ABSTRACT
Monocyte chemoattractant protein 1 (MCP-1) has been implicated as a major modulator in the progression of mammary tumorigenesis, largely due to its ability to recruit macrophages to the tumor microenvironment. Macrophages are key mediators in the connection between inflammation and cancer progression and have been shown to play an important role in tumorigenesis. Thus, MCP-1 may be a potential therapeutic target in inflammatory and difficult-to-treat cancers such as triple negative breast cancer (TNBC). We examined the effect of MCP-1 depletion on mammary tumorigenesis in a model of TNBC. Tumor measurements were conducted weekly (until 22 weeks of age) and at sacrifice (23 weeks of age) in female C3(1)/SV40Tag and C3(1)/SV40Tag MCP-1 deficient mice to determine tumor numbers and tumorvolumes. Histopathological scoring was performed at 12 weeks of age and 23 weeks of age. Gene expression of macrophage markers and inflammatory mediators were measured in the mammary gland and tumor microenvironment at sacrifice. As expected, MCP-1 depletion resulted in decreased tumorigenesis, indicated by reduced primary tumor volume and multiplicity, and a delay in tumor progression represented by histopathological scoring (12 weeks of age). Deficiency in MCP-1 significantly downregulated expression of macrophage markers in the mammary gland (Mertk and CD64) and the tumor microenvironment (CD64), and also reduced expression of inflammatory cytokines in the mammary gland (TNFα and IL-1β) and the tumor microenvironment (IL-6). These data support the hypothesis that MCP-1 expression contributes to increased tumorigenesis in a model of TNBC via recruitment of macrophages and subsequent increase in inflammatory mediators.
Introduction
Breast cancer is the most common cancer and the second leading cause of cancer death among women worldwide.Citation1 In the United States alone, close to 250,000 new cases of invasive breast cancer will be diagnosed and over 40,000 breast cancer deaths will occur in the year 2016.Citation2 The molecular heterogeneity of breast cancers is now well recognized, with at least 5 different subtypes identified through molecular profiling.Citation3 Triple negative breast cancer (TNBC) represents a subtype that more frequently affects younger women and is associated with a high mortality rate.Citation4,5 Triple negative cancers represent between 10–20% of breast cancer cases and are defined as tumors that lack the estrogen receptor (ER), progesterone receptor (PR), and human epidermal growth factor receptor type 2 (Her2/neu) that are known to fuel most breast cancers. TNBC often present as interval cancers (i.e. detected/presenting within 12 months after a mammographic screening in which findings are considered normal) and are significantly more aggressive than tumors of other molecular subtypes.Citation4,6,7 Patients with triple negative cancers have a shorter survival following the first metastatic event,Citation8 and the majority of deaths occur in the first 5 y following diagnosis, despite therapy.Citation9
The majority of breast cancer clinical subtypes respond well to endocrine and targeted Her2 therapies, but due to the lack of well defined clinical targets, TNBCs are nonresponsive to these treatment options and consequently, chemotherapy is the standard of care treatment.Citation10 To treat TNBC more effectively, new molecular targets need to be identified. MCP-1 represents a promising molecular target for therapeutic intervention. MCP-1 (CCL2) is a member of the C-C chemokine family that bind to G protein coupled receptors to regulate macrophage recruitment during wound healing, infection, and inflammation.Citation11 In mammary tumors, overexpression of MCP-1 correlates with increased recruitment of macrophages and infiltration into the tumor environment that can facilitate tumor progression through macrophage-mediated angiogenesisCitation12 and through secretion of growth and survival factors.Citation13 Recent studies demonstrate that MCP-1 also signals to breast cancer cells to regulate survival and invasionCitation14 as well as promote primary tumor growth.Citation15 While these reports indicate that MCP-1 can regulate multiple mechanisms involved in breast cancer progression, its role in TNBC has not yet been fully elucidated.
The purpose of this study was to examine the role of MCP-1 on mammary tumorigenesis in the triple negative C3(1)SV40Tag transgenic mouse model of breast cancer. This was done by crossing the C3(1)SV40Tag mouse, with an MCP-1 knockout mouse to develop an MCP-1 deficient model of mammary tumorigenesis. C3(1)SV40Tag mice lack expression of ER, PR and Her2/neu, and the absence of these has been associated with poor prognosis.Citation16 The overexpression of the early region of SV40 in the mammary epithelium induces mammary tumors in this model due, at least in part, to Tag inactivation of the tumor suppressors p53 and Rb. The expression of this transgene results in the progressive development of mammary lesions that lead to invasive carcinoma formation.Citation17 C3(1)SV40Tag mice develop mammary epithelial atypia at 8 wks of age that progresses to mammary intraepithelial neoplasia (MIN) at 12 wks, which is histologically similar to human ductal carcinoma in situ (DCIS). Invasive carcinomas usually develop at approximately 16 wks of age. We hypothesized that MCP-1 deficiency would reduce primary tumor initiation and tumor growth in a triple negative mouse model of breast cancer and that this would be associated with reduced macrophage markers and inflammatory mediators in the mammary gland and tumor tissue.
Materials & methods
Animals
Female FVB/N mice were bred with male heterozygous C3(1)/SV40Tag mice (a gift from Dr. Jeffrey Green, Chief, Transgenic Oncogenesis and Genomics Section, Laboratory of Cancer Biology and Genetics, National Cancer Institute). The MCP1−/− mice on the FVB/N background were developed in our laboratory. The MCP-1 deficient mice are available at The Jackson Laboratory as Stock No. 030109. Briefly, FVB/N mice were crossed with the C57BL/6 MCP1−/− strain, and then backcrossed an additional 8 times to derive the FVB/N MCP1−/− strain.Citation18 Female MCP1−/− FVB/N mice were then bred with male heterozygous C3(1)/SV40Tag MCP1−/− mice to derive the C3(1)SV40/Tag MCP1−/− strain on the FVB/N background. Female C3(1)/SV40Tag (n = 21) and C3(1)/SV40Tag/MCP−/− (n = 18) offspring were used in these experiments. All experimental mice were bred and cared for in the animal research facility at the University of South Carolina. They were housed, 2–5/cage, maintained on a 12:12-h light-dark cycle in a low stress environment (22°C, 50% humidity, low noise) and given food and water ad libitum. Principles of laboratory animal care were followed, and the Institutional Animal Care and Usage Committee of the University of South Carolina approved all experiments.
