ABSTRACT
Through regulating the expression of hundreds of genes, hypoxia-inducible factor -1(HIF-1) plays a critical role in hypoxic adaption of cancer cells and is considered as a target for cancer therapy. Here we show that montelukast, a clinical leukotriene receptor antagonist for the treatment of asthma, inhibits hypoxia or CoCl2-induced HIF-1α activation and reduces its protein expression in prostate cancer cells. However, the other two leukotriene receptor antagonists, pranlukast and zafirlukast, cannot decrease HIF-1α protein, which indicates that montelukast-induced downregulation of HIF-1α is not mediated by leukotriene receptor. Neither proteasome inhibitor MG132 nor the lysosomal inhibitor chloroquine (CQ) can block montelukast-induced downregulation of HIF-1α protein. Interestingly, GSK2606414, a PKR-like endoplasmic reticulum kinase (PERK) inhibitor, abrogates montelukast-induced downregulation of HIF-1α under hypoxic conditions. However, montelukast increases phosphorylation of eIF-2α at Ser51. Moreover, montelukast inhibits the proliferation of prostate cancer cells, which can be reversed by overexpression of HIF-1α protein. In conclusion, we identify montelukast may be used as a novel agent for the treatment of prostate cancer by decreasing HIF-1α protein translation.
KEY WORDS:
Introduction
Hypoxia-inducible factor-1 (HIF-1) is a master regulator of the cellular response to the hypoxia microenvironment, which is tightly controlled through synthesis and degradation.Citation1 HIF-1 is a heterodimeric protein that is composed of an O2-regulated HIF-1α and a constitutively expressed HIF-1β subunits.Citation2 Under normoxia, HIF-1α is hydroxylated on proline residue 402 and 564 by proline hydroxylase domain (PHD) proteinsCitation3–Citation5 , which is required for the binding of the von Hippel-Lindau protein, an E3 ligase targeting HIF-1α for rapid ubiquitination and proteasomal degradation.Citation6–Citation8 Under hypoxia, PHDs activity is inhibited and HIF-1α accumulates, dimerizes with HIF-1β, thus activates the transcription of hundreds of genes in response to hypoxia, such as CA9, GLUT1.Citation9,Citation10 Hypoxia and overexpression of HIF-1 are associated with radiation therapy and chemotherapy resistance, an increased risk of invasion and metastasis, and a poor clinical prognosis of solid tumors.Citation11 Given the central role in cancer biology, HIF-1α is viewed as a viable prospective target for pharmacologic approaches to the clinical management of solid tumors.Citation12
Prostate cancer is the most common malignancy in men and the second cause of cancer deaths in America.Citation13,Citation14 Prostate cancer gives rise to the regions of hypoxia due to the rapid cell proliferation and the structural and functional abnormality of the intratumoral blood vessels.Citation11 The decreased degradation and increased synthesis of HIF-1α protein levels have been observed in prostate cancer.Citation15–Citation17 HIF-1α has been shown as a promising target for the treatment of prostate cancer. Various types of small molecule inhibitors of HIF-1α have been developed and studied previously,Citation18–Citation21 but none has been clinically used.
In the present study, we identified the antiasthma drug montelukast (MNK) may inhibit the synthesis of HIF-1α in prostate cancer cells. MNK may be repurposed for the treatment of prostate cancer.
Materials and methods
Cell lines, cell culture and reagents
Human PC3, PC3-HRE-LUC and LNCaP cells were cultured in RPMI 1640 supplemented with 10% FBS and 1% penicillin-streptomycin in a humidified incubator at 37 °C and 5% CO2/95% air (v/v). One percent O2 was generated by flushing a 94% N2/5% CO2 mixture into the incubator. Polyclonal antibodies against HIF-1α (BD Biosciences), ubiquitin (Santa Cruz Biotechnology), p62 (Sigma-Aldrich), 4EBP1 (Cell Signaling), p-4EBP1 (Thr70) (Cell Signaling), eIF-2α (Proteintech), p-eIF-2α (Ser51) (Abcam), p-PERK (Thr981) (Aviva Systems Biology), PERK (Proteintech) were used for immunoblot assays. β-tubulin (Santa Cruz Biotechnology) was used as the internal control. MNK was purchased from DALIAN MEILUN BIOLOGY TECHMOLOGY CO.,LTD.. GSK2606414 was purchased from Selleck. Cell Counting Kit-8 (CCK-8) was purchased from Dojindo Molecular Technologies, Inc. (Kumamoto, Japan).
