ABSTRACT
Elaiophylin is a natural compound and a novel and potent inhibitor of late stage autophagy with outstanding antitumor activity in human ovarian cancer cells. However, the possible biologic effects and functional linkage between elaiophylin and multiple myeloma (MM) have not been explored. This study aimed to assess the effect of elaiophylin on MM cells with mutant TP53 and the possible molecular mechanism. The results suggested that elaiophylin exerted anti-myeloma activity by inducing apoptosis and proliferation arrest. As expected, elaiophylin blocked autophagy flux in MM cells. Subsequently, persistent activation of endoplasmic reticulum (ER) stress was induced. Moreover, the apoptotic effect was to some extent attenuated by the ER stress inhibitor tauroursodeoxycholic acid (TUDCA). Further studies indicated that elaiophylin effectively suppressed MM cell growth without obvious side effects in zebrafish embryo and mouse xenograft models. Taken together, our data are the first to demonstrate that exposure of human MM cells with mutant TP53 to elaiophylin blocked autophagy flux and thus induced cell death, which partially involved ER stress-associated apoptosis. Targeted disruption of the cellular protein handling system by elaiophylin is therefore a promising therapeutic strategy for overcoming incurable MM, even when TP53 mutations are present.
Introduction
Multiple myeloma (MM) is the second most commonly diagnosedCitation1 and incurable hematologic malignancy arising from plasma cells.Citation2 Currently, bortezomib (Velcade™) is one of the most promising anti-cancer drugs to treat MM, and it has improved the clinical outcome of MM patients.Citation3-5 In spite of this prominent clinical success, some patients still failed to respond to PIs (proteasome inhibitors)-based therapy due to primary and/or acquisition of resistant mutations.Citation6
The p53 tumor suppressor, which is encoded by TP53, acts as a critical regulator of many important cellular behaviors.Citation7-10 A defective p53 response occurs in >50% of cancers primarily due to gene mutation. Indeed, its dysfunction at the genetic, epigenetic or protein level has been associated with oncogenesis, cancer progression and resistance to therapy.Citation11
Although TP53 mutations are relatively rare in newly diagnosed MM patients, they confer markedly worse overall survival relative to patients without TP53 mutation. Therefore, it is an independent poor prognostic factor.Citation11,12 In accordance with primary MM samples, human myeloma cell lines (HMCLs) with TP53 mutations have similar malignant biologic behaviors.Citation13 Several lines of evidence suggest that MM cells with impaired p53 signaling are more resistant to genotoxic and non-genotoxic drug treatment relative to wild-type counterpart cells.Citation13 Searching for a feasible therapeutic strategy to overcome mutant TP53 clones is an urgent clinical issue.
MM is a clonal B cell malignancy derived from plasma cells that is characterized by prodigious synthesis of immunoglobulins. To avoid excessive accumulation of toxic misfolded immunoglobulins, MM cells particularly depend on protein handling pathways, including the unfolded protein response (UPR), the ubiquitin proteasome system (UPS), and the autophagy process. Together, these pathways maintain intracellular protein homeostasis.Citation14
Autophagy and the UPS are the 2 main mechanisms responsible for intracellular protein clearance. The proteasome digests soluble ubiquitin-conjugated proteins but is not involved in the degradation of all misfolded or damaged proteins. However, the autophagy process can degrade both aggregated and soluble proteins.Citation15,16 High levels of autophagy have been observed in both MM cell lines and primary MM samples.Citation17,18 However, too little or too much autophagy may be deleterious. This biologic process, which makes MM stronger, can also kill it.Citation19 Thus, a steady-state of autophagy may be a key point in regulating and determining the fate of MM cells.Citation19
In addition, the endoplasmic reticulum (ER) is an organelle with crucial biosynthetic functions. Once specific factors induce an imbalance between the ER protein folding capacity and load, the accumulation of unfolded proteins in the ER lumen leads to a condition referred to as “ER stress.”Citation20 This activates the UPR, which is an evolutionarily conserved compensatory reaction. The UPR initially attempts to alleviate the protein load in the ER by coordinating a temporal block in protein translation and initiating a series of gene transcriptional programs to enhance ER folding capacity. If the adaptive UPR fails to reestablish ER homeostasis, persistent and severe ER stress will induce cell death by shifting the UPR to the fatal phase.
Increasing evidence has suggested that autophagy and the UPR are interlinked and exert both suicidal and protective activity, acting as a double-edged sword.Citation21 Disrupting these processes may be a potential therapeutic strategy to overcome resistant malignant clones with TP53 abnormalities.
Previously, by screening the North China Pharmaceutical Group Corporation's pure compound library of microbial origin, our group selected a compound termed “elaiophylin,” which is an autophagy inhibitor.Citation22 Elaiophylin is a macrolide antibiotic extracted from Streptomycin melanosporusCitation23 with significant antitumor efficacy in human ovarian cancer cells both in vitro and in vivo. However, the role of elaiophylin in MM cells with TP53 mutations remains unclear.
In the current study, we analyzed the effects of elaiophylin on MM cells with defective p53 pathway activityCitation18 both in vitro and in vivo. The results revealed that elaiophylin not only blocked autophagy flux but also induced ER stress-associated apoptosis. Additionally, elaiophylin-induced ER stress-associated apoptosis contributed, at least in part, to MM cell death.
Results
Elaiophylin impaired cell viability and colony formation capacity in HMCLs
Elaiophylin is a 16-member macrolide antibiotic with C2 symmetry isolated from Streptomycin melanosporus (Fig. S1).Citation23 To study the effects of elaiophylin on MM cell lines, we treated H929 (TP53 wild-type), KMS11 (TP53 homogenous deletion), U266 (TP53 mutant) and RPMI8226 (TP53 mutant) cells in 96-well plates with various elaiophylin concentrations (0∼1.5 μM) for up for 48 h. Based on Cell Counting Kit-8 (CCK-8) assays, the in vitro viability of all 4 of the cell lines was significantly suppressed by elaiophylin in a time- and dose-dependent manner (). Furthermore, to evaluate the long-term effects of elaiophylin, we performed colony formation assays. As illustrated in and , the colony-forming capacity was decreased with elaiophylin treatment (1.0 μM) by approximately 62%, 70%, 90% and 95% in H929, KMS11, U266 and RPMI8226 cells, respectively, compared with the DMSO control group.
Figure 1. Elaiophylin inhibited cell viability and colony formation in MM cell lines. (A) Cell viability of U266, RPMI8226, KMS11 and H929 cells after 24- and 48-h exposure to various concentrations of elaiophylin (0∼1.5 μM). (B) Effect of bortezomib on the viability of 4 MM cell lines (U266, RPMI8226, KMS11 and H929) for 48 h. (C) Colony formation assay of all cell lines with elaiophylin treatment (1.0 μM) for 14 d. Representative images from the surviving colonies in culture dishes are shown left of (D) the quantification and statistical analysis of the colonies. All data are expressed as the means ± SD for triplicate samples and were analyzed with an unpaired t-test. (*P < 0.05, **P < 0.01, and ***P < 0.001 vs. control).
