expressing PC-3 cell line were tested by Western blot analysis of Ras, Rap1A, and Rab6. The anti-Ras and anti-Rab6 antibodies detect both the unprenylated (aqueous) and prenylated (detergent) forms, requiring an additional separation by TX-114. The anti-Rap1A antibody detects the unprenylated form, a band indicating a reduction in Rap1A geranylgeranylation. As shown by the lack of an aqueous band in combination with no change in the detergent band density, GGOHBP at 1 μM did not alter Ras farnesylation (). Conversely, GGOHBP reduced Rap1A geranylgeranylation detectibly at 0.4 μM as compared to 0.1 μM DGBP (). Finally, GGOHBP reduced Rab6 geranylgeranylation at 1 μM as shown by an aqueous band (). Moving into preventative animal studies, GGOHBP was chosen as the compound of interest due to its previous success in established soft tissue tumors as well as its comparable inhibition to DGBP in vitro with an improved therapeutic index in vivo.Citation52
GGOHBP significantly slowed tumor development in a preventative murine model of human prostate cancer metastasis
The preventative model of prostate cancer metastasis was initiated via tail vein injection of the luciferase-expressing PC-3 cell line 4 d after daily GGOHBP or vehicle treatment began. Due to the previously published observed toxicity of GGOHBP at 1.5 mg/kg daily in the presence of a high tumor burden in mice,Citation52 GGOHBP was given at 1.25 mg/kg daily. shows the distribution of tumors as determined by ex vivo bioluminescence imaging (BLI). Analysis of all mice found lung, mesenteric, and liver tumors to be most common at 70.6%, 47.1%, and 41.2% respectively (). Tumors were additionally found in the thoracic cavity, adrenal glands, heart, kidney, lower back region, diaphragm, thigh, scapula region, and mid back region to varying degrees (). Despite the assignment of mice to treatment and vehicle-treated groups prior to tumor development, the distribution of tumors between the control and treatment groups was fairly equal ().
Table 1. Distribution of tumors as determined by ex vivo BLI. Sites of metastasis are ordered from most common to least common presentation in all mice.
Representative weekly BLIs of control and treated mice are shown in . The dashed red line indicates a required temporary switch to an AMI instrument at weeks 5 and 6. Tumors became visible beginning at week 4 (). As shown, the control mice trended toward a greater number of tumors as compared to the treated mice (). Analysis of whole body photon counts during weeks 1-7 while all mice were still part of the study found a clear separation between the control (solid line) and treatment (dashed line) groups, with the control mice developing tumors more quickly than the treated mice (). By week 7 treated mice had significantly (P < 0.05) lower whole body photon counts as compared to the control mice (). Following imaging at week 7, mice began to be removed from the study due to weight loss greater than 20% and/or illness (). Consequently, the averaged whole body photon counts within the control and treated groups became highly variable from week-to-week (). In spite of variability, the treated mice continued to show a trend toward a reduction in whole body photon counts as compared to the control mice for the remainder of the study ().
Figure 3. Mice receiving daily treatment of GGOHBP have a reduced whole body tumor burden as shown by BLI (a) Representative weekly BLI of control mouse C4M5 and treated mouse C2M2. The red dashed line indicates the missing photographs from weeks 5 and 6 due to a required temporary switch to the AMI instrument. Control mouse C4M5 died during imaging on day 75 (week 11) whereas treated mouse C2M2 was removed from the study on day 117 (between weeks 16 and 17). (b) Whole body photon counts averaged within the vehicle-control (solid line) and GGOHBP (dashed line) treated groups during weeks 1-7 when all mice were alive. (c) Whole body photon counts for the entirety of the study (weeks 1-19) averaged within the control and treated groups. Statistical significance indicated as * (P < 0.05) as determined by Student's T Test. Error bars indicate standard deviation. N = 9 control vehicle-treated mice. N = 8 GGOHBP treated mice.
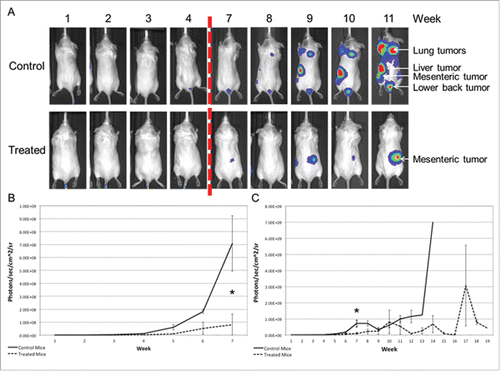
Figure 4. GGOHBP significantly slowed tumor development and prolonged overall survival Time until a whole body tumor burden of greater than (a) 107, (b) 108, or (c) 109 photon counts was detected for each mouse. (d) Weight of mice averaged within the control and treated groups. (e) Kaplan-Meier survival curve. Statistical significance indicated as * (P < 0.05) and ** (P < 0.005) as determined by Student's T Test. Error bars indicate standard deviation.