Western blot
Cell lysates were equally loaded onto SDS-PAGE, electrophoresed and transferred to nitrocellulose membranes (Amersham). The membranes were stained with 0.2% Ponceau S red to ensure equal protein loading. After blocking with 5% nonfat milk in tris-buffered saline, the membranes were incubated with antibodies overnight at 4 °C, followed by horseradish peroxidase-linked secondary antibody (Cell Signaling) for 1 h at room temperature. Detection was performed by SuperSignal West Pico Chemiluminescent Substrate kit (Pierce, Rockford, IL) according to manufacturer's instruction. β-tubulin (Merck, Darmstadt, Germany) was used as internal control.
Transfection assays
One day before transfection, 2.0 × 106 PC3 cells were plated in 60-mm dish with growth medium. Twenty four hours later, the cells were transfected with vector or 3flag-HIF-1α-P2A using Lipofectamine 2000 according to manufacturer's instructions. Six hours later after transfection, PC3 cells were digested using 0.25% trypsin and reseeded with 3.0 × 104 per well into 96- well plates. Twenty four hour later, MNK was added with different concentrations for another 12 h and CCK-8 was added before this time point end.
Luciferase activity assay
Human PC3 cells were transfected with plasmid pGL4.27-HRE-luciferase (Luc), in which the expression of firefly luciferase coding sequence is driven by hypoxia response element (HRE). The HRE contains essential binding sites for HIF-1, which mediates increased transcription in cells that are either exposed to hypoxia or hypoxia mimics.Citation22 Hygromycin B was added at a final concentration of 200 μg/mL for 14 days to screen stable PC3-HRE-LUC cell line. PC3-HRE-LUC cells were seeded in a 12-well plate. The cells were treated with different concentrations of MNK for 6 h and the cell lysates were analyzed by the Luciferase Assay system (Promega) according to the manufacturer's instruction. The luciferase activities were reported as the firefly luciferase.
Quantitative real time PCR
Total RNA was extracted using TRIzol reagent (Invitrogen, Carlsbad, CA, USA) according to the manufacturer's instructions. RNA was treated with DNase (Promega). Complementary DNA was synthesized using the cDNA synthesis Kit (Takara) according to the manufacturer's instructions. Quantitative real time PCR (Q-PCR) was performed with the double-stranded DNA dye FastStart Universal SYBR Green Master reagents (ROX) (Roche) using the ABI 7300 system (Perkin-Elmer). PCR was performed in triplicate and standard deviations representing experimental errors were calculated. The relative mRNA expression was calculated using the 2−ΔΔCt method and the levels of β-actin were used as internal control. Primer pairs used for real-time PCR analysis include: HIF-1α forward 5′ –CTC AAA TAC AAG AAC CTA CTG CTA A-3′, reverse 5′-GTG ATG ATG TGG CAC TAG TA-3′; GLUT1 forward 5′- CTT TGT GGC CTT CTT TGA AGT-3′, reverse 5′-CCA CAC AGT TGC TCC ACA T-3′; CA9 forward 5′-GAC GCC TGG CCG TGT TG-3′, reverse 5′-CTG AGC CTT CCT CAG CGA TT-3′; β-actin forward 5′ –AGA GCT ACG AGC TGC CTG AC-3′, reverse 5′- AGC ACT GTG TTG GCG TAC A-3′.
