ABSTRACT
L-arginine supplementation was recently proved to promote the function of immune cells, especially T-cells, by facilitating T-cell proliferation, differentiation and survival in vivo. Cytotoxic CD8+ plays a crucial role in modulating anti-cancer response mediated by the immune system, but was restricted by exhaustion. Thus, we hypothesized that L-arginine, in combination with α-PD-L1 antibody, may provide a favored environment for T-cell response against osteosarcoma. Immunocompetent BALB/c mouse models bearing orthotopic and metastatic osteosarcoma were established to validate this conjecture. We found that L-arginine significantly elevated the number of splenic CD8+ T-cells, the level of serum interferon-γ, and CD8+ T-cell infiltration. Furthermore, α-PD-L1 antibody protected these amplified CD8+ T-cells from exhaustion, and therefore strengthened the secretion of interferon-γ, granzyme B and perforin by these T-cells. As a result, this combination treatment strategy significantly prolonged survival of osteosarcoma bearing mice, suggesting that L-arginine supplementation in combination with α-PD-L1 antibody may be a promising method for osteosarcoma patients.
Introduction
Immunotherapy is a promising therapeutic strategy against osteosarcoma, a highly aggressive malignant tumor characteristic of forming early lung metastasis and bad prognosis. Conventional therapeutic strategy consists surgical resection, chemotherapy, with or without radiotherapy.Citation1 However, once the tumor relapse or behave as a chemo-resistant phenotype, conventional none-surgical therapy can hardly make efforts in efficiently prolonging the survival. Thus, there is an urgent need to establish an alternative regimen, such as immunotherapy, for osteosarcoma.Citation2
CD8+ T-cell is one of the most effective killer cells in mediating antitumor immune response. Efforts have been made to utilize CD8+ T-cells in adoptive cell transfer immunotherapy against osteosarcoma.Citation3-5 However, there are still some suffocating restrains in CD8+ T-cell-based immunotherapy, such as immune checkpoint, and the tumor immunosuppressive microenvironment. Pathology study has revealed that most of osteosarcoma cells express PD-L1,Citation6 which can dramatically suppress the effects of CD8+ cytotoxic cells (CTLs). Besides, tumors can develop an immunosuppressive environment via different ways, and consequently significantly decrease the amount of tumor infiltrating lymphocytes (TILs).Citation7 These 2 factors in combination can result in depressed CTL homing and impaired CTL function, and finally abrogate the spontaneous immune attack against osteosarcoma.
Recently, antibodies for PD-1/PD-L1 pathway displayed inspiring effects in strengthening the activity of CD8+ T-cells in clinical trials for quite a few kinds of solid malignancies. Considering the expression of PD-L1 on osteosarcoma cells, it is reasonable to evaluate the effect of α-PD-L1 antibody in osteosarcoma.Citation8 Concerning the immunosuppressive environment, L-arginine and arginase (type I and II) were discovered to play critical roles.Citation9,10 L-arginine is an important amino acid for human beings. However, L-arginine is usually scarce in the tumor environment as the tumor cells may over-express arginase,Citation11,12 which leads to T-cell dysfunction and apoptosis. Besides, degradation of L-arginine results in reduced expression of CD3, and consequently impairs T-cell responsiveness.Citation13,14 Thus, following these theories, L-arginine supplementation was recently proved to enhance T-cell survival and antitumor activity.Citation15,16
In this study, we tried to validate our hypothesis that L-arginine supplementation and PD-1/PD-L1 pathway blockade can facilitate spontaneous immune response against osteosarcoma. We established immunocompetent mouse models using BALB/c mice bearing in situ osteosarcoma or metastasized osteosarcoma, and then treated them with L-arginine and/or α-PD-L1 antibody. We discovered that L-arginine supplementation, in combination with α-PD-L1 antibody, displayed encouraging effects in promoting immune response and significantly prolonged the survival.