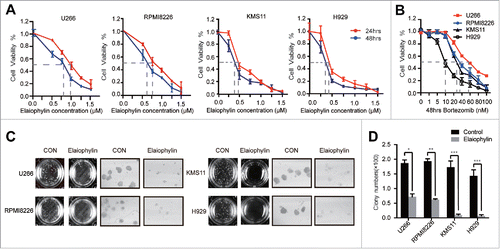
Effects of elaiophylin on TP53 mutant HMCL (U266 and RPMI8226) proliferation and apoptosis
In accordance with a previous study,Citation24 after exposure to bortezomib for 48 h, the TP53 mutant HMCLs (U266 and RPMI8226) proved relatively resistant to this type of proteasome inhibitor,Citation24 with IC50 values of approximately 59 nM and 38 nM, respectively (), whereas the KMS11 and H929 cells exhibited lower IC50 values, approximately 26 nM for KMS11 cells and 10 nM for H929 cells (). In view of the relative non-sensitivity to bortezomib, other genotoxic drugs and an MDM2 inhibitor (nutlin-3),Citation13 we decided to focus on the effects of elaiophylin in these TP53 mutant cells. The data described above represent the overall effects of elaiophylin. Next, we aimed to detect cell proliferation and apoptosis, 2 of the main processes that contribute to cell viability, in response to elaiophylin exposure proliferation and apoptosis were tested using flow cytometry with a Click-iT™ Plus Edu kit and an Annexin V-FITC/PI kit, respectively. The percentage of Edu-positive cells was decreased in a dose-dependent manner in 24 h ( and ). The fraction of Annexin V-positive cells represents the apoptotic cells. The rate of cell apoptosis was increased in a dose- and time-dependent manner (). Consistently, the expression of cleaved caspase-3 (CASP3) and PARP (), as well as the CASP3 enzymatic activity (), was significantly increased after treatment of 24 h, in a concentration-dependent manner. Moreover, elaiophylin was also capable of inducing apoptosis in the other 2 cell lines (Fig. S2A).
Figure 2. Elaiophylin affected cell proliferation and apoptosis. (A and B) The percentage of Edu-positive U266 and RPMI8226 cells was detected via flow cytometry after 24 h of elaiophylin treatment. (C) Flow cytometric analyses of apoptotic effects using an Annexin V-FITC/PtdIns kit. Both cell lines were incubated with elaiophylin (0∼1.5 μM) or DMSO as the control for 24 or 48 h. (D) The expression of cleaved CASP3 and cleaved PARP in 24 h was determined using western blotting. GAPDH served as a loading control. (E) CASP3 enzymatic activity in elaiophylin-treated cells for 24 h is presented as the fold change normalized to that of cells in the DMSO group. The graphs illustrate the means ± SD of 3 independent results, and the data were analyzed via ANOVA (*P < 0.05, **P < 0.01, and ***P < 0.001 vs. control).
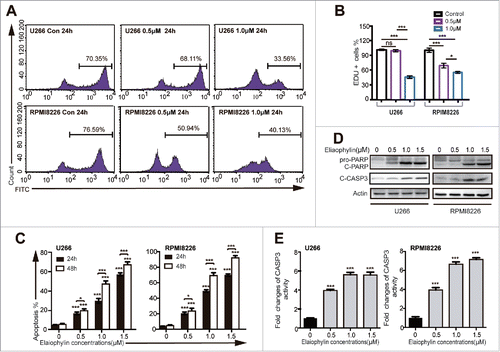
Elaiophylin blocked autophagy flux in TP53 mutant HMCLs
Given that elaiophylin universally interrupts autophagy flux in mammalian solid tumor cells,Citation22 we next assessed whether elaiophylin had the same function in TP53 mutant HMCLs. As shown in , exposure of U266 and RPMI8226 cells to elaiophylin (0.75 μM) for 12 h and 24 h led to markedly increased endogenous MAP1LC3B-II puncta formation, which was quantitated as the average number of MAP1LC3B-II puncta per cell and was shown in . The conversion of MAP1LC3B-I to MAP1LC3B-II is widely recognized as a canonical marker of autophagosome formation,Citation25,26 and it can be increased either by lysosome degradation inhibition or autophagy initiation.Citation27 To evaluate this dynamic autophagy process, we established stable tandem monomeric RFP-GFP-LC3 (tfLC3)-expressing U266 cells to monitor autophagy flux. RFP+GFP+ LC3 was predominantly diffusely distributed in the engineered cells in complete culture medium, with a few RFP+GFP− LC3B dots representing the basal autophagy level. As expected, RFP+GFP− and RFP+GFP+ LC3B puncta were increased upon rapamycin (250 nM) and chloroquine (CQ) (20 μM) treatment, respectively. Elaiophylin (0.75 μM)-treated cells had a greater number of LC3B puncta, especially in RFP+GFP+ vesicles, than other cells ( and ). Additionally, elaiophylin-treated cells did not exhibit a significant increase in the MAP1LC3B-II/GAPDH ratio in CQ-treated cells compared with control cells ( and ). Furthermore, we performed immunoblotting to synchronously monitor the expression of both MAP1LC3B-I/II and SQSTM1/p62 protein, which is an autophagic cargo recognition protein and is abrogated in the final stage of autophagy in the auto-lysosome degradation process.Citation28 Data revealed an accumulation of SQSTM1/p62 followed by increased conversion of MAP1LC3B-I to MAP1LC3B-II ( and ; Fig. S2B). Collectively, these findings indicated that elaiophylin inhibited late stage of autophagy flux in MM cell lines.
Figure 3. Elaiophylin blocked autophagy flux. (A and B) Immunofluorescence microscopy was used to detect endogenous MAP1LC3B-II puncta formation per cell. The data are presented as the means ± SD of 3 samples, with at least 100 cells in every sample (*P < 0.05, **P < 0.01, and ***P < 0.001). (C) Representative fluorescence images and (D) statistical analysis of U266 cells transfected with mRFP-GFP-LC3 lentivirus and treated with rapamycin (250 nM), chloroquine (20 μM) or elaiophylin (0.75 μM) for 12 h. (E) Conversion of MAP1LC3B-I to MAP1LC3B-II was detected via western blot in U266 cells treated with elaiophylin (0.75 μM) in the presence or absence of CQ (20 μM) for 12 h. (F) Quantification of the protein expression is shown as representative column graphs. (G) The protein expression of MAP1LC3B and SQSTM1 was determined with western blotting. RPMI8226 and U266 cells were treated with gradient concentrations of elaiophylin (0.5, 1.0 and 2.0 μM) for 24 h (upper panel) and incubated with 0.75 μM elaiophylin for the indicated time points (lower panel). For all western blots, GAPDH served as a protein loading control. Data (3 independent experiments) are expressed as the means ± SD and were analyzed via ANOVA (*P < 0.05, **P < 0.01, and ***P < 0.001 vs. control).
