ABSTRACT
Background: Eukaryote initiation factor 2 subunit β (eIF2β) plays a crucial role in regulation protein synthesis, which mediates the interaction of eIF2 with mRNA. eIF2β contains evolutionarily conserved polylysine stretches in amino-terminal region and a zinc finger motif in the carboxy-terminus.
Methods: The gene eIF2β was cloned under tetracycline transcription control and the polylysine stretches were deleted by site-directed mutagenesis (eIF2βΔ3K). The plasmid was transfected into HEK 293 TetR cells. These cells were analyzed for their proliferative and translation capacities as well as cell death rate. Experiments were performed using gene reporter assays, western blotting, flow cytometry, cell sorting, cell proliferation assays and confocal immunofluorescence.
Results: eIF2βΔ3K affected negatively the protein synthesis, cell proliferation and cell survival causing G2 cell cycle arrest and increased cell death, acting in a negative dominant manner against the native protein. Polylysine stretches are also essential for eIF2β translocated from the cytoplasm to the nucleus, accumulating in the nucleolus and eIF2βΔ3K did not make this translocation.
Discussion: eIF2β is involved in the protein synthesis process and should act in nuclear processes as well. eIF2βΔ3K reduces cell proliferation and causes cell death. Since translation control is essential for normal cell function and survival, the development of drugs or molecules that inhibit translation has become of great interest in the scenario of proliferative disorders. In conclusion, our results suggest the dominant negative eIF2βΔ3K as a therapeutic strategy for the treatment of proliferative disorders and that eIF2β polylysine stretch domains are promising targets for this.
Abbreviations
Cy3 | = | cyanine 3 |
DAPI | = | 4′,6-diamidino-2-phenylindole |
dEGFP | = | destabilized variant of enhancedgreen fluorescent protein |
EGFP | = | enhancedgreen fluorescent protein |
eIF2β | = | eukaryote initiation factor 2 β |
eIF2βWT | = | wild type eIF2β |
WT | = | wild type eIF2β |
eIF2βΔ3K | = | eIF2β without polylysine stretches |
FITC | = | fluorescein isothiocyanate |
H-DMEM | = | High Glucose Dulbecco's modified Eagle's medium |
Met-tRNAi | = | methionyl-initiator transfer |
RNA, TetR | = | tetracycline repressor |
XTT | = | 2,3-bis-(2-Methoxy-4-Nitro-5-Sulfophenyl)-2H-Tetrazolium-5-Carboxanilide |
Introduction
The initiation of protein synthesis in eukaryotes requires the participation of multiple protein components, known as eukaryotic initiation factors (eIFs),Citation1-3 which form transient and specific complexes following a precise sequence of events.Citation2,4,5 eIF2 is a multifunctional heterotrimeric protein that drives the Met-tRNAi to the P site of the small ribosomal subunit in a GTP-dependent manner, a crucial step in the initiation of protein synthesis.Citation2,5-7 In addition, eIF2 is involved in mRNA binding and start codon recognition.Citation5,8,9 The availability of active eIF2 can be reduced by a wide range of stress conditions, thus repressing the global mRNA translation.Citation10 These effects, which are conserved throughout eukaryotes, act mainly through phosphorylation of the eIF2 α subunit.Citation5,11
Previous studies based on yeast genetic approaches and recombinant mammalian proteins have shown that the β subunit of eIF2 (eIF2β) plays a major role in the binding of eIF2 to other factors: eIF2β binds to eIF2Bϵ,Citation12,13 to eIF5,Citation14,15 to mRNACitation9 and to eIF3.Citation16 eIF2β has also been implicated in eIF2 interactions with other proteins such as DNA-dependent protein kinase (DNA-PK)Citation17 and casein kinase 2 (CK-2).Citation18 Moreover, eIF2β binds to the adaptor protein Nck-1,Citation19 preventing the eIF2α phosphorylation in response to endoplasmic reticulum stress.Citation20
The carboxyl-terminal region of eIF2β contains a zinc-finger motif that contributes to mRNA binding and start codon selection during the scanning process.Citation21 The amino-terminal region of eIF2β, which is conserved from yeast to humans, contains 3 polylysine stretchesCitation22 and phosphorylation sites for CK2 and protein kinase C (PKC).Citation23 The polylysine stretches participate in the binding of eIF2β to eIF5, eIF2Bϵ and mRNA.Citation9,13,15 In yeast, deletion of the polylysine stretches compromises cell growth, which points to an important role for this structural feature.Citation9,15 So far, these effects of eIF2β on cell growth have not yet been explored in mammalian cells, although this alternative could be an important option for designing therapies for proliferative disorders.
The overall increase in protein synthesis capacity is associated with the increase in tumor cell survival, proliferation and development of chemotherapy resistance.Citation24 Studies have shown that the altered expression of many other eIFs is associated with malignant transformation, cancer prognosis and the regulation of gene expression.Citation25 The modified expression levels of eIFs can up- or down-regulate the cytoplasmic translation of several mRNAs encoding important proteins for different cellular processes, such as cell cycle, proliferation, survival and apoptosis.Citation25 Therefore, alteration of their functions could deregulate gene expression and consequently lead to cancer development. Besides, the eIFs cellular localization is important for a variety of other cellular processes.Citation26-31
Evidences have also shown that some eIFs, including eIF2β, have altered cell localization and impair different cellular processes in several tumor types. Studies have also demonstrated that the αCitation32-34 and βCitation35,36 subunits of eIF2 are located in the cytoplasm and can likewise be located in the nucleus of different cell types, but nuclear functions have not yet been described for these proteins. Some other eIFs, such as eIF1A, eIF3, eIF4AIII, eIF4E, eIF4GI, eIF5A and eIF6, have been also detected in the nucleus or nucleolus of mammalian cells.Citation30,37-41 The role of eIFs within the nucleus is unclear, but several reports indicate that they participate in nucleocytoplasmic mRNA transport, mRNA splicing, ribosomal biogenesis, cytokine regulation, and nonsense-mediated mRNA decay.Citation26-31
The aim of this work is to study the effect of eIF2βΔ3K (eIF2β polylysine stretches deleted) protein on protein synthesis, proliferation and cell death, as well as the subcellular location of this eIF2β mutant.
Materials and methods
This is an experimental study performed with human cells line, in a molecular biology laboratory. The institutional Review Board of Post-Graduation Program in Cellular and Molecular Biology gave prior approval for the study procedures.
In summary, we performed site directed mutagenesis on the human eIF2β, deleting the polylysine stretches in its amino-terminal region. Wild type eIF2β (eIF2βWT) and eIF2β without polylysine stretches (eIF2βΔ3K) were cloned, under the control tetracycline-regulated CMV promoter. Details on the methodology used in the assays are described below.
Antibodies and reagents
Mouse anti-IF2β monoclonal antibody (eIF2β moAb) catalog number: sc-133209 was obtained from Santa Cruz Biotechnology. Rabbit anti-TetR polyclonal antibody was obtained from Abcam (catalog number: ab-33249). The human serum used as positive control for nucleolar specific antigen (anti-nucleolus) of ANA Test System was purchased from Immuno Concepts (catalog number: 2023). Anti-rabbit or anti-mouse peroxidase-conjugated and anti-mouse Cy3 or FITC-conjugated antibodies were obtained from Sigma Chemical (catalog number C2181 and F8646). Tetracycline was obtained from Acros Organics (catalog number: 64–75–5) and solubilized in absolute ethanol at 1 mg/mL. RNaseA (20 mg/mL) was purchased from Invitrogen (catalog number: 12091021).
Plasmid constructions
The cDNA encoding full-length human eIF2β (eIF2βWT) was generated by polymerase chain reaction (PCR) using a HeLa cDNA library (Matchmaker cDNA library; Clontech, Palo Alto, CA, USA). Deletion of the polylysine stretches was achieved by site-directed mutagenesis with the Site Mutagenesis System from Promega, using specific oligonucleotides as shown in .
Table 1. Primer sequences used for eIF2β polylysine stretches deletion.
