ABSTRACT
Buformin is an old anti-diabetic agent and manifests potent anti-tumor activities in several malignancies. In the present study, we aimed to explore the functions of buformin in human cervical cancer. As our data shown, buformin exhibited significant anti-proliferative effects in a dose-dependent manner in 4 cervical cancer cell lines. Compared to the control, buformin notably suppressed colony formation and increased ROS production in C33A, Hcc94 and SiHa cells. Flow cytometric analysis showed that buformin induced marked cell cycle arrest but only resulted in mild apoptosis. The invasion of C33A and SiHa cells sharply declined with buformin treatment. Consistently, western blotting showed that buformin activated AMPK and suppressed S6, cyclin D1, CDK4, and MMP9. Moreover, we found that buformin enhanced glucose uptake and LDH activity, increased lactate level, while decreased ATP production in cervical cancer cells. In addition, low doses of buformin synergized with routine chemotherapeutic drugs (such as paclitaxel, cisplatin, and 5-FU) to achieve more significant anti-tumor effects. In vivo, a single use of buformin exerted moderate anti-tumor effects, and the combination with buformin and paclitaxel exhibited even greater suppressive effects. Buformin also consistently showed synergistic effects with paclitaxel in treating primary cultures of cervical cancer cells. Take together, we are the first to demonstrate that buformin suppresses cellular proliferation and invasion through the AMPK/S6 signaling pathway, which arrests cell cycle and inhibits cellular invasion. Buformin also could synergize with routine chemotherapies, producing much more powerful anti-tumor effects. With these findings, we strongly support buformin as a potent choice for treating cervical cancer, especially in combination with routine chemotherapy.
Introduction
In most less-developed countries, cervical cancer remains the leading genital malignancy, causing an inordinate number of deaths and creating great burdens on the local economy and health care system.Citation1,Citation2 In China, there were an estimated 98,900 newly diagnosed cervical cancer cases and 30,500 deaths in 2015.Citation3 Without wide implementation of early screening, a considerable number of patients were diagnosed with advanced invasive cervical cancer when they sought medical advice.Citation3 Although most of these patients responded well to concurrent chemotherapy and radiotherapy, more than 30% of them ultimately died of local recurrence and /or distant metastasis within 5 y of their diagnosis.Citation4 Thus, there is an urgent need to develop new drugs and uncover novel functions for existing drugs.
During the previous century, the biguanides (including metformin, phenformin and buformin) had been widely used to treat type 2 (non-insulin dependent) diabetes mellitus.Citation5,Citation6 Their targets included the insulin receptor, AMPK, mTOR, and many other molecules, eventually improving insulin sensitivity.Citation7,Citation8 Several recent epidemiologic studies have found that the biguanides significantly reduced cancer risk in diabetic patients.Citation9–12 Furthermore, in a large panel of cancer cell lines and animal models, these drugs were found to activate AMPK, block mTOR and insulin/IGF signaling, and inhibit complex I in mitochondria, ultimately resulting in significant suppressive effects on cellular proliferation, adhesion, invasion, angiogenesis, and metabolism.Citation13-17
Compared with metformin and phenformin, our knowledge regarding the anti-cancer effects of buformin is limited.Citation18 In 1980, Alexandrov et al. reported that the postnatal administration of buformin significantly decreased the incidence of malignant neurogenic tumors in rats.Citation19 Moreover, buformin reduced the cancer risk in a female rat model of mammary carcinogenesis induced by 7,12-dimethylbenz(a)anthracene (DMBA), which also prolonged the latent period of many other neoplasms.Citation20 Interestingly, in another mammary cancer rat model, Zhu et al. found that buformin notably decreased cancer incidence, multiplicity, and burden; however, these effects were not seen in rats fed with phenformin or metformin.Citation21 Based on these results, we propose that buformin might be a potent anti-cancer drug for treatment of cervical cancer, and we performed a series of experiments to illustrate this possibility.
Results
Buformin suppresses proliferation and stimulates ROS production by activating AMPK signaling in cervical cancer cells
The anti-proliferative effect of buformin was examined in 4 immortalized human cervical cancer cell lines: C33A, Hcc94, HeLa, and SiHa. As the results of the CCK-8 assay shown, the 72-hour treatment with buformin exhibited significant suppressive effects on cellular growth in C33A, Hcc94, and SiHa cells (IC50 = 236.2 μM, 166.1 μM, and 622.8 μM, respectively, ); but only exerted moderate effects in HeLa cells (IC50 = 1202 μM, ). Compared with normal controls, the 2-week treatment of 100 μM buformin sharply inhibited colony formation in C33A, Hcc94, and SiHa cells (P = 0.001, 0.008, and 0.029, respectively; ). Since biguanide agents could induce the ROS production, we then analyzed ROS changes in the 3 cell lines. After a 6-hour treatment with buformin, ROS production in the 10-μM, 100-μM, and 500-μM groups was much higher than in the control group, and behaved in a dose-dependent manner (). As western bloting analysis shown, buformin activated the phosphorylation of AMPK in a dose-dependent manner while there were no effects on the level of pan-AMPK (). Consequently, the phosphorylation of S6 (a key factor in downstream events of the AMPK signaling pathway) was significantly suppressed ().