Results
Combination therapy significantly improved survival in osteosarcoma tumor bearing
We monitored the survival of mice underwent different treatment regimens in both in situ and metastasis models. L-arginine supplementation alone didn't show statistically significant survival benefit for osteosarcoma bearing mice (). α-PD-L1 antibody, however, significantly prolonged mice survival. For mice receiving PBS, the medium survival of in situ models and metastasis models were 56.5 d and 23.5 days, respectively; while in α-PD-L1 antibody treatment alone groups, the medium survival were 67.5 d (P = 0.0004) and 31.5 d (P = 0.011), respectively. Moreover, combination treatment revealed even stronger benefit. The medium survival in combo group were 79.5 d (P < 0.0001) and 39 d (P < 0.0001), respectively. Although L-arginine supplementation alone didn't result in prolonged survival, the combination treatment with L-arginine and α-PD-L1 antibody displayed much more powerful antitumor abilities than α-PD-L1 antibody treatment alone.
Figure 1. Combination treatment with L-arginine and α-PD-L1 antibody significantly increased survival in mice bearing in situ and metastatic osteosarcoma. (A) The survival curves of mouse bearing in situ osteosarcoma were monitored. (B) The survival curves of mice with metastatic osteosarcoma. n = 10/group. **P < 0.01, ***P < 0.001, and **** P < 0.0001.
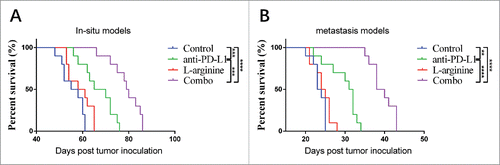
L-arginine supplementation increased the number and activity of CD8+ T-cells
We analyzed the phenotype of splenocytes and lymphocytes in tumor-draining lymph nodes (TDLNs) from different groups in in situ models. In splenic lymphocytes, L-arginine supplementation treatment significantly increased the proportion of CD8+ T-cells (). Although these splenic CD8+ T-cells are usually regarded as rest cells, the elevated number of CD8+ T-cells indicated that L-arginine helped in CD8+ T-cell survival. In addition, more mature dendritic cells (DCs) expressing CD11c and CD86 were found in L-arginine supplementation mice (). In TDLNs, elevated number of CD8+ T-cells were discovered in L-arginine treated mice (L-arginine alone and combo group) (), and these CD8+ T-cells displayed higher level of IFN-γ (), Granzyme B (), and perforin () than that in control or α-PD-L1 antibody treatment alone groups, indicating that L-arginine not only increased the number of CTLs in the TDLN, but also improved the activity of them. Moreover, serum IFN-γ level was evaluated by ELISA. Unsurprisingly, L-arginine treatment elevated serum IFN-γ level (Fig. S1A, B) in both in situ and metastasis models, indicating that L-arginine significantly strengthened immune response.
Figure 2. Combination treatment with L-arginine and α-PD-L1 antibody increased the proportion of CD8+ T-cells and CD86+ CD11c+ dendritic cells in the spleen of mice bearing in situ osteosarcoma. (A) Representative data of proportions of CD8+ T-cells. (B) Pooled data of proportions of CD8+ T-cells in the spleen in different groups. n = 5/group. (C) Representative data of proportions of mature dendritic cells. (D) Pooled data of proportions of mature dendritic cells in the spleen in different groups. n = 5/group. *** P < 0.001, and **** P < 0.0001. Data were presented as mean ± SEM.