The tetracycline-regulated plasmids were constructed from a pcDNA4/TO plasmid (Invitrogen), where the dEGFP cDNA from the pd1EGFP-N1 vector (Clontech) was subcloned at position 1052 (named pJL). The cDNAs of eIF2βWT and eIF2βΔ3K (eIF2β without polylysine stretches) were cloned into pJL and named pJL::eIF2βWT and pJL::eIF2βΔ3K, respectively. eIF2βWT and eIF2βΔ3K are thus under tetracycline-regulated expression control, whereas dEGFP is constitutively expressed.
For enhanced green fluorescent protein (EGFP) fused proteins eIF2βWT and eIF2βΔ3K expression, the respective cDNAs were cloned in the plasmid pEGFP-C1 (Clontech, CA, USA) downstream to EGFP generating pEGFP::eIF2βWT and pEGFP::eIF2βΔ3K. For RNA binding assays, constructs of eIF2β were generated for the analysis of its subcellular localization. The fragments of eIF2βWT, eIF2βΔ3K were cloned into the pcDNA4/TO vector and named pGDS::eIF2βWT, pGDS::eIF2βΔ3K, respectively. In all cases, the resulting sequences were confirmed by DNA sequencing.
Transfection, cell culture and treatments
Hek293TetR (R710–07, Invitrogen, CA, USA) cells were seeded at a density of 1.5 × 105 cells/well in 24-well plates in High Glucose Dulbecco's modified Eagle's medium (H-DMEM) supplemented with 10% fetal bovine serum, 2 mmol/l L-glutamine, 100 units/ml penicillin, and 100 g/ml streptomycin (H-DMEM complete medium) at a final volume of 500 µl. The following day, cells were transfected with pJL, pJL::eIF2βWT or pJL::eIF2βΔ3K using Lipofectamine 2000 (Invitrogen) according to the manufacturer's instructions. After 6 h, the complete medium was changed, and after 24 h, cells were selected with 150 µg/mL of zeocin (Invitrogen, catalog number: R25001).
To analyze eIF2β subcellular localization, 1.5 × 105 Hek293 cells (ATCC CRL-1573) were plated per well in 24-well plates on poly-D-lysine coated coverslips (Ø 10 mm) in H-DMEM complete medium at a final volume of 500 µl and transfected with the plasmids pEGFP-C1, pEGFP::eIF2βWT and pEGFP::eIF2βΔ3K as described, then evaluated by their fluorescence. To study RNA binding to eIF2β, 0.8 × 105 HeLa cells (ATCC CCL-2) were plated per well in 24-well plates on coverslips (Ø 10mm) in H-DMEM complete medium and transfected with the plasmids pcDNA4/TO, pGDS::eIF2βWT, pGDS::eIF2βΔ3K as described and analyzed using immunocytochemistry.
Cell viability assay
Selected Hek293TetR cells expressing pJL, pJL::eIF2βWT or pJL::eIF2βΔ3K were plated in 96-well plates at a density of 6 × 103 cells/well of H-DMEM complete medium at a final volume of 200 µl. Cells were treated with 1 µg/mL tetracycline with renewal of medium and tetracycline every 48 h. Untreated cells (no tetracycline addition) were used as controls. After 24, 48, 72 and 96 h of treatment, cell viability was assayed using the tetrazolium salt XTT (2,3-bis-(2-methoxy-4-nitro-5-sulfophenyl)-2H-tetrazolium-5-carboxanilide) method following the manufacturers (Roche Applied Science; catalog number: 11465015001) recommendations.
Cell proliferation assay
Cell sorting on a BD FACS Aria II (BD Biosciences, San Jose, CA, USA) was used to select populations of Hek293TeTR cells expressing pJL, pJL::eIF2βWT or pJL::eIF2βΔ3K and expressing similar and homogeneous intensity levels of dEGFP. The selected cells were plated in 96-well plates at a density of 100 cells/well in H-DMEM complete medium at a final volume of 200 µl. Cells were treated with tetracycline (1 µg/ml) for 24, 48, 72, 96 h and a half tetracycline dose was added after each 48 h. Untreated cells were used as controls. After 96 h of culture, the treated and untreated cells were incubated with 1 µCi/well of (methyl-3H)thymidine triphosphate (PerkinElmer, MA, USA; catalog number:NET027L) for 24 h, then the cells were transferred to a Cell Harvesters FilterMate Universal Harvester (PerkinElmer, MA, USA) scintillation filter. The samples were counted in a Wallac 1450 Microβ (PerkinElmer, MA, USA) β scintillation counter.
Determination of population doubling
Hek293TetR cells expressing pJL, pJL::eIF2βWT or pJL::eIF2βΔ3K were plated at each passage in 24-well plates at a density of 5 × 104 cells/well at a final volume of 500 µl and treated with 1 µg/mL tetracycline with renewal of medium and tetracycline every 48 h. Untreated cells were used as controls. After each passage, cells were counted and population doubling (PD) was determined according to the formula PD = (log N(t)-logN(to))/log 2, where N(t) is the number of cells per well at time of passage, and N(to) is the number of cells seeded at the previous passage. The sum of PDs was then plotted in relation to the time of culture.
Protein synthesis assay
A destabilized variant of enhanced green fluorescent protein (dEGFP) characterized by a rapid protein turnover and a half-life of 1 hCitation42 was used for the protein synthesis assay.Citation43 Briefly, Hek293TetR cells expressing pJL, pJL::eIF2βWT or pJL::eIF2βΔ3K were plated in 6-well plates at 1.8 × 105 cells/well at a final volume of 2 ml. After 24 h, cells were treated with tetracycline (1 µg/ml) for 24, 48, 72 and 96 h, with renewal of medium and tetracycline every 48 h. Untreated cells were used as controls. Treated and untreated cells were harvested, trypsinized and distributed in 96-well plates for cytometry analysis. Ten thousand events were recorded for the dEGFP expression evaluation in the flow cytometer (Guava Easy CytePlus System, Guava Technologies, Millipore, MA, USA). To improve this assay, Hek293TetR cells expressing pJL, pJL::eIF2βWT or pJL::eIF2βΔ3K were sorted for the homogeneous dEGFP expression range by BD FACS Aria II (BD Biosciences, San Jose, CA, USA) and the same procedure described above was performed for protein synthesis measurement.
Western blotting
0.6 × 106 Hek293TetR cells expressing pJL, pJL::eIF2βWT or pJL::eIF2βΔ3K were washed once with cold phosphate-buffered saline (PBS), lysed in 200 µL Laemmli lysis buffer (60 mMTris-HCl, pH 6.8, 10% glycerol, 2% sodium dodecyl sulfate (SDS), 1% 2-mercaptoethanol and 0.002% bromophenol blue) and boiled for 5 min. The protein extract from 105 cells was loaded per lane, resolved in a gradient SDS-PAGE gel (10–20%) and transferred to nitrocellulose membranes. Mouse anti-eIF2β moAb (1:500) or rabbit anti-TetR antibody (1:500) was used. The peroxidase-conjugated anti-mouse (1:2,000) or anti-rabbit (1:2,000) was used as a secondary antibody.
Cell cycle analysis
The cell cycle analyses were performed with Hek293TetR cells expressing pJL, pJL::eIF2βWT or pJL::eIF2βΔ3K. The cells were plated in 6-well plates at 1.8 × 105 cells/well at a final volume of 2 ml. After 24 h, cells were treated with tetracycline (1 µg/ml) for 48 and 96 h. After each 48 h of treatment, the tetracycline medium was changed and the removed supernatants were centrifuged. The pellet was resuspended and put again on the same culture. Untreated cells were used as controls. Treated and untreated cells were harvested by trypsin digestion and fixed with 0.3% paraformaldehyde/PBS for 10 min and after with 70% cold ethanol/PBS. Before the analysis the cells were washed with PBS and cell pellets were resuspended in 500 μL PBS containing 20µg/ml propidium iodide and 100 µg/ml RNase, incubated for 30 minutes at 37°C. The cell cycle analyses were performed on 5 thousand cellular events using a C6 flow cytometer (AccuriCitometers, USA), doublets discrimination was done on FL-H vs FL2-A graphic. Percentage of each cell cycle phase was analyzed in CFlow software (AccuriCitometers).