Genotyping protocol
Female C3(1)/SV40Tag and C3(1)/SV40Tag/MCP−/− mice were used in all experiments and were genotyped by tail snip at 3 weeks of age for the C3(1) 5′ flanking sequence and MCP-1 gene ablation status using the primer sequences as follows:
C3(1) forward - CAG TGG TTC CCA GAG TCT CA
Tag reverse - CAG AAG CCT CCA AAG TCA GG
MCP-1 Common - TCA TTG GGA TCA TCT TGC TG
MCP-1 Wild-type - TGA CAG TCC CCA GAG TCA CA
MCP-1 Mutant - GCC AGA GGC CAC TTG TGT AG
A snip of mouse tail is added to 150ul of DirectPCR tail buffer (Viagen Biotech Inc., Los Angeles, CA) and 2ul of Proteinase K (Viagen Biotech Inc., Los Angeles, CA) and digested at 55°C overnight. The next day samples were incubated at 95°C for an hour then added to a PCR cocktail for amplification. The PCR cocktail contains DNA template, upstream and downstream primers, ddH20, and GoTaq Green Master Mix (Promega Corp). Samples were run on 2% agarose gel and compared with control samples to determine genotype (556 base pair molecular weight for C3(1)/SV40Tag positive samples).
Diets
The AIN-76A diet was adminsitered to all experimental groups beginning at 4 weeks of age (Bioserv).Citation21 AIN-76A is a purified, balanced diet that is phytoestrogen free. Dietary phytoestrogens have been shown to influence anxiety-related behaviors, fat deposition, blood insulin, leptin and thyroid levels as well as lipogenesis and lipolysis in adipocytes.Citation22
Body weights and tumor palpations
Body weight was monitored weekly. C3(1)/SV40Tag mice typically develop palpable mammary tumors between 12 and 16 weeks of age.Citation19 Tumors were palpable beginning at 12 weeks of age and were measured weekly until 22 weeks of age by the same investigator. Upon palpation of a tumor, calipers were used to measure the longest and shortest diameter of the tumor. The number of tumors within each mouse was recorded and the tumor volume was estimated for each tumor using the formula: 0.52 × (largest diameter) × (smallest diameter)Citation2 as described previously.Citation20
Tissue collection
At 23 weeks of age mice (C3(1)/SV40Tag (n = 15) and C3(1)/SV40Tag/MCP−/− (n = 12)) were killed for tissue collection. We selected this timepoint to avoid the risk of having to sacrifice mice earlier than a pre-determined sacrifice timepoint (i.e 23 weeks in this study); based on our experience with this model mice that reach >24 weeks of age may develop tumors that exceed our IACUC end point criteria. An additional group of mice (C3(1)/SV40Tag (n = 6) and C3(1)/SV40Tag/MCP−/− (n = 6)) were killed at an earlier timepoint (12 weeks of age) for histopathological analysis only. Palpable tumors were dissected from mammary glands and measured grossly to determine tumor volume. A portion of the remaining thoracic mammary gland tissue was then removed from both the right and left side. This tissue was either snap frozen in liquid nitrogen for gene expression analysis or fixed in 4% formaldehyde for immunohistochemical analysis.
Histology
At sacrifice, a portion of mammary gland adipose tissue was excised from each mouse, fixed overnight in 4% formaldehyde, dehydrated with 70% alcohol, and embedded in wax. Paraffin sections were stained with hematoxylin and eosin (H&E). The mammary gland from mice aged 12 and 23 weeks was subsequently evaluated blindly by a pathologist and characterized according to histological alterations observed and the grade of dysplastic changes existed as: no hyperplasia or dysplasia, atypical ductal hyperplasia (ADH)/mammary intraepithelial neoplasia (MIN) low grade, ductal carcinoma in situ (DCIS)/MIN high grade, or invasive adenocarcinoma.Citation19
Real-time quantitative PCR
Mammary adipose tissue total RNA was extracted using Qiagen AllPrep DNA/RNA/Protein minikit (Qiagen). Briefly, DNA was bound to DNAEasy columns supplied by the kit and isolation was performed according to manufacturer instructions. 100% ethanol was added to the flow through from the DNA bound colums which contained the RNA. The RNA-containing flowthrough was then bound to the RNAEasy columns supplied by the kit and centrifuged for 15s and 8000 × g. A series of washes were performed using proprietary buffers included with the kit and the RNA was eluted using RNA-free water. Primary tumor tissue total RNA was extracted using TRIzol reagent (Invitrogen) and chloroform/isopropyl alcohol extraction as described previously.Citation23 Quantification of mammary adipose tissue mRNA gene expression was performed for macrophage markers (CD64 (Fcgr1), which encodes the immunoglobulin receptor CD64 on macrophages and Mer tyrosine kinase (Mertk),which is involved in the phagocytosis of apoptotic cells), cytokines (interleukin (IL)-6 (IL-6), tumor necrosis factor α (TNFα), IL-1β, IL-12, IL-10), and the chemokine (MCP-1). Quanitification of primary tumor mRNA gene expression was performed for macrophage markers (CD64 and Mertk) and cytokines (IL-6, TNFα, and IL1β) (Applied Biosystems, Foster City, CA) as described previously.Citation24 RNA quality and concentration was analyzed using a NanoPhotometer Pearl (Implen). Murine 18s rRNA was used as the housekeeping gene to normalize all of the data obtained. Quantitative reverse transcriptase polymerase chain reaction analysis was performed as per the manufacturer's instructions and all gene expression primers were TaqMan Gene Expression Assays (Applied Biosystems).