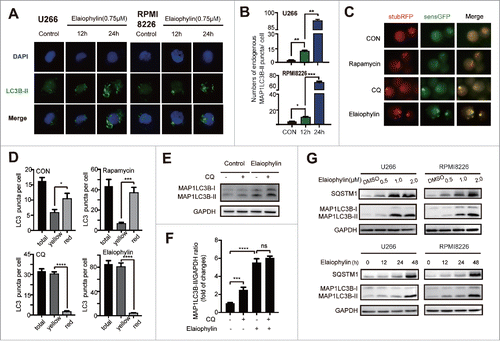
Fatal ER stress following autophagy flux blockage took part in elaiophylin-induced cytotoxicity
The previous data suggested a close link among the UPS, the autophagy-lysosome system, and ER stress.Citation21,29 Commonly, autophagy protects cell viability and is generally induced by nutrient deficiencyCitation30 or by ER stress.Citation31 The basal ongoing ER stress in MM cell lines that synthesize Ig is sufficient to sensitize cells to enhanced apoptosis when autophagy is inhibited.Citation18 Therefore, it is reasonable to speculate that elaiophylin-induced cell death may involve ER stress-associated apoptosis. To verify our hypothesis, we first performed a time-course study of the effect of elaiophylin on the expression of ER stress targets. Interestingly, a statistically significant upregulation of GRP78 (glucose-regulated protein 78 kDa), CHOP (C/EBP-homologous protein), and ATF4 (activating transcription factor 4) was validated using real-time PCR (). Activation of the PERK/ATF4/CHOP and XBP1s branches of the UPR was further confirmed with western blotting (, Fig. S2C). To elucidate the sequential relationship between ER stress activation and autophagy flux inhibition, we next synchronously monitored the expression levels of MAP1LC3B-II and GRP78 at consecutive time intervals with elaiophylin treatment. As expected, ER stress was induced following autophagy flux blockade ( and ). Subsequently, we estimated the role of ER stress-induced apoptosis in elaiophylin-triggered cell death. Tauroursodeoxycholic acid (TUDCA) is a well-known chemical chaperone capable of alleviating ER stress.Citation32,33 In the context of MM, TUDCA can also reduce ER stress induced by tunicamycin (Fig. S3). We exposed cells to elaiophylin (1.0 μM) co-incubated with TUDCA (0.75 mM) for 24 h. Immunoblot results showed that TUDCA modestly ameliorated elaiophylin-induced ER stress, as evidenced in part by downregulation of GRP78 and CHOP. Meanwhile, TUDCA reduced cleaved PARP and cleaved-CASP3 (). Importantly, cell apoptosis was rescued to a certain extent (∼). Taken together, our findings indicated that elaiophylin disrupted unfolded protein degradation homeostasis to initiate a fatal phase through autophagy flux blockade, triggering cell death in part via ER stress-induced apoptosis.
Figure 4. Elaiophylin sequentially induced ER stress. (A) Assessment of 78-kDa glucose-regulated protein (GRP78), C/EBP homologous protein (CHOP) and activating transcription factor 4 (ATF4) expression using real-time PCR in cells treated with elaiophylin (0.75 μM) for 18 and 36 h and (B) protein expression of GRP78, phospho-PERK (Thr980), ATF4, CHOP and XBP1s detected with western blotting. (C) Western blotting analysis of sequential protein expression changes in GRP78 and MAP1LC3B in cells incubated with elaiophylin (0.75 μM) for successive time points (0, 3, 6, 12, 24 and 36 h). (D) Quantification of the western blots is shown as representative column graphs. GAPDH served as a loading control. The data are shown as the means ± SD (3 independent experiments) and were analyzed using ANOVA (*P < 0.05 vs. 0 h).
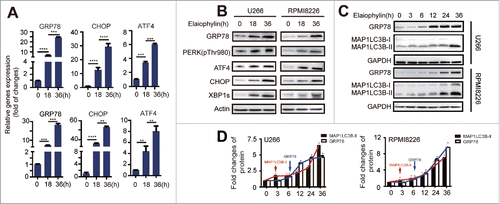
Figure 5. Elaiophylin-induced cell death partially involved ER stress-mediated apoptosis in TP53 mutant MM cell lines. U266 and RPMI8226 cells were cultured with elaiophylin (1.0 μM) or DMSO in the presence or absence of tauroursodeoxycholic acid (TUDCA, 0.75 mM) for 24 h. (A, B and C) FACS analysis of cell apoptosis. The data are presented as the means ± SD of triplicate experiments (**P < 0.01). (D) The protein expression of cleaved PARP, cleaved CASP3, GRP78 and CHOP determined by immunoblotting. GAPDH served as a loading control.
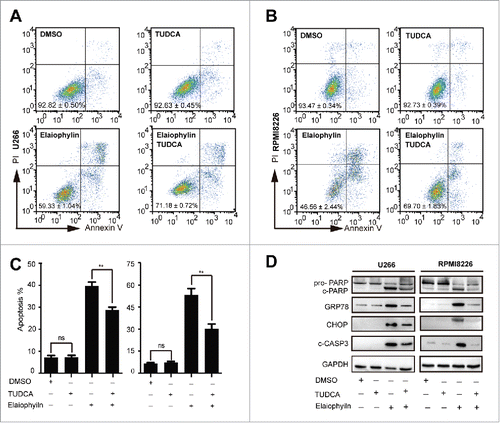
Preliminary screening of elaiophylin anti-myeloma efficacy and safety in a MM zebrafish xenograft model
Recent research has introduced a novel, rapid and manageable platform for drug screening in MM cell lines using a zebrafish xenograft model.Citation34 Having determined the efficacy of elaiophylin in vitro on TP53 mutant MM cells, we next used this platform for preliminary screening of the anti-myeloma efficacy and safety of elaiophylin in vivo. We selected U266 cell-derived xenograft zebrafish embryos successfully stained with CM-Dil, and randomly assigned them into 3 groups. For drug efficacy evaluation, they were treated with either DMSO (Control), 50 μM elaiophylin, or 10 nM bortezomib as a negative control based on a previous report.Citation34 In this previously published manuscript, a PI-resistant RPMI8226 CDX (cell line-derived xenograft) zebrafish model exhibited a relatively poor response to 10 nmol/L bortezomib.Citation24 Therefore, it is logical to postulate that another PI-resistant U266CDX zebrafish model would possess the same drug response.Citation34 To assess the side effects, the other 2 cohorts of zebrafish embryo counterparts with Matrigel injection were treated with either 50 μM elaiophylin or pure E3 buffer ( and ). Changes in the xenograft tumor volumes were estimated continuously at 12, 36 and up to 72 h post treatment (hpt). The “response standard” was identified as described previously.Citation34 As shown in and , the growth of tumor xenografts was significantly suppressed in the elaiophylin-treated group relative to the DMSO-treated group. As expected, the response of U266 cells to elaiophylin (50 μM) was over 55%, while the response to DMSO was less than 10% (). To further evaluate the overall efficacy and relative safety in vivo, we compared the accumulated survival rate of zebrafish larvae at the end time point, 72 hpt. We observed that the survival rate of elaiophylin-treated MM cell xenograft zebrafish larvae was effectively improved, without obvious side effects on the healthy counterparts, at least with regard to viability ().
Figure 6. Elaiophylin suppressed tumor growth in a U266 xenograft zebrafish model. (A) Flowchart representing the procedures for zebrafish embryo MM xenograft model establishment and the design of the experimental grouping (G1: MM+DMSO, G2: MM+10 nM bortezomib, G3: MM + 50 μM elaiophylin, G4: wild-type + 50 μM elaiophylin, G5: wild-type + E3 buffer). (B) Fluorescence microscopy images of the CM-Dil-labeled U266 MM cells injected into the perivitelline space of zebrafish larvae (Danio rerio). (C) Representative fluorescence microscopy images of the CM-Dil-stained U266 cell xenograft growth with different treatments (Control: DMSO, bortezomib: 10 nM, elaiophylin: 50 μM) of zebrafish larvae in the perivitelline area at 0, 12, 36 and 72 h post treatment (hpt). (D) Growth curves of tumor xenografts in 3 groups (G1∼G3, n = 30 for each group). (E) Percentage of zebrafish larvae without response in the 3 groups (G1∼G3, n = 30 for each group). (F) Cumulative survival rate of zebrafish embryo xenografts 72 hpt in 5 different groups.