Cell death analysis
Hek293TetR cells expressing pJL, pJL::eIF2βWT or pJL::eIF2βΔ3K were plated at each passage in 24-well plates at a density of 5 × 104 cells/well at a final volume of 500 µl and treated with 1 µg/mL tetracycline with renewal of medium and tetracycline every 48 h. Untreated cells were used as controls. After 48 h and 96 h passage, dead cells were measured by trypan blue exclusion method. The cell suspension was diluted 1:1 with 0.4% trypan blue and viable and non-viable cells were counted on Neubauer chamber.
Subcellular location prediction
Subcelullar location of each construct was previously analyzed by using MultiLocCitation44 and PSORTIICitation45 subcellular prediction algorithms (https://abi.inf.uni-tuebingen.de/Services/MultiLoc and http://psort.hgc.jp/form2.html, respectively)
Immunocytochemistry and fluorescence analysis
Twenty-four hours after transfection with pEGFP-C1, pEGFP::eIF2βWT or pEGFP::eIF2βΔ3K, Hek293 cells were fixed with 4% formaldehyde/PBS for 20 min, permeabilized with 0.5% Triton X-100/PBS for 10 min, and then incubated with 3% BSA/PBS for 1 h. Cells were incubated with anti-nucleolus for 2 hours, followed by anti-human Cy3-conjugated Ig (1:100) for 1 hour, and finally labeled with DAPI 1 µg/mL (Invitrogen) for 10 min. Images were acquired from numerous fields using a 4 X or 60 X objective (oil immersion) on a confocal microscope (FluoView FV1000, Olympus, Japan).
RNA binding assay
0.8 × 105 HeLa cells/well were plated in 24-well plates on coverslips (Ø 10mm) in H-DMEM complete medium at a final volume of 500 µL and transfected with pGDS::eIF2βWT on the next day. Twenty-four hours after transfection, cells were fixed with methanol for 5 min at −20°C followed by acetone for 2 min at −20°C and permeabilized with 0.1% Triton X-100/PBS for 3 min on ice, and then incubated with 3% BSA/PBS for 1 h. The RNAse reaction was performed using 0.4 mg/ml RNase A/PBS for 60 min at 37°C. Cells were incubated overnight at 4°C with eIF2β moAb (1:100), then incubated with anti-mouse Cy3-conjugated Ig (1:100) for 1 hour. The cells were incubated with anti-nucleolus for 2 hours, followed by anti-human FITC-conjugated Ig (1:100) for 1 hour, and finally labeled with DAPI 1 µg/mL (Invitrogen) for 10 minutes. Images were acquired from numerous fields using a 4 X or 60 X objective (oil immersion) on a confocal microscope (FluoView FV1000, Olympus, Japan).
Statistical analysis
Data were normalized, plotted using Graph Pad Prism 4 software and presented as the means ± the standard error. To analyze cell death the Kruskal Wallis test was used, followed by post-hoc multiple pairwise Mann-Whitney tests. To analyze the dEGFP expression, cell viability and cumulative population doubling data, analysis of variance (ANOVA) was used, followed by Tukey HSD post-hoc test, while cell proliferation was analyzed by pairwise t-tests. A P-value below 0.05 was considered significant.
Results
Overexpression of eIF2βΔ3K decreases the protein synthesis
We performed site directed mutagenesis on the human eIF2β, deleting the polylysine stretches () in the amino-terminal region of eIF2β. Wild type eIF2β (eIF2βWT) and eIF2β without polylysine stretches (eIF2βΔ3K) were cloned, under the control of a tetracycline-regulated promoter, in a plasmid that also expresses a dEGFP in a constitutive manner. This reporter gene has a rapid turnover and, therefore, its expression becomes dependent on the functionality of the expressed form of eIF2β.
Figure 1. Effect of eIF2βΔ3K expression on protein synthesis. (A) Domain structure of eIF2β. Black boxes indicate polylysine stretches, dotted boxes indicate a cysteine zinc finger domain, and vertically and diagonally striped boxes indicate interpolylysine regions of eIF2β. (B) Western blot analysis of eIF2β expression after tetracycline induction. The whole protein cell extract of 105 Hek293TetR cells containing pJL (empty vector), pJL::eIF2βWT or pJL::eIF2βΔ3K were loaded into an SDS-PAGE gel and subjected to western blot analysis with anti-eIF2β and anti-TetR. The second column of each construction shows cells that were induced by tetracycline (1 µg/ml) for 72 h. The western blot was probed with antibody against tetracycline repressor protein (TetR) and used as protein loading control and to show the constitutive TetR expression in the Hek293 cell line. (C) eIF2β expression was induced by tetracycline (1 µg/mL) for 24 to 96 h in Hek293TetR cells expressing pJL, pJL::eIF2βWT or pJL::eIF2βΔ3K. Qualitative analysis of the overall protein synthesis was performed observing dEGFP expression by fluorescence microscopy. The scale bars represent 100 μm. (D) After cell sorting of Hek293TetR cells expressing pJL, pJL::eIF2βWT or pJL::eIF2βΔ3K, protein synthesis was measured using dEGFP expression in 24, 48, 72 and 96 h by flow cytometry. The anisomycin 5 µg/mL was used as a control of the protein synthesis inhibition in Hek293TetR cells transfected with pJL. The results are presented as the mean of 3 independent experiments. (E) Flow cytometry graph representative of 96 h tetracycline treatment showing the dEGFP expression shift as a consequence of eIF2βΔ3K overexpression. Purple full histogram represents untreated cells, and green line histogram represents protein synthesis induced by tetracycline (1 µg/ml).
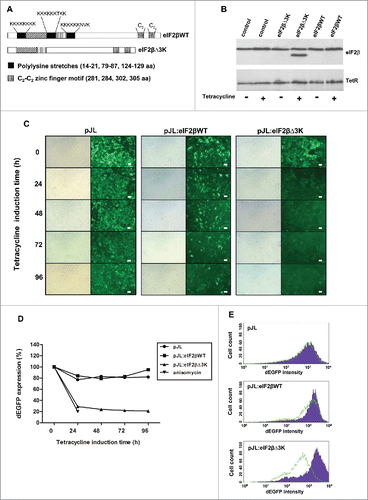
Hek293TetR cells were transfected with the empty vector (pJL), the pJL::eIF2βWT construct or the pJL::eIF2βΔ3K construct and selected with zeocine. Expression of eIF2β before and after tetracycline treatment was analyzed by western-blot in control cells transfected with pJL, pJL::eIF2βWT or pJL::eIF2βΔ3K. Tetracycline treatment in transfected cell induced the expression of both form of eIF2β (eIF2βWT and eIF2βΔ3K) () in a time dependent way (). To assess the effects of eIF2βΔ3K overexpression on protein synthesis, we analyzed the expression level of dEGFP as a protein synthesis marker by fluorescence microscopy and flow cytometry. eIF2βΔ3K expression led to a significant decrease in dEGFP expression after 24 h whereas exogenous expression of eIF2βWT did not affect dEGFP expression (). This was quantitatively confirmed by flow cytometry as dEGFP expression levels decreased drastically. The stable transfectants were first cell sorted based on their dEGFP expression level to obtain a homogeneous dEGFP expression range of each one. Cells were then treated with tetracycline for up to 96 h. Protein synthesis rate as reflected by dEGFP expression decreased approximately 80% at each of the time points for the eIF2βΔ3K plasmid but not for the eIF2βWT plasmid () based on the mean fluorescence intensity of each sample. The decrease in the expression levels obtained through eIF2βΔ3K expression was similar to that obtained using anisomycin, a classic protein synthesis inhibitor (). A representative histogram at 96 h of tetracycline treatment is shown in .