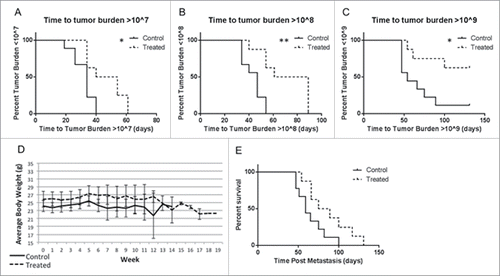
Since the study was intended to analyze the effects of GGDPS inhibition on the development of tumors, all mice were monitored by weekly BLI for a whole body photon count greater than 107, 108, or 109 (). As shown by Kaplan Meier plots, the treated mice took significantly longer to develop whole body photons counts greater than 107 (P < 0.05, ), 108 (P < 0.005, ), or 109 (P < 0.05, ) as compared to the control mice. At the end of the study, 8 out of 9 control mice (88.9%) had whole body tumor burdens greater than 109 () while only 3 out of 8 treated mice (37.5%) showed this level of tumor burden.
Considering the significant issue of weight loss in a previously published study using GGOHBP in mice with a high tumor burden,Citation52 weight was of high concern despite using a lower dose of GGOHBP. As shown by the averaged weights of control (solid line) and treated (dashed line) mice in , daily treatment with 1.25 mg/kg GGOHBP for up to 135 d did not cause significant weight loss. Correspondingly, the Kaplan Meier survival curve showed a notable, albeit non-significant (P = 0.0518), increase in the survival of the treated mice as compared to the control mice ().
Rap1A geranylgeranylation was reduced in all soft tissue tumors of treated mice and not in non-tumor burdened tissues or control mice
Next, we analyzed the effects of GGOHBP on Rap1A geranylgeranylation in non-tumor burdened and tumor burdened tissues by Western blot analysis using the anti-Rap1A antibody previously described. As shown by the lack of a Rap1A band compared to the positive control GGOHBP treatment in luciferase-expressing PC-3 cells, all non-tumor bearing heart (), liver (), kidney (), adrenal glands (), and lung () tissues of control and treated mice showed no detectable alterations in Rap1A geranylgeranylation. Conversely, the lung tumors (), thigh tumors (), mesenteric tumors (), kidney tumors (), liver tumors (), heart tumors (), thoracic cavity tumors (), adrenal gland tumors (), scapula region tumors (), lower back region tumors (), and diaphragm tumors () showed a reduction of Rap1A geranylgeranylation in all treated mice and none of the control mice.
Figure 5. Rap1A geranylgeranylation is not altered in non-tumor burdened heart, liver, kidney, adrenal glands, and lung tissues of GGOHBP treated mice Representative Western blot analysis of Rap1A in non-tumorous (a) heart, (b) liver, (c) kidney, (d) adrenal glands and (e) lung tissues from control and treated mice. Control mouse C4M5 had too high of a lung tumor burden to collect non-tumorous lung tissue and so was not included. The anti-Rap1A antibody detects the unprenylated form of Rap1A as shown by the positive control GGOHBP treatment in luciferase-expressing PC-3 cells.
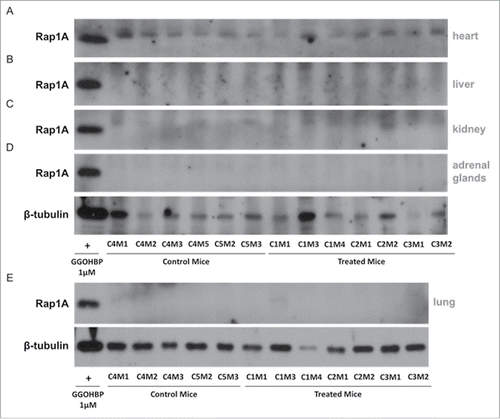
Figure 6. Rap1A geranylgeranylation is reduced in all soft tissue tumors of GGOHBP treated mice Representative Western blot analysis of Rap1A in (a) lung tumors, (b) thigh tumors, (c) mesenteric tumors, (d) kidney tumors, (e) liver tumors, (f) heart tumors, (g) thoracic cavity tumors, (h) adrenal gland tumors, (i) scapula tumors, (j) lower back tumors, and (k) diaphragm tumors of control and treated mice. The anti-Rap1A antibody detects the unprenylated form of Rap1A as shown by the positive control GGOHBP treatment in luciferase-expressing PC-3 cells.
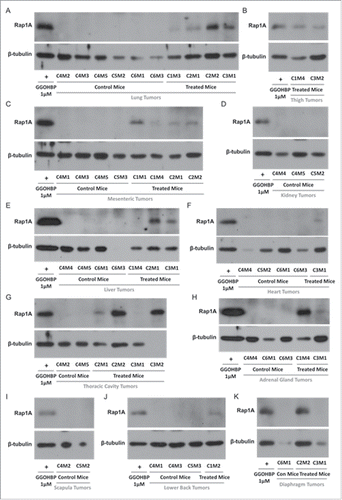
Discussion
Previously published work by our lab found novel compound GGOHBP to significantly reduce the weight of established adrenal gland tumors in treated mice as compared to control mice.Citation52 However, this prior study failed to identify an effect of GGOHBP to alter established bone tumors. We believe this is due to a reduced bone affinity of the novel GGDPS inhibitor compared to the clinically used NBPs. Consequently, in the treatment experiment the large tumor burden in the bones masked any effects of GGOHBP on whole body tumor burden.Citation52 In our present study we found GGOHBP significantly reduced whole body tumor burden, increased time until tumors developed, and prolonged overall survival. The observed discrepancy between the 2 animal models was likely due to dissimilar tumor distribution and the use of luciferase-expressing PC-3 cells as compared to luciferase-expressing 22Rv1 cells in the previous study. Our present study inoculated mice with PC-3-luc cells via tail vein injection, a method previously shown to favor soft tissue tumors. Additionally, we began daily GGOHBP or vehicle treatment 4 d prior to tumor injection, allowing us to study GGOHBP as a preventative of prostate cancer metastasis as opposed to a treatment of high tumor burden as published previously.