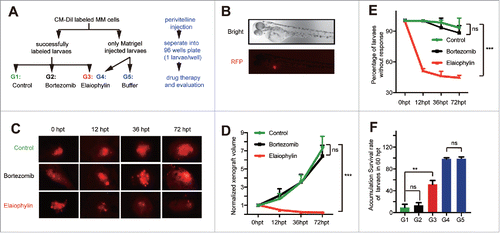
Elaiophylin exerted anti-myeloma activity in a mouse xenograft model
The in vivo effect of elaiophylin on ovarian cancer growth and metastasis has previously been evaluated in a mouse xenograft model.Citation22 To study the therapeutic benefits of elaiophylin in MM, we examined the ability of this natural compound to suppress TP53 mutant MM cells in xenograft mice (4–6 weeks old). As illustrated in , elaiophylin effectively inhibited tumor growth in vivo. In the elaiophylin treatment group, the mean tumor volumes were reduced by approximately 32% (P < 0.001) compared with the vehicle group. Karyophknosis and nuclear fragmentation were obviously apparent via H&E staining of the treated group samples (, the left panel). The immunohistochemistry images revealed a marked increase in cleaved-CASP3 expression in the tumor tissues under elaiophylin therapy compared with the control tissues (, the right panel and ). Western blotting results from the whole cell lysate of xenograft tumors indicated that the expression of the ER stress-induced apoptosis markers GRP78, CHOP, XBP1s, cleaved-CASP3 and PARP was upregulated in the treated group. Meanwhile, the conversion of MAP1LC3B-I to MAP1LC3B-II and accumulation of SQSTM1/p62 were effectively induced by elaiophylin ().
Figure 7. Elaiophylin effectively inhibited MM cells in a xenograft NOD/SCID mouse model. (A) Photographs of tumors isolated from mice after approximately 3 weeks of treatment with elaiophylin or vehicle. (B) Growth curves of the xenografts in NOD/SCID mice. (C) The statistical analysis of tumor gross weight. (D) Representative images of H&E staining (left panel) and immunohistochemical staining for cleaved CASP3 (right panel) in the tumor tissues from 2 groups. (E) Quantitation of the number of cleaved CASP3-positive cells under high magnification in 8 individual fields. (F) Western blot analyses of related protein expression in samples from the xenograft tumors in 2 groups. GAPDH was used as a loading control.
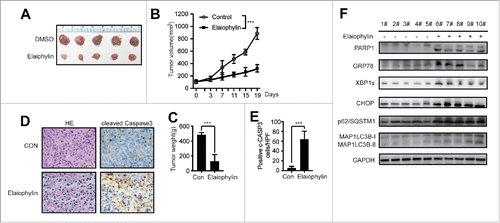
In vivo toxicity evaluation revealed that elaiophylin is a potent and safe natural compound
Drug treatment-related side effects in patients are a major issue that often hinders clinical translation. To evaluate toxicity at the effective therapeutic dosage, elaiophylin was injected into C57BL/6 mice according to the protocol described above. Elaiophylin-treated mice did not exhibit weight loss relative to the vehicle group (Fig. S4A). Dynamic analysis of the complete peripheral blood counts (Fig. S4B) and the histological morphology of the major organs (liver, spleen, kidney and bone marrow) (Fig. S4D) displayed no statistical differences between the treated and vehicle group. At the end point of the experiment, analysis of mice serum AST/ALT and blood urea nitrogen (BUN) levels (Fig. S4C) did not reveal signs of liver or kidney toxicity in the elaiophylin group compared with the control group.
Discussion
The unique secretory feature of plasma cells makes MM a paradigmatic malignancy for potent strategies that target the secretory apparatus.Citation35 Research in the past decade has highlighted the important role of ER stress and autophagy in the maintenance of intracellular protein homeostasis. In the present study, we have confirmed that elaiophylin inhibited autophagy flux in MM cells and exerted anti-myeloma activity in TP53 mutant MM cells both in vitro and in vivo. We also revealed that ER stress-associated apoptosis plays a significant role in elaiophylin-induced cell death. This is a first report indicating a possible functional linkage between elaiophylin and ER stress-mediated apoptosis. Collectively, our findings suggested that blocking autophagy flux shifted the UPR balance to the alarm phase, resulting in death of TP53 mutant MM cells.
A previous study verified that elaiophylin disrupts autophagy flux by triggering lysosome membrane permeability (LMP) in ovarian cancer.Citation22 Because the lysosome is an evolutionarily conserved organelle in eukaryotic cells, the function of elaiophylin is likely universal. As expected, elaiophylin strongly blocked autophagy flux in MM cell lines, similar to the function in ovarian cancer cells, and led to a cytotoxic response. In the case of myeloma cells, the protein folding capacity of the ER is overloaded, resulting in a lower threshold for drug-induced UPR induction and ER stress-associated apoptosis.Citation14 If any protein handling machinery is tampered with, for example, via PIs or autophagy inhibitors,Citation18 MM cells may be susceptible to cell death.Citation12 Thus, we reasonably inferred that elaiophylin theoretically may induce ER stress-associated apoptosis.
Our findings are in agreement with this hypothesis and indicate that GRP78 gradually released following autophagy inhibition, which is a UPR signal to induce ER stress. PIs are well known to shift the balance to fatal ER stress in cells with a high-level of UPR activity, as indicated by abundant XBP1s.Citation36 Accordingly, upregulation of XBP1s protein, which is a transcription factor activated by ER stress, was detected. As long as the cells fail to adapt to ER stress and restore ER homeostasis, the UPR signal will be switched from an adaptive to a cell death pathway. Many of these maladaptive pro-apoptotic programs are specifically activated by the PERK/ATF4/CHOP pathway.Citation37 Intriguingly, our data indicated prolonged upregulation of phospho-PERK (Thr980), ATF4 and CHOP expression. Combined with apoptosis and cleaved CASP3 activation, which is primarily regulated by the CHOP cascade,Citation38,39 the results suggested robust activation of ER stress-induced apoptosis. To exclude off-target effects, we further performed “rescue” experiments using TUDCA, a chemical agent to abolish ER stress, co-cultured with elaiophylin. Both ER stress (GRP78 and CHOP) and apoptosis (cleaved CASP3 and PARP) markers decreased to some extent. These data strongly suggested that elaiophylin-mediated fatal ER stress induced by switching UPR signaling to a pro-apoptotic state, at least in part, played an obvious role in cell apoptosis via autophagy inhibition in MM cells, including TP53 mutant cells.
Although an accumulating number of studies have sought to identify molecular agents of the cross-talk between autophagy and ER stress, the selection of potential druggable targets in cancer cells that respond to therapeutics is still very limited.Citation21 In fact, there is an increasing list of inhibitors that block autophagy flux in combination with ER stress stimulation as a potential strategy for eliciting apoptosis.Citation40 Most combinations involve synergistic effects with several surprising exceptions. For example, chloroquine (CHQ) or 3-methyladenine (3-MA) in combination with bortezomib is antagonistic.Citation18,41 These inconsistencies may be attributable to the different stage at which the autophagy inhibitor acts. Notably, we should carefully select the inhibitor type and combination dosage due to complex reciprocal regulation of protein handling pathways in response to drug treatment.Citation40 Therefore, a single multiple function drug has also been of great interest. HSP90 inhibitors, for instance, upregulate other HSPs and activate the UPR.Citation42-44 However, HSP90 inhibitors have poor activity in clinical treatment of MM.Citation45 In terms of therapeutic outcome, drugs capable of inducing the pro-apoptotic branch of the UPR as well as simultaneously inhibiting its pro-survival function should maximize the therapeutic benefit.Citation21 To clear the accumulation of misfolded protein aggregates from the ER, the UPR may upregulate autophagy machinery,Citation46,47 which is mainly activated to protect against cell death.Citation15 Bafilomycin A1 (Baf) with bortezomib, an activator of the UPR,Citation14 exerted synergistic anti-myeloma activity.In addition, 3-MA worked synergistically with thapsigargin to increase the apoptosis response.Citation48 Elaiophylin, as a single agent, plays dual roles in blocking autophagy flux and triggering ER stress. The autophagy inhibition activity of elaiophylin could theoretically protract activation of the UPR until a critical threshold is surpassed, leading to a pro-apoptotic result.
