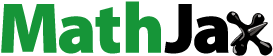
ABSTRACT
Ovarian cancer ranks fifth in cancer related deaths for women in USA. The high mortality rate associated with ovarian cancer is due to diagnosis at later stages of disease and the high recurrence rate of 60–80%. Recurrent ovarian cancers are more likely to present as multidrug resistance (MDR) leading to unfavorable response from 2nd and 3rd line chemotherapy. Nanoemulsions (NEs) are emerging as an attractive drug delivery system to overcome MDR challenges. NEs can also minimize exposure of therapeutic cargo to normal tissues potentially reducing side effects. In >80% of ovarian cancers, Folate Receptor-α (FR-α) is expressed at 10- to 100-fold higher levels than on non-pathological tissues. Therefore, folate (FA) is being evaluated as an active targeting moiety for FR-α+ ovarian cancer. To improve therapeutic outcome with reduced toxicity, we developed NMI-500, a FA targeted gadolinium (Gd) annotated NE loaded with docetaxel (DTX). NMI-500 has been developed as theranostic agents as Gd will enable physician to acquire real time pharmacodynamics data on NE + DTX accumulation in target lesions. In present study, characterization for key translational metrics of NMI-500 showed size distribution in range of 120 to 150 nm and zeta potential around −45 mV. Active targeting of FA was evaluated against FR-α+ KB cells and results demonstrated significant improvement in cell association which was surface ligand density dependent. We found that NMI-500 was able to inhibit tumor growth in a spontaneous transgenic ovarian cancer model with improved safety profile and this growth inhibition could be longitudinally followed by MRI. These results indicate NMI-500 warrants advancement to clinical trials.
Introduction
Ovarian cancer is an insidious disease with approximately 22,000 new cases per year and ∼ 14,000 deaths annually.Citation1 These statistics have been long standing even while therapeutic breakthroughs in other cancers, such as multiple myeloma, myelodysplastic syndromes, breast and others, have brought about significant decreases in mortality over the last two decades.Citation1,2 Ovarian cancer is most often (∼60% of cases) diagnosed in late stages (III – IV) with ∼20% of these patients presenting as drug resistant following a first course of carboplatin/paclitaxel. In addition, there is a high rate of recurrence and development of drug resistance among the patients who initially respond well to standard chemotherapy. The care of these patients could drastically improve if a therapy existed that could be specifically targeted to tumor cells and non-invasively monitored to ensure tumors were being inundated with life-saving chemotherapy. If such a therapy was not working, and no signal is observed in the tumor, this information could provide physicians valuable time to change therapies potentially producing better patient outcomes. The tools for this change in clinical paradigm exist today.
To ultimately change care for patients presenting with advanced ovarian cancer, new strategies have to be developed. One potentially effective strategy is to combine therapeutic and diagnostic into a single modality designated a theranostic. To accomplish this goal, a suitable platform is needed to be selected to carry a therapeutic payload and act as a diagnostic that specifically targets ovarian cancer cells. We have developed a highly flexible stable oil in water nanoemulsion (NE) platform that fits all the required attributes for the clinical development of a theranostic approach suitable for treating ovarian cancer patients.Citation3–5 Using this NE platform, we designed and created stable NMI-500, a folate (FA) targeted theranostic that has the capacity to: carry a lethal payload of docetaxel (DTX); overcome ATP-binding cassette (ABC) gene family mediated drug efflux;Citation6–11 specifically interact with folate receptor-α (FR-α) which is commonly expressed on ovarian tumor cells;Citation11,12 and act as an MRI contrast agent so DTX pharmacodynamics and disease burden can be tracked by a non-invasive, clinically relevant imaging modality (Fig. S1).Citation4,5,13
FR-α is one of three isoforms of the folate receptor: α, β, & γ. FR-α is a 38 kD glycosyl-phosphatidylinositol-anchored glycoprotein that binds FA with a Kd<1 nM, ∼10-fold more affinity than the reduced form of FA and is highly expressed in a number of human cancers including ovarian, lung, breast, renal cell carcinoma, brain, and head & neck cancers.Citation14 FR-α mediates FA uptake through endocytosis, showing very high affinity but low capacity. An important aspect for FR-α targeting is that cargos transported by this endocytotic mechanism are delivered intracellularly in a nondestructive manner.Citation15 Since the macromolecule remains intact following endocytosis it can fully exert its biological function inside the cell (). After endocytosis, FR is recycled, returning to the cell surface to bind to more FA. Importantly, FA valency on the surface of nanoparticles has been shown not to impact the rate of FR-α internalization which means the number of FA on the surface of a nanoparticle can be increased to the desired level without detrimentally impacting either the FR-α on the surface of the cell or the receptor recycle rate.Citation16 Clinically this is important as there should be no implications on the dosage schedule for FA-targeted therapies as their ability to bind to tumors will not be detrimentally impacted by FR-α turnover or recycling times.Citation16 The biological properties of the endocytosis FA pathway and expression patterns associated with stage of ovarian cancer make FR-α attractive for the development of theranostics. NMI-500 takes advantage of these unique endocytotic properties to deliver DTX past cell surface resistance mechanisms like the ABC-gene family transporters which are known to pump out many hydrophobic chemotherapies.Citation11
Figure 1. Schematic representation of receptor mediated endocytosis of NMI-500 and mechanism of docetaxel cellular uptake and efflux responsible for MDR.
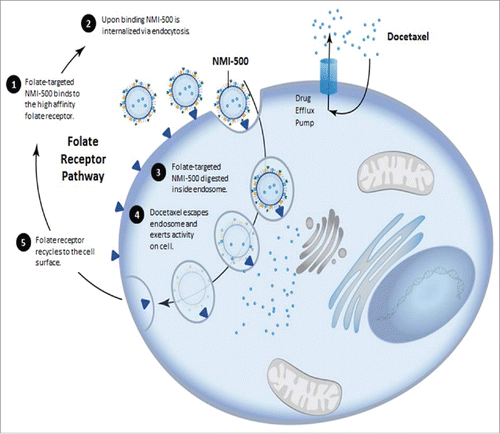
Herein we articulate the in vitro and in vivo data supporting NMI-500′s development as a potential theranostic tool for treatment of ovarian cancer. In vitro we show NMI-500 has broad cytotoxic potential against the NCI 60 cancer cell line panel and continue to prove its ability to overcome P-glycoprotein (P-gp) mediated resistance facilitated by FA targeting ligand on the surface of NMI-500. We and others showed that receptor mediated endocytosis can be used to overcome drug resistance mechanisms associated with drug influx/efflux transport issues at the cell surface.Citation6–11 We also showed that receptor mediated endocytosis of targeted NE annotated with MRI contrast agents results in enhanced specificity and accumulation in subcutaneous flank tumors more effectively than non-targeted NE.Citation5,11 This tumor accumulation can occur by either first or second order kinetics depending upon the nature of targeting ligand and the relative expression level of the cognate receptor on target and non-target tissue.Citation5,11 We demonstrated that having multiple ligands on a surface, NE changes the cognate receptor interactions from one driven by affinity to one governed by avidity, a much stronger interaction. Therefore NMI-500 has the potential to interact with tumors expressing even low levels of FR-α.Citation12 This specificity opens a significant opportunity to use MRI signal as a surrogate pharmacodynamics marker for drug accumulation. MRI T1 signal intensity is linearly correlated with the Gd concentration on the surface of the NE. Therefore, knowing this relationship potentiates the ability to correlate drug payload delivered to MRI diagnostic signal detected.Citation17,18
Male mice stably expressing TgMISIIR-Tag-DR26 that are crossed with wild type C57BL/6 females produce female offspring that develop bilateral ovarian carcinoma with 100% penetrance.Citation19 The morphology of these ovarian tumors is similar to human serous epithelial ovarian cancers. More importantly necropsy consistently reveals bilateral ovarian masses and extensive dissemination of the tumor, with invasion of the omentum, mesentery, and peritoneal and visceral serosa.Citation18 This model has been used previously to track disease progression longitudinally with MRI when female mice were treated with the m-TOR inhibitor Everolimus.Citation20 Using this transgenic model a 30 month long preclinical trial was conducted to assess NMI-500′s effect on spontaneous epithelial ovarian cancer. Once female mice were confirmed to have developed bilateral epithelial ovarian cancer, treatment was initiated and disease progression was followed by MRI. Interestingly, FR-α expression was confirmed by IHC but expression levels were not correlative with NMI-500 treatment outcomes. Our preclinical investigation suggests NMI-500, a FA targeted, Gd annotated, DTX carrying theranostic NE may offer a novel and promising approach for the treatment of patients who present with advanced ovarian cancer.