We decided use the PC-3 tail vein model with prophylactic treatment for several reasons. Firstly, the preventative dosing allowed the maximal response to the effects of GGOHBP to be observed. It was interesting to us that we saw only soft tissue effects in the 22Rv1 model. In this prior study using a treatment schedule where we waited for the tumors to establish we only saw a decrease in the adrenal gland tumors. Other sites of soft tissue metastasis were not prevalent enough to see any real difference, and the total tumor burden in all cases was driven by large metastatic burden in the jaw/maxillary region. While we believed that the nature of our compounds would allow higher distribution to extra-skeletal sites the complete lack of effect on tumor burden in the bone was striking. Several prior studies had documented increasing soft tissue tumor burden with prophylactically delivered bisphosphonates in breast cancer bone metastasis models.Citation54,55 Other models have shown conflicting results with one study showing an increase in skeletal tumor burden after treatment with pamidronate (a potent nitrogen bisphosphonate).Citation56 In the pamidronate study increased tumor burden in bone was accompanied by an increase in trabecular bone matrix.
Tumor inoculation by tail vein injection of the luciferase-expressing PC-3 cell line led to tumor development in soft tissues with no development in bone. In spite of assigning the control and treatment groups 4 d prior to tumor inoculation there was a fairly equal distribution of tumors between the 2 groups. Unlike our previous murine study using a higher dose of GGOHBP, weight loss was not an issue at 1.25 mg/kg daily for up to 135 d. The stability in weight observed in the present study was likely due to the intentional reduction of GGOHBP dosage. However, the mice in the previous murine study had a high tumor burden from the start of treatment, while the present preventative study showed lower and slower tumor development, likely also accounting for the stable weights.
In our previous in vitro work on the IBP we have routinely used Rap1A geranylgeranylation as a biomarker of target engagement. Because of the connection of Rap1A signaling and prostate cancer metastasisCitation27 as well its utility as a surrogate marker to assess for Rho family geranylgeranylation we utilized it in these current studies. Western blot analysis of non-tumor burdened and tumor-burdened tissues found a reduction in Rap1A geranylgeranylation in all tumors in the treatment group but none of the control mice or non-tumor bearing tissues. This robust pattern of target engagement in the tumor xenografts is likely due to the high expression of the Rap1A protein in the tumor cells. This anti-body has been shown to react with both human and murine Rap1A protein ruling out this as a source of the large differences in signal.Citation52 Consequently, the observed prolongation of time until tumors developed in the treated mice was likely due to the corresponding reduction in protein geranylgeranylation. Direct cytotoxic or growth inhibiting effects of GGOHBP accounting for the observed reduction in metastatic tumor burden cannot be ruled out. However, GGOHBP shows minimal effect on MTT activity in the luciferase-expressing PC-3 cells in vitro, suggesting it is not especially cytotoxic to these tumor cells.
In conclusion, we found GGOHBP inhibition of GGDPS significantly slowed soft tissue metastatic tumor development and increased the survival of treated mice in a preventative murine model of human prostate cancer metastasis. This reduction in soft tissue tumor burden corresponded to a biochemical reduction in Rap1A geranylgeranylation that was not seen in non-tumor burdened tissues or control mice, reiterating a role for protein geranylgeranylation in prostate cancer progression. Our studies here are limited to Rap1A as a biomarker of mechanism engagement with GGOHBP. It is not immediately clear if reduction in geranylgeranylated Rap1A is directly responsible for the decreased tumor burden or if other mechanisms are at play. It will be interesting to look at changes in total protein levels for this signaling molecule as well as the modified and unmodified levels of other key Rho family members such as Rac1 under these conditions. Studies aimed at the elucidation of the roles of other Rho family proteins in the effects of treatment with inhibitors of GGDPS are ongoing.
Materials and methods
Cell culture
Luciferase-expressing PC-3 cells were obtained from PerkinElmer (Boston, MA) and maintained in F12-K media supplemented with 10% heat-inactivated FBS, 1% L-glutamine, 1% amphotericin B, and 1% pen-strep (Life Technologies, Grand Island, NY). Cells were grown at 37°C with 5% CO2, split weekly, and discarded after 8 passages.