Figure 1. Buformin suppresses proliferation and stimulates ROS production by activating AMPK signaling in cervical cancer cells. (A) Buformin exhibited significant suppressive effects on cellular growth in C33A, Hcc94, and SiHa cells (IC50 = 236.2 μM, 166.1 μM, and 622.8 μM, respectively), but only exerted moderate effects in HeLa cells (IC50 = 1202 μM); (B) Compared with normal controls, buformin sharply inhibited colony formation in C33A, Hcc94, and SiHa cells (P = 0.001, 0.008, and 0.029, respectively); (C) After a 6-hour treatment with buformin, the ROS production in the 10-μM, 100-μM, and 500-μM groups was much higher than in the control group, and behaved in a dose-dependent manner; (D) Buformin activated the phosphorylation of AMPK in a dose-dependent manner while there were no effects on the level of pan-AMPK. Consequently, the phosphorylation of S6 (a key factor in downstream events of the AMPK signaling pathway) was significantly suppressed. *: p < 0.05; **: p < 0.01.
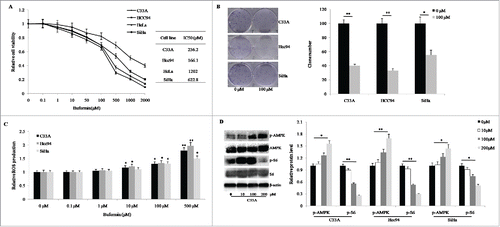
Buformin arrests cell cycle progression but has no effect on cellular apoptosis in cervical cancer cells
We investigated the effects of buformin on cell cycle kinetics and apoptosis by treating cervical cancer cells with different doses of buformin (0 μM, 10 μM, 100 μM, or 200 μM). According with the results from flow cytometry, significant (in C33A and Hcc94 cells) and moderate (in SiHa cells) G1-phase arrest were detected in the 100-μM and 200-μM groups (P<0.001, <0.001, = 0.017, respectively; and ). Western blots showed that cyclin D1 and CDK4 protein expression was markedly downregulated by the treatment with buformin in a dose-dependent manner in all 3 cervical cancer cell lines ().
Figure 2. Buformin arrests cell cycle progression in cervical cancer cells. (A and B) According to the results from flow cytometry, significant (in C33A and Hcc94 cells) and moderate (in SiHa cells) G1-phase arrest were detected in the 100-μM and 200-μM groups (P < 0.001, <0.001, = 0.017, respectively); (C) Western blots showed that cyclin D1 and CDK4 protein expression was markedly downregulated by the treatment with buformin in a dose-dependent manner in all 3 cervical cancer cell lines. *: p < 0.05; **: p < 0.01; ***: p < 0.001.
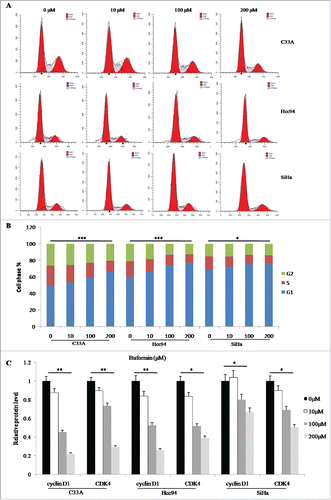
Twenty-four-hour treatment with different concentrations of buformin, however, only induced mild apoptosis in C33A cells (P = 0.045, and ); while no significant changes in apoptosis were detected in Hcc94 or SiHa cells ( and ).
Figure 3. Buformin exihibits slight effect on cellular apoptosis in cervical cancer cells. (A and B) Twenty-four-hour treatment with different concentrations of buformin, induced mild apoptosis in C33A cells (P = 0.045); while no significant changes in apoptosis were detected in Hcc94 or SiHa cells. *: p < 0.05; NS: not significant.
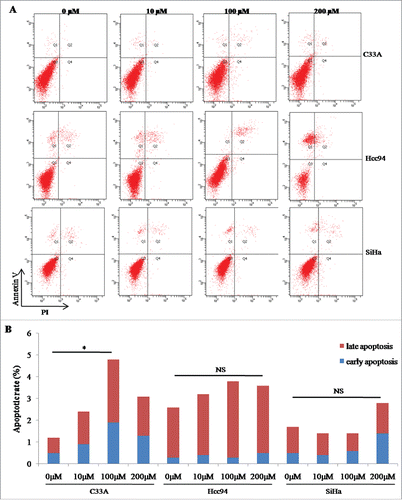
Buformin inhibits cellular invasion by downregulating MMP9
Since biguanides have been reported to be involved in regulating tumor invasion, we then used a transwell system to investigate the effects of buformin on cellular invasive capabilities. Compared to the negative control, 24-hour treatment with buformin significantly decreased the number of cells that penetrated through the artificial matrix (P = 0.006 and 0.021, respectively; and ). We also detected the expression of MMP9, a known target of buformin and another key invasion regulator. As shown in and , buformin downregulated the protein expression of MMP9 in both C33A and SiHa cells (P = 0.027 and 0.41, respectively).
Figure 4. Buformin inhibits cellular invasion by downregulating MMP9. (A and B) Compared to the negative control, 24-hour treatment with buformin significantly decreased the number of C33A and SiHa cells that penetrated through the artificial matrix (P = 0.006 and 0.021, respectively); (C and D) we also found that buformin notably downregulated the protein expression of MMP9 in both C33A and SiHa cells (P = 0.027 and 0.41, respectively). *: p < 0.05; **: p < 0.01.