Statistical analysis
All data were analyzed using commercial software (GraphPad Software, Prism 7). A 2 tailed t-test was used to compare differences across groups. The non-parametric Mann-Whitney U test was used to compare group differences in histopathological stage within each timepoint. Statistical significance was set with an α value of P ≤ 0.05. Data are represented as mean ± SEM.
Results
MCP-1 deficiency decreases tumor volume and tumor number in mammary primary tumor palpations
Body weight measurements were taken weekly throughout the experimental period to confirm normal growth patterns following deletion of MCP-1. MCP-1 ablation did not significantly alter total body weight for the C3(1)/SV40Tag/MCP-1−/− mice when compared with the MCP-1 sufficient mice at any point during the experiment (). Treatment groups reported final body weight measurements of 24.25 g ± 0.65 and 22.94 g ± 0.90 for C3(1)/SV40Tag and C3(1)/SV40Tag/MCP-1−/− groups, respectively. Tumor palpations began at 12 weeks of age and continued until 22 weeks of age, which allowed us to crudely measure tumor burden between groups without anesthesizing the animals. All experimental mice had tumors by 22 weeks of age. C3(1)/SV40Tag/MCP-1−/− mice showed a significant decrease in the development of primary tumor number compared with C3(1)/SV40Tag mice beginning at 16 weeks of age (0.25 ± 0.18 compared with 1.33 ± 0.35) that continued until the final week (age 22 weeks) of palpation measurements (1.67 ± 0.31 compared with 4.20 ± 0.33) (). Both experimental groups had similar trends in primary tumor volume growth throughout the experiment until week 22 when the C3(1)/SV40Tag mice had a significantly large increase in tumor volume growth (1033.05 ± 324.35mmCitation3) compared with the MCP-1 deficient mice (246.78 ± 119.34mmCitation3) () (P<0.05). Overall these data indicate that MCP-1 ablation delays tumor development in the C3(1)/SV40Tag mouse model. Although mice were housed 2–5/cage depending on availablilty from the colony, there is no indication that mouse number per cage within each group had any effect on tumorigenesis.
Figure 1. Body weight characteristics and tumor statistics. (A) Body weight in grams. (B) Total tumor number per mouse. (C) Total tumor volume per mouse. *P<0.05. Data are represented as ± SEM, C3(1)/SV40Tag n = 15, C3(1)/SV40Tag/MCP-1−/− n = 12.
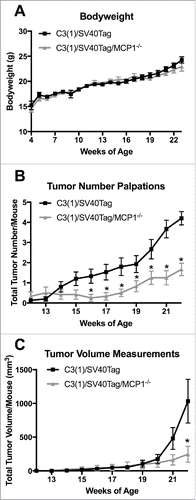
Loss of MCP-1 results in decreased primary tumor initiation and tumor volume
Measurements taken at sacrifice for total primary tumor volume and total primary tumor number for animals in each group indicated significant differences in tumorigenesis with MCP-1 manipulation in this model of TNBC. MCP-1 expression resulted in significantly increased primary mammary tumor multiplicity and growth compared with the MCP-1 deficient group; mean tumor number/mouse () and mean tumor volume/mouse () determined at necropsy were 15.86 ± 1.07 and 3126.62 ± 771.83 mm3 for C3(1)/SV40Tag, and 6.67 ± 1.46 and 935.78 ± 232.11 mm3 for C3(1)/SV40Tag/MCP-1−/− mice, respectively (P < 0.05).
Deletion of MCP-1 expression delays tumor progression in the early stages of tumorigenesis
There was no difference in the percentage of samples classified in each pathological stage between the groups at 23 weeks of age (). The early stage of tumorigenesis in this model is classified as atypical ductal hyperplasia (ADH, low grade MIN lesion), which is characterized as having more than one layer of disorganized and atypical epithelial cells with frequent mitotic figures, lining the mammary ducts. In the current investigation, 15.4% of C3(1)/SV40Tag and 10.0% of C3(1)/SV40Tag/MCP-1−/− mice classified into this early tumorigenic stage. The next progressive level is ductal carcinoma in situ (DCIS, high grade MIN lesion), classified as the filling and expansion of ducts with highly dysplastic epithelial cells confined still in the ducts without breaching the underlying basal lamina. We report that 30.8% and 30.0% of samples for C3(1)/SV40Tag and C3(1)/SV40Tag/MCP-1−/− mammary glands scored into this stage, respectively. The final stage is invasive adenocarcinoma which is described as poorly differentiated adenocarcinoma characterized by sheets of neoplastic cells occasionally forming glandular structures separated by fine fibrovascular stroma.Citation25 The majority of mice in the current study were classified into this advanced stage; 53.8% C3(1)/SV40Tag and 60% of C3(1)/SV40Tag/MCP-1−/− mice (). These findings imply that there were no differences in the pathology between the experimental groups at this stage of tumorigenesis. However, this may not be entirely surprising given the advanced stage of tumorigenesis at which these analyses were performed. Therefore, we performed an additional experiment in which the histopathology was examined at a much earlier stage of tumorigenesis (i.e., 12 weeks of age) (). By 12 weeks of age, this cancer model is characterized by progression into MIN/high grade.Citation19 Consistent with the tumor burden data, histopathology in the mammary gland of mice killed at 12 weeks of age clearly showed a delay in tumor progression for MCP-1 deficient mice compared with C3(1)/SV40Tag mice. We report that 50% of the C3(1)/SV40Tag animals progressed into high grade lesions (MIN, high grade), while 50% of samples were of low grade lesions (MIN, low grade); whereas, 100% of MCP-1 deficient mice remain classified into the early atypical ductal hyperplasia (ADH). Given the small sample size (n = 6), these comparisons did not reach statistical significance (P =.08 for MIN, low grade and P =.18 for MIN high grade); however, the data are strongly suggestive of a delay in tumor progression for MCP-1 deficient mice compared with C3(1)/SV40Tag mice. are representative images of tumorigenesis of each histopathological stage for this TNBC mouse model.