Figure 2. Western blot analysis of eIF2βWT and eIF2βΔ3K after induction with tetracycline (1 μg/mL). A shows eIF2β either endogenous and expressed from pJL::eIF2βWT, line 1: expression of eIF2βΔ3K after 24 hours of induction used as control, lines 2 to 6 expression of eIF2βWT induced after 1, 24, 48, 72 and 96 hours, respectively. B shows eIF2βΔ3K expression induced after 1, 24, 48, 72 and 96 hours, in lines 1 to 5, respectively.
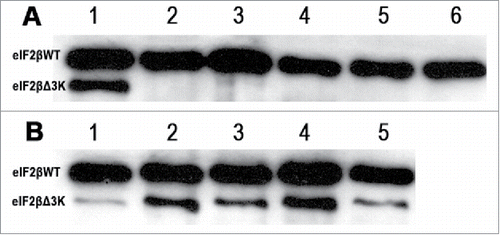
Deletion of the polylysine stretches in eIF2β decreases cell proliferation and viability and increases cell death
Since overexpression of eIF2βΔ3K reduces protein synthesis, we further analyzed the effect of this truncated form on cell proliferation and viability. Hek293TetR cells expressing pJL, pJL::eIF2βWT or pJL::eIF2βΔ3K were sorted. Deletion of the polylysine stretches in eIF2β impairs cell viability significantly by XTT assay (), as eIF2βΔ3K overexpression led to a 30% decrease in cell viability after 96 h of tetracycline treatment. Cell death analysis by trypan blue method also showed that cell viability decreases approximately 3 and 10 times after 48 and 96 h of eIF2βΔ3K expression, respectively (). Cell proliferation was then measured by (methyl-3H) thymidine incorporation. eIF2βΔ3K overexpression led to a decrease of 40%, 55% and 20% in (methyl-3H)thymidine incorporation after 24, 48 and 96 h of tetracycline treatment, respectively, when compared with the endogenous eIF2βWT (). Population doubling analysis showed that endogenous eIF2βΔ3K expression impairs cell growth in comparison to both empty vector and eIF2βWT expressing cells, leading to cell growth arrest ().
Figure 3. Effects of polylysine stretches deletion on cell viability. Gene expression of eIF2βWT or eIF2βΔ3K was induced by tetracycline (1 µg/mL) in Hek293TetR cells transfected with pJL (empty plasmid), pJL::eIF2βWT or pJL::eIF2βΔ3K. (A) Cell viability was analyzed using XTT assay after 24 to 96 h of tetracycline treatment. The viability results are expressed as the percentage (mean ± standard error mean, SEM) relative to untreated cells containing the corresponding construct. (B) Cell death was analyzed using Trypan blue exclusion method after 48 and 96 h of tetracycline induction. The death results are expressed as the percentage (mean ± SEM) of non-viable cells for each construct. Results are presented as the mean of 3 independent experiments. *indicates P < 0.05 and **indicates P < 0.01.
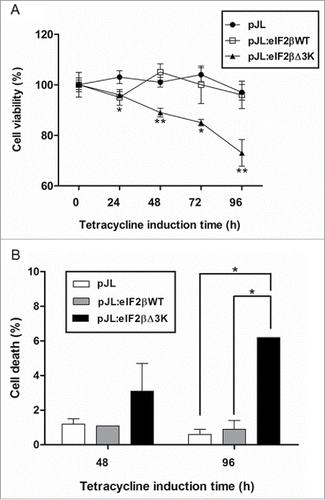
Figure 4. Deletion of the polylysine stretches affects cell proliferation. Gene expression was induced by tetracycline (1 µg/mL) in Hek293TetR cells containing pJL (empty plasmid), pJL::eIF2βWT or pJL::eIF2βΔ3K. (A) Cells were selected by cell sorting to obtain similar dEGFP expression range. Proliferation of the selected cells was analyzed by (methyl-3H)thymidine incorporation after 24, 48 and 96 h of tetracycline induction. The proliferation results are expressed as the percentage (mean ± SEM) of (methyl-3H)thymidine incorporation relative to empty plasmid at each time point. (B) Cumulative population doublings of cells were measured after 48 to 144 h of tetracycline induction. The results are expressed as the percentage (mean ± SEM) relative to tetracycline untreated cells of the corresponding construct. Results are presented as the mean of 3 independent experiments. *indicates P < 0.05, **indicates P < 0.01 and ***indicates P < 0.001.
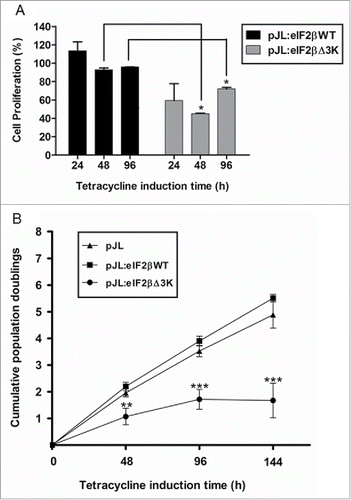
eIF2βΔ3K causes G2 cell cycle arrest
Hek293TetR cells expressing pJL, pJL::eIF2βWT or pJL::eIF2βΔ3K were cultured, treated with tetracycline for 48 and 96 h and the cell cycle analysis was performed by flow cytometry. Our results showed an increase of cells in G2 phase in tetracycline treated cells expressing eIF2βΔ3K (25%) when compared with untreated cells expressing the same plasmid (13%) after 96 h of tetracycline treatment (). This increase reached 29% after 48 h of eIF2βΔ3K expression. This result was not observed on the empty vector or eIF2βWT expressing cells.
Figure 5. Effect of eIF2βΔ3K expression on cell cycle. Gene expression was induced by tetracycline (1 µg/mL) in Hek293TetR cells containing pJL (empty plasmid), pJL::eIF2βWT or pJL::eIF2βΔ3K for 96h. Cell cycle analysis was performed by flow cytometer thought PI determined DNA content and analyzed in 96h with or without tetracycline treatment. The results are expressed as the percentage of cells in different cell cycle phase. Results are presented as the mean of 3 independent experiments. Tet = tetracycline treatment.
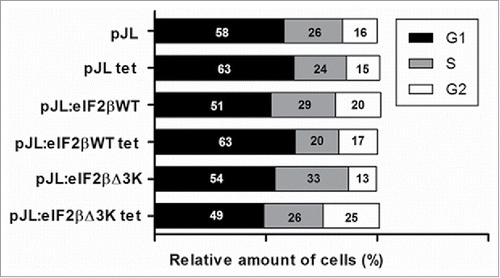
eIF2β is present in the nucleolus of human cell lines and polylysine stretches are essential for its nucleolar localization
In silico analysis using MultiLoc and PSORTII subcellular prediction algorithms indicated that eIF2β might be present in the nucleus. We therefore characterized the subcellular localization of EGFP-eIF2βWT and EGFP-eIF2βΔ3K fusion proteins in Hek293 cells. EGFP-eIF2βWT showed cytoplasmatic and nucleolar localization in Hek293 cells; however, upon overexpression of EGFP-eIF2βΔ3K, nucleolar localization was abrogated ().
Figure 6. Subcellular localization of eIF2βWT and eIF2βΔ3K in Hek293 cell lines. Cells were transfected with empty vector pEGFP-C1 (n = 73) or plasmids carrying the fusion proteins pEGFP::eIF2βWT (n = 210) or pEGFP::eIF2βΔ3K (n = 173). Twenty-four hours after transfection, cells were fixed and submitted to immunocytochemistry using anti-nucleolus human serum. DAPI was used to stain the nucleus. The subcellular localization was analyzed by confocal microscopy. Arrow shows eIF2β nucleolar staining. (n) is the number of observed cells. The scale bars represent 10 µm.