GGDPS enzyme assays
GGDPS enzyme assays were implemented as previously described.Citation57 Briefly, enzymes were diluted to 2 μg/mL in 10 mM HEPES pH 7.5, 500 mM NaCl, 5% glycerol, 1mM TCEP, and 5 μg/mL BSA and pre incubated with inhibitors in the reaction buffer (0.5 mM TCEP, 50 μg/mL BSA, 50 mM Tris pH 7.7, and 2 mM MgCl2) for 10 minutes at room temperature. Enzyme assay reactions were initiated by the simultaneous addition of 10 μM FPP and 10 μM14 C-IPP and allowed to proceed at 37°C for 15 min, at which point no more than 20% of the substrate was used. Reactions were terminated by the addition of 200 μL saturated NaCl (1 g NaCl in 2.8 mL double deionized water) and isoprenoids were extracted with 1 mL saturated butanol. Liquid scintillation counting was used to detect incorporated14C.
MTT activity assays
Luciferase-expressing PC-3 cells were plated in a 96-well plate at 10,000 cells/well in supplemented F12-K media and given 24 hrs to adhere before initiating treatment with the indicated concentrations for 44 hrs. After 3-(4,5-dimethylthiazole-2-yl)-2,5-diphenyltetrazolium bromide (MTT) was added at 0.6 mg/mL, the plates were incubated at 37°C for 4 hrs followed by the addition of MTT stop solution (80% 2-propanol, 10% Triton X-100, and 10% 1N HCl) with gentle agitation overnight. Absorbance was measured at 540 nm with a 650 nm reference wavelength.
TX-114 separationCitation58
After 48 hrs of treatment, luciferase-expressing PC-3 cells were lysed in Triton X-114 (TX-114) detergent (1% TX-114, 150 mM NaCl, 20 mM Tris). Lysates were incubated at 37°C until they became cloudy at which point they were centrifuged for 2 min at 14,000 g and immediately separated into their aqueous (supernatant) and detergent (pellet) phases. TX-114 was added to the aqueous phases at 1% of the volume. Buffer B (150 mM NaCl and 20 mM Tris) was added to the detergent phases to bring them to ¼ the volume of their respective aqueous phases. Both phases were incubated at 37°C until they became cloudy followed by centrifugation at 14,000 g for 2 min, immediate separation of the aqueous and detergent phases, and the addition of TX-114 or Buffer B respectively, repeating an additional 3 times. Following the final separation of phases, Buffer B was added to the detergent phases to bring them to the same volume as their respective aqueous phases. TX-114 was not added to the final separation of the aqueous phases. Protein levels were quantified using the BCA assay method.Citation59
Western blot analysis
Luciferase-expressing PC-3 cells were plated at 1 million cells/plate in 60 mm culture dishes for 24 hrs followed by treatment with the indicated concentrations of compounds for 48 hrs. Cells were lysed in RIPA buffer (0.15 M NaCl, 0.05 M Tris HCl, 1% w/v sodium deoxycholate, 1% w/v SDS, 1% w/v TX-100, and 1 mM EDTA) or TX-114 detergent as indicated, both supplemented with 1:100 phenylmethylsulfonyl fluoride and protease inhibitor cocktail. Protein levels were quantified by the BCA assay method.Citation59 Equal protein quantities were resolved by SDS-polyacrylamide gel electrophoresis and transferred at 4°C overnight onto polyvinylidene fluoride membranes. Membranes were blocked in 5% non-fat dry milk diluted in TBS-TW at 37°C for 45 min. The anti-pan-Ras (diluted 1:200), anti-Rap1A (diluted 1:250), anti-Rab6 (diluted 1:200), anti-β-tubulin (diluted 1:250), and anti-calnexin (diluted 1:1000) primary antibodies were incubated on a rotisserie at 4°C overnight. Secondary antibodies were diluted 1:2000 and incubated on a rotisserie at 37°C for 1 hr. Proteins were visualized using ECL detection (GE Healthcare, Buckinghamshire, UK). Anti-pan-Ras was obtained from BD Biosciences (San Jose, CA). Anti-Rap1A (sc-1482), anti-Rab6 (sc-310), anti-β-tubulin (sc-9140), anti-calnexin (sc-23954) and secondary antibody donkey anti-goat IgG-HRP (sc-2033) were obtained from Santa Cruz Biotechnology (Santa Cruz, CA). Secondary HRP-conjugated anti-mouse (NXA931) and anti-rabbit (NA934) were obtained from GE Healthcare (Buckinghamshire, UK).
Preventative metastatic murine model
All animal work was completed in accordance with University of Iowa Animal Care and Use Committee policies. Male SCID mice aged 6-8 weeks were randomized into 2 groups prior to treatment (treatment group N = 8, and control group N = 9). Daily subcutaneous injections of 1.25 mg/kg GGOHBP or the PBS-vehicle began on day 1. Four days later, 6 × 106 luciferase-expressing PC-3 cells were injected into the tail vein of each mouse. Tumor burden was monitored weekly using bioluminescence imaging (BLI). Following a greater than 20% weight loss or poor body condition, mice were euthanized, ex vivo BLI completed, and all adrenal glands, kidneys, liver, lungs, heart and any tumors identified by ex vivo BLI were collected.