Figure 3. Mammary gland histopathology for each treatment group. (A) Graph of mammary gland pathology score for mice at 12 weeks and 23 weeks of age for both groups. (B) Representative images of atypical ductal hyperplasia (ADH, low grade MIN lesion) characterized by several layers of disarranged atypical epithelial cells with frequent mitotic figures. (C) Representative images of ductal carcinoma in situ (DCIS, high grade MIN lesion) characterized by filling and expansion of ducts with dysplastic epithelial cells without breach of the basement membrane or invasion of the surrounding adipose tissue. (D) Representative images of invasive adenocarcinoma characterized by pleomorphic neoplastic cells arranged in sheets or in tubules separated by fine fibrovascular stroma. shown at 10x magnification. Data are represented as percentage of total samples for each time point/group, 12 week time point: C3(1)/SV40Tag n = 6, C3(1)/SV40Tag/MCP-1−/− n = 6, 23 week time point: C3(1)/SV40Tag n = 15, C3(1)/SV40Tag/MCP-1−/− n = 12.
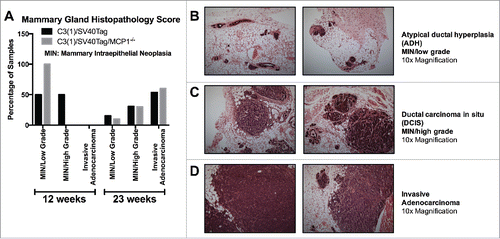
MCP-1 deficiency results in a decline of macrophage markers and localized inflammatory mediators in the mammary gland and tumor microenvironment
At 23 weeks of age, the mRNA expression of the macrophage marker, Mertk, was significantly downregulated in the mammary gland of MCP-1 deficient mice (P<0.05), while macrophage marker CD64 had a decreasing downtrend with P = 0.06 (). Pro-inflammatory cytokine IL-1β was significantly decreased (P<0.05) and pro-inflammatory cytokines IL-6 and TNFα indicated a downtrend in mRNA expression in the mammary gland with p = 0.09 and p = 0.06, respectively. Cytokines IL-12 and IL-10 had no change in mRNA expression in the mammary gland and, as expected, MCP-1 expression was not detected in the mammary gland of C3(1)/SV40Tag/MCP-1−/− mice, thus confirming their knockout genotype status ().
Figure 4. Macrophage markers and localized inflammation in the mammary gland and tumor. (A) Relative gene expression of macrophage markers in the mammary gland. (B) Relative gene expression of inflammatory mediators in the mammary gland. C3(1)/SV40Tag n = 10, C3(1)/SV40Tag/MCP-1−/− n = 7. (C) Relative gene expression of macrophage markers in the tumor. (D) Relative gene expression of inflammatory mediators in the tumor. *P≤0.05. Data are represented as ± SEM, C3(1)/SV40Tag n = 13, C3(1)/SV40Tag/MCP-1−/− n = 10.
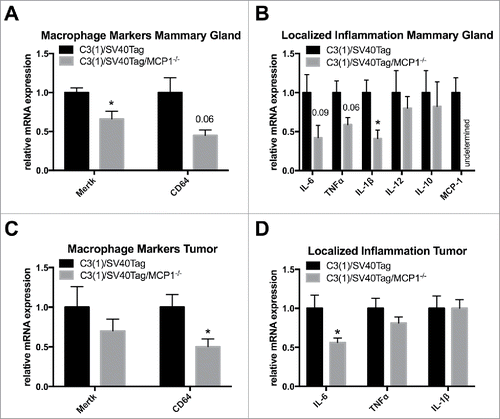
Deletion of MCP-1 expression resulted in a significant decrease in mRNA expression of macrophage marker CD64 in the tumor environment (P<0.05) but there was no significant change in Mertk expression (). MCP-1 ablation also significantly downregulated mRNA expression of the pro-inflammatory cytokine IL-6 in the tumor environment (P < 0.05); however, there was no change in TNFα and IL-1β expression in the tumor environment ().
Discussion
Studies indicate that MCP-1 regulates multiple mechanisms of breast cancer progression.Citation13 Experimental evidence has shown MCP-1 to be the major chemokine for macrophage recruitment into the tumor microenvironment,Citation26 a process that is known to drive tumorigenic events. However, the importance of this chemokine in the progression of tumorigenesis in a triple negative model of breast cancer has not been well established. Therefore, using a gene deletion approach in the C3(1)SV40Tag mouse, we developed a C3(1)SV40Tag/MCP-1−/− mouse to examine the effects of MCP-1 deficiency on TNBC that is closely associated with poor prognosis in human breast cancer. Results show that deficiency of MCP-1 reduces mammary tumorigenesis with a significant decrease in primary tumor volume and tumor number. Consistent with the primary tumor data, histopathology in the mammary gland of mice killed at an early timepoint, but well established stage for this breast cancer model, showed a delay in tumor progression for MCP-1 deficient mice. MCP-1 deficient mice showed a reduction in mRNA expression of macrophage markers (CD64 and Mertk) and inflammatory cytokines in the mammary gland that are known to play a role in breast cancer progression.Citation27,28 Additionally, tumor tissue gene expression of the macrophage marker CD64 and inflammatory cytokine IL-6 were also significantly reduced in the MCP-1 deficient mice. These data support a beneficial effect of MCP-1 deficiency on mammary tumorigenesis in the C3(1)/SV40Tag mouse model of TNBC that is associated with a reduction in macrophage markers and inflammatory mediators in the mammary gland and tumor enviroment.