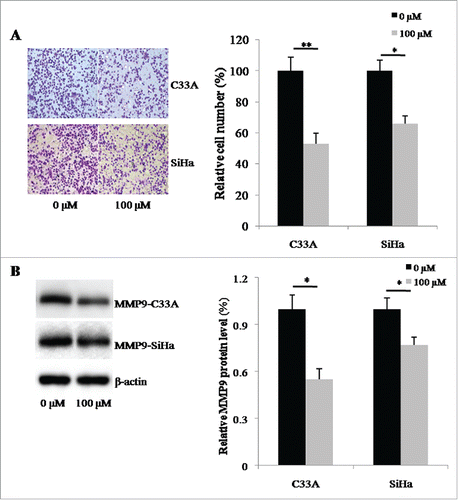
Buformin restricted ATP production and increased lactate level in cervical cancer cells
Given the anti-diabetic roles of buformin, we further tested the alterations of glucose metabolism in cervical cancer cells. As shown in , the 24-hour treatment with buformin significantly enhanced the glucose uptake of all the three cervical cancer cells in a dose-dependent manner (P = 0.026, 0.005 and 0.037, respectively). Moreover, buformin also increased LDH activity (P = 0.021, 0.017 and 0.042, respectively, ) and lactate production (P = 0.005, 0.009 and 0.028, respectively, ) in these cervical cancer cell lines. Eventually, the ATP production was sharply declined by the treatment of buformin in cervical cancer cells (P = 0.001, 0.001 and 0.003, respectively, ).
Figure 5. Buformin decreased ATP level through promoting LDH activity and upregulating lactate production in cervical cancer cells. (A) the 24-hour treatment with buformin significantly enhanced the glucose uptake of all the three cervical cancer cells in a dose-dependent manner (P = 0.026, 0.005 and 0.037, respectively). (B and C) buformin increased LDH activity (P = 0.021, 0.017 and 0.042, respectively) and lactate production (P = 0.005, 0.009 and 0.028, respectively) in these cervical cancer cell lines; (D) the ATP production was sharply declined by the treatment of buformin in cervical cancer cells (P = 0.001, 0.001 and 0.003, respectively). *: p < 0.05; **: p < 0.01.
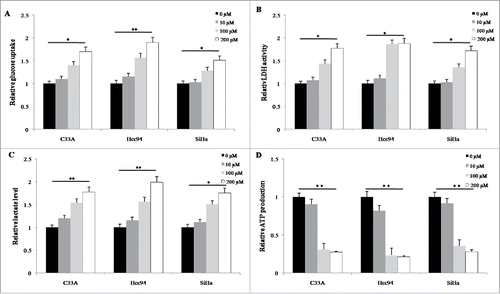
Buformin synergizes with chemotherapy in cervical cancer cell lines and primary cultured cervical cancer tissues
We evaluated the synergistic effects between buformin and conventional chemotherapeutic agents. In C33A cells, low-dose buformin (1μM) significantly enhanced the anti-proliferative effects when combined with cisplatin, paclitaxel, or 5-FU (). We then calculated the synergistic effects of buformin and cisplatin, paclitaxel, or 5-FU using the Chou-Talalay equation. As shown in , 1 μM buformin manifested potent synergistic effects with the 3 chemotherapeutic drugs in cervical cancer cells (CI <1 indicated a synergistic effect between the two drugs).
Figure 6. Buformin synergizes with chemotherapy in cervical cancer cells. (A) In C33A cells, low-dose buformin (1μM) significantly enhanced the anti-proliferative effects when combined with paclitaxel, cisplatin, or 5-FU; (B) 1 μM buformin manifested potent synergistic effects with the 3 chemotherapeutic drugs in C33A, Hcc94, and SiHa cells (CI < 1); (C) In 8 primarily cultured human cervical cancer tissues, the addition of 1 μM buformin significantly increased cellular sensitivity to paclitaxel in 75% (6/8) of samples.
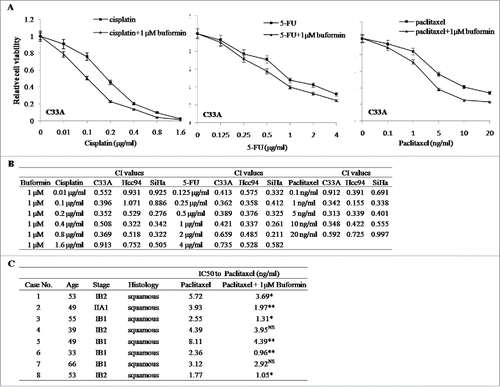
To validate our findings, we further incubated 8 fresh cervical cancer tissues in primary culture, and tested the effects of buformin on cellular responses to paclitaxel. As shown in , the addition of 1 μM buformin significantly increased cellular sensitivity to paclitaxel in 75% (6/8) of samples.