Cell proliferation assay
Cytotoxicity of MNK to prostate cancer cells was examined by the CCK-8 assay. Cells were seeded in a 96-well culture plate at 5 × 104 /well and treated with different concentrations of MNK for 12 h and 24 h, then CCK-8 was added for further assay. The absorbance at 450 nm was measured using a Synergy H4 Hybrid Microplate Reader. The cell proliferation inhibition ratio (%) was calculated with the following formula: inhibition ratio = (ODcontrol – ODtreated)/ODcontrol × 100%.
Statistical analysis
The data are presented as the means ± SD of at least three independent experiments. Student's t-tests were performed using GraphPad Prism 5.0 software (GraphPad Software) to evaluate the difference between two different treatments while for multiple comparisons such as dose dependent effect, one-way ANOVA was used for statistical analysis. P < 0.05 was considered statistically significant.
Results
MNK inhibits HIF-1α activity
To screen inhibitors of HIF-1α, we engineered a reporter cell line PC3-HRE-LUC, which contains reporter gene for hypoxia-inducible expression of firefly luciferase. Exposure of PC3-HRE-LUC to hypoxia condition (1% O2) significantly increased the luciferase activity. Using this model, we found that MNK, a FDA approved drug for the treatment of asthma, significantly inhibited hypoxia-induced upregulation of the luciferase activity (). Consistent with this finding, MNK treatment decreased HIF-1α target genes (). These data demonstrated that MNK inhibited HIF-1α activity.
Figure 1. MNK inhibits HIF-1α activity. (A). PC3 cells stably transfected with pGL4.27-HRE-LUC were pretreated with different concentrations of MNK under hypoxia (1% O2) for 5 h, the luciferase activity was examined. (B). Q-PCR analysis of indicated HIF-1α target genes in LNCaP cells treated with 60 μM MNK under hypoxia for 5 h. Columns represent fold changes. Error bars indicate mean ± SD. ∗, P < 0.05; ∗∗∗, P < 0.001.
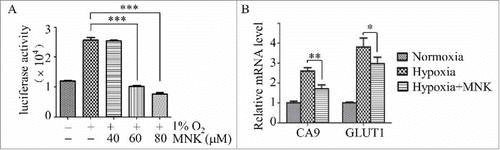
MNK decreases HIF-1α protein
In order to determine the possible mechanisms of MNK-induced inhibition of HIF-1α activity, we first investigated whether MNK could affect the protein level of HIF-1α. PC3 and LNCaP prostate cancer cells were treated with different concentrations of MNK in the presence of hypoxia (1% O2) or hypoxia mimic agent cobalt chloride (CoCl2). As shown in and , MNK decreased HIF-1α protein in a dose-dependent manner. To determine whether the decreased protein level of HIF-1α is due to its transcription inhibition, we treated the two cells with different concentrations of MNK under hypoxia for 6 h. HIF-1α mRNA was evaluated by Q-PCR. However, MNK did not decrease the mRNA of HIF-1α in PC3 and LNCaP prostate cancer cells (Fig. S1).
Figure 2. MNK decreases HIF-1α protein. PC3 cells (A) and LNCaP cells (B) were treated with the indicated concentrations of MNK under hypoxia (left) for 5 h or cobalt chloride for 6 h (right). Cell lysates were subjected to immunoblot assays for HIF-1α and β-tubulin. The experiments were repeated three times.