Results
Synthesis, preparation and characterizationof nanoemulsions (NE)
With the goal of effectively targeting FR-α that is overexpressed by many types of cancers, we labeled the surface of our NE with FA in the reduced form. To reduce the potential interactions of neighboring folates, we synthesized a FA-PEG-lipid conjugate that contains additional charged residue in between the PEG and FA moiety.Citation21 The FA-targeted DSPE-PEG2000-Cys-FA was synthesized using carbodiimide chemistry and purified by dialysis. The reaction scheme used resulted in the formation of gamma-carboxyl linked isomer of the FA-conjugate which is predominant product of the reaction.Citation22,23 NMR spectroscopy was used to confirm all the individual components and the final purified conjugate.Citation5 To label NEs with a Gd-chelate on the surface to use in magnetic resonance imaging studies, we synthesized and characterized Gd-labeled lipidated chelate ePE-DTPA-Gd according to our previously published method.Citation5
The optimized theranostic NE formulations consisted of DTX (0.2% w/v), flaxseed oil (20% w/v), egg lecithin (1.2%, w/v), DSPE-PEG2000-Cys-FA, mPEG2000-DSPE (0.3% w/v) and Gd-DTPA-ePE (2% w/v). mPEG2000-DSPE was incorporated in the formulation to increase the mean residence time in the blood. NEs were produced using high shear microfluidization process, with processing conditions (25,000 psi for 10 cycles) optimized to obtain NEs with particle size ∼150 nm. The lipid core of the formulation is flaxseed oil which is rich in omega-3 and omega-6 poly unsaturated fatty acids (PUFA) and can solubilize a significant amount of hydrophobic DTX.
The average particle size of the blank NE was 120–150 nm (). The incorporation of DTX did not significantly change the hydrodynamic particle size and size remained between 120–140 nm. The mPEG2000-DSPE used in the formulations are negatively chargedCitation24 resulting in overall negative zeta potential ranging from −29 to −48 mV (). These results are consistent with the other previously described stable NEs.Citation4,5,11,12 NEs for cellular uptake assay were formulated with Rh-PE integrated into the lipid monolayer of the NEs instead of including DTX in the oil-core. The physical properties of the rhodamine labeled-NEs were not significantly different compared to the non-labeled NEs ().
Table 1. Characterization of NEs.
For in vitro and in vivo studies, the DTX concentration in the NMI-500 NE formulations ranged from 1.8 to 2 mg/ml as determined by HPLC-UV analysis. The high drug encapsulation efficiency (>90%) of DTX in NE is attributable to its hydrophobic nature and an excellent ability of PUFA rich oil core at sequestering hydrophobic drugs. Together, these results demonstrate successful formulation of NEs with consistent particle size, zeta potential and loading efficiency.
FA targeted NEs (selectively) bind to FR-α expressing cells
To evaluate the FR-α targeting efficiency of NMI-500, KB-WT and KB-PR10 cells, that express high levels of FR-α, were used as in vitro models. A2780, SKOV-3, KB-WT and KB-PR10 cell pellets were subjected to immunohistochemical staining. A2780 were FR-α negative whereas SKOV-3 demonstratred patchy and uneven staining rendering it unsuitable model cell line. On the other hand, KB-WT and KB-PR10 both cell lines expressed uniformly high levels of FR-α () due to which they were selected as model of choice. We examined the effect of increasing FA density on the surface of the NE to evaluate the relative avidity for FR-α+ KB/WT and KB-PR10 cells. To evaluate cell association of NE using flow cytometry, NEs were fluorescently labeled using Rh-PE. Rh-PE-NEs targeted with and without FA on the surface were incubated with KB-WT and KB-PR10 cells ( & , respectively) and analyzed by flow cytometry. To test the relative avidity of the FA-NEs, a competitive binding assay was established by pretreating cells with ∼5000-fold higher free FA (1 mM) and comparing to the uptake of Rh-PE containing FA-NEs to cells without added FA competitor. Human physiological plasma concentration of FA are approximately 50 nMCitation25 and the concentration of free FA we used is much higher than would normally be observed in the clinic. Cells were treated with NE containing Rh-PE labeled with 100, 300, 1200 or 3600 FA molecules per particle. In the absence of free FA competitor, we observed a dose dependent increase in uptake of FA-RhNE labeled with 100, 300, 1200 and 3600 FA molecules/NE in KB and KB-PR10 cell lines ( & ). This result indicates that the rate at which the FA-RhNE is internalized is dependent on the number of FA molecules available on the surface. With free FA competition, cells treated with 100 through 3600 molecules/NE were able to overcome the competition to a similar extent, resulting in about ∼50% uptake compared to control. KB-WT and KB-PR10 cells treated with 3600 FA molecules/NE showed significantly higher uptake compared to NT and 100 to 1200 FA molecules/NE either in presence or absence of free FA. We believe this increase could be attributed to higher avidity due to higher density of FA on the NE surface. However, NE with 3600 FA molecules/NE are physically unstable for periods longer than a week and were therefore not pursued for further studies. FA targeted Rh-NEs were able to overcome free FA competition even when free FA were used at concentrations 500 to 5000-fold greater than the concentration of FA present in the NE. We conclude that the increase in uptake on KB-WT and KB-PR10 cells is due to the enhanced avidity of the FA-NE for FR-α+ cells. The surface area and fluidity of the monolayer of the NE's enable favorable proximity of a large number of FA molecules on a single semi-solid surface compared to the affinity of a random, single FA molecule freely rotating in solution. Based on these results and stability data, all further studies were conducted with FA-targeted NEs labeled with 1200 molecules of FA/NE.
Figure 2. FA targeted nanoemulsions significantly enhances cellular uptake in FR-α positive cells. IHC staining of FR- α in (A) A2780 (B) SKOV-3 (C) KB-WT and (D) KB-PR10 cells. Flow cytometry analysis of cellular uptake of the Rh-labeled NEs with varying amount of FA in (E) KB-WT cells, (F) KB-PR10 cells with or without pretreatment with FA (1 mM).