Another novel aspect of this study is the use of a well-established MM zebrafish embryo xenograft model first reported by Munshi NC et al. in evaluating drug efficacy.Citation34 This easy, quick and economical model should be further evaluated as a tool to screen and predict drug sensitivity in MM. The elaiophylin dosage that could efficiently reduce the mortality of MM zebrafish embryos is non-toxic to healthy counterparts, at least in the short term, consistent with previous evidence that elaiophylin is relative safe and has anti-ovarian cancer activity in vivo.Citation22 This suggested that elaiophylin may also be a promising anti-myeloma drug candidate with low cytotoxicity. These data were further confirmed with an in vivo xenograft mouse model in our study (, Fig. S4). Moreover, the results provided evidence that this zebrafish embryo xenograft model can support MM cell growth may provide a unique microenvironment. Even so, it may not possess all the characteristics of the real bone marrow microenvironment, such as osteocytes. Increasing evidence underscores that MM cells take part in activating autophagic osteocyte death. Deregulation of osteocytes, in turn, leads to osteolytic bone disease (OBD), which serves as a frequent complication of MM and promotes the progression of disease. The 5T33MM murine model, one of the best mammalian animal models suitable for investigating both malignant cell biology and unique bone lesion diseases,Citation49 is possible to sketch a panorama of the effect of elaiophylin on MM in vivo and therefore provide more relevant and credible preclinical data.
To our knowledge, this is the first report that elaiophylin possesses cytotoxicity toward MM. In addition, this drug not only exerts blockade effects on autophagy flux but also activates ER stress-induced apoptosis, which at least in part is responsible for the anti-myeloma effect of elaiophylin. MM cells are heavily reliant on intracellular protein homeostasis for survival. Compared with other cells, MM cells have a higher UPR baseline, which means it is much easier to surpass the threshold of the survival-to-fatal phase in these cells. Disruption of this process is equivalent to targeting the Achille's heel of MM.Citation50 Importantly, this approach is a lethal backup pathway in cancer cells with defective apoptotic signaling.Citation51,52 In conclusion, elaiophylin may provide a novel strategy to overcome MM with defective p53 pathway and likely other PI-resistant MM sub-clones.
Materials and methods
Cell lines and culture
The HMCLs, U266, RPMI8226, KMS11 and H929 were purchased from the American Type Culture Collection (ATCC, Rockville, MD, USA) and cultured in RPMI-1640 (HyClone) containing 2 mM L-glutamine and 10% (v/v) fetal bovine serum (Gibco). All cells were cultured in a humidified incubator at 37°C containing 5% CO2.
Reagents and antibodies
Elaiophylin was provided by the North China Pharmaceutical Group Corporation New Drug R&D center (Hebei, China). Tauroursodeoxycholic acid (TUDCA; purity≥95%) was purchased from Sigma-Aldrich (St Louis, MO, USA). Bortezomib (BZ; purity>99%) was from Active Biochem (Maplewood, NJ). Tunicamycin (Tm; #12819) was obtained from Cell Signaling Technology. Primary antibodies against MAP1LC3B (#3868), PARP (#9542) and pThr980-PERK (#3179S) were from Cell Signaling Technology. Anti-GAPDH (10494–1-AP), anti-ACTIN (60008–1-Ig), anti-ATF4 (10835–1-AP) and anti-XBP1s (24868–1-AP) were purchased from Proteintech. SQSTM1/p62 Lck ligand (#610832) was purchased from BD Biosciences. Antibodies specific for GRP78 (ab108615), CHOP (ab11419), and cleaved CASP3 (ab32042) were obtained from Abcam. HRP- and Alex Flour 488-conjugated secondary antibodies were purchased from AntGene.
CCK8 assay for cell viability detection
Cell viability in vitro was determined with a Cell Counting Kit-8 assay (CCK-8) (Dojindo, Mashikimachi, Japan) according to the manufacturer's instructions. Briefly, cells (1.5 × 104 cells per well) were seeded into 96-well plates in a final volume of 100 μl of complete culture medium with the indicated drug concentrations (0∼1.5 μM) and time intervals (0, 24 and 48 h). The number of viable cells was measured as a percentage of the optical density (OD) value of the indicated treatment samples to DMSO control samples at a wavelength of 450 nm using an Absorbance Microplate Reader (SpectraMax® 190, California, USA).
Colony formation assay
Cells were plated in a 24-well plate at a density of 400∼600 cells per well with 1 ml of fresh complete RPMI-1640 culture medium containing 1% methylcellulose and 1.0 μM elaiophylin. After 14 d of treatment, the colonies in each well were counted under an inverted phase-contrast microscope (Olympus, Japan).
Apoptosis analysis
For apoptosis detection, cells were harvested and stained using a propidium iodide and Annexin V-FITC Apoptosis Detection kit (BD Pharmingen™, San Jose, CA, USA) following the manufacturer's protocol. All samples were measured with a flow cytometer (BD FACSCalibur, Becton Dickinson, USA) and analyzed using FlowJo (TreeStar) software.
Edu cell proliferation assay
To evaluate cell proliferation, a Click-iT™ Plus Edu flow cytometry (Invitrogen, USA) assay was performed. Cells (3 × 105 in 2 ml of complete culture medium per well) were seeded in 12-well plates and treated with elaiophylin (0, 0.5 and 1.0 μM) for 24 h. According to the protocol recommendation, Click-iT™ Edu was added at a final concentration of 10 μM for 4 h before cell harvest. Thereafter, collected cells were washed with 1 ml of PBS containing 1% (m/v) BSA and then fixed with 100 μl of Click-iT™ fixative for 15 min. Cells were washed again, resuspended in 50 μl of 1 × Click-iT™ saponin-based permeabilization and wash reagent for another 15 min incubation. For click labeling, cells were mixed with 500 μl of Click-iT™ Plus reaction cocktail for 30 min and finally washed and resuspended with a suitable volume of PBS containing 1% (m/v) BSA. For the detection of Edu with Alexa Fluor™ 488 picolyl azide, 488 nm excitation and a green emission filter were used with a BD FACSCalibur flow cytometer (San Jose, CA, USA). During all procedures, samples were protected from light.
Caspase-3 (CASP3) activity assay
Cells were cultured with the indicated drug concentration in a 10-cm dish at a density of 1.5 × 105/ml. CASP3 activity was evaluated using a caspase-3 activity kit (Beyotime, China) assay. Following the manufacturer's instructions, treated cells were lysed with lysis buffer (2 × 106 cells/100 μl buffer) for 15 min on ice followed by washing with ice-cold PBS. Then, 10 μl of cell lysate, 80 μl of reaction buffer and 10 μl of 2 mM CASP3 substrate were mixed and incubated in 96-well microtiter plates at 37°C for 4 h. CASP3 activity was quantified with an Absorbance Microplate Reader (SpectraMax® 190, California, USA) at a wavelength of 405 nm. CASP3 activity is presented as the fold change relative to the enzyme activity of the control.