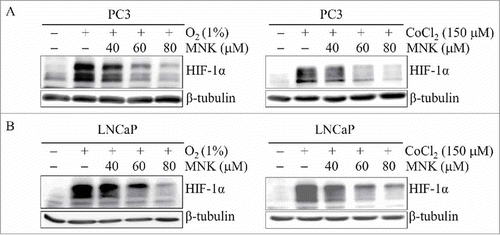
MNK-induced HIF-1α protein reduction is independent on protein degradation and leukotriene receptor
The most common degradation pathway of HIF-1α is the ubiquitin-proteasome pathway.Citation23–Citation25 However, proteasome inhibitor MG132 could not block MNK-induced reduction of HIF-1α in PC3 and ubiquitin was used as positive control of MG132 ( and Fig. S2A). Moreover, MNK also reduced HIF-1α protein in VHL deficient RCC4 cancer cells,Citation25 indicating that MNK-induced reduction of HIF-1α protein is independent of ubiquitin-proteasome pathway (Fig. S3). It is reported that HIF-1α is also degraded through autophagy-lysosomal pathway.Citation26 To test if MNK-induced HIF-1α reduction is through this pathway, we treated PC3 cells with lysosome inhibitor chloroquine (CQ) together with MNK. MNK could still reduce HIF-1α protein in PC3 cells and p62 was assayed as positive control of CQ, indicating that MNK-induced reduction of HIF-1α protein is independent of autophagy-lysosomal degradation pathway ( and Fig. S2B). Also, the half-life of HIF-1α protein was not changed after MNK treatment (Fig. S4). Taken together, these results indicate that MNK may decrease HIF-1α protein by a mechanism that does not involve inhibition of HIF-1α protein degradation.
Figure 3. MNK-induced HIF-1α protein reduction is independent on protein degradation and leukotriene receptor. PC3 cells were treated with MNK for 6 h in the presence or absence of MG132 (A) or CQ (B) in the presence of 150 μM CoCl2. The indicated proteins were examined by western blot. (C). PC3 cells were treated with different concentrations of zafirlukast and pranlukast for 6 h in the presence of 150 μM CoCl2. Cell lysates were subjected to immunoblot assays. β-tubulin was used as loading control. All experiments were repeated three times. Za, zafirlukast; Pra, pralukast.
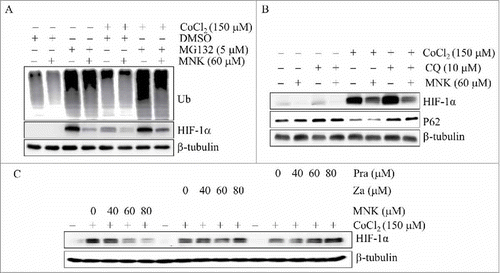
Because MNK is a leukotriene receptor antagonist, we assumed that MNK might inhibit HIF-1α through leukotriene receptor. To test this hypothesis, we treated PC3 cells with different concentrations of two other leukotriene receptor antagonists, pranlukast and zafirlukast, and examined their effects on the protein level of HIF-1α. However, pranlukast and zafirlukast does not decrease HIF-1α protein under hypoxia or in the presence of hypoxia mimics ( and Fig. S2C). These data indicate that MNK-induced downregulation of HIF-1α is independent of leukotriene receptor.
PERK is involved in MNK-induced reduction of HIF-1α
We found that MNK increased C/EBP homologous protein (CHOP) (data not shown), an endoplasmic reticulum stress marker,Citation27 we suspected that PKR-like Endoplasmic Reticulum Kinase (PERK, EIF2AK3) might be involved in this process. To test this hypothesis, we used GSK2606414, an inhibitor of PERK, to treat PC3 and LNCaP prostate cancer cells in the presence of MNK and CoCl2. GSK2606414 partially reversed MNK-induced HIF-1α inhibition in PC3 and LNCaP cells ( and Fig. S5A-5B). These data indicated that PERK participated in regulating the synthesis of HIF-1α.
Figure 4. PERK is involved in MNK-induced reduction of HIF-1α (A). PC3 cells were treated with 80 μM MNK in the presence of 150 μM CoCl2 for 6 h in the presence or absence of GSK2606414. The indicated proteins were examined by western blot. β-tubulin was used as loading control. The experiments were repeated three times.