Tissue harvesting and lysing
Frozen non-tumor burdened and tumor-burdened tissues were thawed on ice and weighed. Tissue aliquots of approximately 0.05 g were collected and lysed in RIPA buffer (0.15 M NaCl, 0.05 M Tris HCl, 1% w/v sodium deoxycholate, 1% w/v Triton-X 10, 1 mM EDTA) containing 1:100 protease inhibitor cocktail and phenylmethylsulfonyl fluoride. Tissues were sonicated, incubated at room temperature for 30 min with constant agitation, and centrifuged at 14000 g for 20 min at 4°C, collecting the supernatant and repeating centrifugation.
Disclosure of potential conflicts of interest
Ray Hohl and Jeffrey Neighbors are named as inventors on patents held by the University of Iowa Research Foundation that describe preparation of some GGDPS inhibitors as well as their use as potential anti-cancer agents. We are also founders of the company Terpenoid Therapeutics, Inc. and hold equity interest in this company.
Acknowledgments
The authors would like to thank Professor James E. Dunford at the University of Oxford for kindly providing the GGDPS enzyme. DGBP and GGOHBP were supplied by Terpenoid Therapeutics Inc. Coralville, IA.
Funding
The National Institute of Health Pharmacological Sciences Training Grant T32 GM067795 supported this work. This project was supported in part by the Roy J. Carver Charitable Trust, the Roland W. Holden Family Program for Experimental Therapeutics.
References
- Pirtskhalaishvili G, Hrebinko RL, Nelson JB. The treatment of prostate cancer: an overview of current options. Cancer Pract 2001; 9(6):295-306; PMID:11879332; http://dx.doi.org/10.1046/j.1523-5394.2001.96009.x
- Mettlin C. Changes in patterns of prostate cancer care in the United States: results of American College of Surgeons Commission on Cancer studies, 1974–1993. Prostate 1997; 32(3):221-6; PMID:9254902; http://dx.doi.org/10.1002/(SICI)1097-0045(19970801)32:3%3c221::AID-PROS9%3e3.0.CO;2-N
- Oh WK, Kantoff PW. Management of hormone refractory prostate cancer: current standards and future prospects. J Urol 1998; 160(4):1220-9; PMID:9751323; http://dx.doi.org/10.1016/S0022-5347(01)62501-1
- O'Brien LE, Jou TS, Pollack AL, Zhang QH, Hansen SH, Yurchenco P, Mostov KE. Rac1 orientates epithelial apical polarity through effects on basolateral laminin assembly. Nat Cell Biol 2001; 3(9):831-8; PMID:11533663; http://dx.doi.org/10.1038/ncb0901-831
- Matsumoto Y, Tanaka K, Harimaya K, Nakatani F, Matsuda S, Iwamoto Y. Small GTP-binding protein, Rho, both increased and decreased cellular motility, activation of matrix metalloproteinase 2 and invasion of human osteosarcoma cells. Japanese J Cancer Res 2001; 92(4):429-38; PMID:11346466; http://dx.doi.org/10.1111/j.1349-7006.2001.tb01113.x
- Worthylake RA, Lemoine S, Watson JM, Burridge K. RhoA is required for monocyte tail retraction during transendothelial migration. J Cell Biol 2001; 154(1):147-60; PMID:11448997; http://dx.doi.org/10.1083/jcb.200103048
- Braga VMM, Betson M, Li XD, Lamarche-Vane N. Activation of the small GTPase Rac is sufficient to disrupt cadherin-dependent cell-cell adhesion in normal human keratinocytes. Mol Biol Cell 2000; 11(11):3703-21; PMID:11071901; http://dx.doi.org/10.1091/mbc.11.11.3703
- Braga VMM, Machesky LM, Hall A, Hotchin NA. The small GTPases rho and rac are required for the establishment of cadherin-dependent cell-cell contacts. J Cell Biol 1997; 137(6):1421-31; PMID:9182672; http://dx.doi.org/10.1083/jcb.137.6.1421
- Quinlan MP. Rac regulates the stability of the adherens junction and its components, thus affecting epithelial cell differentiation and transformation. Oncogene 1999; 18(47):6434-42; PMID:10597245; http://dx.doi.org/10.1038/sj.onc.1203026
- Zondag GCM, Evers EE, ten Klooster JP, Janssen L, van der Kammen RA, Collard JG. Oncogenic Ras downregulates Rac activity, which leads to increased Rho activity and epithelial-mesenchymal transition. J Cell Biol 2000; 149(4):775-81; PMID:10811819; http://dx.doi.org/10.1083/jcb.149.4.775
- Clark EA, Golub TR, Lander ES, Hynes RO. Genomic analysis of metastasis reveals an essential role for RhoC. Nature 2000; 406(6795):532-5; PMID:10952316; http://dx.doi.org/10.1038/35020106