Both rodent and clinical studies have associated MCP-1 expression level with tumorigenesis.Citation13,29,30 Our results are consistent with previous studies that have linked MCP-1 gene knockdown or ablation with a reduction in primary tumorigenesis in various cancer models. In a study previously conducted by our laboratory, MCP-1 deficiency resulted in a reduction of overall intestinal polyp number in a mouse model of intestinal tumorigenesis.Citation31 Similarly, Fridlander et al., demonstrated a beneficial effect of MCP-1 neutralization in a murine model of non-small cell lung cancer.Citation32 However, there have been relatively few mechanistic studies to link MCP-1 expression to breast cancer progression, and in particular TNBC. MCP-1 is expressed at relatively low levels in normal mammary epithelial tissue and circulating plasma, but increases dramatically with breast cancer progression.Citation13,33-35 Recently, MCP-1 has been shown to signal to breast cancer cells to regulate survival and invasion,Citation14 promote primary tumor growth,Citation15 and mediate breast cancer metastasis.Citation36 Interestingly, TNBC educated macrophages secrete higher amounts of MCP-1 than macrophages co-cultered with estrogen receptor positive breast cancer.Citation37 Most likely, cancer cell/macrophage crosstalk in TNBC initiates a vicious circle where both cell types reinforce each other.Citation38,39 MCP-1 also correlates with high tumor grade, increased metastasis and is associated with low levels of cell differentiation and poor prognosis in breast cancer.Citation40 In a study previously conducted by our laboratory, we used a pharmacological approach and examined the effects of an MCP-1 inhibitor on mammary tumorigenesis in this same C3(1)SV40Tag mouse model. The chemical MCP-1 inhibitor led to a significant reduction in tumor multiplicity but did not delay the initial palpable tumor nor slow the tumor growth as tumor volume and latency were similar between treatment groups.Citation41 A potential limitation of that study was the long-term treatment protocol used as the inhibitor was given in the food for a period of 16 weeks. Thus, it is possible that the animals developed a tolerance to the inhibitor treatment as a decrease in circulating levels of MCP-1 in plasma was not observed. This suggests that the overall effectiveness of the treatment dose, timing and administration method may not have been optimal in this animal model. Given the promising results of MCP-1 inhibition, but also considering the limitations of that study, we developed the MCP-1 global knockout C3(1)SV40Tag mouse to more conclusively examine the role of MCP-1 on TNBC. In the present investigation, we show for the first time that genetic knockdown of MCP-1 expression can lead to a significant reduction in mammary tumor volume and multiplicity in the C3(1)SV40Tag transgenic mouse model of TNBC.
Macrophages play a key role in tumorigenesis; they are a major player in the inflammatory response that contributes to cellular proliferation, transformation, promotion, invasion, angiogenesis, apoptosis and metastasis.Citation42,43 Given that MCP-1 has been implicated as the most important chemokine for macrophage recruitment into the tumor microenvironment, we next examined the effect of MCP-1 deficiency on select macrophage markers in the mammary tissue and tumor microenvironment. In the past several years, considerable progress has been made in distinguishing macrophages from dendritic cells, 2 immune cells of similar origin reflected by overlapping functions and molecular profiles. More recently, 2 mRNA transcripts that are unique to macrophages and not dendritic cells have been identified; Mertk, which is involved in the phagocytosis of apoptotic cells, and Fcgr1, which encodes the immunoglobulin receptor CD64 on macrophages.Citation44 Studies have implicated Mertk to not only be highly elevated in human breast carcinoma samples, but also mediate efferocytosis in macrophages and advance tumor progression through the inhibition of immune checkpoints.Citation45 In this experiment, MCP-1 depletion resulted in a reduction in both Mertk and CD64 mRNA expression in the mammary gland, and also CD64 mRNA expression in the tumor tissue. These results are consistent with a very recent study in which Fang et al., demonstrated a reduction in the recruitment of macrophages to the tumor microenvironment with targeted MCP-1 gene silencing in a model of TNBC cancer.Citation15 Similarly, previous work by our group has shown equivalent results using pharmacological inhibition of MCP-1 in TNBC.Citation41 These findings should be substantiated through the use of cell sorting and flow cytometry to solidly establish the role of MCP-1 depletion on macrophage infiltration and specific macrophage phenotypes that may be associated with TNBC.
Studies have established that the mutual interaction of macrophages with cancer cells enhances survival, invasion, promotes primary tumor growth and increases the production of inflammatory cytokines to transform the tumor microenvironment so that it favors tumorigenesis.Citation14,15 The inflammatory cytokines TNFα, IL-1β, and IL-6 have been associated with poor prognosis and increasing risks of metastasis in breast cancer in addition to being related to tumor macrophage expression of MCP-1.Citation13,28 We investigated the expression of these inflammatory cytokines in the surrounding dysplastic mammary tissue and in the tumor environment. Our findings indicate that the absence of MCP-1 expression significantly corresponds with decreases in mRNA expression of TNFα, IL-1β, and IL-6 in the mammary gland and decreases in IL-6 expression in the tumor. These data are supported by Ueno et al, that demonstrated MCP-1 expression levels are associated with IL-6 expression levels in human breast cancer tissues.Citation13 There are consistent data showing increased levels of IL-6 in breast cancer patients when compared with healthy controls and increased levels of IL-6 have also been correlated with clinical tumor stage, lymph node infiltration and recurrent disease.Citation46 IL-6 levels have also been associated with poor prognosis for time-to-progression and death in advanced stage patients.Citation47
In summary, we report for the first time that ablation of MCP-1 expression through genetic deletion delays mammary tumorigenesis and downregulates localized inflammation in the C3(1)/SV40Tag model of TNBC. Although, MCP-1 expression has been connected with regulating multiple mechanisms of breast cancer progression, its involvement in tumorigenesis in a TNBC model has not been fully established. MCP-1 provides a direct link between inflammation and macrophage recruitment and not surprisingly, therefore has an emergent role in the etiopathogenesis of breast cancer. Given the potential clinical implications, more research is needed to fully elucidate the role of this chemokine in breast cancer progression so that targeted therapies can be developed.