Quantitative real-time PCR (qRT-PCR)
Total RNA was extracted using TRIzol reagent (Ambion, USA). RNA quantification and mRNA integrity were tested with a NanoDrop micro-volume spectrophotometer (Thermo Fisher, USA) and agarose gel electrophoresis. Reverse transcription was performed using 2 μg of total RNA in a ThermoScript RT-PCR System (Invitrogen, USA). For semi-quantification of genes, we used All-in-One™ qPCR Mix (GeneCopoeia, USA) in a total volume of 20 μl. Then, qRT-PCR was performed in a CFX96 Touch™ Real-Time PCR Detection System (BioRad, USA) according to the following thermocycler program: pre-incubation 5 min at 95°Cfollowed by 35 cycles of 15 s at 95°C, 15 s at 60°C, and 30 s at 72°C. Amplicons of GAPDH, GRP78, CHOP, ATF4 and GADD34 were amplified with primers (Table S1). Relative gene expression was calculated using the 2-ΔΔCt method. The expression of genes of interest was normalized to GAPDH.
Immunofluorescence (IF) staining
For the detection of the endogenous formation of autophagosomes, we performed an immunofluorescence staining assay. After treatment with different elaiophylin concentrations for 24 h, cells were immediately attached to glass slides using cytospin, fixed with 4% paraformaldehyde and permeabilized with 0.2% Triton X-100. After being blocked at room temperature with 5% BSA for 15 min, the cells were incubated with primary anti-MAP1LC3B-II antibody (1:50) at 4°C overnight. The next day, cells were washed with PBS and stained with Alex Flour 488-conjugated secondary antibody (1:200) at 37°C for 1 h. Nuclei were stained with 4′,6-diamidino-2-phenylindole (DAPI, Molecular Probes/Invitrogen, USA). All the images were captured using a fluorescence microscope (Olympus DP73, Tokyo, Japan).
Western blot analysis
Elaiophylin-treated cells were harvested and lysed in RIPA buffer (Beyotime, China) containing fresh protease inhibitor cocktail and PMSF (Roche, USA) for 30 min. After ultrasound pyrolysis, samples were centrifuged at 12000 rpm in 4°C for 20 min. Supernatants were collected, and the total protein concentration was determined using a Bradford assay kit (Thermo Scientific, USA) according to the manufacturer's instruction. Cell lysates (20 μg) were separated in a 12% SDS polyacrylamide gel via electrophoresis and transferred onto a PVDF membrane (Millipore, MA) using a Trans-Blot Turbo ™ Transfer System (BioRad, USA). To mitigate nonspecific binding, membranes were soaked in 5% skim milk for 1 h and incubated overnight at 4°C with specific primary antibodies. The blots were incubated with HRP-conjugated secondary antibodies at room temperature for 1 h on the next day and were washed with TBS containing 0.2% Tween (TBS-T). Protein bands were revealed with a Western Blotting Detection kit (Advansta, WesternBright™ ECL, USA). Images were visualized with a Molecular Imager ChemiDoc™ XRS+ (BioRad, USA).
Tandem mRFP-GFP-LC3 fluorescence microscopy
Tandem monomeric RFP-GFP-LC3 (tfLC3) was used to monitor autophagy flux as described previously. Briefly, U266 cells were transduced using a lentiviral vector encoding tfLC3. To evaluate tandem fluorescent LC3 puncta, at least 48 h after tfLC3 transfection, cells were washed with fresh culture medium, and rapamycin (250 nM), CQ (20 μM) and elaiophylin (0.75 μM) were added. Approximately 12 h later, the cells were then sent out for fluorescence microscopy analysis (Olympus DP73, Tokyo, Japan).
Immunohistochemistry
All xenograft tumors were surgically isolated from each mouse and fixed in formalin, embedded in paraffin and sectioned for further study. Reaction conditions for the primary anti-cleaved CASP3 antibody, a suitable HRP-labeled secondary antibody and DAB as the chromagen, were first optimized with positive control slides. Subsequently, histologic micrographs were captured with a Zeiss KS400 image system (Jena, Germany), and 8 randomized fields of each stained section were selected for quantitative analysis. Cells with strong or moderate brown staining were counted as positive for cleaved CASP3. All assessments were performed by 3 independent observers.
Maintenance of zebrafish
Zebrafish (Danio rerio) were raised under a 14 h light/10 h dark cycle. From 24–36 h post fertilization (hpf), the dechorionated embryos were soaked in E3 embryo medium containing 0.2 mM 1-phenyl-2-thiourea (PTU, Sigma-Aldrich, P7629) at 28.5°C for subsequent experiments.
Establishment of an in vivo xenograft zebrafish model with a MM cell line
A xenograft zebrafish MM model was established as described previously.Citation24 Briefly, CM-DIL (C7000, Invitrogen, USA) labeled U266 cells were washed in PBS, resuspended in Matrigel (Cultrex Basement Membrane Extract, R&D Systems) with 8000 cells/μl, and microinjected (approximately 5–10 nl cell suspension) into the perivitelline space of 48 hpf embryos at a proper pressure and time using a pneumatic Pico-pump injector (C-13509, Harvard Apparatus). Injection of the same volume of Matrigel without cells was used as a mock control. Tumor xenograft volume was estimated by measurement of the fluorescence density using ImageJ software.
In vivo treatment
Female NOD/SCID mice (4 to 6 weeks old) were subcutaneously injected with U266 cells (1 × 107) in 100 μl of PBS mixed with an equal volume of Matrigel basement membrane matrix (BD, USA). When mice exhibited palpable approximately 100–130 mm3 tumors, they were randomly divided into 2 cohorts that received elaiophylin (2 mg/kg, i.p., every 2 days, a total of 10 doses) or DMSO as previously reported.Citation22 Tumor volume was estimated every 4 d for the duration of treatment using a standard formula (length × width2 × 0.5). Tumors were finally harvested to measure the weight and fixed in formalin at the experimental end point. Photographs of the tumors were also taken.
Female C57BL/6 mice (4 to 6 weeks) were randomly assigned into an elaiophylin or control group (n = 5 each group). Mice in each group were given an intraperitoneal injection of elaiophylin or DMSO at the same dosage mentioned above. Body weight and a peripheral blood cell count were examined for each mouse at the indicated time points. At the end point of the experiment, all the mice were humanely killed to exam the ALT, AST, BUN and pathological morphology of some organs. All the mice were purchased from HFK (Beijing, China) and housed under specific pathogen-free (SPF) conditions, in compliance with the Guide for the Care and Use of Laboratory Animals of Tongji Hospital.
Statistical analysis
The continuous data are presented as the means ± standard deviation (SD) of at least 3 independent experiments. A 2-tailed Student's t-test for paired groups and one-way analysis of variance (ANOVA) followed by Newman-Keuls test for multi-group comparisons were used to determine significant differences using GraphPad software version 6.0 (GraphPad Software, San Diego, CA, USA). In all cases, P < 0.05 was considered statistically significant. *P < 0.05, **P < 0.01, and ***P < 0.001.
Disclosure of potential conflicts of interest
No potential conflicts of interest were disclosed.
Supplemental_Materials.doc
Download MS Word (1.5 MB)Funding
This study was supported by grants from the Key Program of the National Natural Science Foundation of China (no.81630006) and the General Program of the National Natural Science Foundation of China (no. 81570196).