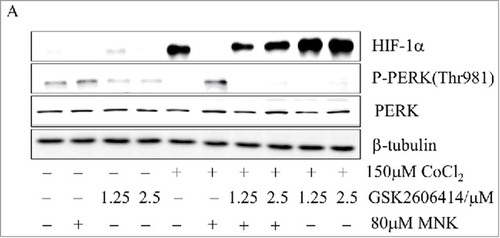
MNK induces phosphorylation of eIF-2α and inhibits translation of HIF-1α
Since GSK2606414 reversed MNK-induced HIF-1α inhibition, we examined the effect of MNK on PERK phosphorylation under hypoxia. The results showed that phosphorylation of PERK and eIF-2α were significantly induced (Fig. S6). The polypeptide chain translation initiation factor eIF2 is a frequent target of regulation and its activity is often rate-limiting for protein synthesis.Citation28 We found that MNK increased the phosphorylation level of eIF-2α in PC3 and LNCaP prostate cancer cells without changing the protein level of total eIF-2α in the presence of CoCl2 (-), indicating the inhibition of protein synthesis. Then the vector and EIF2S1-S51A overexpression PC3 cells were treated with or without MNK under hypoxia (1% O2) for 6 h. It demonstrated that the Ser51 phosphorylation of eIF-2α is necessary to the reduction of HIF-1α protein resulting from MNK treatment under hypoxic conditions (). So MNK induces Ser51 phosphorylation of eIF-2α and causes HIF-1α protein translation inhibition ().
Figure 5. MNK induces phosphorylation of eIF-2α and inhibits translation of HIF-1α PC3 or LNCaP cells were treated with indicated concentrations of MNK in the presence or absence of 150 μM CoCl2 or under hypoxia (1% O2) for 6 h, cell lysates were prepared and the indicated proteins were examined by western blot (A-C). (D). PC3 cells transfected with vector and EIF2S1-S51A plasmid then treated with 60 μM MNK under hypoxia (1% O2) for 6 h. Cell lysates were subjected to immunoblot assays. β-tubulin was used as loading control. (E). MNK leads to the phosphorylation of eIF-2α (Ser51), which in turn results in the inhibition of protein translation including HIF-1α.
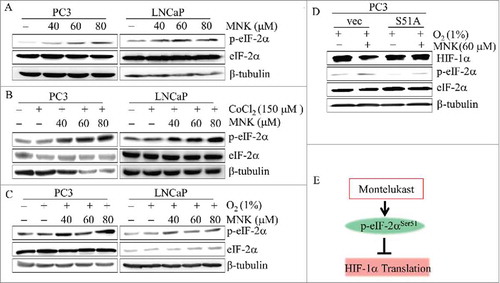
HIF-1α participates in MNK-induced proliferation inhibition of PC3 cells
Since HIF-1α has been reported to promote cancer progression and tumor growth,Citation29 we next investigate the effect of montelukast on the proliferation of prostate cancer cells. PC3 cells were treated with different concentrations of MNK for 24 hours, cell proliferation was evaluated by CCK-8 assay. As shown in , MNK showed inhibitory effects on the proliferation of PC3 cells. However, the same doses of MNK were not toxic to normal cell (Fig. S7). To determine the role of HIF-1α in the proliferation inhibitory effect of MNK, HIF-1α-P2A, a plasmid contains Pro-to-Ala missense mutations at the residues subjected to hydroxylation,Citation30 was transiently transfected into PC3 cells and the cells were treated with MNK. MNK-induced proliferation inhibition of PC3 cells was partially reversed by overexpression of HIF-1α-P2A (). Moreover, HIF-1α knockdown by short hairpin RNA significantly enhanced the effect of MNK on cell viability in the presence of CoCl2 (). These data indicate that HIF-1α is involved in the inhibitory effect of MNK in prostate cancer cells.
Figure 6. HIF-1α participates in MNK-induced proliferation inhibition of PC3 cells (A). PC3 cells were incubated with indicated concentrations of MNK for 12 h, and then cell proliferation was evaluated by CCK-8 assay. (B). PC3 cells transfected with HIF-1α-P2A were treated with MNK for 12 h and cell proliferation was evaluated by CCK-8 assay. (C). HIF-1α gene expression was reduced by short hairpin RNA (shRNA), and then the cells were treated with MNK in the presence of CoCl2. Then the cell proliferation was evaluated by CCK-8 assay. ∗, P < 0.05; ∗∗, P < 0.01. All experiments were repeated three times.