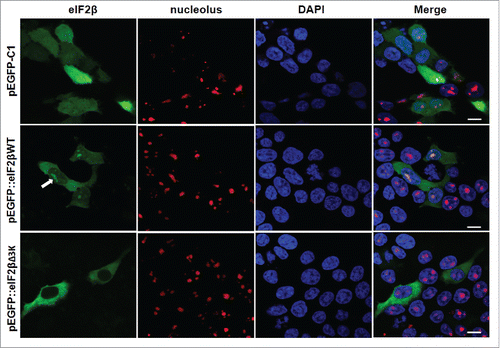
RNA is required for nucleolar localization of eIF2β
The main functions of eIF2β in the initiation translation process in the cytoplasm are to bind mRNA and facilitate AUG initial codon recognition. We are focusing on the lysine stretches and the zinc finger motifs that are known to be nucleic acid-binding motifs. Since the nucleolus is the major site of rRNA transcription and processing into pre-ribosomal particles, we examined the possible binding role of eIF2β to RNA in the nucleolus.
Using confocal microscopy, we analyzed the effect of RNase digestion on eIF2βWT localization in HeLa cells. RNase A treatment of permeabilized transfected HeLa cells extinguished the nucleolar distribution of eIF2β, but not its cytoplasmic localization (). Control experiments demonstrated that RNase A digestion also extinguished the nucleolar labeling stained with anti-nucleolus specific antibody without affecting neither DNA nor the nuclear structure as seen with the DAPI counter-stain (). Together, these data indicate that the nucleolar localization of eIF2β is stabilized by its interaction with RNA.
Figure 7. RNA is required for nucleolar localization of eIF2β. HeLa cells were grown on microscopic slides and transfected with pGDS::eIF2βWT. Twenty-four hours after transfection, cells were fixed with methanol (5 min, −20°C) followed by acetone (2 min, −20°C) and permeabilized with 0.1% Triton X-100 for 3 min on ice. The digestion with 0.4 mg/ml RNase A was performed in PBS for 60 min at 37°C. The cells were washed in PBS and processed for immunocytochemistry using moAb anti-eIF2β sc-133209. Control = buffer without RNase A. The anti-nucleolus human serum was used to monitor the efficiency of RNase A digestion. DAPI was used to stain DNA. The scale bars represent 10 μm.
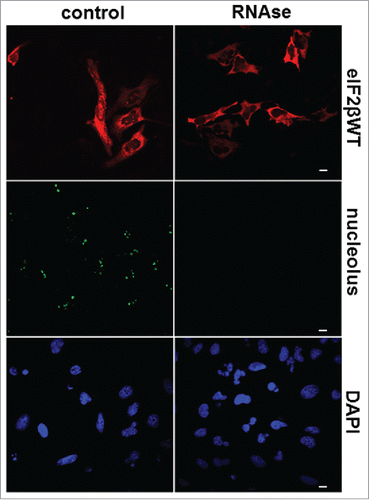
Discussion
The balance between cell survival and death is a conditional factor to cancer formation and progression. In normal cells, several key pathways determine cell protection against tumor development inducing cell death; however, some adaptive responses facilitate the survival and growth of tumor cells under severe stresses. It has already been shown that translation inhibitors can induce cell death by multiple mechanisms.Citation46 The protein synthesis blocking is, therefore, of great interest for cancer treatment, since it affects multiple processes required for tumor expansion. Altered expression of many eIFs is associated with malignant transformation, cancer prognosis and the regulation of gene expression in mammalian cells;Citation25,47,47-50 at the same time, it regulates the translation of mRNAs compromised with cell cycle, proliferation, survival and apoptosis processes.Citation25 Moreover, eIFs functionally interact with oncogenes and are often primary targets of signal transduction pathways similar to proto-oncogenes that underlie most human cancers.Citation49,50
It is well known that several eIFs show altered expression in different tumor types,Citation25,40,49,50,51,52,53,54 but depend on the modifications they present an ambiguos behavior. For example, some evidence suggests that eIFs may be a new group of tumor suppressorsCitation47,48 and, on the other hand, it has been reported that increased eIF2 activity induces neoplastic transformation and is associated with several cancers in vivo.Citation55
In addition, some potential anti-cancer substances have been suggested to act through eIF2 regulation thus reducing protein translation rate and cell cycle progression.Citation56 However, all of these studies have been focused with phosphorilation/dephosphorilation process as a mean to interrupt cell proliferation.
Phosphorylation at serine 51 of the eIF2 α subunit may be an important determinant of this behavior in response to stress. Nevertheless, depending on the duration of the stress injury 2 events may occur. Thus, a short-term eIF2α phosphorylation induces cell tumor survival while the induction of a long-term phosphorylation is associated with cell death.Citation51 KoromilasCitation51 has proposed that regulation of eIF2α phosphorylation at serine 51 is important to determine cell fate in response to stress, exhibiting a pro-survival function in response to several stress conditions. Therefore, eIF2α phosphorylation could significantly promote tumor progression and resistance to therapy or exert a suppressive effect on tumorigenesis.Citation57
RosenwaldCitation55 has proposed that during tumor progression cells might lose the eIF2α functionality to avoid the inhibition of protein synthesis by eIF2α phosphorylation. Hence, β and γ eIF2 subunits would be able to maintain the functional eIF2 complex and the protein synthesis process and thus sustain the cancer progression in tumor cells. In this way, it is important explore the functions of the others subunits of eIF2, such as eIF2β, on the tumor formation and progression and its efficacy in anti-tumor therapies.
A study on the eIF2β role in cancer progression has pointed to the eIF2β anti-tumoral effect.Citation58 Heterozygous deletion of eIF2β gene reduces testicular germ cell tumors (TGCTs) in mice and homozygous deletion of eIF2β leads to mice embryonic death.Citation58 This effect shows that germ cell development and TGCT pathogenesis are sensitive to changes in the rate of translation close related to the availability and modulation of the eIF2β.
The eIF2β protein contains one evolutionarily conserved sequence in its aminoterminal region consisting of 3 stretches of polylysines that are implicated in the binding of eIF2β to eIF5, eIF2Bϵ and mRNA.Citation9,13,15 In this work, we first studied if polylysine stretches deletion would affect the protein synthesis in human cells. Data from some studies suggest that the polylysine stretches are not required for eIF2 trimer formation in yeast and that the carboxyl-terminal region of eIF2β is sufficient for the formation of a complex with eIF2α.Citation9 However, considering that the polylysine stretches are evolutionarily conserved and that they were shown to be important for the binding to others eIFs and mRNA, we hypothesized that polylysine residues deletion might affect the translation initiation multifactor complex formationCitation59 and thus interfere with the global translation processes. In fact, the expression of human eIF2β in which the polylysine stretches were deleted (eIF2βΔ3K) caused a decrease in the rate of protein synthesis in this study. This finding is comparable to the classic protein synthesis inhibitor, anisomycin. Our results agree with data from our previous in vitro study using yeast eIF2β mutants that showed that the polylysine stretches deletion reduces global protein synthesis processCitation9 and it is also lethal for yeast cells.Citation9,15 Now, we were able to reproduce this theory in human cells.
Our experiments obtained the reduction in both human cell viability and cell proliferation upon expression of eIF2βΔ3K, a small lysine region. A previous study has also used mutation to slow down the rate of protein synthesis, but only with full deletion of the amino-terminal region of human eIF2β, decreasing the translation rate and cell viability.Citation35 In the same way that other mutant forms of eIFs,Citation60-63 the mutant eIF2βΔ3K proposed here also interferes on the cell cycle progression.
In human cells, translational control by eIF4E overexpression contributes to the G1–S progression.Citation64 The mammalian eIF4E controls the translation of D cyclins mRNAs which are involved on G1-S cell-cycle progression, so that eIF4E overexpression increases expression of cyclin D1.Citation65,66 Moreover, nuclear eIF4E enhances the transport of the D1 cyclin mRNA to cytoplasm.Citation67 On the other hand, the eIF4A mutants, as well as a strain overexpressing a dominant negative construct of eIF4A, lead to arrest in G2 phase.Citation60 The mutant eIF2βΔ3K also causes a G2/M cell cycle arrest. Together, our results indicate that this mutant protein functionally acts as a dominant negative inhibitor of protein synthesis, competing with the endogenous form of eIF2β, and thereby affecting cell survival.