References
- Siegel R, Naishadham D, Jemal A. Cancer statistics, 2013. CA Cancer J Clin 2013; 63:11-30; PMID:23335087; https://doi.org/10.3322/caac.21166
- Usmani SZ, Crowley J, Hoering A, Mitchell A, Waheed S, Nair B, AlSayed Y, Vanrhee F, Barlogie B. Improvement in long-term outcomes with successive Total Therapy trials for multiple myeloma: are patients now being cured? Leukemia 2013; 27(1):226-32; PMID:22705990; https://doi.org/10.1038/leu.2012.160
- Adams J. Proteasome inhibition in cancer: Development of PS-341. Semin Oncol 2001; 28(6):613-9; PMID:11740819
- Jung L, Holle L, Dalton WS. Discovery, Development, and clinical applications of bortezomib. Oncology 2004; 18(14 Suppl 11):4-13; PMID:15688597
- Moreau P, Richardson PG, Cavo M, Orlowski RZ, San Miguel JF, Palumbo A, Harousseau JL. Proteasome inhibitors in multiple myeloma: 10 years later. Blood 2012; 120(5):947-59; PMID:22645181; https://doi.org/10.1182/blood-2012-04-403733
- Mateos MV. Management of treatment-related adverse events in patients with multiple myeloma. Cancer Treat Rev 2010; 36(Suppl 2):S24-32; PMID:20472185; https://doi.org/10.1016/S0305-7372(10)70009-8
- Vogelstein B, Lane D, Levine AJ. Surfing the p53 network. Nature 2000; 408:307-10; PMID:11099028; https://doi.org/10.1038/35042675
- Saha MN, Jiang H, Jayakar J, Reece D, Branch DR, Chang H. MDM2 antagonist nutlin plus proteasome inhibitor velcade combination displays a synergistic anti-myeloma activity. Cancer Biol Ther 2010; 9:936-44; PMID:20418664; https://doi.org/10.4161/cbt.9.11.11882
- Drach J, Ackermann J, Fritz E, Kromer E, Schuster R, Gisslinger H, DeSantis M, Zojer N, Fiegl M, Roka S, et al. Presence of a p53 gene deletion in patients with multiple myeloma predicts for short survival after conventional-dose chemotherapy. Blood 1998; 92:802-9; PMID:9680348
- Vousden KH, Lu X. Live or let die: the cell's response to p53. Nat Rev Cancer 2002; 2:594-604; PMID:12154352; https://doi.org/10.1038/nrc864
- Chng WJ, Price-Troska T, Gonzalez-Paz N, Van Wier S, Jacobus S, Blood E, Henderson K, Oken M, Van Ness B, Greipp P, et al. Clinical significance of TP53 mutation in myeloma. Leukemia 2007; 21(3):582-4; PMID:17215851; https://doi.org/10.1038/sj.leu.2404524
- Tiedemann RE, Gonzalez-Paz N, Kyle RA, Santana-Davila R, Price-Troska T, Van Wier SA, Chng WJ, Ketterling RP, Gertz MA, Henderson K, et al. Genetic aberrations and survival in plasma cell leukemia. Leukemia 2008; 22(5):1044-52; PMID:18216867; https://doi.org/10.1038/leu.2008.4
- Teoh PJ, Chung TH, Sebastian S, Choo SN, Yan J, Ng SB, Fonseca R, Chng WJ. p53 haploinsufficiency and functional abnormalities in multiple myeloma. Leukemia 2014; 28(10):2066-74; PMID:24625551; https://doi.org/10.1038/leu.2014.102
- Auner HW, Cenci S. Recent advances and future directions in targeting the secretory apparatus in multiple myeloma. Br J Haematol 2015; 168:14-25; PMID:25296649; https://doi.org/10.1111/bjh.13172
- Mizushima N, Levine B, Cuervo AM. Klionsky DJ Autophagy fights disease through cellular self-digestion. Nature 2008; 451(7182):1069-75; PMID:18305538; https://doi.org/10.1038/nature06639
- Levine B, Kroemer G. Autophagy in the pathogenesis of disease. Cell 2008; 132:27-42; PMID:18191218; https://doi.org/10.1016/j.cell.2007.12.018
- Aronson LI, Davenport EL, Giuntoli SG, Srikanth M, Smith E, Morgan GJ, Davies F. Autophagy is a key myeloma survival pathway that can be manipulated therapeutically to enhance apoptosis. Blood 2010;116(21):4083; https://www.bloodjournal.org/content/116/21/4083
- Hoang B, Benavides A, Shi Y, Frost P, Lichtenstein A. Effect of autophagy on multiple myeloma cell viability. Mol Cancer Ther 2009; 8(7):1974-84; PMID:19509276; https://doi.org/10.1158/1535-7163.MCT-08-1177
- Carroll RG, Martin SJ. Autophagy in multiple myeloma: what makes you stronger can also kill you. Cancer cell 2013; 23(4):425-6; PMID:23597558; https://doi.org/10.1016/j.ccr.2013.04.001
- Gorlach A, Klappa P, Kietzmann T. The endoplasmic reticulum: folding, calcium homeostasis, signaling, and redox control. Antiox Redox Signal 2006; 8:1391-418; PMID:16986999; https://doi.org/10.1089/ars.2006.8.1391
- Verfaillie T, Salazar M, Velasco G, Agostinis P. Linking ER stress to autophagy: Potential implications for cancer therapy. Int J Cell Biol 2010; 2010:930509; PMID:20145727; https://doi.org/10.1155/2010/930509
- Zhao X, Fang Y, Yang Y, Qin Y, Wu P, Wang T, Lai H, Meng L, Wang D, Zheng Z, et al. Elaiophylin, a novel autophagy inhibitor, exerts antitumor activity as a single agent in ovarian cancer cells. Autophagy 2015; 11(10):1849-63; PMID:25893854; https://doi.org/10.1080/15548627.2015.1017185
- Arai M. Azalomycins B and F, two new antibiotics. I. Production and isolation. J Antibiot 1960; 13: 46-50; PMID:13848830
- Bianchi G, Oliva L, Cascio P, Pengo N, Fontana F, Cerruti F, Orsi A, Pasqualetto E, Mezghrani A, Calbi V, et al. The proteasome load versus capacity balance determines apoptotic sensitivity of multiple myeloma cells to proteasome inhibition. Blood 2009; 113:3040-9; PMID:19164601; https://doi.org/10.1182/blood-2008-08-172734
- Mizushima N, Klionsky DJ. Protein turnover via autophagy: Implications for metabolism. Ann Rev Nutr 2007; 27:19-40; PMID:17311494; https://doi.org/10.1146/annurev.nutr.27.061406.093749
- Deretic V, Saitoh T, Akira S. Autophagy in infection, inflammation and immunity. Nat Rev Immunol 2013; 13:722-37; PMID:24064518; https://doi.org/10.1038/nri3532
- Klionsky DJ, Abdalla FC, Abeliovich H, Abraham RT, Acevedo-Arozena A, Adeli K, Agholme L, Agnello M, Agostinis P, Aguirre-Ghiso JA, et al. Guidelines for the use and interpretation of assays for monitoring autophagy. Autophagy 2012; 8:445-544; PMID:22966490; https://doi.org/10.4161/auto.19496
- Pankiv S, Clausen TH, Lamark T, Brech A, Bruun JA, Outzen H, Øvervatn A, Bjørkøy G, Johansen T. p62/SQSTM1 binds directly to Atg8/LC3 to facilitate degradation of ubiquitinated protein aggregates by autophagy. J Biol Chem 2007; 282:24131-45; PMID:17580304; https://doi.org/10.1074/jbc.M702824200