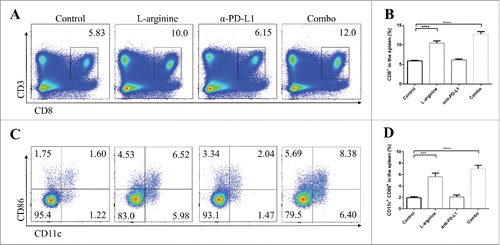
Figure 3. Combined treatment significantly elevated the number and activity of CD8+ T-cells in tumor-draining lymph nodes. (A) Representative data of proportions of CD8+ T-cells in TDLNs. (B) Pooled data of proportions of CD8+ T-cells in TDLNs in different groups. Representative data of proportions of IFN-γ+ (C), granzyme-B+ (E), and perforin+ (G) cells in CD8+ cells from TDLNs. Pooled data of frequencies of IFN-γ+ (D), granzyme-B+ (F), and perforin+ (H) cells in CD8+ cells in TDLNs from different groups. n = 5/group. * P < 0.05, ** P < 0.01, *** P < 0.001, and **** P < 0.0001. Data were presented as mean ± SEM.
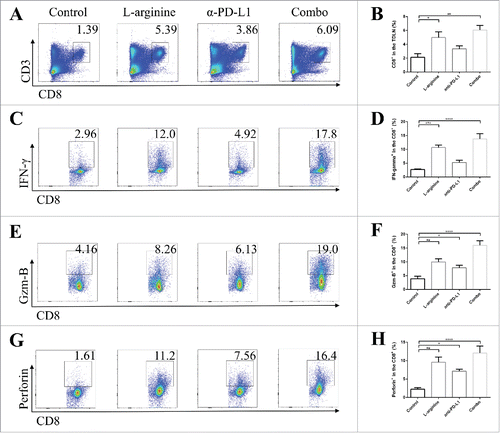
L-arginine supplementation elevated the number of TILs but severely restricted to T-cell exhaustion
In both in situ models and metastasis models, flow cytometry analyses showed significantly elevated number of TILs in L-arginine treated mice when compared with vehicle control mice. L-arginine supplementation alone achieved a 3-fold (in situ model) () or over 2-fold (metastasis model) () increase of intratumoral CD8+ T-cells. However, most of these intratumoral CD8+ T-cells were PD-1 positive (), while very few of them expressed IFN-γ (), which indicated that although more CD8+ CTLs were successfully homing to the tumor site after L-arginine supplementation, these cells failed to present their antitumor ability. Although L-arginine treatment seemed to have a trend in elevating the proportion of PD-1+ cells in CD8+ T-cells (; ), there was no statistical significance when compared with that in control mice. The exhausted phenotype of CD8+ TILs may account for the modest antitumor effect of L-arginine supplementation alone as was shown in .
Figure 4. Combined treatment significantly elevated the number and activity of CD8+ T-cells in orthotopic tumors. (A) Representative data of proportions of CD8+ T-cells in tumor. (B) Pooled data of proportions of CD8+ T-cells in tumor in different groups (calculated from CD8+/total events). Representative data of proportions of PD-1+ (C) and IFN-γ+ (E) cells in intratumoral CD8+ cells. Pooled data of frequencies of PD-1+ (D) and IFN-γ+ (F) cells in intratumoral CD8+ cells from different groups. n = 5/group. * P < 0.05, ** P < 0.01, *** P < 0.001, and **** P < 0.0001. Data were presented as mean ± SEM.
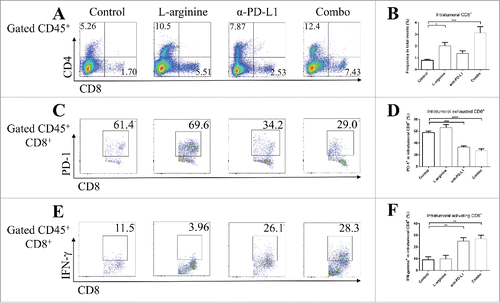
Figure 5. Combined treatment promoted immune response in pulmonary metastasized osteosarcoma. (A) Representative data of frequencies of CD8+ T-cells in metastasized osteosarcoma. (B) Pooled data of proportions of CD8+ T-cells in tumor in different groups (calculated from CD8+/total events). Representative data of proportions of PD-1+ (C) and IFN-γ+ (E) cells in pulmonary intratumoral CD8+ cells. Pooled data of frequencies of PD-1+ (D) and IFN-γ+ (F) cells in intratumoral CD8+ cells from different groups. n = 5/group. * P < 0.05, ** P < 0.01, *** P < 0.001, and **** P < 0.0001. Data were presented as mean ± SEM.