Disclosure of potential conflicts of interest
No potential conflicts of interest were disclosed.
Funding
This work was supported by grants from the National Institutes of Health (F31CA183458 to T.L.C. and R21CA167058, R21CA175636, R21CA191966 and K01AT007824 to E.A.M.).
References
- Group, U.S Cancer Statistics Working. United States Cancer Statistics: 1999–2012 Incidence and Mortality Web-based Report 2015, Department of Health and Human Services, Centers for Disease Control and Prevention, and National Cancer Institute; http://dx.doi.org/10.1517/14656566.2015.1102226
- Society, American Cancer. Learn about cancer: Breast Cancer. What are the key statistics about breast cancer? 2016 05/04/2016 [cited 2016 06/30]; Available from: http://www.cancer.org/cancer/breastcancer/detailedguide/breast-cancer-key-statistics
- Sorlie T, Tibshirani R, Parker J, Hastie T, Marron JS, Nobel A, Deng S, Johnsen H, Pesich R, Geisler S, et al. Repeated observation of breast tumor subtypes in independent gene expression data sets. Proc Natl Acad Sci U S A 2003; 100(14):8418-23; PMID:12829800; http://dx.doi.org/10.1200/JCO.2014.58.022510.1073/pnas.0932692100
- Haffty BG, Yang Q, Reiss M, Kearney T, Higgins SA, Weidhaas J, Harris L, Hait W, Toppmeyer D. Locoregional relapse and distant metastasis in conservatively managed triple negative early-stage breast cancer. J Clin Oncol 2006; 24(36):5652-7; PMID:17116942; http://dx.doi.org/10.1158/0008-5472.CAN-11-277810.1200/JCO.2006.06.5664
- Dolle JM, Daling JR, White E, Brinton LA, Doody DR, PorterL, Malone KE. Risk factors for triple-negative breast cancer in women under the age of 45 years. Cancer Epidemiol Biomarkers Prev 2009; 18(4):1157-66; PMID:19336554; http://dx.doi.org/10.18632/oncotarget.250510.1158/1055-9965.EPI-08-1005
- Dent R, Trudeau M, Pritchard KI, Hanna WM, Kahn HK, Sawka CA, Lickley LA, Rawlinson E, Sun P, Narod SA. Triple-negative breast cancer: clinical features and patterns of recurrence. Clin Cancer Res 2007; 13(15 Pt 1):4429-34; PMID:17671126; http://dx.doi.org/10.1158/2326-6066.CIR-13-022410.1158/1078-0432.CCR-06-3045
- Harris LN, Broadwater G, Lin NU, Miron A, Schnitt SJ, Cowan D, Lara J, Bleiweiss I, Berry D, Ellis M, et al. Molecular subtypes of breast cancer in relation to paclitaxel response and outcomes in women with metastatic disease: results from CALGB 9342. Breast Cancer Res 2006; 8(6):R66; PMID:17129383; http://dx.doi.org/10.1186/bcr1622
- Badve S, Dabbs DJ, Schnitt SJ, Baehner FL, Decker T, Eusebi V, Fox SB, Ichihara S, Jacquemier J, Lakhani SR, et al. Basal-like and triple-negative breast cancers: a critical review with an emphasis on the implications for pathologists and oncologists. Mod Pathol 2011; 24(2):157-67; PMID:21076464; http://dx.doi.org/10.1186/s40425-015-0067-z10.1038/modpathol.2010.200
- Tischkowitz M, Brunet JS, Begin LR, Huntsman DG, Cheang MC, Akslen LA, Nielsen TO, Foulkes WD. Use of immunohistochemical markers can refine prognosis i triple negative breast cancer. BMC Cancer 2007; 7:134; PMID:17650314; http://dx.doi.org/10.1016/S1471-4906(03)00132-710.1186/1471-2407-7-134
- Rodler E, Korde L, Gralow J. Current treatment options in triple negative breast cancer. Breast Dis 2010; 32(1-2):99-122; PMID:21778572; http://dx.doi.org/10.1084/jem.2004202810.3233/BD-2010-0304
- Deshmane SL, Kremlev S, Amini S, Sawaya BE. Monocyte chemoattractant protein-1 (MCP-1): an overview. J Interferon Cytokine Res 2009; 29(6):313-26; PMID:19441883; http://dx.doi.org/10.1038/onc.2016.3710.1089/jir.2008.0027
- Ohta M, Kitadai Y, Tanaka S, Yoshihara M, Yasui W, Mukaida N, Haruma K, Chayama K. Monocyte chemoattractant protein-1 expression correlates with macrophage infiltration and tumor vascularity in human gastric carcinomas. Int J Oncol 2003; 22(4):773-8; PMID:12632067; http://dx.doi.org/10.1016/j.cell.2015.08.016
- Ueno T, Toi M, Saji H, Muta M, Bando H, Kuroi K, Koike M, Inadera H, Matsushima K. Significance of macrophage chemoattractant protein-1 in macrophage recruitment, angiogenesis, and survival in human breast cancer. Clin Cancer Res 2000; 6(8):3282-9; PMID:10955814; http://dx.doi.org/10.1182/blood-2006-06-031856
- Fang WB, Jokar I, Zou A, Lambert D, Dendukuri P, Cheng N. CCL2/CCR2 chemokine signaling coordinates survival and motility of breast cancer cells through Smad3 protein- and p42/44 mitogen-activated protein kinase (MAPK)-dependent mechanisms. J Biol Chem 2012; 287(43):36593-608; PMID:22927430; http://dx.doi.org/10.1074/jbc.M11067520010.1074/jbc.M112.365999