Buformin synergizes with paclitaxel to suppress the growth of cervical cancer xenografts
We additionally established a nude mouse model bearing SiHa xenografts to validate the anti-tumor effects of buformin. As the growth curve demonstrates, compared to the control group, tumor growth was significantly suppressed in both the buformin and paclitaxel groups (P = 0.037 and 0.015, respectively, ). Moreover, the combined therapy of buformin and paclitaxel showed even greater suppressive effects (P = 0.002, compared to the control group, ). Consistently, tumor weights showed similar trends in the three treatment groups (P = 0.039, 0.033 and 0.01, respectively, ). Then we performed the immunostaining to test the alterations of key proteins. Comparing to the control, the expression of Ki-67 were dowregulated nearly 50% in tumors treated with buformin or paclitaxel (P = 0.021 and 0.036, respectively, and ), which was further suppressed to just 20% in the combination group (P = 0.006, and ). As a direct target of buformin, the phosphorylation of AMPK was upregulated in tisses treated with buformin and the combined therapy (P = 0.042 and 0.033, respectively, and ), while no change was detected in the control group or the paclitaxel group ( and ). To investigate the metabolic changes in SiHa xenografts, we test the lactate concentration and LDH activity in tumor tissues. Compared to the control, both the lactate level and LDH activity were significantly upregulated in mice treated with buformin or buformin plus paclitaxel, while mild changes were detected in mice treated with paclitaxel ( and ). Considering the anti-diabetic role of buformin, we also monitored body weights and blood glucose levels during the entire experiment. Compared to the control, neither the body weights of mice or blood glucose levels differed significantly in the other three groups ( and ). In addition, no significant effects on the key biochemical indexes (including liver/renal functions and lipid metabolism) in these mice treated with buformin ().
Figure 7. Buformin synergizes with paclitaxel to suppress the growth of SiHa xenografts. (A) As the growth curve demonstrates, compared to the control group, tumor growth was significantly suppressed in both the buformin and paclitaxel groups (P = 0.037 and 0.015, respectively). Moreover, the combined therapy of buformin and paclitaxel showed even greater suppressive effects (P = 0.002, compared to the control group); (B) tumor weights in each group also presented similar trends as the tumor growth curve (P = 0.039, 0.033 and 0.01, respectively); (C and D) Comparing to the control, the expression of Ki-67 were dowregulated nearly 50% in tumors treated with buformin or paclitaxel (P = 0.021 and 0.036, respectively), which was further suppressed to just 20% in the combination group (P = 0.006). As a direct target of buformin, the phosphorylation of AMPK was upregulated in tisses treated with buformin and the combined therapy (P = 0.042 and 0.033, respectively), while no change was detected in the control group or the paclitaxel group. (E and F) compared to the control, both the lactate level and LDH activity were significantly upregulated in mice treated with buformin or buformin plus paclitaxel, while mild changes were detected in mice treated with paclitaxel; (G and H) compared to the control, neither the body weights of mice or blood glucose levels differed significantly in the other three groups. *: p < 0.05; **: P < 0.01; NS: not significant.
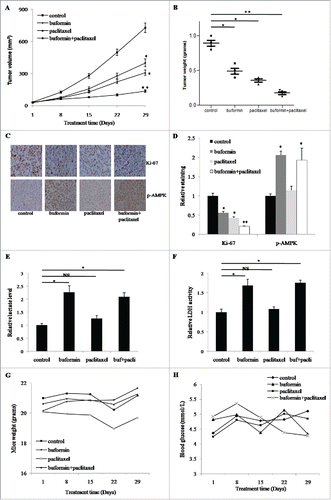
Table 1. The influence of different therapies on the key plasma analytes.
Discussion
As an important class of anti-diabetic drugs, the biguanides have shown promising anti-tumorigenic activity in many human malignancies.Citation17,Citation22-26 There are presently over 50 clinical trials exploring the efficacy of metformin and phenformin in various cancers (www.clinicaltrials.gov/). Due to the risk of lactic acidosis, buformin is now only prescribed for the treatment of type 2 diabetes mellitus in several countries, including Romania, Hungary, Taiwan, and Japan. However, recent work proved that lactic acidosis only occurred in patients with impaired renal function.Citation27,Citation28 In addition, buformin has been proposed to be more appealing since it shows greater lipophilicity and the ability to inhibit mitochondrial complex I and thereby suppress ATP production.Citation29,Citation30 Thus, we first investigated the efficacy of buformin to treat cervical cancer.
As the CCK-8 and colony formation assays showed, the proliferation of cervical cancer cells was significantly suppressed by buformin in a dose-dependent manner. Functional analysis illustrated that buformin could increase ROS levels, arrest cell cycle progression, and inhibit cellular invasion; all of these were concomitant with the activation of AMPK signaling and inhibition of its downstream proteins, including S6, cyclin D1, CDK4, and MMP9. Our results strongly support the potential of buformin to treat cervical cancer. In agreement with our data, the anti-tumor effects of buformin were also previously detected in the other two cancers. In breast cancer cells and premalignant mammary tissues with erbB-2 overexpression, stemness was significantly suppressed by buformin via deactivation of mTOR, ER, β-catenin, or other signaling pathways.Citation31 Similarly, Kilgore et al. demonstrated that treatment with buformin resulted in cell cycle arrest, induction of apoptosis, and reduction in adhesive and invasive properties; all of these phenomena were accompanied by AMPK activation and inhibition of phosphorylated-S6.Citation17 Interestingly, our results did not show a significant alteration in cellular apoptosis, even at the high dose of buformin, which might be due to the different biologic behaviors observed between the two cancer types.