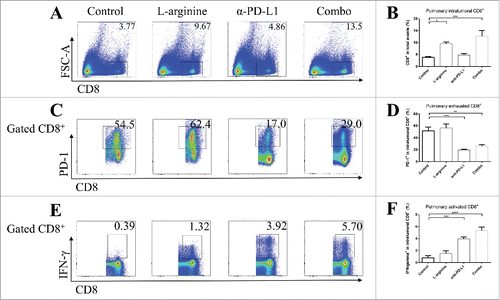
α-PD-L1 antibody treatment strengthened intratumoral T-cell response
We tried to validate the role α-PD-L1 antibody played in both models. Although α-PD-L1 antibody treatment alone didn't show significant benefit for peripheral CD8+ T-cells, it made bona fide effects in CTLs in TDLNs; elevated secretion of granzyme-B () and IFN-γ () by CD8+ T-cells were found in α-PD-L1 antibody treated mice. In tumor tissues from both models, albeit no statistically significant elevation in the number of intratumoral CD8+ T-cells, α-PD-L1 antibody seemed to down-regulate the expression of PD-1 on CD8+ T-cells ( and ) and increased the level of IFN-γ by CD8+ T-cells ( and ) than control mice.
In mice receiving combination treatment with L-arginine and α-PD-L1 antibody, the immune response was profoundly triggered. As mentioned above, L-arginine helped in CD8+ T-cell survival and activation, and α-PD-L1 antibody protected the intratumoral CTLs from exhaustion. Dramatically increased secretion of IFN-γ by intratumoral CD8+ T-cells was discovered in combo group, much higher than in L-arginine alone group ( and ). Furthermore, the proportion of exhausted cells (PD-1 positive) in intratumoral CD8+ T-cells was much lower than L-arginine alone group ( and ). Combination treatment increased CTL infiltration, facilitated anticancer immune response and protected effective cells from exhaustion.
Combination treatment inhibited the proliferation of myeloid-derived suppressive cells
Myeloid-derived suppressive cells (MDSCs) are powerful immune suppressive roles in the tumor microenvironment. We analyzed intratumoral MDSCs in both in situ models and metastasis models. L-arginine treatment alone had a trend in inhibiting the proliferation of MDSC, but α-PD-L1 antibody and combination treatment reduced the frequencies of MDSC within the tumor () in orthotopic models. Study on MDSCs in metastasis models didn't reveal changes in population. We also analyzed MDSCs in the spleen and TDLNs in both models, but no statistical difference was found (data not shown).
Figure 6. L-arginine plus α-PD-L1 antibody inhibited MDSC proliferation in orthotopic osteosarcoma models. (A) Representative data of frequencies of CD11b+ Gr-1+ MDSCs in intratumoral CD45+ cells. (B) Pooled data of proportions of MDSCs in tumor in different groups (calculated from CD11b+ Gr-1+/CD45+). n = 5/group. * P < 0.05 and ** P < 0.01. Data were presented as mean ± SEM.
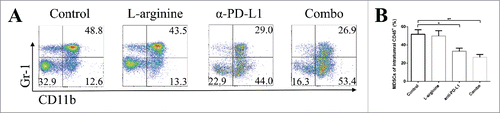
Discussion
CD8+ cytotoxic T-cells play crucial roles in cellular immune response against tumor. Numerous studies are focusing using CD8+ T-cells for cancer immunotherapy, including osteosarcoma.Citation17-19 However, the underlying mechanisms affecting T-cell activation, differentiation, function and survival are still largely unknown. Any method which can facilitate T-cell activity will undoubtedly help in cancer immunotherapy.