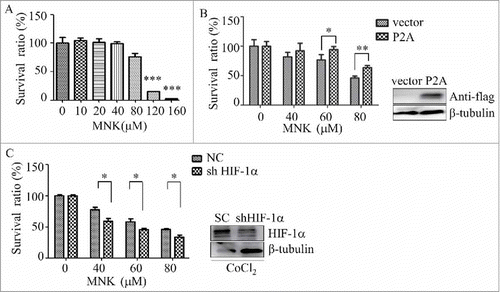
Discussion
HIF-1α, the master regulator of the cellular response to the hypoxia microenvironment, is increased in prostate cancerCitation15-Citation17 and is viewed as a viable prospective target to treat prostate cancer.Citation12 In the present study, we demonstrated that MNK, an anti-asthma drug, could reduce HIF-1α protein through inhibiting translation. This study provides novel insights into the anti-cancer effect of MNK.
The repurpose of old drug for new use is an attracting idea in the development of anticancer drugs. Many non-antitumor drugs have antitumor effects like thalidomide against multiple myeloma and metformin for inhibiting proliferation of ovarian and breast cancer.Citation31,Citation32 MNK, a cysteinyl-leukotriene receptor (Cys-LTR) antagonist, has long been used in the treatment of asthma and to relieve symptoms of seasonal allergic rhinitis. In the present study, we showed that MNK was a novel HIF-1α inhibitor and had anti-proliferation activity in prostate cancer cells. Consistent with our results, several groups also have reported that MNK and other Cys-LTR antagonists show activity against cancer cells.Citation33,Citation34 These data suggest MNK might be repurposed for the treatment of certain kinds of cancer. It has been reported that human is tolerant to a dose of MNK as high as 1000 mg. Therefore, it is deserved to verify the anti-cancer effect of MNK in preclinical and clinical trial in the future.
The role of HIF-1α in the anticancer activity of MNK has not been investigated previously. We found that MNK was a novel inhibitor of HIF-1α, which was supported by following evidences: MNK could inhibit the transcription activity of HIF-1α and repress its downstream target genes such as CA9 and GLUT1; MNK significantly reduced the protein level of HIF-1α. Moreover, we showed that overexpression of HIF-1α could partially reversed MNK-induced proliferation inhibition of prostate cancer cells. These data support a role of HIF-1α in the anti-cancer effect of MNK. However, we don't rule out other targets of MNK such as Cys-LTR, which contributes its anti-prostate cancer effect as described previously.Citation33
Regulating the proteasome-mediated degradation of HIF-1α is the major pathway in controlling HIF-1α protein level in cells. And some types of cancer cells such as RCC4 maintain high HIF-1α protein through disrupting the VHL-mediated degradation of HIF-1α. Interestingly, MNK reduces the HIF-1α protein through a degradation-independent pathway, as proteasome inhibitor or autophagy inhibitor could not reverse MNK-induced decrease of HIF-1α. Our finding that MNK reduces HIF-1α protein in VHL-deficient RCC4 cells also supports this idea. We further demonstrated that MNK-induced decrease of HIF-1α is associated with repressing of translation. It has been reported that the translation of HIF-1α could be regulated by mTOR signal pathway, and its activation results in phosphorylation of its substrates, such as eukaryotic initiation factor 4E-binding protein 1(4EBP1).Citation35,Citation36 Phosphorylation by mTOR on Thr70 prevents the binding of 4EBP1 to eIF4E, thus inhibiting translation of protein.Citation37 Rapamycin blocks HIF-1 protein synthesis by inhibiting mTOR signal pathway.Citation36 However, our results showed that MNK did not affect p-4EBP1 (Thr70) or total 4EBP1, indicating mTOR signal pathway may not be involved in MNK-induced inhibition of HIF-1α protein synthesis (Fig. S8).