For advanced cervical cancer patients, especially those with tumor recurrence or distant metastasis, chemotherapy might be more beneficial. The common chemotherapeutic drugs for cervical cancer include cisplatin, carboplatin, paclitaxel, 5-FU, and some others.Citation32 Considering that metformin has been reported to be able to improve chemosensitivity in various cancers.Citation22,Citation23,Citation33,Citation34 We herein investigated the influence of buformin on cellular sensitivity to routine chemotherapeutic drugs. Encouragingly, buformin exhibited significant synergistic effects with cisplatin, paclitaxel, and 5-FU. Moreover, we used the nude mouse model and 8 human cervical cancer samples in primary culture to further confirm that buformin could enhance the anti-tumor effects of paclitaxel. Our present knowledge of the influence of buformin on routine chemotherapies is quite limited, and this warrants further basic research and additional clinical trials.
As a classical biguanide reagent, buformin was first used to treat diabetes because it enhanced cellular sensitivity to insulin but seldom caused hypoglycemia.Citation30 However, it was withdrawn from the market or disallowed by the drug regulatory agencies of most countries due to the risk of severe lactic acidosis.Citation28,Citation35 Later studies found that lactic acidosis was rare and only occurred in those patients with renal insufficiency, and that the lactic acidosis could be avoided by adding 2-deoxyglucose (2-DG) and circumvented by supplementing with thiamine.Citation36,Citation37 In the mouse model, we demonstrated that buformin moderately increased the lactate production and LDH activity in tumor tissues, while the blood lactate level was not significantly affected. These findings supported that buformin was safe for these individuals with normal renal functions. Further, we proved that blood glucose and body weights were only mildly influenced in mice during the whole treatment with buformin. Based on these results, we believe that buformin exhibits strong potential as a safe anti-tumor drug.
In conclusion, we herein are the first to report that buformin can suppress the proliferation and invasion of cervical cancer by activating AMPK signaling and inhibiting its downstream targets, such as mTOR/S6, cyclin D1, CDK4, and MMP9. Moreover, we found that buformin showed significant synergistic effects with routine chemotherapy and marked anti-cancer efficacy. These findings suggest that buformin is a potent anti-cancer drug that targets tumor metabolism in cervical cancer.
Materials and methods
Tissue collection and culture
Tumor tissues from 8 cervical cancer patients were used for the primary culture. All these patients provided the informed consent and our protocol was approved by the ethical committee at First Affiliated Hospital of Zhengzhou University (Zhengzhou, China). In brief, fresh tissues (larger than 1 cm3) were obtained during surgery, immediately minced into tiny pieces and then digested in the 0.2% collagenase IA solution for 2–4 hours at 37°C. Finally, the tumor cells were collected and seeded into 96-well plates for CCK-8 assay.
Cell lines and reagents
C33A, Hcc94, SiHa, and HeLa cell lines were obtained from the Biochemistry and Cell Biology Institute of Chinese Academy of Sciences (Shanghai, China). The media used (with 10% fetal bovine serum added) were MEM (Hyclone, Logan, UT, USA) for C33A, RPMI 1640 (Hyclone) for SiHa and HCC 94, and DMEM (Hyclone) for HeLa. Buformin was purchased from Santa Cruz Biotechnology (Santa Cruz, CA, USA). Paclitaxel, 5-FU, and cisplatin were purchased from Abmole Bioscience (Shanghai, China). All other reagents were obtained from Sigma-Aldrich (CA, USA) unless noted otherwise.
CCK-8 assay
A cell counting kit-8 (CCK-8, Abmole Bioscience) was used to detect the anti-growth effects of buformin and other drugs. Briefly, 2 × 103 tumor cells/well were seeded in 96-well plates and cultured for at least 8 hours, and the cells were then treated with different drugs for 72 hours in an incubator. The CCK-8 solution was added to each well and the absorbance at 490 nm was determined on a microplate reader. This procedure was performed in triplicate and repeated 3 times.
Colony formation assay
One hundred cells/well were seeded in 6-well plates and cultured at least for 8 hours, and treated with 100 μM buformin or PBS (negative control). Two weeks later, cells were stained with 0.1% crystal violet and clones (≥50 cells) were counted on a microscope. This procedure was performed in duplicate and repeated 3 times.
Reactive oxygen species assay
A reactive oxygen species assay kit (ROS, Beyotime, Shanghai, China) was used to detect the production of ROS with buformin treatment. In brief, 1 × 104 cells/well were seeded in black 96-well plates and routinely cultured overnight. The cells were then treated with different doses of buformin for 6 hours, and 10 μM of DCFH-DA was added for another 20 minutes. Finally, the fluorescence (excitation = 488 nm, emission = 525 nm) was determined on a plate reader. This procedure was performed in triplicate and repeated 3 times.
Flow cytometry
We investigated cellular apoptotic status by using an Annexin V-FITC/PI apoptosis detection kit purchased from CoWin Biosciences (Beijing, China). The procedures were performed strictly following the manufacturer's instructions. First, 1 × 106 cells were plated into a 60-mm dish and cultured overnight before treatment with different doses of buformin. Twenty-four hours later, the cells were gently harvested and stained with Annexin V and PI, and the apoptotic rate was determined on a FACS caliber system (BD Biosciences, CA, USA).
For cell cycle detection, 1 × 106 cells were cultured and treated with buformin for 24 hours as described above. The cells were then harvested and stained with PI, and cell cycle distribution was determined on the FACS caliber system. This procedure was repeated 3 times.