- Moretti L, Cha YI, Niermann KJ, Lu B. Switch between apoptosis and autophagy: radiation-induced endoplasmic reticulum stress? Cell cycle 2007; 6:793-8; PMID:17377498; https://doi.org/10.4161/cc.6.7.4036
- Codogno P, Meijer AJ. Autophagy and signaling: their role in cell survival and cell death. Cell Death Differ 2005; 12(Suppl 2):1509-18; PMID:16247498; https://doi.org/10.1038/sj.cdd.4401751
- Bernales S, McDonald KL, Walter P. Autophagy counterbalances endoplasmic reticulum expansion during the unfolded protein response. PLoS Biol 2006; 4:e423; PMID:17132049; https://doi.org/10.1371/journal.pbio.0040423
- Ozcan U, Yilmaz E, Ozcan L, Furuhashi M, Vaillancourt E, Smith RO, Görgün CZ, Hotamisligil GS. Chemical chaperones reduce ER stress and restore glucose homeostasis in a mouse model of type 2 diabetes. Science 2006; 313:1137-40; PMID:16931765; https://doi.org/10.1126/science.1128294
- Vandewynckel YP, Laukens D, Devisscher L, Paridaens A, Bogaerts E, Verhelst X, Van den Bussche A, Raevens S, Van Steenkiste C, Van Troys M, et al. Tauroursodeoxycholic acid dampens oncogenic apoptosis induced by endoplasmic reticulum stress during hepatocarcinogen exposure. Oncotarget 2015; 6:28011-25; PMID:26293671; https://doi.org/10.18632/oncotarget.4377
- Lin J, Zhang W, Zhao JJ, Kwart AH, Yang C, Ma D, Ren X, Tai YT, Anderson KC, Handin RI, et al. A clinically relevant in vivo zebrafish model of human multiple myeloma to study preclinical therapeutic efficacy. Blood 2016; 128:249-52; PMID:27207793; https://doi.org/10.1182/blood-2016-03-704460
- Clarke HJ, Chambers JE, Liniker E, Marciniak SJ. Endoplasmic reticulum stress in malignancy. Cancer cell 2014; 25:563-73; PMID:24823636; https://doi.org/10.1016/j.ccr.2014.03.015
- Obeng EA, Carlson LM, Gutman DM, Harrington WJ, Jr., Lee KP, Boise LH. Proteasome inhibitors induce a terminal unfolded protein response in multiple myeloma cells. Blood 2006; 107:4907-16; PMID:16507771; https://doi.org/10.1182/blood-2005-08-3531
- Hiramatsu N, Chiang WC, Kurt TD, Sigurdson CJ, Lin JH. Multiple mechanisms of unfolded protein response-induced cell death. Am J Pathol 2015; 185:1800-8; PMID:25956028; https://doi.org/10.1016/j.ajpath.2015.03.009
- Walter P, Ron D. The unfolded protein response: From stress pathway to homeostatic regulation. Science 2011; 334:1081-6; PMID:22116877; https://doi.org/10.1126/science.1209038
- Xie Q, Khaoustov VI, Chung CC, Sohn J, Krishnan B, Lewis DE, Yoffe B. Effect of tauroursodeoxycholic acid on endoplasmic reticulum stress-induced caspase-12 activation. Hepatology 2002; 36:592-601; PMID:12198651; https://doi.org/10.1053/jhep.2002.35441
- Aronson LI, Davies FE. DangER: Protein ovERload. Targeting protein degradation to treat myeloma. Haematologica 2012; 97:1119-30; PMID:22580998; https://doi.org/10.3324/haematol.2012.064923
- Kawaguchi T, Miyazawa K, Moriya S, Ohtomo T, Che XF, Naito M, Itoh M, Tomoda A. Combined treatment with bortezomib plus bafilomycin A1 enhances the cytocidal effect and induces endoplasmic reticulum stress in U266 myeloma cells: crosstalk among proteasome, autophagy-lysosome and ER stress. Int J Oncol 2011; 38:643-54; PMID:21174067; https://doi.org/10.3892/ijo.2010.882
- Davenport EL, Moore HE, Dunlop AS, Sharp SY, Workman P, Morgan GJ, Davies FE. Heat shock protein inhibition is associated with activation of the unfolded protein response pathway in myeloma plasma cells. Blood 2007; 110:2641-9; PMID:17525289; https://doi.org/10.1182/blood-2006-11-053728
- Mitsiades CS, Mitsiades NS, McMullan CJ, Poulaki V, Kung AL, Davies FE, Morgan G, Akiyama M, Shringarpure R, Munshi NC, et al. Antimyeloma activity of heat shock protein-90 inhibition. Blood 2006; 107:1092-100; PMID:16234364; https://doi.org/10.1182/blood-2006-11-053728
- Davenport EL, Zeisig A, Aronson LI, Moore HE, Hockley S, Gonzalez D, Smith EM, Powers MV, Sharp SY, Workman P, et al. Targeting heat shock protein 72 enhances Hsp90 inhibitor-induced apoptosis in myeloma. Leukemia 2010; 24:1804-7; PMID:20703255; https://doi.org/10.1038/leu.2010.168
- Richardson PG, Mitsiades CS, Laubach JP, Lonial S, Chanan-Khan AA, Anderson KC. Inhibition of heat shock protein 90 (HSP90) as a therapeutic strategy for the treatment of myeloma and other cancers. Br J Haematol 2011; 152:367-79; PMID:21219297; https://doi.org/10.1111/j.1365-2141.2010.08360.x
- Kouroku Y, Fujita E, Tanida I, Ueno T, Isoai A, Kumagai H, Ogawa S, Kaufman RJ, Kominami E, Momoi T. ER stress (PERK/eIF2alpha phosphorylation) mediates the polyglutamine-induced LC3 conversion, an essential step for autophagy formation. Cell Death Differ 2007; 14:230-9; PMID:16794605; https://doi.org/10.1038/sj.cdd.4401984
- Ogata M, Hino S, Saito A, Morikawa K, Kondo S, Kanemoto S, Murakami T, Taniguchi M, Tanii I, Yoshinaga K, et al. Autophagy is activated for cell survival after endoplasmic reticulum stress. Mol Cell Biol 2006; 26:9220-31; PMID:17030611; https://doi.org/10.1128/MCB.01453-06
- Michallet AS, Mondiere P, Taillardet M, Leverrier Y, Genestier L, Defrance T. Compromising the unfolded protein response induces autophagy-mediated cell death in multiple myeloma cells. PloS one 2011; 6:e25820; PMID:22028791; https://doi.org/10.1371/journal.pone.0025820
- Lwin ST, Edwards CM, Silbermann R. Preclinical animal models of multiple myeloma. BoneKEy Rep 2016; 5:772; PMID:26909147; https://doi.org/10.1038/bonekey.2015.142
- Vincenz L, Jager R, O'Dwyer M, Samali A. Endoplasmic reticulum stress and the unfolded protein response: targeting the Achilles heel of multiple myeloma. Mol Cancer Ther 2013; 12:831-43; PMID:23729400; https://doi.org/10.1158/1535-7163.MCT-12-0782
- Eisenberg-Lerner A, Bialik S, Simon HU, Kimchi A. Life and death partners: apoptosis, autophagy and the cross-talk between them. Cell Death Differ 2009; 16:966-75; PMID:19325568; https://doi.org/10.1038/cdd.2009.33
- Maiuri MC, Zalckvar E, Kimchi A, Kroemer G. Self-eating and self-killing: crosstalk between autophagy and apoptosis. Nat Rev Mol Cell Biol 2007; 8:741-52; PMID:17717517; https://doi.org/10.1038/nrm2239