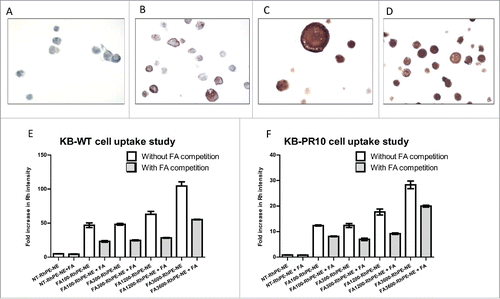
Docetaxel loaded FA-NEs are cytotoxic in cancer cells
NMI-500 is designed to deliver hydrophobic DTX to FR-α+ cancer cells. Therefore, after confirming that FA-NEs increase association to FR-α+ cancer cells, it was necessary to evaluate whether DTX retains active once encapsulated in an oil core of NEs. To do this, cell viability was assayed in KB-WT and KB-PR10 cells treated with: 1) DTX dissolved in DMSO; 2) paclitaxel dissolved in DMSO; and multifunctional DTX-loaded NEs 3) FA100-NMI-500, and 4) FA1200-NMI-500) and IC50 values obtained (). Treatment with paclitaxel confirmed the presence of P-gp-mediated resistance, with an approximately 11-fold higher IC50 against P-gp+ KB-PR10 cells (∼ 33 nM) compared to P-gp− KB-WT cells (3 nM). No cross resistance was observed for DTX, as KB-PR10 and KB-WT showed similar sensitivity at ∼ 1 nM (). Importantly, NT-NMI-500, FA100-NMI-500 and FA1200-NMI-500 also produced similar IC50s compared to DTX alone, in the range of 1 to 10 nM; these results confirming DTX retains activity once loaded into NEs.
Table 2. IC50 of docetaxel against KB-WT and KB-PR10 cells.
To further evaluate the growth inhibitory properties of NMI-500 in cell lines derived from ovarian carcinomas and other cancers, we screened the NCI-60 Human Tumor Cell Lines. Treatment with NMI-500 was compared to DTX in 60 different cancer cell lines (). The central line represents 50% Growth Inhibition (GI50), and bars on the left of the central line represent lower GI50 for NMI-500 compared to DTX and bars on the right of central line represents higher GI50 for NMI-500 compared to DTX, respectively. Treatment with NMI-500 resulted in superior (34/60) or equal (3/60) growth inhibition than DTX in more than half of the cell lines tested, including OVCAR-3, OVCAR-5, OVCAR-8 and SKOV-3 ovarian carcinoma cell lines. Several cell lines demonstrated lower GI50 of NMI-500 compared to DTX, including NCI/ADR-RES (an adriamycin resistant deriviative of OVCAR-8 cells). Interestingly, OVCAR-8 a slightly higher GI50 value for NMI-500 whereas NCI/ADR-RES showed significantly lower GI50 value for NMI-500 compared to DTX. NCI/ADR-RES exerts its resistance mechanism by overexpressing P-gp and DTX is P-gp substrate. However, NMI-500 apparently bypassed the P-gp drug efflux mechanism and increased concentration of DTX in NCI/ADR-RES cells to induce cell death ultimately resulting in significantly lower GI50. These results are consistent with our previously published data on SKOV-3 and SKOV-3TR.Citation11 Overall, these results suggest that NMI-500 inhibits the growth of a wide variety of cancer cell lines, including ovarian carcinoma cells.
Figure 3. NCI-60 Human Cancer Cell Screen of NMI-500. NMI-500 was incubated with 60 different cell lines for 72 hrs and GI50 was determined. GI50 of NMI-500 was compared to GI50 of DTX for each cell line. Bars on the left of central line represent lower GI50 and bars on the right of central line represents higher GI50 for NMI-500 compared to DTX.
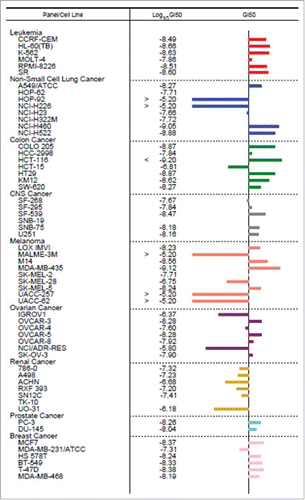
Docetaxel loaded FA-NEs exhibit favorable pharmacokinetic profiles
Essential to the development of an effective theranostic NE agent are demonstration of favorable pharmacokinetic profiles. The pharmacokinetics of NMI-500 was evaluated in BALB/c mice post tail vein injection of NMI-500, NT-NMI-500 and Taxotere (DTX). The amount of DTX present in plasma at predetermined time points was evaluated using LC-MS and show improved circulation T1/2 for DTX when administered as NMI-500 compared to when administered as taxotere (). Specifically, T1/2 for taxotere, NT-NMI-500 and NMI-500 were 1.4 h, 3.2 h and 6.1 h respectively (). The Cmax for all three formulations were 5 minutes which is consistent with i.v. administration of drug. DTX is also detected in plasma for longer times when administered as NMI-500 (24 hr) compared to NT-NMI-500 (12 hr) or taxotere (6 hr). Detection limit for docetaxel using validated LC-MS method was set at 10 ng/ml. For taxotere, docetaxel concentration fell below detection limit post 6 hr which is consistent with other published data.Citation26 The improved circulation half-life of DTX when loaded in NEs is consistent with previously published data, where we showed improved circulation time for Gd-annotated NEs compared to magnevist alone, or of novel fatty acid platinum derivatives when loaded in multifunctional NEs.Citation12 Thus, like other agents, delivery of DTX via targeted FA-NEs shows improved pharmacokinetics.
Figure 4. Pharmacokinetic profile of Taxotere, NT-NMI-500 and NMI-500. Balb/c mice were injected with formulation at 10 mg/kg of DTX intravenously via tail vein. Blood was collected at various time points and DTX concentration was measured in plasma using LC-MS. Pharmacokinetic parameters were calculated using Non-compartmental analysis using Phoenix-WinNonlin 6.2 version.
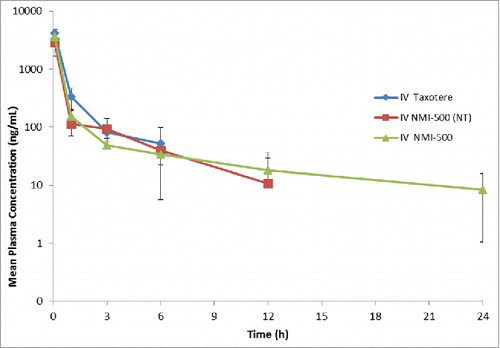
Table 3. Pharmacokinetic parameters of docetaxel administered in various formulations.