Invasion assay
A transwell system (Corning, NY, USA) was used to test invasive abilities of cervical cancer cells. In brief, 1 × 105 cells were loaded onto the upper chamber which was embedded with Matrigel (BD Biosciences, CA, USA), and 400 µl of medium with 10% FBS was added to the lower chamber. PBS (control) or 100 μM buformin was added to the upper chamber 6 hours later and the cells were further cultured for 24 hours. Then the chamber was gently rinsed and cells that remained on the membrane were fixed with methanol and stained with crystal violet. Cell number was counted on an inverted microscope.
ATP assay
A commercial ATP assay kit (Beyotime) was used to detect the level of ATP in cancer cells. Briefly, 5 × 103 cells/well were routinely seeded into 96-well plates and then treated with buformin for 24 hours. To measure the ATP level, 100 μl ATP detecting solution was added into each well and the luminescence intensity was read on a illuminometer. This procedure was repeated 3 times.
Lactate assay
The lactate assay kit (AAT Bioquest, CA, USA) was used to evaluate the alterations of lactate production. In brief, after the treatment with different doses of buformin, 10 μl of the culture medium was mixed with 40 μl of distilled water and 50 μl of lactate detecting solution. Then mixture was incubated for 30 min at 37°C and the absorbance at 490 nm was determined on a plate reader. This procedure was repeated 3 times.
Glucose uptake assay
4 × 103 cells/well were seeded in the 96-well plates (black) and treated by buformin for 24 hours. Next, these cells were cultured in the glucose-free medium (containing 100 μg/ml 2-NBDG) for 15 min. Then the medium was removed and cells were gently rinsed 3 times using the HBSS. The fluorescence intensity was measured on the plate reader (excitation wavelength = 485 nm, emission wavelength = 530 nm). This procedure was repeated 3 times.
LDH activity assay
The cellular LDH activity was measured using a LDH assay kit purchased from Beyotime. All procedures were performed strictly following the manufacturer's instructions. The absorbance at 490 nm was determined on the plate reader. This experiment was repeated 3 times.
Western blotting analysis
Total protein was collected from cervical cancer cells using RIPA buffer (Beyotime) and quantified using a BCA Protein Quantification Kit (Beyotime). The protein samples were separated on a 10% SDS-PAGE gel and then transferred to PVDF membranes (Beyotime). Appropriate primary and secondary antibodies were added to the membrane solution samples (see below), and the specific bands were developed using a commercial ECL chemiluminescence kit (Beyotime). The primary antibodies targeting AMPK, p-AMPK, S6, p-S6, cyclin D1, CDK4, MMP9, and GAPDH (the internal control) were all purchased from Cell Signaling Technology (Danvers, MA, USA).
Immunohistochemical staining
Slide sections cut at 4-μm thickness were dewaxed and hydrated, and heated antigen retrieval was performed in citrate buffer (10 mmol/L, pH = 6.0). Next, the slides were incubated with 5% BSA at 37°C for 10 minutes and then further incubated overnight with primary antibodies at 4°C. On the 2nd day, the slides were reacted with secondary antibody and staining was developed using a DAB chromogenic reagent kit (Beyotime) following the manufacturer's instructions. The results were scored by 2 experienced pathologists.
Tumor xenograft growth assay
Our animal-handling protocol was approved by the Committee of Laboratory Animal Care at Jiangnan University. Twenty-four female Balb/c nude mice were purchased from Slac Biotechnology (Shanghai, China) and randomized into 4 groups: control, buformin (gavage, 200 mg/kg/day, 5 days/week; 4 weeks in total), paclitaxel (intraperitoneal injection, 15 mg/kg/twice a week, 8 times in total), and buformin+paclitaxel. At the start, 5 × 106 SiHa cells were injected subcutaneously into the left axilla, and the mice were routinely raised for about 10 d. The treatments with different drugs were given when tumor volume reached approximately 100 mm3. Tumor diameters (tumor volume = length × width2/2) and blood glucose were each measured once per week. All mice were sacrificed on the 28th day, and tumor tissues and blood samples were collected for laboratory analyses.
Statistical analyses
Student's t tests, χ2 test, and ANVOA were used according to the data category. SPSS version 19.0 (Chicago, IL, USA) was used for data analysis. P<0.05 was considered to be statistically significant.
Disclosure of potential conflicts of interest
No potential conflicts of interest were disclosed.
Acknowledgments
Our study was supported by grants from NSFC (No. 81502262). We would like to thank LetPub (www.letpub.com) for providing linguistic assistance during the preparation of this manuscript.
Additional information
Funding
References
- Torre LA, Bray F, Siegel RL, Ferlay J, Lortet-Tieulent J, Jemal A. Global cancer statistics, 2012. CA Cancer J Clin. 2015;65:87–108. doi:10.3322/caac.21262. PMID:25651787.
- Small W, Jr., Bacon MA, Bajaj A, Chuang LT, Fisher BJ, Harkenrider MM, Jhingran A, Kitchener HC, Mileshkin LR, Viswanathan AN, et al. Cervical cancer: A global health crisis. Cancer. 2017;123:2404–2412. doi:10.1002/cncr.30667. PMID:28464289.
- Chen W, Zheng R, Baade PD, Zhang S, Zeng H, Bray F, Jemal A, Yu XQ, He J. Cancer statistics in China, 2015. CA Cancer J Clin. 2016;66:115–132. doi:10.3322/caac.21338. PMID:26808342.