- Maltese WA. Posttranslational modification of proteins by isoprenoids in mammalian-cells. Faseb J 1990; 4(15):3319-28; PMID:2123808
- Adamson P, Etienne S, Couraud PO, Calder V, Greenwood J. Lymphocyte migration through brain endothelial cell monolayers involves signaling through endothelial ICAM-1 via a Rho-dependent pathway. J Immunol 1999; 162(5):2964-73
- Clarke S. Protein isoprenylation and methylation at carboxyl-terminal cysteine residues. Ann Rev Biochem 1992; 61:355-86; PMID:1497315; http://dx.doi.org/10.1146/annurev.bi.61.070192.002035
- Lyons LS, Rao S, Balkan W, Faysal J, Maiorino CA, Burnstein KL. Ligand-Independent Activation of Androgen Receptors by Rho GTPase Signaling in Prostate Cancer. Mol Endocrinol 2008; 22(3):597-608; PMID:18079321; http://dx.doi.org/10.1210/me.2007-0158
- Knight-Krajewski S, Welsh CF, Liu Y, Lyons LS, Faysal JM, Yang ES, Burnstein KL. Deregulation of the Rho GTPase, Rac1, suppresses cyclin-dependent kinase inhibitor p21(CIP1) levels in androgen-independent human prostate cancer cells. Oncogene 2004; 23(32):5513-22; PMID:15077174; http://dx.doi.org/10.1038/sj.onc.1207708
- Engers R, Ziegler S, Mueller M, Walter A, Willers R, Gabbert HE. Prognostic relevance of increased Rac GTPase expression in prostate carcinomas. Endocr Relat Cancer 2007; 14(2):245-56; PMID:17639041; http://dx.doi.org/10.1677/ERC-06-0036
- Lin K-T, Gong J, Li C-F, Jang T-H, Chen W-L, Chen H-J, Wang L-H. Vav3-Rac1 Signaling Regulates Prostate Cancer Metastasis with Elevated Vav3 Expression Correlating with Prostate Cancer Progression and Posttreatment Recurrence. Cancer Research 2012; 72(12):3000-9; PMID:22659453; http://dx.doi.org/10.1158/0008-5472.CAN-11-2502
- Qin J, Xie Y, Wang B, Hoshino M, Wolff DW, Zhao J, Scofield MA, Dowd FJ, Lin MF, Tu Y. Upregulation of PIP3-dependent Rac exchanger 1 (P-Rex1) promotes prostate cancer metastasis. Oncogene 2009; 28(16):1853-63; PMID:19305425; http://dx.doi.org/10.1038/onc.2009.30
- Kobayashi T, Inoue T, Shimizu Y, Terada N, Maeno A, Kajita Y, Yamasaki T, Kamba T, Toda Y, Mikami Y, Yamada T, Kamoto T, Ogawa O, Nakamura E. Activation of Rac1 Is Closely Related to Androgen-Independent Cell Proliferation of Prostate Cancer Cells Both in Vitro and in Vivo. Mol Endocrinol 2010; 24(4):722-34; PMID:20203103; http://dx.doi.org/10.1210/me.2009-0326
- Suzuki S, Pitchakarn P, Sato S, Shirai T, Takahashi S. Apocynin, an NADPH oxidase inhibitor, suppresses progression of prostate cancer via Rac1 dephosphorylation. Exp Toxicol Pathol 2013; 65(7-8):1035-41; PMID:23664830; http://dx.doi.org/10.1016/j.etp.2013.03.002
- Hattori M, Minato N. Rap1 GTPase: Functions, regulation, and malignancy. J Biochem 2003; 134(4):479-84; PMID:14607972; http://dx.doi.org/10.1093/jb/mvg180
- Yajnik V, Paulding C, Sordella R, McClatchey AI, Saito M, Wahrer DCR, Reynolds P, Bell DW, Lake R, van den Heuvel S, Settleman J, Haber DA. DOCK4, a GTPase activator, is disrupted during tumorigenesis. Cell 2003; 112(5):673-84; PMID:12628187; http://dx.doi.org/10.1016/S0092-8674(03)00155-7
- Zhang LZ, Li CW, Mahmood R, van Golen K, Greenson J, Li GY, D'Silva NJ, Li XQ, Burant CF, Logsdon CD, Simeone DM. Identification of a putative tumor suppressor gene Rap1GAP in pancreatic cancer. Cancer Res 2006; 66(2):898-906; PMID:16424023; http://dx.doi.org/10.1158/0008-5472.CAN-05-3025
- Gao L, Feng YF, Bowers R, Becker-Hapak M, Gardner J, Council L, Linette G, Zhao HB, Cornelius LA. Ras-associated protein-1 regulates extracellular signal-regulated kinase activation and migration in melanoma cells: Two processes important to melanoma tumorigenesis and metastasis. Cancer Res 2006; 66(16):7880-8; PMID:16912161; http://dx.doi.org/10.1158/0008-5472.CAN-06-0254
- Crawford NPS, Ziogas A, Peel DJ, Hess J, Anton-Culver H, Hunter KW. Germline polymorphisms in SIPA1 are associated with metastasis and other indicators of poor prognosis in breast cancer. Breast Cancer Res 2006; 8(2); PMID:16563182; http://dx.doi.org/10.1186/bcr1389