Docetaxel loaded FA-NEs inhibit in vivo tumor growth
We next evaluated the efficacy of NMI-500 to inhibit ovarian carcinoma growth in vivo in a transgenic mouse model of ovarian carcinoma. Female TgMISIIR-TAg mice develop spontaneous ovarian carcinoma that resembles human high grade serous ovarian carcinoma with 100% penetrance.Citation18,19 To assess the effect of NMI-500 on tumor growth, female TgMISIIR-TAg transgenic mice were imaged by MRI to confirm the presence of ∼100 mm3 ovarian tumors at baseline () and then randomized into cohorts for treatment with: 1) NMI-500; 2) NT-NMI-500; 3) DTX (Taxotere); or 4) blank NEs (blank) as a negative control. Mice on study were dosed weekly by tail vein injection with 10 mg/kg NT-NMI-500, NMI-500 or DTX, and treated for up to 8 weeks. Mice were weighed each week prior to dosing and exhibited no signs of toxicity or significant weight loss (). Tumor growth was monitored and quantified in all drug-treated and control mice by weekly MRI. Longitudinal tumor growth data demonstrated a highly significant (p < 0.001) delay in tumor growth rate and reduction in the size of ovarian tumors (p = 0.003) in the NMI-500-treated mice compared to blank NE- or NT-NMI-500-treated controls ( and Supplemental Fig. 1B). Caliper measurements confirmed the tumor volumes calculated from MRI datasets, showing significantly smaller tumors in NMI-500-treated mice (). The therapeutic efficacy of NMI-500 was similar to that observed in DTX-treated mice (). In addition, significantly fewer NMI-500-treated mice had ascites present at the time of necropsy; 4/21 (19%) mice compared to 12/20 (60%) vehicle-treated control mice, p = 0.01 (). Immunohistochemical staining of formalin fixed paraffin embedded tumor tissues revealed slightly increased levels of cleaved caspase-3 and phopsho-H2AX, but these differences were not significant (). Taken together, these results show that NMI-500 treatment results in significant inhibition of tumor growth and ascites production compared to the blank or NT-NMI-500. The anti-tumor activity of NMI-500 is on par with DTX.
Figure 5. NMI-500 treatment inhibits ovarian tumor growth and ascites production in TgMISIIR-TAg transgenic mice. Spontaneous tumor development and growth in TgMISIIR-TAg mice was monitored by weekly magnetic resonance imaging (MRI) and drug treatment initiated when tumor volume reached ∼100 mm3. Mice were randomized into four groups (n = 20–21 mice/group) and treated with blank NE, NT-NMI-500, NMI-500 or DTX (Taxotere). (A) Tumor volumes calculated from MRI datasets at baseline (start) and after 8 weeks of treatment. (B) Tumor growth rates (slope) computed from longitudinal MRI data. (C) Total tumor volume calculated by caliper measurement of ovaries at necropsy. (D) The presence or absence of malignant ascites determined at necropsy. The MRI data for tumor growth rate were analyzed by the Wilcoxon signed-rank test, final tumor volumes by the Mann-Whitney t test and ascites fraction by the Fisher's exact two-sided test. P values less <0.05 were considered significant (*p ≤ 0.05, **p ≤ 0.01, ***p ≤ 0.001).
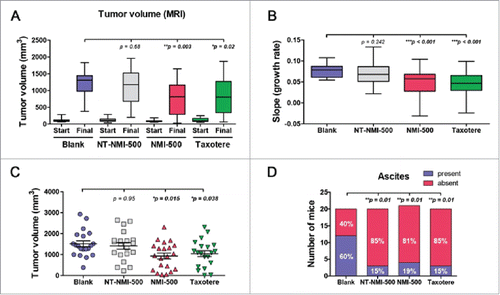
Discussion
FR-α expression is reported in a majority ovarian cancers.Citation27,28 However, FR-α expression and its prognostic association with progression free survival (PFS) or overall survival (OS) is complex.Citation29 FR-α expression has been generally associated with tumor progression and prognosis although this generalization does not hold true for all cases as for patients with high grade serous carcinoma, where overall survival benefit was identified during the first two years of follow up.Citation28,30 FR-α expression has been linked to apoptosis resistance mediated primarily through changes in the Bcl-2 network shifting towards an anti-apoptotic balance. This change in apoptotic potential may explain the relationship identified between poor prognosis and high FR-α expression levels. The selection of DTX as the payload for NMI-500 was purposefully done to respond to the observed anti-apoptotic potential of higher FR-α expression levels on the most lethal tumors. DTX, at concentration levels 100-fold less than paclitaxel, leads to the phosphorylation of Bcl-2 releasing Bax and Bak proteins, two pro-apoptotic proteins that play a major role in mitochondrial outer membrane permeabilization, a pivotal event in the intrinsic apoptosis pathway.Citation31,32 Thus, its delivery by FR-α mediated endocytosis might preferentially kill cells with a strong anti-apoptotic bias.
Normal tissue expression of FR-α is much lower and limited primarily to kidney tubuli, lung epithelium in the apical cell, the choroid plexus, and placenta.Citation33 In ovarian and other cancers of epithelial origin FR-α is expressed on the blood accessible basal and lateral membranes of the cancer tissue making FR-α an attractive tumor associated target.Citation28 Therapies targeting FR-α are pursuing an array of mechanisms of action (MOA) to eliminate FR-α expressing tumors. MOA showing promising clinical results include; Farletuzumab, an antibody that generates antibody–dependent cell-mediated cytotoxicity (ADCC); Mirvetuximab soravtansine is an antibody drug conjugate carrying maytansinoid DM4 which induces cell-cycle arrest and cell death by targeting microtubules; and Endocyte has created FA targeted small molecule drug conjugates (SMDC) vintafolide, and EC1456.Citation28 Unfortunately, Farletuzumab and vintafolide both failed to hit clinical milestones in phase 3 suitable for FDA approval however in stratified FR-α expressing cohorts improvement in clinical benefit was observed.Citation34,35 These clinical failures highlight the complexity of targeting FR-α for therapeutic benefit but also underscore the importance of creating a FR-α treatment modality that can closely integrate FR-α diagnostic capabilities to monitor responses during treatment. Our data indicate that NMI-500 has attributes that may make it an effective therapy for ovarian cancer overcoming some of the limitations with the first generation of therapies targeting FR-α.
In vitro data presented herein shows that the endocytosis process becomes significantly more efficient with an increase in valance of targeting FA on the surface of the NE. This is an important aspect of targeting FR-α as the normal adult has a concentration of FA in their blood of 4.5–45.3 nM. Important for preclinical development is that mice have ∼250 nM, rats ∼180 nm and rabbits have ∼130 nM free FA concentrations in their blood.Citation36 The dissociation constant of FA for FR-α is ∼ 1 nM. Thus it is necessary for any FA-targeted therapy to overcome competition of normal physiological FA levels for FR-α on cancer cells which appears to be achieved with NMI-500 based on our studies. While free FA can eliminate some binding of rhodamine labeled NMI-500 derivatives, significant cellular uptake was quantifiable by FACS. This enhancement means the avidity of NMI-500 is much greater than 1 nM as FA-labelled NE could not be fully competed off with up to 5,000-times free FA. Therefore the enhanced binding strength should improve uptake in ovarian cancers which is what we see in the efficacy studies. Data suggest NMI-500 should effectively bind and be endocytosed into tumor cells in most FR-α+ preclinical models chosen to study as it was effective at binding FR- α+ cells even when challenged with up to 1 mM free FA.