- Mountzios G, Soultati A, Pectasides D, Pectasides E, Dimopoulos MA, Papadimitriou CA. Developments in the systemic treatment of metastatic cervical cancer. Cancer Treat Rev. 2013;39:430–443. doi:10.1016/j.ctrv.2012.05.009. PMID:22727690.
- Bailey CJ. Biguanides and NIDDM. Diabetes Care. 1992;15:755–772. doi:10.2337/diacare.15.6.755. PMID:1600835.
- Fujimoto S. Therapeutic utility of biguanides in the treatment of NIDDM. Nihon Rinsho. 1999;57:657–662. PMID:10199150.
- McIntyre HD, Ma A, Bird DM, Paterson CA, Ravenscroft PJ, Cameron DP. Metformin increases insulin sensitivity and basal glucose clearance in type 2 (non-insulin dependent) diabetes mellitus. Aust N Z J Med. 1991;21:714–719. doi:10.1111/j.1445-5994.1991.tb01375.x. PMID:1759920.
- Lisch HJ, Sailer S, Braunsteiner H. The effect of biguanides on insulin sensitivity of maturity onset diabetics (author's transl). Wien Klin Wochenschr. 1980;92:266–269. PMID:6996339.
- Wang CP, Lehman DM, Lam YF, Kuhn JG, Mahalingam D, Weitman S, Lorenzo C, Downs JR, Stuart EA, Hernandez J, et al. Metformin for reducing Racial/Ethnic difference in Prostate Cancer Incidence for Men with Type II diabetes. Cancer Prev Res (Phila). 2016;9:779–787. doi:10.1158/1940-6207.CAPR-15-0425. PMID:27026681.
- Arima R, Marttila M, Hautakoski A, Arffman M, Sund R, Ilanne-Parikka P, Kangaskokko J, Laara E, Puistola U, Hinkula M. Antidiabetic medication, statins and the risk of endometrioid endometrial cancer in patients with type 2 diabetes. Gynecol Oncol. 2017;146:636–641. doi:10.1016/j.ygyno.2017.06.011. PMID:28645427.
- Liu F, Yan L, Wang Z, Lu Y, Chu Y, Li X, Liu Y, Rui D, Nie S, Xiang H. Metformin therapy and risk of colorectal adenomas and colorectal cancer in type 2 diabetes mellitus patients: A systematic review and meta-analysis. Oncotarget. 2017;8:16017–16026. PMID:27926481.
- Tseng CH. Metformin and lung cancer risk in patients with type 2 diabetes mellitus. Oncotarget. 2017;8:41132–41142. PMID:28456789.
- Anisimov VN, Berstein LM, Popovich IG, Zabezhinski MA, Egormin PA, Tyndyk ML, Anikin IV, Semenchenko AV, Yashin AI. Central and peripheral effects of insulin/IGF-1 signaling in aging and cancer: antidiabetic drugs as geroprotectors and anticarcinogens. Ann N Y Acad Sci. 2005;1057:220–234. doi:10.1196/annals.1356.017. PMID:16399897.
- Dykens JA, Jamieson J, Marroquin L, Nadanaciva S, Billis PA, Will Y. Biguanide-induced mitochondrial dysfunction yields increased lactate production and cytotoxicity of aerobically-poised HepG2 cells and human hepatocytes in vitro. Toxicol Appl Pharmacol. 2008;233:203–210. doi:10.1016/j.taap.2008.08.013. PMID:18817800.
- Appleyard MV, Murray KE, Coates PJ, Wullschleger S, Bray SE, Kernohan NM, Fleming S, Alessi DR, Thompson AM. Phenformin as prophylaxis and therapy in breast cancer xenografts. Br J Cancer. 2012;106:1117–1122. doi:10.1038/bjc.2012.56. PMID:22361631.
- Orecchioni S, Reggiani F, Talarico G, Mancuso P, Calleri A, Gregato G, Labanca V, Noonan DM, Dallaglio K, Albini A, et al. The biguanides metformin and phenformin inhibit angiogenesis, local and metastatic growth of breast cancer by targeting both neoplastic and microenvironment cells. Int J Cancer. 2015;136:E534–544. doi:10.1002/ijc.29193. PMID:25196138.
- Kilgore J, Jackson AL, Clark LH, Guo H, Zhang L, Jones HM, Gilliam TP, Gehrig PA, Zhou C, Bae-Jump VL. Buformin exhibits anti-proliferative and anti-invasive effects in endometrial cancer cells. Am J Transl Res. 2016;8:2705–2715. PMID:27398153.
- Hanly EK, Darzynkiewicz Z, Tiwari RK. Biguanides and targeted anti-cancer treatments. Genes Cancer. 2015;6:82–83. PMID:26000092.
- Alexandrov VA, Anisimov VN, Belous NM, Vasilyeva IA, Mazon VB. The inhibition of the transplacental blastomogenic effect of nitrosomethylurea by postnatal administration of buformin to rats. Carcinogenesis. 1980;1:975–978. doi:10.1093/carcin/1.12.975. PMID:11272113.
- Anisimov VN, Aleksandrov VA, Belous NM, Vasil'eva IA, Matson VB. Inhibition of the transplacental blastomogenic effect of N-nitrosomethylurea in rats by buformin. Biull Eksp Biol Med. 1980;89:88–90. PMID:6996759.