L-arginine, regarded as a nutritional agent in the past, was recently identified to be an important immune stimulator.Citation15 T-cells activation consumes large amount of L-arginine, and the underlying mechanism was nicely demonstrated.Citation15 Given the fact that arginase-mediated L-arginine depletion profoundly suppress T-cell immune response,Citation20,21 L-arginine supplementation is a good idea to feed the T-cells, and to inhibit tumor growth. However, malignancies, especially solid tumors, can escape from or impair immune attack by multiple mechanisms. PD-1/PD-L1 pathway was one of the most important role in inhibiting immune response.Citation22,23 Without controlling the immune checkpoint, the effect of T-cell-mediated anticancer response will be severely weakened.
In the current study, we evaluated the combination treatment with L-arginine and α-PD-L1 antibody for osteosarcoma. We demonstrated that mice receiving L-arginine treatment showed significantly strengthened T-cell activity. Elevated levels of IFN-γ, Granzyme B, and perforin secretion were detected. However, although assays on peripheral blood and lymphocytes revealed promising results, single-agent treatment with L-arginine still made modest effects in prolonging mouse survival. In tumor tissues, the strengthened T-cells were severely restricted by immune checkpoint, which may be correlated with the expression of PD-L1 on osteosarcoma cells. The administration of α-PD-L1 antibody protected the intratumoral T-cells from exhaustion, while the number of T-cells were still limited. Thus, the combination treatment with both L-arginine, the “fertilizer,” and α-PD-L1 antibody, the “protector,” successfully promote immune response and prolonged mice survival. Cytotoxic CD8+ T-cells, no matter in the TDLNs or in tumor tissues, secreted significantly higher levels of IFN-γ, Granzyme B, and perforin, and consequently control tumor promotion. Moreover, immune suppressor roles, MDSCs, were also inhibited after L-arginine treatment. In addition, we observed decreased expression of PD-1 on intratumoral CD8+ T-cells, which was not seen in single-agent treated mice. We conjectured that it might be associated with decreased number of intratumoral MDSCs. Although L-arginine supplementation showed a trend in strengthening PD-1 expression on CD8+ T-cells, there was no statistical significance. Also, we didn't find any evidence that L-arginine had the possibility in promoting T-cell exhaustion.
Clinical trials for osteosarcoma using different kinds of immunotherapies such as adoptive cell transfer and PD-1/PD-L1 antibodies are ongoing.Citation3 However, the response rates, no matter using HER-2 specific chimeric antigen receptor T-cells or immune checkpoint inhibitors, are still far from that in leukemias. Solid tumors escape from immune response via multiple ways, including immune suppressive microenvironment and overexpressed immune checkpoint ligands/receptors. L-arginine, a nutritional agent, can be used in combination with other therapies, and theoretically reach at least an additive, or even synergistic effects. The authors can expect that cocktail immunotherapy, combing adoptive cell transfer, adjuvants such as L-arginine, and checkpoint inhibitors, may achieve most powerful anticancer effects.
In conclusion, this is the first study demonstrating that L-arginine supplementation in combination with α-PD-L1 antibody can suppress osteosarcoma growth in vivo and prolong survival. Combination treatment stimulated immune response by triggering CD8+ T-cell activity and suppressing MDSCs. Our results suggest that L-arginine and α-PD-L1 antibody may be a promising therapeutic strategy for treatment of osteosarcoma patients.
Materials and methods
Animals, cell line, and reagents
Female BALB/c mice age 4–5 weeks were purchased from Shanghai experimental animal center and maintained in gnotobiotic animal facility properly in Zhejiang Chinese Medical University. All animal procedures were following protocols approved by the animal care and use committee from our institutions.
The K7M2 mouse osteosarcoma cell line was obtained from the Cell Bank of Chinese Academy of Science in Shanghai. Cells were cultured in Dulbecco's Modified Eagle's Medium (DMEM) supplemented with 10% fetal bovine serum, 1% L-glutamine, and 1% penicillin-streptomycin.