In addition to polylysine stretches fundamental role on the cytoplasmic activity of eIF2β, our in silico analysis revealed that they could act as nuclear localization signal (NLS) in this eIF2 subunit. Translation is a process taking place in the cytoplasm, however, some studies have shown that several eIFs, including eIF2, present nuclear localization where they can play different functions unrelated to global protein synthesis process.Citation17,36,67-69
eIF2β, together with eIF2α, eIF2γ, eIF4A, eIF5A and eIF6, has also been identified as nucleolar in HeLa cells by proteomic approaches,Citation37,38 but this data was not validated until now. Experimentally, we observe that eIF2βWT overexpression leads to its nuclear translocation, with a strong accumulation in the nucleolus. However, the nucleolar localization was abrogated in the mutant eIF2βΔ3K.
The nucleolus is a sub-nuclear compartment where the synthesis and maturation of rRNA occur, as well as the assembly of rRNA into ribosomal particles. Some reports have shown that the nucleolus is also implicated in gene silencing, cell cycle regulation, viral replication, signal recognition particle assembly, senescence, modification of small RNPs, nuclear export pathways, tRNA processing, p53 regulation and telomerase function.Citation70-72 Our data indicate that eIF2β nucleolar localization is dependent upon its RNA binding, and that nucleolar integrity is required for its accumulation in this compartment, suggesting that eIF2β might also act in some process involving RNA in the nucleolus.
Taken together, our data report extensively that eIF2β expression actively controls protein synthesis, and consequently interferes in cell proliferation and survival, corroboring early studies.Citation9,35 Also, we showed that eIF2β is found in the nucleolus. Our main findings are that eIF2β polylysine stretches are essential for cell proliferation and for eIF2β nucleolar localization. The alterations of eIF2β cytoplasmic functions on the translation process should be considered as the main factor affecting the cell proliferation and viability. Furthermore, changes on the eIF2β subcellular location by polylysine stretches deletion could also be interfering in cell survival and this request for further investigation. Inhibiting drugs and molecules of global translation process may be effective in several tumor types including even classically chemo-resistant cancers. Therefore, we propose that the polylysine stretch domains of eIF2β be promising targets for efficient antiproliferative therapeutic strategies and that the dominant negative construct eIF2βΔ3K could be a very interesting tool for use in treatment of abnormal cell proliferation events such as tumors or increased immune proliferation.
Disclosure of potential conflicts of interest
No potential conflicts of interest were disclosed.
Acknowledgments
The authors are grateful to Dr. Sergio Echeverrigaray for critical analysis and text review and to Dr. Dominique Charron for valuable advice.
Funding
This work was supported by grants from CNPq (no. 481621/2007–5), FIPE-HCPA (no. 05–576) and PRONEX/FAPERGS/CNPq (no. 10/0044–3). G.D.S. was supported by fellowships from CAPES. N.O.M. was supported by grants from PNPD/CAPES (no. 23038.8306/2010–62). J.P.L. was supported by fellowships from ABTO/SFT.
References
- Sonenberg N, Dever TE. Eukaryotic translation initiation factors and regulators. Curr Opin Struct Biol 2003; 13(1):56-63; PMID:12581660; https://doi.org/10.1016/S0959-440X(03)00009-5
- Jackson RJ, Hellen CU, Pestova TV. The mechanism of eukaryotic translation initiation and principles of its regulation. Nat Rev Mol Cell Biol 2010; 11(2):113-27; PMID:20094052; https://doi.org/10.1038/nrm2838
- Sonenberg N, Hinnebusch AG. Regulation of translation initiation in eukaryotes: Mechanisms and biological targets. Cell 2009; 136(4):731-45; PMID:19239892; https://doi.org/10.1016/j.cell.2009.01.042
- Kapp LD, Lorsch JR. The molecular mechanics of eukaryotic translation. Annu Rev Biochem 2004; 73:657-704; PMID:15189156; https://doi.org/10.1146/annurev.biochem.73.030403.080419
- Hinnebusch AG. Molecular mechanism of scanning and start codon selection in eukaryotes. Microbiol Mol Biol Rev 2011; 75(3):434-67; PMID:21885680; https://doi.org/10.1128/MMBR.00008-11
- Lorsch JR, Dever TE. Molecular view of 43 S complex formation and start site selection in eukaryotic translation initiation. J Biol Chem 2010; 285(28):21203-7; PMID:20444698; https://doi.org/10.1074/jbc.R110.119743
- Kapp LD, Lorsch JR. GTP-dependent recognition of the methionine moiety on initiator tRNA by translation factor eIF2. J Mol Biol 2004; 335(4):923-36; PMID:14698289; https://doi.org/10.1016/j.jmb.2003.11.025
- Castilho-Valavicius B, Thompson GM, Donahue TF. Mutation analysis of the Cys-X2-Cys-X19-Cys-X2-Cys motif in the beta subunit of eukaryotic translation initiation factor 2. Gene Expr 1992; 2(3):297-309; PMID:1450666.
- Laurino JP, Thompson GM, Pacheco E, Castilho BA. The beta subunit of eukaryotic translation initiation factor 2 binds mRNA through the lysine repeats and a region comprising the C2-C2 motif. Mol Cell Biol 1999; 19(1):173-81; PMID:9858542; https://doi.org/10.1128/MCB.19.1.173
- Proud CG. eIF2 and the control of cell physiology. Semin Cell Dev Biol 2005; 16(1):3-12; PMID:15659334; https://doi.org/10.1016/j.semcdb.2004.11.004
- Hinnebusch AG, Lorsch JR. The mechanism of eukaryotic translation initiation: New insights and challenges. Cold Spring Harb Perspect Biol 2012; 4(10):pii: a011544; PMID:22815232; https://doi.org/10.1101/cshperspect.a011544
- Kimball SR. Eukaryotic initiation factor eIF2. Int J Biochem Cell Biol 1999; 31(1):25-9; PMID:10216940; https://doi.org/10.1016/S1357-2725(98)00128-9
- Kimball SR, Heinzinger NK, Horetsky RL, Jefferson LS. Identification of interprotein interactions between the subunits of eukaryotic initiation factors eIF2 and eIF2B. J Biol Chem 1998; 273(5):3039-44; PMID:9446619; https://doi.org/10.1074/jbc.273.5.3039
- Das S, Maitra U. Mutational analysis of mammalian translation initiation factor 5 (eIF5): Role of interaction between the beta subunit of eIF2 and eIF5 in eIF5 function in vitro and in vivo. Mol Cell Biol 2000; 20(11):3942-50; PMID:10805737; https://doi.org/10.1128/MCB.20.11.3942-3950.2000
- Asano K, Krishnamoorthy T, Phan L, Pavitt GD, Hinnebusch AG. Conserved bipartite motifs in yeast eIF5 and eIF2Bepsilon, GTPase-activating and GDP-GTP exchange factors in translation initiation, mediate binding to their common substrate eIF2. EMBO J 1999; 18(6):1673-88; PMID:10075937; https://doi.org/10.1093/emboj/18.6.1673
- Valásek L, Nielsen KH, Hinnebusch AG. Direct eIF2-eIF3 contact in the multifactor complex is important for translation initiation in vivo. EMBO J 2002; 21(21):5886-98; PMID:12411506; https://doi.org/10.1093/emboj/cdf563