- Bailey CL, Kelly P, Casey PJ. Activation of Rap1 Promotes Prostate Cancer Metastasis. Cancer Res 2009; 69(12):4962-8; PMID:19470770; http://dx.doi.org/10.1158/0008-5472.CAN-08-4269
- Xiang J, Bian CD, Wang H, Huang SS, Wu DL. MiR-203 down-regulates Rap1A and suppresses cell proliferation, adhesion and invasion in prostate cancer. J Exp Clin Cancer Res 2015; 34; http://dx.doi.org/10.1186/s13046-015-0125-x
- O'Brien LE, Jou TS, Pollack AL, Zhang Q, Hansen SH, Yurchenco P, Mostov KE. Rac1 orientates epithelial apical polarity through effects on basolateral laminin assembly. Nat Cell Biol 2001; 3:831-8; PMID:11533663; http://dx.doi.org/10.1038/ncb0901-831
- Worthylake RA, Lemoine S, Watson JM, Burridge K. RhoA is required for monocyte tail retraction during transendothelial migration. The J Cell Biol 2001; 154(1):147-160; PMID:11448997; http://dx.doi.org/10.1083/jcb.200103048
- Matsumoto Y, Tanaka K, Harimaya K, Nakatani F, Matsuda S, Iwamoto Y. Small GTP-binding protein, Rho, both increased and decreased cellular motility, activation of matrix metalloproteinase 2 and invasion of human osteosarcoma cells. Jpn J Cancer Res 2001; 92:429–438
- Giehl K. Oncogenic Ras in tumour progression and metastasis. Biol Chem 2005; 386(3):193-205; PMID:15843165
- Faried A, Faried LS, Kimura H, Nakajima M, Sohda M, Miyazaki T, Kato H, Usman N, Kuwano H. RhoA and RhoC proteins promote both cell proliferation and cell invasion of human oesophageal squamous cell carcinoma cell lines in vitro and in vivo. Eur J Cancer 2006; 42(10):1455-65; PMID:16750623; http://dx.doi.org/10.1016/j.ejca.2006.02.012
- Mack NA, Whalley HJ, Castillo-Lluva S, Malliri A. The diverse roles of Rac signaling in tumorigenesis. Cell Cycle 2011; 10(10):1571-1581; PMID:21478669; http://dx.doi.org/10.4161/cc.10.10.15612
- Thurnher M, Gruenbacher G, Nussbaumer O. Regulation of mevalonate metabolism in cancer and immune cells. Biochim Biophys Acta 2013; 1831(6):1009-15; PMID:23524243; http://dx.doi.org/10.1016/j.bbalip.2013.03.003
- Zhu Y, Casey PJ, Kumar AP, Pervaiz S. Deciphering the signaling networks underlying simvastatin-induced apoptosis in human cancer cells: evidence for non-canonical activation of RhoA and Rac1 GTPases. Cell Death Dis 2013; 4:e568; PMID:23559002; http://dx.doi.org/10.1038/cddis.2013.103
- Jaiswal M, Dvorsky R, Amin E, Risse SL, Fansa EK, Zhang SC, Taha MS, Gauhar AR, Nakhaei-Rad S, Kordes C, Koessmeier KT, Cirstea IC, Olayioye MA, Haussinger D, Ahmadian MR. Functional cross-talk between ras and rho pathways: a Ras-specific GTPase-activating protein (p120RasGAP) competitively inhibits the RhoGAP activity of deleted in liver cancer (DLC) tumor suppressor by masking the catalytic arginine finger. J Biol Chem 2014; 289(10):6839-49; PMID:24443565; http://dx.doi.org/10.1074/jbc.M113.527655
- Demierre MF, Higgins PD, Gruber SB, Hawk E, Lippman SM. Statins and cancer prevention. Nat Rev Cancer 2005; 5(12):930-42; PMID:16341084; http://dx.doi.org/10.1038/nrc1751
- Bjarnadottir O, Romero Q, Bendahl PO, Jirstrom K, Ryden L, Loman N, Uhlen M, Johannesson H, Rose C, Grabau D, Borgquist S. Targeting HMG-CoA reductase with statins in a window-of-opportunity breast cancer trial. Breast Cancer Res Treat 2013; 138(2):499-508; PMID:23471651; http://dx.doi.org/10.1007/s10549-013-2473-6
- Zhang J, Yang Z, Xie L, Xu L, Xu D, Liu X. Statins, autophagy and cancer metastasis. Int J Biochem Cell Biol 2013; 45(3):745-52; PMID:23147595; http://dx.doi.org/10.1016/j.biocel.2012.11.001
- Roy M, Kung HJ, Ghosh PM. Statins and prostate cancer: role of cholesterol inhibition vs. prevention of small GTP-binding proteins. American Journal of Cancer Research 2011; 1(4):542-561; PMID:21984972
- Russell RGG, Rogers MJ. Bisphosphonates: From the laboratory to the clinic and back again. Bone 1999; 25(1):97-106; PMID:10423031; http://dx.doi.org/10.1016/S8756-3282(99)00116-7
- Russell RGG. Bisphosphonates: The first 40 years. Bone 2011; 49(1):2-19; PMID:21555003; http://dx.doi.org/10.1016/j.bone.2011.04.022
- Coxon JP, Pickering LM, Colston KW. Zoledronic acid inhibits adhesion of breast and prostate cancer cells via inhibition of protein prenylation. Journal of Bone and Mineral Research 2003; 18(7):1359-9