Phosphorylation of eIF2, which is an important translation initiator, affects the GDP-GTP exchange rate, which is catalyzed by eIF2B thereby perturbing ternary complex(eIF2-GTP-Met tRNAi) formation and the recycling of eIF2, thus inhibiting global translation.Citation38,Citation39 MNK could increase the phosphorylation of eIF2 and therefore decrease overall protein synthesis rates, including HIF-1α. This may explain why overexpression of HIF-1α only partially reversed the effect of MNK on cell proliferation. There are several kinases responsible for the phosphorylation of eIF-2α, including heme-regulated eIF-2α kinase, RNA-dependent Protein Kinase (PKR, EIF2AK2), PKR-like Endoplasmic Reticulum Kinase (PERK, EIF2AK3) and General Control Non-derepressible 2 kinase (GCN2, EIF2AK4).Citation40 Because MNK could evoke endoplasmic reticulum stress, as indicated by the upregulation of CHOP, we deduced that PERK may be involved in the phosphorylation of eIF-2α. As expected, inhibition of PERK by GSK2606414 rescued MNK-induced HIF-1α degradation in PC3 and LNCaP prostate cancer cells. Taken together, we proposed that MNK inhibited the growth of prostate cancer cells by hindering the translation of HIF-1α via the increase of p-eIF-2α (Ser51).
In conclusion, we demonstrated that MNK could reduce the protein level of HIF-1α through repression of its protein translation. This study reveals novel Cys-LTR-independent action of MNK and provides novel insights into the anti-cancer effect of MNK.
Disclosure of Potential Conflicts of Interest
No potential conflicts of interest were disclosed.
2017CBT10784R-f07-z-bw.pptx
Download MS Power Point (4.4 MB)Additional information
Funding
References
- Kaelin WG, Jr., Ratcliffe PJ. Oxygen sensing by metazoans: the central role of the HIF hydroxylase pathway. Mol Cell. 2008;30:393–402. doi:10.1016/j.molcel.2008.04.009. PMID:18498744
- Wang GL, Jiang BH, Rue EA, Semenza GL. Hypoxia-inducible factor 1 is a basic-helix-loop-helix-PAS heterodimer regulated by cellular O2 tension. Proc Natl Acad Sci U S A. 1995;92:5510–4. doi:10.1073/pnas.92.12.5510. PMID:7539918
- Epstein AC, Gleadle JM, McNeill LA, Hewitson KS, O'Rourke J, Mole DR, Mukherji M, Metzen E, Wilson MI, Dhanda A, et al. C. elegans EGL-9 and mammalian homologs define a family of dioxygenases that regulate HIF by prolyl hydroxylation. Cell. 2001;107:43–54. doi:10.1016/S0092-8674(01)00507-4. PMID:11595184
- Bruick RK, McKnight SL. A conserved family of prolyl-4-hydroxylases that modify HIF. Science. 2001;294:1337–40. doi:10.1126/science.1066373. PMID:11598268
- Huang J, Zhao Q, Mooney SM, Lee FS. Sequence determinants in hypoxia-inducible factor-1alpha for hydroxylation by the prolyl hydroxylases PHD1, PHD2, and PHD3. J Biol Chem. 2002;277:39792–800. doi:10.1074/jbc.M206955200. PMID:12181324
- Maxwell PH, Wiesener MS, Chang GW, Clifford SC, Vaux EC, Cockman ME, Wykoff CC, Pugh CW, Maher ER, Ratcliffe PJ, et al. The tumour suppressor protein VHL targets hypoxia-inducible factors for oxygen-dependent proteolysis. Nature. 1999;399:271–5. doi:10.1038/20459. PMID:10353251