FA has emerged as an important targeting moiety for cancer and also inflammatory diseases as it can readily bind to activated monocytes, macrophages, and dendritic cells which express the FR.Citation37 In fact FA targeted nanoparticles enhance entry and particle retention to the Mononuclear Phagocyte System (MPS) over non FA targeted particles following systemic administration into mice.Citation37 Additionally it has been shown that the MPS can serve as a drug storage depot.Citation37 We suggest it is possible that the pharmacokinetics of NMI-500 could be influenced by uptake by the MPS and could serve as an additional advantageous property of NMI-500. Evidence supporting this hypothesis are exhibited in our data presented here and previously published.Citation11 In our previously published MRI imaging study we showed that tumor accumulation for NMI-500 is governed by second order kinetics while a similar EGFR targeted NE showed first order accumulation kinetics in SKOV-3 tumors modelCitation5 suggesting that elements not associated with first pass blood flow and long-lasting NEs design are influencing tumor accumulation of NMI-500. In our transgenic mouse model study NT-NMI-500 showed solid tumor growth inhibition comparable to the placebo control group even though it delivered the same amount of DTX as Taxotere and NMI-500 groups, suggestive that additional factors should be considered.
In a pre-clinical “trial” study in a transgenic mouse model TgMISIIR-Tag-DR26 which develops spontaneous epithelial ovarian cancer that exhibits morphology similar to human epithelial ovarian cancer, female TgMISIIR-Tag-DR26 transgenic mice which developed bilateral ovarian serous adenocarcinomas accompanied by ascites and peritoneal dissemination were tested with NT-NMI-500 and NMI-500. Treatments lasted seven weeks and the cohort treated with NMI-500 showed statistically significant tumor growth inhibition compared to control NEs or NT- NMI-500. Tumor growth inhibition was similar for DTX and NMI-500. At the conclusion of the study, for the NMI-500 treated group, we examined remaining tumor tissue by IHC to identify if there was a correlation between FR-α expression in the remaining tumor and tumor response rate. No direct correlation between FR-α expression and the observed response was identified. This lack of correlation, Supplemental Table 1 between tumor FR-α expression and response to NMI-500 might be explained if the observed response is the sum of drug delivery occurring by two different delivery modes, direct i.e., interaction between blood circulating NMI-500/tumor and indirect delivery by cells from the MPS system. Supporting a role for the MPS system in NMI-500 delivery is our unpublished observation that in arthritic rat model fluorescently-labeled FA targeted NE are taken up by FR expressing inflammatory cells which appear to accumulate in the arthritic knees of the rat over a 24 hour period while the non-targeted control NEs showed no accumulation (data not published). In the present studies the mice have intact MPS systems which might be capable of an absorbing NMI-500 and delivering it to the developing tumors as they migrate to sites of inflammation. We expect to examine this possibility in the future to ascertain if some of the solid tumor efficacy is partially driven by DTX deposition effect due to sequestration in the mononuclear phagocyte system. In summary we have developed data suggesting NMI-500 has properties suitable for clinical translation as a third generation FA-targeted therapy for ovarian and other highly lethal FR+ cancers.
Materials and methods
Materials
DTX (D-1000) was obtained from LC Laboratories. L-α-phosphatidylethanolamine transphosphatidylated (Egg, Chicken) (ePE) (841118) and 1,2-dioleoyl-sn-glycero-3-phosphoethanolamine-N-(lissamine rhodamine B sulfonyl) (ammonium salt) (Rh-PE) (810150) were purchased from Avanti Polar Lipids. Egg phosphotidylcholine (Lipoid® E80, egg lecithin) was purchased from Lipoid GmbH. 1,2-distearoyl-sn-glycero-3-phosphoethanolamine-N-[methoxy- (polyethyleneglycol)-2000] (mPEG2000-DSPE) and 1,2-distearoyl-sn-glycero-3-phosphoethanolamine-N-[maleimide (polyethylene glycol)-2000] (ammonium salt) (DSPE-PEG2000-MAL) was purchased from Laysan Bio Inc. Poly unsaturated fatty acid (PUFA) rich flaxseed oil (006420) was obtained from Puritan's Pride Inc. Cysteine (W326305), folic acid (FA) (F7876), diethylenetriaminepentaacetic acid dianhydride (DTPA) (284025), 3-[4,5-dimethyl thiazolyl]-2,5-diphenyl tetrazolium bromide (MTT reagent) (M2128), gadolinium (III) chloride (GdCl3) anhydrous (439770), and N,N'-dicyclohexylcabodiimide (DCC) (D80002) were obtained from Sigma Chemicals. All other chemicals and solvents used were of the highest available grade.
Dialysis bags with Molecular Weight Cut off (MWCO) of 3.5 kD were obtained from Spectrum Laboratories, Inc. KB-WT and KB-PR10 HeLa contaminant cells were purchased from American Type Culture Collections. Folate Receptor alpha IHC Assay Kit was obtained from Biocare Medical (DV2004LX). All other chemicals and solvents used were of the highest available grade.
Preparation and characterization of nanoemulsions (NEs)
NE formulation encapsulating DTX in its lipid core and gadolinium (Gd) and FA on the surface was prepared by a high shear homogenization method using lab scale LV1 Microfluidizer (Microfluidcis Corporation, Newton, MA) (Fig. S2). Lipidated FA and Gd-DTPA-ePE were synthesized as previously published.Citation38 Aqueous phase of the NE formulation was prepared by dissolving lipidated FA (FA-PEG2000-DSPE, 4 mg, 0.08% w/v), mPEG2000DSPE (15 mg, 0.3% w/v), egg lecithin (120 mg, 2.4% w/v), and Gd-DTPA-ePE (100 mg, 2% w/v) in 4 ml of 2.21% w/v glycerol in water for injection. The resulting solution was stirred for 2 h while vortexing every 15 min for 10 sec to achieve complete dissolution of all components. Oil phase was prepared by mixing flaxseed oil (1 g, 20% w/v) with 10 mg of DTX dissolved in chloroform. Chloroform was evaporated under steady stream of nitrogen gas. After this, aqueous and oil phases were heated (60°C, 2 min), mixed and homogenized at 25,000 psi for 10 cycles to obtain FA-targeted (1200 molecules of FA/particle) Gd-labeled DTX loaded NE formulations (NMI-500). NEs with varying density of FA on the surface were prepared by altering FA amount in above recipe. Non-targeted (NT-NMI-500) formulations were prepared without adding lipidated FA to water phase.
In addition, NEs containing Rh-PE were synthesized to perform cell association studies. The above recipe was kept the same except for the addition of Rh-PE (0.5 mg, 0.01% w/v) instead of DTX. Rh-PE used was dissolved in chloroform and added to flaxseed oil, and the chloroform was evaporated under steady stream of nitrogen gas. After this, aqueous and oil phases were heated (60°C, 2 min), mixed and homogenized at 25,000 psi for 10 cycles to obtain FA-targeted Rh-loaded NE formulations (FA-Rh-NE). Rh-loaded NEs with varying density of FA were prepared by altering FA amount in above recipe. Non-targeted formulations were prepared without adding lipidated FA to water phase (NT-Rh-NE).
Particle size distribution of all NE formulations were determined with dynamic light scattering method using Zetasizer (NanoZS90, Malvern, UK). An aliquot (10 µl) from each NEs was diluted in 10 ml of deionized water and size distribution was determined at 90° angle at 25°C using refractive index of 1.45. Polydispersity index (PDI) was used as a measure of uniformity in formulation. The zeta potential values of NEs were determined with Zetasizer (NanoZS90, Malvern, UK) based on the electrophoretic mobility of the NE droplets according to manufacturer's protocol.