L-arginine was purchased from Sigma-Aldrich and diluted with PBS before use. Anti-mouse PD-L1 was obtained from Bio X Cell (West Lebanon, NH, USA) and diluted in PBS.
Tumor models and treatment
To establish in situ osteosarcoma models, 1 × 106 K7M2 cells in 20μl PBS was injected into tibia medullary cavity. For metastasis models, 5 × 105 cells in 100μl PBS was administrated via the tail vein. One week after tumor inoculation (Day 7), the mice were randomized into 4 different treatment groups, each having at least 10 mice per group as follows: vehicle control (PBS), L-arginine single-agent treatment (L-arg), α-PD-L1 antibody single-agent treatment (α-PD-L1), and combination treatment (Combo). L-arginine was oral administrated daily at 2 g/kg body weight for 2 weeks (metastasis models) or 4 weeks (in situ models). α-PD-L1 antibody was injected i.p. 3 times a week at 200 μg for 2 weeks (metastasis models) or 4 weeks (in situ models). Survival rate was monitored.
Sample processing
For mice to be prepared for ELISA and flow cytometry analysis, 5 mice from each group were anesthetized by i.p. administration of Ketamine (9 mg/ml in saline) and Xylazine (0.9 mg/ml in saline). Peripheral blood was collected via cardia puncture when the mice were anesthetized. Then the spleens, in situ tumor tissues, the lungs and lymph nodes were harvested. The spleens were mashed, underwent red blood cell lysis, and passed through 40 μm cell strainers. Similarly, lymph nodes were mashed and passed through 40 μm cell strainers. For tumor tissues, the tumors were disaggregated by razors and incubated at 37°C for 2 hours in DMEM with collagenase type IV (2 mg/ml, Sigma), DNase (0.1 mg/ml, Sigma), and hyaluronidase (0.1 mg/ml, Sigma). Cell suspensions were passed through 70 μm cell strainers to remove aggregates. All the cells mentioned above were washed with cell staining buffer (Biolegend, San Diego, CA, USA) and then ready for antibody incubation.
ELISA
Serum IFN-γ concentration was evaluated by ELISA (R&D Systems, Minneapolis, MN, USA) following the manufacturer's instructions.
Flow cytometry
The following antibodies were purchased from Biolegend: anti-CD3 (clone 17A2), anti-CD45 (clone 30-F11), anti-CD4 (clone GK1.5), anti-CD8α (clone 53–6.7), anti-IFN-γ (clone XMG1.2), anti-CD11b (clone M1/70), and anti-Gr-1 (Clone RB6–8C5). Conjugated antibodies obtained from eBioscience were as follows: anti-granzyme B (clone NGZB), anti-perforin (clone eBioOMAK-D), and anti-PD-1 (clone J43). Live cells were determined by LIVE/DEAD® staining (ThermoFisher). For cell surface markers, cells were stained with antibodies at room temperature for 30 minutes. For intracellular antigen detection, an intracellular staining kit containing fixation/permeabilization reagents from eBioscience was used. Flow cytometry analyses were performed on BD LSRFortessa (BD Biosciences). For splenocytes and cells from lymph nodes, 1 × 105 events were collected for each sample. For intracellular lymphocytes, 6 × 106 events for each tumor sample were collected to obtain enough data. All gating strategies were determined by FMO (fluorescence minus one). Flowjo 10 was used to analyze the data.
Statistical analysis
Survival curves were illustrated using Kaplan-Meyer method. Statistical analysis was determined by one-way ANOVA with Bonferroni's multiple comparison. All the analysis was performed on GraphPad Prism 6. Data were presented as mean ± SEM. P < 0.05 was regarded as statistical significant.
Disclosure of potential conflicts of interest
No potential conflicts of interest were disclosed.