- Fang WB, Yao M, Brummer G, Acevedo D, Alhakamy N, Berkland C, Cheng N. Targeted gene silencing of CCL2 inhibits triple negative breast cancer progression by blocking cancer stem cell renewal and M2 macrophage recruitment. Oncotarget 2016; 7(31): 49349-67; PMID:27283985; http://dx.doi.org/10.18632/oncotarget.9885
- Bennett CN, Green JE. Genomic analyses as a guide to target identification and preclinical testing of mouse models of breast cancer. Toxicol Pathol 2010; 38(1):88-95; PMID:20080934; http://dx.doi.org/10.1016/j.intimp.2016.03.00210.1177/0192623309357074
- Maroulakou IG, Anver M, Garrett L, Green JE. Prostate and mammary adenocarcinoma in transgenic mice carrying a rat C3(1) simian virus 40 large tumor antigen fusion gene. Proc Natl Acad Sci U S A 1994; 91(23):11236-40; PMID:7972041; http://dx.doi.org/10.1038/nrclinonc.2016.3610.1073/pnas.91.23.11236
- Cranford TL, Enos RT, Velazquez KT, McClellan JL, Davis JM, Singh UP, Nagarkatti M, NagarkattiS, Robinson CM, Murphy EA. Role of MCP-1 on inflammatory processes and metabolic dysfunction following high-fat feedings in the FVB/N strain. Int J Obes (Lond) 2016; 40(5):844-51; PMID:26620890; http://dx.doi.org/10.1158/1078-0432.CCR-14-270810.1038/ijo.2015.244
- Green JE, Shibata MA, Yoshidome K, Liu ML, Jorcyk C, Anver MR, Wigginton J, Wiltrout R, Shibata E, Kaczmarczyk S, et al. The C3(1)/SV40 T-antigen transgenic mouse model of mammary cancer: ductal epithelial cell targeting with multistage progression to carcinoma. Oncogene 2000; 19(8):1020-7; PMID:10713685; http://dx.doi.org/10.2217/imt-2016-004810.1038/sj.onc.1203280
- Steiner J, Davis J, McClellan J, Enos R, Carson J, Fayad R, Nagarkatti M, Nagarkatti P, Altomare D, Creek K, et al. Dose-dependent benefits of quercetin on tumorigenesis in the C3(1)/SV40Tag transgenic mouse model of breast cancer. Cancer Biol Ther 2014; 15(11):1456-67; PMID:25482952; http://dx.doi.org/10.1016/j.cell.2015.08.01210.4161/15384047.2014.955444
- Enos RT, Davis JM, Velazquez KT, McClellan JL, Day SD, Carnevale KA, Murphy EA. Influence of dietary saturated fat content on adiposity, macrophage behavior, inflammation, and metabolism: composition matters. J Lipid Res 2013; 54(1):152-63; PMID:23103474; http://dx.doi.org/10.18632/oncotarget.368210.1194/jlr.M030700
- Warden CH, Fisler JS. Comparisons of diets used in animal models of high-fat feeding. Cell Metab 2008; 7(4):277; PMID:18396128; http://dx.doi.org/10.1126/scitranslmed.aad711810.1016/j.cmet.2008.03.014
- Velazquez KT, Enos RT, McClellan JL, Cranford TL, Chatzistamou I, Singh UP, Nagarkatti M, NagarkattiS, Fan D, Murphy EA. MicroRNA-155 deletion promotes tumorigenesis in the azoxymethane-dextran sulfate sodium model of colon cancer. Am J Physiol Gastrointest Liver Physiol 2016; 310(6):G347-58; PMID:26744471; http://dx.doi.org/10.1172/JCI8001110.1152/ajpgi.00326.2015
- Enos RT, Velazquez KT, McClellan JL, Cranford TL, Walla MD, Murphy EA. Lowering the dietary omega-6: omega-3 does not hinder nonalcoholic fatty-liver disease development in a murine model. Nutr Res 2015; 35(5):449-59; PMID:25934114; http://dx.doi.org/10.1016/j.nutres.2015.04.003
- Hoenerhoff MJ, Shibata MA, Bode A, Green JE. Pathologic progression of mammary carcinomas in a C3(1)/SV40 T/t-antigen transgenic rat model of human triple-negative and Her2-positive breast cancer. Transgenic Res 2011; 20(2):247-59; PMID:20549348; http://dx.doi.org/10.1007/s11248-010-9406-5
- Balkwill FR, Mantovani A. Cancer-related inflammation: common themes and therapeutic opportunities. Semin Cancer Biol 2012; 22(1):33-40; PMID:22210179; http://dx.doi.org/10.1016/j.semcancer.2011.12.005
- Rose DP, GracheckJ, Vona-Davis L. The Interactions of Obesity, Inflammation and Insulin Resistance in Breast Cancer. Cancers (Basel) 2015; 7(4):2147-68; PMID:26516917; http://dx.doi.org/10.3390/cancers7040883
- Allavena P, Sica A, Solinas G, Porta C, Mantovani A. The inflammatory micro-environment in tumor progression: the role of tumor-associated macrophages. Crit Rev Oncol Hematol 2008; 66(1):1-9; PMID:17913510; http://dx.doi.org/10.1016/j.critrevonc.2007.07.004
- Balkwill F. Cancer and the chemokine network. Nat Rev Cancer 2004; 4(7):540-50; PMID:15229479; http://dx.doi.org/10.1038/nrc1388
- Bailey C, Negus R, Morris A, Ziprin P, Goldin R, Allavena P, Peck D, Darzi A. Chemokine expression is associated with the accumulation of tumour associated macrophages (TAMs) and progression in human colorectal cancer. Clin Exp Metastasis 2007; 24(2):121-30; PMID:17390111; http://dx.doi.org/10.1007/s10585-007-9060-3