A high-performance liquid chromatography (HPLC) assay was performed to measure DTX loading and encapsulation efficiency of the NEs. A Waters LC system (model 2487, Waters Corporation, Milford) comprising of a quaternary pump, an auto sampler, and UV-detector was used for the analysis. The LC system was interfaced with Empower 3 software for remote controlling, data acquisition and processing. The mobile phase consisted of acetonitrile:water (50:50 v/v) was pumped to a reverse phase C18 column (4.6 mm × 15 mm, 5 µm, Hypersil Gold, US) at a flow rate of 1 mL/min. NEs (10 µL) were diluted in 990 µl of acetonitrile, vortexed and centrifuged at 10,000 rpm for 10 min. Supernatant (10 µl) was injected and monitored for DTX elution at 230 nm.
Cell culture conditions
KB-WT, paclitaxel sensitive and KBPR-10, paclitaxel resistant cells were cultured in RPMI-1640 media without FA supplemented with 10% fetal bovine serum and 1% penicillin/streptomycin and were maintained in a humidified 95% O2/5% CO2 atmosphere at 37°C. KB-PR10 cells were maintained in media containing 10 nM paclitaxel.
Determination of H-scores using IHC for human FR-α
The degree of expression of FR-α of KB-WT and KB-PR10 was determined by using the FR-α IHC Assay Kit. Samples were prepared using three to four million cells of each cell line. Briefly, the cells were centrifuged and pellets were washed with PBS. Cells were fixed on a slide using 10% formalin solution and embedded with paraffin. The slides were then subjected to deparaffinization and rehydrated at room temperature by washing the cells in a series of reagents including xylene, reagent alcohol, 95% alcohol, 80% alcohol and finally with reagent-quality water, blocked for peroxidase. For antigen retrieval, the samples were preheated for 40 minutes at 95°C, and cooled for 20 min, incubated in Diva Decloaker solution (Cat # DV2004LX; Biocare Medial, CA) which was prepared by diluting it 1:10 (1 part Diva to 9 parts of reagent-quality water) before staining. For staining, the Diva Decloaker retrieval solution (without slides) was preheated to 95°C for 30 minutes in the Decloaking Chamber and the slides were preheated to retrieve the antigen at 95°C for 40 minutes and allowed to cool until the color of the solution changes to orange, which indicates the correct pH at high temperature. After the protein block, the cells were incubated for 30 minutes at room temperature with FR-α Negative Control Reagent (Cat # BRI4008AA; Biocare Medical, CA), wash in TBS buffer, probed with MACH 4 Mouse Probe (Cat # UP534, Biocare Medical, CA). The samples were then incubated for 10 minutes at room temperature with MACH 4 HRP Polymer (Cat # MRH534; Biocare Medical, CA), washed in TBS buffer, incubate for 5 minutes at room temperature with 1.0 mL of Betazoid DAB Substrate Buffer and counterstained with hematoxylin. Samples were mounted using a permanent mounting medium on a coverslip for subsequent imaging. To determine FR-α protein expression on the cell membrane, staining patterns were evaluated and expression scoring was based on examining all tumor cells on the slide. Evaluation of the stained samples was accomplished in a semi quantitative manner with a light microscope to assess the degree of circumferential membranous staining in the tumor cell population. The findings were classified into 1 of 4 categories: 0 = no staining in tumor cells above background; 1+ = weak staining; 2+ = moderate staining; and 3+ = strong staining in cells. A staining result of 0 was scored as IHC negative, whereas a staining result of 1+, 2+, or 3+ was scored as IHC positive.Citation14 H-score was calculated using following formula:
Cell uptake/association study
Cellular uptake of NT-Rh-NE and FA-Rh-NE in KB-WT and KB-PR10 cells was investigated by flow cytometry. Cells were seeded in three 6-well plates and grown for 24 hours in a 37°C incubator with 5% CO2. To confirm active targeting using FA, half of the wells were pretreated with 1 mM free FA for 1 hr. Cells were then treated with 100-fold diluted NT-Rh-NE or FA-Rh-NE (either with 100, 300, 1200 or 3600 FA molecules per particle) in serum-free RPMI1640 media for 1 hr. Untreated cells with or without pretreatment were taken as control. After 1 hr incubation, media was removed and wells were washed with 1x PBS three times. Cells were then trypsinized and centrifuged at 2500 rpm for 5 minutes to get cell pellet. The supernatant was decanted and the cell pellet was resuspended in 400 μl of 1% BSA in 1x PBS solution. Samples were then subjected to FACS where Rh intensity representing NE association with cells was measured from at least 10,000 gated cells.
Cytotoxicity in drug-sensitive and drug-resistant tumor cells
KB-WT and KB-PR10 cells were seeded in 96-well plates at 3,000 cells per well 24 h prior to treatment. Cells were then treated with DTX dissolved in DMSO, PTX dissolved in DMSO, NT-NMI-500, FA100-NMI-500 (NMI-500 targeted with 100 molecules of FA/particle) and FA1200-NMI-500 (NMI-500 targeted with 1200 molecules of FA/particle). Treatment concentrations of DTX and PTX ranged from 10 pM to 100 μM over eight orders of magnitude. Untreated cells were used as negative control. After 72 hours, cells were washed with complete media followed by treatment with 50μL of Thiazolyl Blue Tetrazolium Bromide MTT solution (2 mg/mL). Cells were then incubated for another 3 hours followed by addition of 150 μl of DMSO to dissolve crystals produced by live cells. Plates were subjected to shaker to achieve complete dissolution of crystals for 15 minutes before being measuring absorbance (ABS) with a Bio-Tek Synergy HT plate reader (Winooski, VT) at 570 nm. Percent cell viability was calculate using following formula and IC50 values were calculated using GraphPad Prism.
NCI-60 human tumor cell lines screen
NCI-60 Human Tumor Cell Lines Screen evaluates novel compounds for their growth inhibition effects against 60 different human tumor cell lines, representing leukemia, melanoma and cancers of the lung, colon, brain, ovary, breast, prostate and kidney. We submitted NMI-500 to Developmental Therapeutics Program (DTP) at National Cancer Institute (NCI) for screening and its growth inhibition effects were compared against DTX on single dose basis.
Pharmacokinetic study
Procedures involving these mice were approved by the Agilux Institutional Animal Care and Use Committee (IACUC) and were conducted at Agilux. Mice were maintained under pathogen free conditions and standard diet. Pharmacokinetic profile of DTX administered in excipients present in commercially available formulation (Taxotere) as well as multi-functional NE, NMI-500 were evaluated in Balb/c after IV administration at 10 mg/kg via tail vein. After, 5 min, 1 hr, 3 hr, 6 hr, 12 hr, and 24 hr, blood was collected from mice (n = 3 for each time point) in heparinized tubes. These blood samples were processed to separate plasma and the DTX concentrations in the plasma samples were determined by LC-MS method. Pharmacokinetic parameters for NMI-500 and taxotere were calculated by non-compartmental analysis using Phoenix-WinNonlin 6.2 version.