Supplemental_Data.zip
Download Zip (973.6 KB)References
- Ferrari S, Serra M. An update on chemotherapy for osteosarcoma. Expert Opin Pharmacother 2015; 16(18):2727-36; PMID:26512909; http://dx.doi.org/10.1517/14656566.2015.1102226
- Wang Z, Li B, Ren Y, Ye Z. T-cell-based immunotherapy for osteosarcoma: challenges and opportunities. Front Immunol 2016; 7:353; PMID:27683579; http://dx.doi.org/10.3389/fimmu.2016.00353
- Ahmed N, Brawley VS, Hegde M, Robertson C, Ghazi A, Gerken C, Liu E, Dakhova O, Ashoori A, Corder A, et al. Human Epidermal Growth Factor Receptor 2 (HER2) -specific chimeric antigen receptor-modified T cells for the immunotherapy of HER2-positive sarcoma. J Clin Oncol 2015; 33(15):1688-96; PMID:25800760; http://dx.doi.org/10.1200/JCO.2014.58.0225
- Huang G, Yu L, Cooper LJ, Hollomon M, Huls H, Kleinerman ES. Genetically modified T cells targeting interleukin-11 receptor alpha-chain kill human osteosarcoma cells and induce the regression of established osteosarcoma lung metastases. Cancer Res 2012; 72(1):271-81; PMID:22075555; http://dx.doi.org/10.1158/0008-5472.CAN-11-2778
- Li B, Zhu X, Sun L, Yuan L, Zhang J, Li H, Ye Z. Induction of a specific CD8+ T-cell response to cancer/testis antigens by demethylating pre-treatment against osteosarcoma. Oncotarget 2014; 5(21):10791-802; PMID:25301731; http://dx.doi.org/10.18632/oncotarget.2505
- Shen JK, Cote GM, Choy E, Yang P, Harmon D, Schwab J, Nielsen GP, Chebib I, Ferrone S, Wang Y. Programmed cell death ligand 1 expression in osteosarcoma. Cancer Immunol Res 2014; 2(7):690-8; PMID:24866169; http://dx.doi.org/10.1158/2326-6066.CIR-13-0224
- Salvatore V, Focaroli S, Teti G, Mazzotti A, Falconi M. Changes in the gene expression of co-cultured human fibroblast cells and osteosarcoma cells: the role of microenvironment. Oncotarget 2015; 6(30):28988-98; PMID:26418748; http://dx.doi.org/10.18632/oncotarget.4902
- Lussier DM, Johnson JL, Hingorani P, Blattman JN. Combination immunotherapy with alpha-CTLA-4 and alpha-PD-L1 antibody blockade prevents immune escape and leads to complete control of metastatic osteosarcoma. J Immunother Cancer 2015; 3:21; PMID:25992292; http://dx.doi.org/10.1186/s40425-015-0067-z
- Bronte V, Serafini P, Mazzoni A, Segal DM, Zanovello P. L-arginine metabolism in myeloid cells controls T-lymphocyte functions. Trends Immunol 2003; 24(6):302-6; PMID:12810105; http://dx.doi.org/10.1016/S1471-4906(03)00132-7
- Bronte V, Kasic T, Gri G, Gallana K, Borsellino G, Marigo I, Battistini L, Iafrate M, Prayer-Galetti T, Pagano F, et al. Boosting antitumor responses of T lymphocytes infiltrating human prostate cancers. J Exp Med 2005; 201(8):1257-68; PMID:15824085; http://dx.doi.org/10.1084/jem.20042028
- Patil MD, Bhaumik J, Babykutty S, Banerjee UC, Fukumura D. Arginine dependence of tumor cells: targeting a chink in cancer's armor. Oncogene 2016; 35(38):4957-72; PMID:27109103; http://dx.doi.org/10.1038/onc.2016.37