- McClellan JL, Davis JM, Steiner JL, Enos RT, Jung SH, Carson JA, Pena MM, Carnevale KA, Berger FG, Murphy EA. Linking tumor-associated macrophages, inflammation, and intestinal tumorigenesis: role of MCP-1. Am J Physiol Gastrointest Liver Physiol 2012; 303(10):G1087-95; PMID:23019193; http://dx.doi.org/10.1152/ajpgi.00252.2012
- Fridlender ZG, Buchlis G, Kapoor V, Cheng G, Sun J, Singhal S, Crisanti MC, Wang LC, Heitjan D, Snyder LA, et al. CCL2 blockade augments cancer immunotherapy. Cancer Res 2010; 70(1):109-18; PMID:20028856; http://dx.doi.org/10.1158/0008-5472.CAN-09-2326
- Valkovic T, Lucin K, Krstulja M, Dobi-Babic R, Jonjic N. Expression of monocyte chemotactic protein-1 in human invasive ductal breast cancer. Pathol Res Pract 1998; 194(5):335-40; PMID:9651946; http://dx.doi.org/10.1016/S0344-0338(98)80057-5
- Soria G, Yaal-Hahoshen N, Azenshtein E, Shina S, Leider-Trejo L, Ryvo L, Cohen-Hillel E, Shtabsky A, Ehrlich M, Meshel T, et al. Concomitant expression of the chemokines RANTES and MCP-1 in human breast cancer: a basis for tumor-promoting interactions. Cytokine 2008; 44(1):191-200; PMID:18790652; http://dx.doi.org/10.1016/j.cyto.2008.08.002
- Yao M, Yu E, Staggs V, Fan F, Cheng N. Elevated expression of chemokine C-C ligand 2 in stroma is associated with recurrent basal-like breast cancers. Mod Pathol 2016; 29(8):810-23; PMID:27125354; http://dx.doi.org/10.1038/modpathol.2016.78
- Qian BZ, Li J, Zhang H, Kitamura T, Zhang J, Campion LR, Kaiser EA, Snyder LA, Pollard JW. CCL2 recruits inflammatory monocytes to facilitate breast-tumour metastasis. Nature 2011; 475(7355):222-5; PMID:21654748; http://dx.doi.org/10.1038/nature10138
- Hollmen M, Roudnicky F, Karaman S, Detmar M. Characterization of macrophage–cancer cell crosstalk in estrogen receptor positive and triple-negative breast cancer. Sci Rep 2015; 5:9188; PMID:25776849; http://dx.doi.org/10.1038/srep09188
- Su S, Liu Q, Chen J, Chen J, Chen F, He C, Huang D, Wu W, Lin L, Huang W, et al. A positive feedback loop between mesenchymal-like cancer cells and macrophages is essential to breast cancer metastasis. Cancer Cell 2014; 25(5):605-20; PMID:24823638; http://dx.doi.org/10.1016/j.ccr.2014.03.021
- Patsialou A, Wyckoff J, Wang Y, Goswami S, Stanley ER, Condeelis JS. Invasion of human breast cancer cells in vivo requires both paracrine and autocrine loops involving the colony-stimulating factor-1 receptor. Cancer Res 2009; 69(24):9498-506; PMID:19934330; http://dx.doi.org/10.1158/0008-5472.CAN-09-1868
- Soria G, Ben-Baruch A. The inflammatory chemokines CCL2 and CCL5 in breast cancer. Cancer Lett 2008; 267(2):271-85; PMID:18439751; http://dx.doi.org/10.1016/j.canlet.2008.03.018
- Steiner JL, Davis JM, McClellan JL, Guglielmotti A, Murphy EA. Effects of the MCP-1 synthesis inhibitor bindarit on tumorigenesis and inflammatory markers in the C3(1)/SV40Tag mouse model of breast cancer. Cytokine 2014; 66(1):60-8; PMID:24548426; http://dx.doi.org/10.1016/j.cyto.2013.12.011
- Mantovani A, Allavena P, Sica A, Balkwill F. Cancer-related inflammation. Nature 2008; 454(7203):436-44; PMID:18650914; http://dx.doi.org/10.1038/nature07205
- Solinas G, Germano G, Mantovani A, Allavena P. Tumor-associated macrophages (TAM) as major players of the cancer-related inflammation. J Leukoc Biol 2009; 86(5):1065-73; PMID:19741157; http://dx.doi.org/10.1189/jlb.0609385
- Gautier EL, Shay T, Miller J, Greter M, Jakubzick C, Ivanov S, Helft J, Chow A, Elpek KG, Gordonov S, et al. Gene-expression profiles and transcriptional regulatory pathways that underlie the identity and diversity of mouse tissue macrophages. Nat Immunol 2012; 13(11):1118-28; PMID:23023392; http://dx.doi.org/10.1038/ni.2419
- Nguyen KQ, Tsou WI, Calarese DA, Kimani SG, Singh S, Hsieh S, Liu Y, Lu B, Wu Y, Garforth SJ, et al. Overexpression of MERTK receptor tyrosine kinase in epithelial cancer cells drives efferocytosis in a gain-of-function capacity. J Biol Chem 2014; 289(37):25737-49; PMID:25074939; http://dx.doi.org/10.1074/jbc.M114.570838
- Knupfer H, Preiss R. Significance of interleukin-6 (IL-6) in breast cancer (review). Breast Cancer Res Treat 2007; 102(2):129-35; PMID:16927176; http://dx.doi.org/10.1007/s10549-006-9328-3
- Salgado R, Junius S, Benoy I, Van Dam P, Vermeulen P, Van Marck E, Huget P, Dirix LY. Circulating interleukin-6 predicts survival in patients with metastatic breast cancer. Int J Cancer 2003; 103(5):642-6; PMID:12494472; http://dx.doi.org/10.1002/ijc.10833