- Zhu Z, Jiang W, Thompson MD, Echeverria D, McGinley JN, Thompson HJ. Effects of metformin, buformin, and phenformin on the post-initiation stage of chemically induced mammary carcinogenesis in the rat. Cancer Prev Res (Phila). 2015;8:518–527. doi:10.1158/1940-6207.CAPR-14-0121. PMID:25804611.
- Hanna RK, Zhou C, Malloy KM, Sun L, Zhong Y, Gehrig PA, Bae-Jump VL. Metformin potentiates the effects of paclitaxel in endometrial cancer cells through inhibition of cell proliferation and modulation of the mTOR pathway. Gynecol Oncol. 2012;125:458–469. doi:10.1016/j.ygyno.2012.01.009. PMID:22252099.
- Lengyel E, Litchfield LM, Mitra AK, Nieman KM, Mukherjee A, Zhang Y, Johnson A, Bradaric M, Lee W, Romero IL. Metformin inhibits ovarian cancer growth and increases sensitivity to paclitaxel in mouse models. Am J Obstet Gynecol. 2015;212:479 e471–479 e410. doi:10.1016/j.ajog.2014.10.026.
- Della Corte CM, Ciaramella V, Di Mauro C, Castellone MD, Papaccio F, Fasano M, Sasso FC, Martinelli E, Troiani T, De Vita F, et al. Metformin increases antitumor activity of MEK inhibitors through GLI1 downregulation in LKB1 positive human NSCLC cancer cells. Oncotarget. 2016;7:4265–4278. doi:10.18632/oncotarget.6559. PMID:26673006.
- Guo Z, Zhao M, Howard EW, Zhao Q, Parris AB, Ma Z, Yang X. Phenformin inhibits growth and epithelial-mesenchymal transition of ErbB2-overexpressing breast cancer cells through targeting the IGF1R pathway. Oncotarget. 2017;8:60342–60357. PMID:28947975.
- Shi P, Liu W, Tala Wang H, Li F, Zhang H, Wu Y, Kong Y, Zhou Z, Wang C, et al. Metformin suppresses triple-negative breast cancer stem cells by targeting KLF5 for degradation. Cell Discov. 2017;3:17010. doi:10.1038/celldisc.2017.10. PMID:28480051.
- Wittmann P, Haslbeck M, Bachmann W, Mehnert H. Lactic acidosis in diabetics on biguanides (author's transl). Dtsch Med Wochenschr. 1977;102:5–10. doi:10.1055/s-0028-1104832. PMID:11984.
- Krishnamurthy M, Sahouria JJ, Desai R, Caguiat J. Buformin-induced lactic acidosis–a symptom of modern healthcare malady. J Am Geriatr Soc. 2004;52:1785. doi:10.1111/j.1532-5415.2004.52479_7.x. PMID:15450070.
- Anisimov VN, Semenchenko AV, Yashin AI. Insulin and longevity: antidiabetic biguanides as geroprotectors. Biogerontology. 2003;4:297–307. doi:10.1023/A:1026299318315. PMID:14618027.
- Yano A, Kubota M, Iguchi K, Usui S, Hirano K. Buformin suppresses the expression of glyceraldehyde 3-phosphate dehydrogenase. Biol Pharm Bull. 2006;29:1006–1009. doi:10.1248/bpb.29.1006. PMID:16651735.
- Parris AB, Zhao Q, Howard EW, Zhao M, Ma Z, Yang X. Buformin inhibits the stemness of erbB-2-overexpressing breast cancer cells and premalignant mammary tissues of MMTV-erbB-2 transgenic mice. J Exp Clin Cancer Res. 2017;36:28. doi:10.1186/s13046-017-0498-0. PMID:28193239.
- Yee GP, de Souza P, Khachigian LM. Current and potential treatments for cervical cancer. Curr Cancer Drug Targets. 2013;13:205–220. doi:10.2174/1568009611313020009. PMID:23259831.
- Qi X, Xu W, Xie J, Wang Y, Han S, Wei Z, Ni Y, Dong Y, Han W. Metformin sensitizes the response of oral squamous cell carcinoma to cisplatin treatment through inhibition of NF-kappaB/HIF-1alpha signal axis. Sci Rep. 2016;6:35788. doi:10.1038/srep35788. PMID:27762347.
- Liu Y, He C, Huang X. Metformin partially reverses the carboplatin-resistance in NSCLC by inhibiting glucose metabolism. Oncotarget. 2017;8:75206–75216. PMID:29088858.
- Gan SC, Barr J, Arieff AI, Pearl RG. Biguanide-associated lactic acidosis. Case report and review of the literature. Arch Intern Med. 1992;152:2333–2336. doi:10.1001/archinte.1992.00400230129023. PMID:1444694.
- Lea MA, Chacko J, Bolikal S, Hong JY, Chung R, Ortega A, Desbordes C. Addition of 2-deoxyglucose enhances growth inhibition but reverses acidification in colon cancer cells treated with phenformin. Anticancer Res. 2011;31:421–426. PMID:21378320.
- Godo S, Yoshida Y, Fujita M, Kudo D, Nomura R, Shimokawa H, Kushimoto S. The dramatic recovery of a Patient with Biguanide-associated Severe Lactic Acidosis following Thiamine supplementation. Intern Med. 2017;56:455–459. doi:10.2169/internalmedicine.56.7754. PMID:28202871.