Efficacy study against spontaneousmouse model (FCCC)
The C57BL/6J TgMISIIR-TAg transgenic mouse model employed for this study has been described and develop spontaneous ovarian tumors with 100% penetrance.Citation18,19 Procedures involving these mice were approved by the Fox Chase Cancer Center (FCCC) Institutional Animal Care and Use Committee (IACUC) and were conducted at FCCC. Mice were maintained under pathogen free conditions on a standard rodent diet (2018SX Teklad Global, Harlan Laboratories) at FCCC. Female TgMISIIR-TAg mice were generated by breeding male TgMISIIR-TAg mice to wild type C57BL/6J females. Offspring of these crosses were genotypedCitation18 and transgene positive female offspring were selected for drug efficacy studies. Ovarian tumor growth was monitored and quantified by weekly in vivo imaging using a 7 Tesla vertical wide-bore magnet, equipped with a Bruker DRX 300 spectrometer.Citation19 Weekly baseline images were initiated when mice were 8–10 weeks old to detect and confirm the presence of ovarian tumor(s), and tumor volume was determined using three dimensional MRI data sets and volumetric analysis.Citation19 When at least one ovary exhibited enlargement to ∼100 mm3, mice were randomized to one of four treatment groups to evaluate drug efficacy: 1) blank NE (blank); 2) (Non targeted-NMI-500; 3) FA receptor alpha-targeted NE with 1200 molecules of FA/NE NMI-500 (NMI-500); and 4) DTX (Taxotere). All mice were treated with formulations once a week intravenously via tail vein injection at 10 mg/kg of DTX. Mice were imaged 24 hours post drug treatment (weekly) by MRI and tumor volumes calculated as described.Citation19,39 Mice were euthanized when total ovarian tumor volume (right + left) reached ∼1500 mm3 or when mice met humane criteria for euthanasia in accordance with FCCC IACUC policy.
Mice were euthanized, necropsied and examined for tumors and ascites production. Reproductive tracts were removed and ovarian tumors length (L) and width (W) were measured with calipers and tumor volume calculated (L × W2 × 0.5). Individual portions of tumors were fixed in 10% neutral buffered formalin, paraffin embedded and sectioned for H&E staining and immunohistochemical detection of FR-α, Ki-67, cleaved caspase-3 and phospo-H2AX.Citation18 Immunostained tissue sections were analyzed using the Vectra Multispectral Imaging System to compute a composite score (H-score) of intensity (0, 1, 2, and 3) and extent (percent) of staining in ten random fields of tumor tissue present in each tissue section.Citation40
Data analysis
Data are reported as average ± standard deviation. Comparisons between the groups were made using student's t-test. The p < 0.05 values were considered statistically significant. All statistical analysis was performed using Graphpad Prism. All MRI data and endpoint analyses (tumor volume, presence or absence of ascites) were evaluated by a statistician (Dr. S. Litwin, FCCC). Tumor growth rates were calculated from the weekly MRI data and by caliper measurements and analyzed by Wilcoxon two-sample, two-sided tests comparing tumor volume in all treatment pairs. Immunostaining ascites volume was measured and compared by two-sided Fisher's exact tests. Analysis of immunohistochemical staining was performed by comparison of the computed H-scores for each case by one-way ANOVA tests across all four treatment groups and by Mann-Whitney non-parametric test to compare staining in individual treatment groups to the control (blank) treated mice. In all cases, p < 0.05 were considered significant.
sup_mat_1395118_KCBT.zip
Download Zip (307.3 KB)Acknowledgments
Research reported in this publication was supported by the National Cancer Institute of the National Institutes of Health under Awards Number R01 CA158881, Number U54 CA151881 and Nemucore Medical Innovations, Inc. This project was funded in part by R01 CA136596 (DCC) and the Fox Chase Cancer Center Core Grant NCI P30 CA006927, and utilized FCCC Laboratory Animal, Biological Imaging/Small Animal Imaging, Histopathology, Biosample Repository and Biostatistics Facilities. We would also like to thank you to the late Dr. Susan Keyes, Nicole Stephenson, Laurie Cote, Phil Heisler, Brian Zifcak and Keri Hetherman for the valuable discussions, technical writing, and administrative assistance.
Additional information
Funding
References
- Siegel RL, Miller KD, Jemal A. Cancer statistics, 2016. CA Cancer J Clin. 2016;66:7–30.
- Parker SL, Tong T, Bolden S, Wingo PA. Cancer statistics, 1996. CA Cancer J Clin. 1996;46:5–27.
- Ganta S, Talekar M, Singh A, Coleman TP, Amiji MM. Nanoemulsions in Translational Research-Opportunities and Challenges in Targeted Cancer Therapy. AAPS Pharm Sci Tech. 2014;15:694–708.
- Ganta S, Singh A, Patel NR, Cacaccio J, Rawal YH, Davis BJ, Amiji MM, Coleman TP. Development of EGFR-Targeted Nanoemulsion for Imaging and Novel Platinum Therapy of Ovarian Cancer. Pharm Res. 2014;31:2490–502.
- Ganta S, Singh A, Kulkarni P, Keeler AW, Piroyan A, Sawant RR, Patel NR, Davis B, Ferris C, O'Neal S, et al. EGFR Targeted Theranostic Nanoemulsion for Image-Guided Ovarian Cancer Therapy. Pharm Res. 2015;32:2753–63.
- Goren D, Horowitz AT, Tzemach D, Tarshish M, Zalipsky S, Gabizon A. Nuclear delivery of doxorubicin via folate-targeted liposomes with bypass of multidrug-resistance efflux pump. Clin Cancer Res. 2000;6:1949–57.
- Gabizon A, Tzemach D, Gorin J, Mak L, Amitay Y, Shmeeda H, Zalipsky S. Improved therapeutic activity of folate-targeted liposomal doxorubicin in folate receptor-expressing tumor models. Cancer Chemother Pharmacol. 2010;66:43–52.
- Shmeeda H, Mak L, Tzemach D, Astrahan P, Tarshish M, Gabizon A. Intracellular uptake and intracavitary targeting of folate-conjugated liposomes in a mouse lymphoma model with up-regulated folate receptors. Mol Cancer Ther. 2006;5:818–24.
- Kim D, Gao ZG, Lee ES, Bae YH. In vivo evaluation of doxorubicin-loaded polymeric micelles targeting folate receptors and early endosomal pH in drug-resistant ovarian cancer. Mol Pharm. 2009;6:1353–62.
- Kim D, Lee ES, Oh KT, Gao ZG, Bae YH. Doxorubicin-loaded polymeric micelle overcomes multidrug resistance of cancer by double-targeting folate receptor and early endosomal pH. Small. 2008;4:2043–50.
- Ganta S, Singh A, Rawal Y, Cacaccio J, Patel NR, Kulkarni P, Ferris CF, Amiji MM, Coleman TP. Formulation development of a novel targeted theranostic nanoemulsion of docetaxel to overcome multidrug resistance in ovarian cancer. Drug Deliv. 2014:1–13.
- Patel NR, Piroyan A, Nack AH, Galati CA, McHugh M, Orosz S, Keeler AW, O'Neal S, Zamboni WC, Davis B, et al. Design, Synthesis, and Characterization of Folate-Targeted Platinum-Loaded Theranostic Nanoemulsions for Therapy and Imaging of Ovarian Cancer. Mol Pharm. 2016;13:1996–2009.