- Coxon JP, Pickering LM, Kirby RS, Colston KW. Zoledronic acid inhibits adhesion of prostate cancer cells to mineralized matrix principally through inhibition of protein geranylgeranylation via a caspase-independent mechanism. Journal of Bone and Mineral Research 2004; 19(9):1598-8
- Eidtmann H, Bundred NJ, DeBoer R, Llombart A, Davidson N, Neven P, Von MG, Miller J, Schenk A, Coleman R. The effect of zoledronic acid on aromatase inhibitor associated bone loss in postmenopausal women with early breast cancer receiving adjuvant letrozole: 36 months follow-up of ZO-FAST. Cancer Res 2009; 69(2):74S-74S
- Gnant M, Mlineritsch B, Luschin-Ebengreuth G, Kainberger F, Kaessmann H, Piswanger-Soelkner JC, Seifert M, Ploner F, Menzel C, Dubsky P, et al. Adjuvant endocrine therapy plus zoledronic acid in premenopausal women with early-stage breast cancer: 5-year follow-up of the ABCSG-12 bone-mineral density substudy. Lancet Oncol 2008; 9(9):840-9; PMID:18718815; http://dx.doi.org/10.1016/S1470-2045(08)70204-3
- Gnant M, Mlineritsch B, Schippinger W, Luschin-Ebengreuth G, Postlberger S, Menzel C, Jakesz R, Seifert M, Hubalek M, Bjelic-Radisic V, et al. Endocrine Therapy plus Zelodronic Acid in Premenopausal Breast Cancer (vol 360, pg 679, 2009). N Engl J Med 2009; 360(22):2379-2379
- Ben-Aharon I, Vidal L, Rizel S, Yerushalmi R, Shpilberg O, Sulkes A, Stemmer SM. Bisphosphonates in the Adjuvant Setting of Breast Cancer Therapy-Effect on Survival: A Systematic Review and Meta-Analysis. Plos One 2013; 8(8):9; http://dx.doi.org/10.1371/journal.pone.0070044
- Dudakovic A, Tong H, Hohl RJ. Geranylgeranyl diphosphate depletion inhibits breast cancer cell migration. Invest New Drugs 2011; 29(5):912-20; PMID:20480384; http://dx.doi.org/10.1007/s10637-010-9446-y
- Wasko BM, Dudakovic A, Hohl RJ. Bisphosphonates Induce Autophagy by Depleting Geranylgeranyl Diphosphate. Journal of Pharmacology and Experimental Therapeutics 2011; 337(2):540-6; PMID:21335425; http://dx.doi.org/10.1124/jpet.110.175521
- Reilly JE, Neighbors JD, Tong H, Henry MD, Hohl RJ. Targeting geranylgeranylation reduces adrenal gland tumor burden in a murine model of prostate cancer metastasis. Clin Exp Metastasis 2015; 32(6):555-66; PMID:26070429; http://dx.doi.org/10.1007/s10585-015-9727-0
- Shevrin DH, Gorny KI, Kukreja SC. Patterns of metastasis by the human-prostate cancer cell-line PC-3 in athymic nude-mice. Prostate 1989; 15(2):187-94; PMID:2529482; http://dx.doi.org/10.1002/pros.2990150212
- Sasaki A, Boyce BF, Story B, Wright KR, Chapman M, Boyce R, Mundy GR, Yoneda T. Bisphosphonate risedronate reduces metastatic human breast-cancer burden in bone in nude-mice. Cancer Research 1995; 55(16):3551-7; PMID:7627963
- Yoneda T, Michigami T, Yi B, Williams PJ, Niewolna M, Hiraga T. Use of bisphosphonates for the treatment of bone metastasis in experimental animal models. Cancer Treatment Reviews 1999; 25(5):293-9; PMID:10544073; http://dx.doi.org/10.1053/ctrv.1999.0133
- Kostenuik PJ, Orr FW, Suyama K, Singh G. Increased growth-rate and tumor burden of spontaneously metastatic walker-256 cancer-cells in the skeleton of bisphosphonate-treated rats. Cancer Research 1993; 53(22):5452-7; PMID:8221685
- Dunford JE, Thompson K, Coxon FP, Luckman SP, Hahn FM, Poulter CD, Ebetino FH, Rogers MJ. Structure-activity relationships for inhibition of farnesyl diphosphate synthase in vitro nd inhibition of bone resorption in vivo by nitrogen-containing bisphosphonates. Journal of Pharmacology and Experimental Therapeutics 2001; 296(2):235-42; PMID:11160603
- Hancock JF, Magee AI, Childs JE, Marshall CJ. ALL RAS PROTEINS ARE POLYISOPRENYLATED BUT ONLY SOME ARE PALMITOYLATED. Cell 1989; 57(7):1167-77; PMID:2661017; http://dx.doi.org/10.1016/0092-8674(89)90054-8
- Smith PK, Krohn RI, Hermanson GT, Mallia AK, Gartner FH, Provenzano MD, Fujimoto EK, Goeke NM, Olson BJ, Klenk DC. Measurement of Protein Using Bicinchoninic Acid. Analytical Biochemistry 1985; 150(1):76-85; PMID:3843705; http://dx.doi.org/10.1016/0003-2697(85)90442-7