- Ting NS, Kao PN, Chan DW, Lintott LG, Lees-Miller SP. DNA-dependent protein kinase interacts with antigen receptor response element binding proteins NF90 and NF45. J Biol Chem 1998; 273(4):2136-45; PMID:9442054; https://doi.org/10.1074/jbc.273.4.2136
- Llorens F, Roher N, Miró FA, Sarno S, Ruiz FX, Meggio F, Plana M, Pinna LA, Itarte E. Eukaryotic translation-initiation factor eIF2beta binds to protein kinase CK2: Effects on CK2alpha activity. Biochem J 2003; 375(Pt 3):623-31; PMID:12901717; https://doi.org/10.1042/bj20030915
- Kebache S, Zuo D, Chevet E, Larose L. Modulation of protein translation by Nck-1. Proc Natl Acad Sci U S A 2002; 99(8):5406-11; PMID:11959995; https://doi.org/10.1073/pnas.082483399
- Kebache S, Cardin E, Nguyên DT, Chevet E, Larose L. Nck-1 antagonizes the endoplasmic reticulum stress-induced inhibition of translation. J Biol Chem 2004; 279(10):9662-71; PMID:14676213; https://doi.org/10.1074/jbc.M310535200
- Donahue TF, Cigan AM, Pabich EK, Valavicius BC. Mutations at a Zn(II) finger motif in the yeast eIF-2 beta gene alter ribosomal start-site selection during the scanning process. Cell 1988; 54(5):621-32; PMID:3136928; https://doi.org/10.1016/S0092-8674(88)80006-0
- Pathak VK, Nielsen PJ, Trachsel H, Hershey JW. Structure of the beta subunit of translational initiation factor eIF-2. Cell 1988; 54(5):633-9; PMID:3044606; https://doi.org/10.1016/S0092-8674(88)80007-2
- Welsh GI, Price NT, Bladergroen BA, Bloomberg G, Proud CG. Identification of novel phosphorylation sites in the beta-subunit of translation initiation factor eIF-2. Biochem Biophys Res Commun 1994; 201(3):1279-88; PMID:8024572; https://doi.org/10.1006/bbrc.1994.1843
- Robert F, Roman W, Bramoullé A, Fellmann C, Roulston A, Shustik C, Porco JA Jr5, Shore GC6, Sebag M7, Pelletier J8. Translation initiation factor eIF4F modifies the dexamethasone response in multiple myeloma. Proc Natl Acad Sci U S A 2014; 111(37):13421-6; PMID:25197055; https://doi.org/10.1073/pnas.1402650111
- Yin JY, Dong Z, Liu ZQ, Zhang JT. Translational control gone awry: A new mechanism of tumorigenesis and novel targets of cancer treatments. Biosci Rep 2011; 31(1):1-15; PMID:20964625; https://doi.org/10.1042/BSR20100077
- Ferraiuolo MA, Lee CS, Ler LW, Hsu JL, Costa-Mattioli M, Luo MJ, Reed R, Sonenberg N. A nuclear translation-like factor eIF4AIII is recruited to the mRNA during splicing and functions in nonsense-mediated decay. Proc Natl Acad Sci U S A 2004; 101(12):4118-23; PMID:15024115; https://doi.org/10.1073/pnas.0400933101
- Miluzio A, Beugnet A, Volta V, Biffo S. Eukaryotic initiation factor 6 mediates a continuum between 60S ribosome biogenesis and translation. EMBO Rep 2009; 10(5):459-65; PMID:19373251; https://doi.org/10.1038/embor.2009.70
- Kafasla P, Barrass JD, Thompson E, Fromont-Racine M, Jacquier A, Beggs JD, Lewis J. Interaction of yeast eIF4G with spliceosome components: Implications in pre-mRNA processing events. RNA Biol 2009; 6(5):563-74; PMID:19838078; https://doi.org/10.4161/rna.6.5.9861
- McKendrick L, Thompson E, Ferreira J, Morley SJ, Lewis JD. Interaction of eukaryotic translation initiation factor 4G with the nuclear cap-binding complex provides a link between nuclear and cytoplasmic functions of the m(7) guanosine cap. Mol Cell Biol 2001; 21(11):3632-41; PMID:11340157; https://doi.org/10.1128/MCB.21.11.3632-3641.2001
- Choe J, Ryu I, Park OH, Park J, Cho H, Yoo JS, Chi SW, Kim MK, Song HK, Kim YK. eIF4AIII enhances translation of nuclear cap-binding complex-bound mRNAs by promoting disruption of secondary structures in 5′UTR. Proc Natl Acad Sci U S A 2014; 111(43):E4577-86; PMID:25313076; https://doi.org/10.1073/pnas.1409695111
- Baboo S, Cook PR. “Dark matter” worlds of unstable RNA and protein. Nucleus 2014; 5(4):281-6; PMID:25482115; https://doi.org/10.4161/nucl.29577
- Lobo MV, Martin ME, Pérez MI, Alonso FJ, Redondo C, Alvarez MI, Salinas M. Levels, phosphorylation status and cellular localization of translational factor eIF2 in gastrointestinal carcinomas. Histochem J 2000; 32(3):139-50; PMID:10841309; https://doi.org/10.1023/A:1004091122351
- Tejada S, Lobo MV, Garcia-Villanueva M, Sacristán S, Pérez-Morgado MI, Salinas M, Martín ME. Eukaryotic initiation factors (eIF) 2alpha and 4E expression, localization, and phosphorylation in brain tumors. J Histochem Cytochem 2009; 57(5):503-12; PMID:19188486; https://doi.org/10.1369/jhc.2009.952929
- Goldstein EN, Owen CR, White BC, Rafols JA. Ultrastructural localization of phosphorylated eIF2alpha [eIF2alpha(P)] in rat dorsal hippocampus during reperfusion. Acta Neuropathol 1999; 98(5):493-505; PMID:10541873; https://doi.org/10.1007/s004010051115
- Llorens F, Duarri A, Sarró E, Roher N, Plana M, Itarte E. The N-terminal domain of the human eIF2beta subunit and the CK2 phosphorylation sites are required for its function. Biochem J 2006; 394(Pt 1):227-36; PMID:16225457; https://doi.org/10.1042/BJ20050605
- Bohnsack MT, Regener K, Schwappach B, Saffrich R, Paraskeva E, Hartmann E, Görlich D. Exp5 exports eEF1A via tRNA from nuclei and synergizes with other transport pathways to confine translation to the cytoplasm. EMBO J 2002; 21(22):6205-15; PMID:12426392; https://doi.org/10.1093/emboj/cdf613
- Andersen JS, Lyon CE, Fox AH, Leung AK, Lam YW, Steen H, Mann M, Lamond AI. Directed proteomic analysis of the human nucleolus. Curr Biol 2002; 12(1):1-11; PMID:11790298; https://doi.org/10.1016/S0960-9822(01)00650-9
- Leung AK, Trinkle-Mulcahy L, Lam YW, Andersen JS, Mann M, Lamond AI. NOPdb: Nucleolar Proteome Database. Nucleic Acids Res 2006; 34(Database issue):D218-20; PMID:16381850; https://doi.org/10.1093/nar/gkj004
- Mingot JM, Vega S, Cano A, Portillo F, Nieto MA. eEF1A mediates the nuclear export of SNAG-containing proteins via the Exportin5-aminoacyl-tRNA complex. Cell Rep 2013; 5(3):727-37; PMID:24209753; https://doi.org/10.1016/j.celrep.2013.09.030
- Brina D, Miluzio A, Ricciardi S, Biffo S. eIF6 anti-association activity is required for ribosome biogenesis, translational control and tumor progression. Biochim Biophys Acta 2015; 1849(7):830-5; PMID:25252159; https://doi.org/10.1016/j.bbagrm.2014.09.010
- Jiang Q, Li F, Shi K, Wu P, An J, Yang Y, Xu C. Involvement of p38 in signal switching from autophagy to apoptosis via the PERK/eIF2alpha/ATF4 axis in selenite-treated NB4 cells. Cell Death Dis 2014; 5:e1270; PMID:24874742; https://doi.org/10.1038/cddis.2014.200
- Andersen JB, Sternberg C, Poulsen LK, Bjorn SP, Givskov M, Molin S. New unstable variants of green fluorescent protein for studies of transient gene expression in bacteria. Appl Environ Microbiol 1998; 64(6):2240-6; PMID:9603842