- Chang CH, Qiu J, O'Sullivan D, Buck MD, Noguchi T, Curtis JD, Chen Q, Gindin M, Gubin MM, van der Windt GJ, et al. Metabolic competition in the tumor microenvironment is a driver of cancer progression. Cell 2015; 162(6):1229-41; PMID:26321679; http://dx.doi.org/10.1016/j.cell.2015.08.016
- Rodriguez PC, Quiceno DG, Ochoa AC. L-arginine availability regulates T-lymphocyte cell-cycle progression. Blood 2007; 109(4):1568-73; PMID:17023580; http://dx.doi.org/10.1182/blood-2006-06-031856
- Rodriguez PC, Zea AH, Culotta KS, Zabaleta J, Ochoa JB, Ochoa AC. Regulation of T cell receptor CD3zeta chain expression by L-arginine. J Biol Chem 2002; 277(24):21123-9; PMID:11950832; http://dx.doi.org/10.1074/jbc.M110675200
- Geiger R, Rieckmann JC, Wolf T, Basso C, Feng Y, Fuhrer T, Kogadeeva M, Picotti P, Meissner F, Mann M, et al. L-arginine modulates T cell metabolism and enhances survival and anti-tumor activity. Cell 2016; 167(3):829-42; PMID:27745970; http://dx.doi.org/10.1016/j.cell.2016.09.031
- Cao Y, Wang Q, Du Y, Liu F, Zhang Y, Feng Y, Jin F. l-arginine and docetaxel synergistically enhance anti-tumor immunity by modifying the immune status of tumor-bearing mice. Int Immunopharmacol 2016; 35:7-14; PMID:27003114; http://dx.doi.org/10.1016/j.intimp.2016.03.002
- Jackson HJ, Rafiq S, Brentjens RJ. Driving CAR T-cells forward. Nat Rev Clin Oncol 2016; 13(6):370-83; PMID:27000958; http://dx.doi.org/10.1038/nrclinonc.2016.36
- Robbins PF, Kassim SH, Tran TL, Crystal JS, Morgan RA, Feldman SA, Yang JC, Dudley ME, Wunderlich JR, Sherry RM, et al. A pilot trial using lymphocytes genetically engineered with an NY-ESO-1-reactive T-cell receptor: long-term follow-up and correlates with response. Clin Cancer Res 2015; 21(5):1019-27; PMID:25538264; http://dx.doi.org/10.1158/1078-0432.CCR-14-2708
- Lettieri CK, Appel N, Labban N, Lussier DM, Blattman JN, Hingorani P. Progress and opportunities for immune therapeutics in osteosarcoma. Immunotherapy 2016; 8(10):1233-44; PMID:27605071; http://dx.doi.org/10.2217/imt-2016-0048
- Ho PC, Bihuniak JD, Macintyre AN, Staron M, Liu X, Amezquita R, Tsui YC, Cui G, Micevic G, Perales JC, et al. Phosphoenolpyruvate is a metabolic checkpoint of anti-tumor T cell responses. Cell 2015; 162(6):1217-28; PMID:26321681; http://dx.doi.org/10.1016/j.cell.2015.08.012
- Dufait I, Schwarze JK, Liechtenstein T, Leonard W, Jiang H, Escors D, De Ridder M, Breckpot K. Ex vivo generation of myeloid-derived suppressor cells that model the tumor immunosuppressive environment in colorectal cancer. Oncotarget 2015; 6(14):12369-82; PMID:25869209; http://dx.doi.org/10.18632/oncotarget.3682
- Zou W, Wolchok JD, Chen L. PD-L1 (B7-H1) and PD-1 pathway blockade for cancer therapy: mechanisms, response biomarkers, and combinations. Sci Transl Med 2016; 8(328):324r-8r; PMID:26936508; http://dx.doi.org/10.1126/scitranslmed.aad7118
- Chen L, Han X. Anti-PD-1/PD-L1 therapy of human cancer: past, present, and future. J Clin Invest 2015; 125(9):3384-91; PMID:26325035; http://dx.doi.org/10.1172/JCI80011