- Swanson SD, Kukowska-Latallo JF, Patri AK, Chen C, Ge S, Cao Z, Kotlyar A, East AT, Baker JR. Targeted gadolinium-loaded dendrimer nanoparticles for tumor-specific magnetic resonance contrast enhancement. Int J Nanomedicine. 2008;3:201–10.
- Fisher RE, Siegel BA, Edell SL, Oyesiku NM, Morgenstern DE, Messmann RA, Amato RJ. Exploratory study of 99 mTc-EC20 imaging for identifying patients with folate receptor-positive solid tumors. J Nucl Med. 2008;49:899–906.
- Leamon CP, Low PS. Delivery of macromolecules into living cells: a method that exploits folate receptor endocytosis. Proc Natl Acad Sci USA. 1991;88:5572–6.
- Bandara NA, Hansen MJ, Low PS. Effect of receptor occupancy on folate receptor internalization. Mol Pharm. 2014;11:1007–13.
- Tiwari SB, Tan Y, Amiji M. Preparation and In Vitro Characterization of Multifunctional Nanoemulsions for Simultaneous MR Imaging and Targeted Drug Delivery. J Biomed Nanotechnol. 2006;2:1–8.
- Connolly DC, Bao R, Nikitin AY, Stephens KC, Poole TW, Hua X, Harris SS, Vanderhyden BC, Hamilton TC. Female mice chimeric for expression of the simian virus 40 TAg under control of the MISIIR promoter develop epithelial ovarian cancer. Cancer Res. 2003;63:1389–97.
- Hensley H, Quinn BA, Wolf RL, Litwin SL, Mabuchi S, Williams SJ, Williams C, Hamilton TC, Connolly DC. Magnetic resonance imaging for detection and determination of tumor volume in a genetically engineered mouse model of ovarian cancer. Cancer Biol Ther. 2007;6:1717–25.
- Mabuchi S, Altomare DA, Connolly DC, Klein-Szanto A, Litwin S, Hoelzle MK, Hensley HH, Hamilton TC, Testa JR. RAD001 (Everolimus) delays tumor onset and progression in a transgenic mouse model of ovarian cancer. Cancer Res. 2007;67:2408–13.
- Reddy J, Abburi C, Hofland H, Howard S, Vlahov I, Wils P, Leamon C. Folate-targeted, cationic liposome-mediated gene transfer into disseminated peritoneal tumors. Gene Therapy. 2002:9.
- Gabizon A, Horowitz AT, Goren D, Tzemach D, Mandelbaum-Shavit F, Qazen MM, Zalipsky S. Targeting folate receptor with folate linked to extremities of poly (ethylene glycol)-grafted liposomes: in vitro studies. Bioconjug Chem. 1999;10:289–98.
- Wang S, Lee RJ, Mathias CJ, Green MA, Low PS. Synthesis, purification, and tumor cell uptake of 67 Ga-deferoxamine-folate, a potential radiopharmaceutical for tumor imaging. Bioconjug Chem. 1996;7:56–62.
- Garbuzenko O, Zalipsky S, Qazen M, Barenholz Y. Electrostatics of PEGylated micelles and liposomes containing charged and neutral lipopolymers. Langmuir. 2005;21:2560–8.
- Reddy JA, Xu L-C, Parker N, Vetzel M, Leamon CP. Preclinical Evaluation of 99 mTc-EC20 for Imaging Folate Receptor–Positive Tumors. J Nucl Med. 2004;45:857–66.
- Chu KS, Hasan W, Rawal S, Walsh MD, Enlow EM, Luft JC, Bridges AS, Kuijer JL, Napier ME, Zamboni WC, et al. Plasma, tumor and tissue pharmacokinetics of Docetaxel delivered via nanoparticles of different sizes and shapes in mice bearing SKOV-3 human ovarian carcinoma xenograft. Nanomed: Nanotechnol, Biol, Med. 2013;9:686–93.
- Luyckx M, Votino R, Squifflet JL, Baurain JF. Profile of vintafolide (EC145) and its use in the treatment of platinum-resistant ovarian cancer. Int J Womens Health. 2014;6:351–8.
- Vergote IB, Marth C, Coleman RL. Role of the folate receptor in ovarian cancer treatment: evidence, mechanism, and clinical implications. Cancer Metastasis Rev. 2015;34:41–52.
- Wen Y, Graybill WS, Previs RA, Hu W, Ivan C, Mangala LS, Zand B, Nick AM, Jennings NB, Dalton HJ, et al. Immunotherapy targeting folate receptor induces cell death associated with autophagy in ovarian cancer. Clin Cancer Res. 2015;21:448–59.
- Kobel M, Madore J, Ramus SJ, Clarke BA, Pharoah PD, Deen S, Bowtell DD, Odunsi K, Menon U, Morrison C, et al. Evidence for a time-dependent association between FOLR1 expression and survival from ovarian carcinoma: implications for clinical testing. An Ovarian Tumour Tissue Analysis Consortium Study. Br J Cancer. 2014;111:2297–307.
- Brunelle JK, Letai A. Control of mitochondrial apoptosis by the Bcl-2 family. J Cell Sci. 2009;122:437–41.
- Gligorov J, Lotz JP. Preclinical pharmacology of the taxanes: implications of the differences. Oncologist. 2004;9(Suppl 2).3–8.
- Parker N, Turk MJ, Westrick E, Lewis JD, Low PS, Leamon CP. Folate receptor expression in carcinomas and normal tissues determined by a quantitative radioligand binding assay. Anal Biochem. 2005;338:284–93.
- Sato S, Itamochi H. Profile of farletuzumab and its potential in the treatment of solid tumors. Onco Targets Ther. 2016;9:1181–8.
- Vergote I, Leamon CP. Vintafolide: a novel targeted therapy for the treatment of folate receptor expressing tumors. Ther Adv Med Oncol. 2015;7:206–18.
- Leamon CP, Reddy JA, Dorton R, Bloomfield A, Emsweller K, Parker N, Westrick E. Impact of high and low folate diets on tissue folate receptor levels and antitumor responses toward folate-drug conjugates. J Pharmacol Exp Ther. 2008;327:918–25.
- Li T, Gendelman HE, Zhang G, Puligujja P, McMillan JM, Bronich TK, Edagwa B, Liu XM, Boska MD. Magnetic resonance imaging of folic acid-coated magnetite nanoparticles reflects tissue biodistribution of long-acting antiretroviral therapy. Int J Nanomed. 2015;10:3779–90.
- Ganta S, Singh A, Rawal Y, Cacaccio J, Patel NR, Kulkarni P, Ferris CF, Amiji MM, Coleman TP. Formulation development of a novel targeted theranostic nanoemulsion of docetaxel to overcome multidrug resistance in ovarian cancer. Drug delivery. 2014:1–13.
- Hensley HH, Roder NA, O'Brien SW, Bickel LE, Xiao F, Litwin S, Connolly DC. Combined in vivo molecular and anatomic imaging for detection of ovarian carcinoma-associated protease activity and integrin expression in mice. Neoplasia. 2012;14:451–62.
- Liu M, Mor G, Cheng H, Xiang X, Hui P, Rutherford T, Yin G, Rimm DL, Holmberg J, Alvero A, et al. High frequency of putative ovarian cancer stem cells with CD44/CK19 coexpression is associated with decreased progression-free intervals in patients with recurrent epithelial ovarian cancer. Reprod Sci. 2013;20:605–15.