- Quiñones B, Massey S, Friedman M, Swimley MS, Teter K. Novel cell-based method to detect Shiga toxin 2 from Escherichia coli O157:H7 and inhibitors of toxin activity. Appl Environ Microbiol 2009; 75(5):1410-6; PMID:19139230; https://doi.org/10.1128/AEM.02230-08
- Höglund A, Dönnes P, Blum T, Adolph HW, Kohlbacher O. MultiLoc: Prediction of protein subcellular localization using N-terminal targeting sequences, sequence motifs and amino acid composition. Bioinformatics 2006; 22(10):1158-65; PMID:16428265; https://doi.org/10.1093/bioinformatics/btl002
- Nakai K, Horton P. PSORT: A program for detecting sorting signals in proteins and predicting their subcellular localization. Trends Biochem Sci 1999; 24(1):34-6; PMID:10087920; https://doi.org/10.1016/S0968-0004(98)01336-X
- Lindqvist LM, Vikström I, Chambers JM, McArthur K, Ann Anderson M, Henley KJ, Happo L, Cluse L, Johnstone RW, Roberts AW. Translation inhibitors induce cell death by multiple mechanisms and Mcl-1 reduction is only a minor contributor. Cell Death Dis 2012; 3:e409; PMID:23059828; https://doi.org/10.1038/cddis.2012.149
- De Benedetti A, Graff JR. eIF-4E expression and its role in malignancies and metastases. Oncogene 2004; 23(18):3189-99; PMID:15094768; https://doi.org/10.1038/sj.onc.1207545
- Dong Z, Zhang JT. Initiation factor eIF3 and regulation of mRNA translation, cell growth, and cancer. Crit Rev Oncol Hematol 2006; 59(3):169-80; PMID:16829125; https://doi.org/10.1016/j.critrevonc.2006.03.005
- Silvera D, Formenti SC, Schneider RJ. Translational control in cancer. Nat Rev Cancer 2010; 10(4):254-66; PMID:20332778; https://doi.org/10.1038/nrc2824
- Stumpf CR, Ruggero D. The cancerous translation apparatus. Curr Opin Genet Dev 2011; 21(4):474-83; PMID:21543223; https://doi.org/10.1016/j.gde.2011.03.007
- Koromilas AE. Roles of the translation initiation factor eIF2α serine 51 phosphorylation in cancer formation and treatment. Biochem Biophys Acta 2015; 1849(7):871-80; PMID:25497381
- Mathews MB, Hershey JW. The translation factor eIF5A and human cancer. Biochim Biophys Acta 2015; 1849(7):836-44; PMID:25979826; https://doi.org/10.1016/j.bbagrm.2015.05.002
- Spilka R, Ernst C, Bergler H, Rainer J, Flechsig S, Vogetseder A, Lederer E, Benesch M, Brunner A, Geley S. eIF3a is over-expressed in urinary bladder cancer and influences its phenotype independent of translation initiation. Cell Oncol (Dordr) 2014; 37(4):253-67; PMID:25070653; https://doi.org/10.1007/s13402-014-0181-9
- Li Z, Lin S, Jiang T, Wang J, Lu H, Tang H, Teng M, Fan J. Overexpression of eIF3e is correlated with colon tumor development and poor prognosis. Int J Clin Exp Pathol 2014; 7(10):6462-74; PMID:25400724
- Rosenwald IB. The role of translation in neoplastic transformation from a pathologist's point of view. Oncogene 2004; 23(18):3230-47; PMID:15094773; https://doi.org/10.1038/sj.onc.1207552
- Palakurthi SS, Fluckiger R, Aktas H, Changolkar AK, Shahsafaei A, Harneit S, Kilic E, Halperin JA. Inhibition of translation initiation mediates the anticancer effect of the n-3 polyunsaturated fatty acid eicosapentaenoic acid. Cancer Res 2000; 60(11):2919-25; PMID:10850438
- Zheng Q, Ye J, Cao J. Translational regulator eIF2α in tumor. Tumour Biol 2014; 35(7):6255-64; PMID:24609900; https://doi.org/10.1007/s13277-014-1789-0
- Heaney JD, Michelson MV, Youngren KK, Lam MT, Nadeau JH. Deletion of eIF2beta suppresses testicular cancer incidence and causes recessive lethality in agouti-yellow mice. Hum Mol Genet 2009; 18(8):1395-404; PMID:19168544; https://doi.org/10.1093/hmg/ddp045
- Singh CR, Yamamoto Y, Asano K. Physical association of eukaryotic initiation factor (eIF) 5 carboxyl-terminal domain with the lysine-rich eIF2beta segment strongly enhances its binding to eIF3. J Biol Chem 2004; 279(48):49644-55; PMID:15377664; https://doi.org/10.1074/jbc.M409609200
- Nurse P, Thuriaux P, Nasmyth K. Genetic control of the cell division cycle in the fission yeast Schizosaccharomyces pombe. Mol Gen Genet 1976; 146(2):167-78; PMID:958201; https://doi.org/10.1007/BF00268085
- Kronja I, Orr-Weaver TL. Translational regulation of the cell cycle: When, where, how and why? Philos Trans R Soc London B Biol Sci. 2011; 366(1584):3638-52; PMID:22084390; https://doi.org/10.1098/rstb.2011.0084
- Franklin-Dumont TM, Chatterjee C, Wasserman SA, Dinardo S. A novel eIF4G homolog, Off-schedule, couples translational control to meiosis and differentiation in Drosophila spermatocytes. Development 2007; 134(15):2851-61; PMID:17611222; https://doi.org/10.1242/dev.003517
- Baker CC, Fuller MT. Translational control of meiotic cell cycle progression and spermatid differentiation in male germ cells by a novel eIF4G homolog. Development 2007; 134(15):2863-9; PMID:17611220; https://doi.org/10.1242/dev.003764
- Fingar DC, Richardson CJ, Tee AR, Cheatham L, Tsou C, Blenis J. mTOR controls cell cycle progression through its cell growth effectors S6K1 and 4E-BP1/eukaryotic translation initiation factor 4E. Mol Cell Biol 2004; 24(1):200-16; PMID:14673156; https://doi.org/10.1128/MCB.24.1.200-216.2004
- Rosenwald IB, Lazaris-Karatzas A, Sonenberg N, Schmidt EV. Elevated levels of cyclin D1 protein in response to increased expression of eukaryotic initiation factor 4E. Mol Cell Biol 1993; 13(12):7358-63; PMID:8246956; https://doi.org/10.1128/MCB.13.12.7358
- Rosenwald IB, Kaspar R, Rousseau D, Gehrke L, Leboulch P, Chen JJ, Schmidt EV, Sonenberg N, London IM. Eukaryotic translation initiation factor 4E regulates expression of cyclin D1 at transcriptional and post-transcriptional levels. J Biol Chem 1995; 270(36):21176-80; PMID:7673150; https://doi.org/10.1074/jbc.270.36.21176
- Rousseau D, Kaspar R, Rosenwald I, Gehrke L, Sonenberg N. Translation initiation of ornithine decarboxylase and nucleocytoplasmic transport of cyclin D1 mRNA are increased in cells overexpressing eukaryotic initiation factor 4E. Proc Natl Acad Sci U S A 1996; 93(3):1065-70; PMID:8577715; https://doi.org/10.1073/pnas.93.3.1065
- Chan CC, Dostie J, Diem MD, Feng W, Mann M, Rappsilber J, Dreyfuss G. eIF4A3 is a novel component of the exon junction complex. RNA 2004; 10(2):200-9; PMID:14730019; https://doi.org/10.1261/rna.5230104
- Dostie J, Lejbkowicz F, Sonenberg N. Nuclear eukaryotic initiation factor 4E (eIF4E) colocalizes with splicing factors in speckles. J Cell Biol 2000; 148(2):239-47; PMID:10648556; https://doi.org/10.1083/jcb.148.2.239
- Olson MO, Hingorani K, Szebeni A. Conventional and nonconventional roles of the nucleolus. Int Rev Cytol 2002; 219:199-266; PMID:12211630
- Sirri V, Urcuqui-Inchima S, Roussel P, Hernandez-Verdun D. Nucleolus: The fascinating nuclear body. Histochem Cell Biol 2008; 129(1):13-31; PMID:18046571; https://doi.org/10.1007/s00418-007-0359-6
- Mayer C, Grummt I. Cellular stress and nucleolar function. Cell Cycle 2005; 4(8):1036-8; PMID:16205120; https://doi.org/10.4161/cc.4.8.1925