- Cockman ME, Masson N, Mole DR, Jaakkola P, Chang GW, Clifford SC, Maher ER, Pugh CW, Ratcliffe PJ, Maxwell PH, et al. Hypoxia inducible factor-alpha binding and ubiquitylation by the von Hippel-Lindau tumor suppressor protein. J Biol Chem. 2000;275:25733–41. doi:10.1074/jbc.M002740200. PMID:10823831
- Tanimoto K, Makino Y, Pereira T, Poellinger L. Mechanism of regulation of the hypoxia-inducible factor-1 alpha by the von Hippel-Lindau tumor suppressor protein. EMBO J. 2000;19:4298–309. doi:10.1093/emboj/19.16.4298. PMID:10944113
- Sadlecki P, Bodnar M, Grabiec M, Marszalek A, Walentowicz P, Sokup A, Zegarska J, Walentowicz-Sadlecka M. The role of Hypoxia-inducible factor-1 alpha , glucose transporter-1, (GLUT-1) and carbon anhydrase IX in endometrial cancer patients. Biomed Res Int. 2014;2014:616850. doi:10.1155/2014/616850. PMID:24745019
- McDonald PC, Dedhar S. Carbonic anhydrase IX (CAIX) as a mediator of hypoxia-induced stress response in cancer cells. Subcell Biochem. 2014;75:255–69. doi:10.1007/978-94-007-7359-2_13. PMID:24146383
- Ruan K, Song G, Ouyang G. Role of hypoxia in the hallmarks of human cancer. J Cell Biochem. 2009;107:1053–62. doi:10.1002/jcb.22214. PMID:19479945
- Melillo G. Targeting hypoxia cell signaling for cancer therapy. Cancer Metastasis Rev. 2007;26:341–52. doi:10.1007/s10555-007-9059-x. PMID:17415529
- Siegel RL, Miller KD, Jemal A. Cancer statistics, 2016. CA Cancer J Clin. 2016;66:7–30. doi:10.3322/caac.21332. PMID:26742998
- Attard G, Parker C, Eeles RA, Schroder F, Tomlins SA, Tannock I, et al. Prostate cancer. Lancet. 2016;387:70–82. doi:10.1016/S0140-6736(14)61947-4. PMID:26074382
- Zhong H, De Marzo AM, Laughner E, Lim M, Hilton DA, Zagzag D, Buechler P, Isaacs WB, Semenza GL, Simons JW, et al. Overexpression of hypoxia-inducible factor 1alpha in common human cancers and their metastases. Cancer Res. 1999;59:5830–5. PMID:10582706
- Talks KL, Turley H, Gatter KC, Maxwell PH, Pugh CW, Ratcliffe PJ, Harris AL. The expression and distribution of the hypoxia-inducible factors HIF-1alpha and HIF-2alpha in normal human tissues, cancers, and tumor-associated macrophages. Am J Pathol. 2000;157:411–21. doi:10.1016/S0002-9440(10)64554-3. PMID:10934146
- Zhong H, Hanrahan C, van der Poel H, Simons JW. Hypoxia-inducible factor 1alpha and 1beta proteins share common signaling pathways in human prostate cancer cells. Biochem Biophys Res Commun. 2001;284:352–6. doi:10.1006/bbrc.2001.4981. PMID:11394885
- Miranda E, Nordgren IK, Male AL, Lawrence CE, Hoakwie F, Cuda F, Court W, Fox KR, Townsend PA, Packham GK, et al. A cyclic peptide inhibitor of HIF-1 heterodimerization that inhibits hypoxia signaling in cancer cells. J Am Chem Soc. 2013;135:10418–25. doi:10.1021/ja402993u. PMID:23796364
- Sato M, Hirose K, Kashiwakura I, Aoki M, Kawaguchi H, Hatayama Y, Akimoto H, Narita Y, Takai Y. LW6, a hypoxia-inducible factor 1 inhibitor, selectively induces apoptosis in hypoxic cells through depolarization of mitochondria in A549 human lung cancer cells. Mol Med Rep. 2015;12:3462–8. doi:10.3892/mmr.2015.3862. PMID:26017562
- Manohar SM, Padgaonkar AA, Jalota-Badhwar A, Rao SV, Joshi KS. Cyclin-dependent kinase inhibitor, P276-00, inhibits HIF-1alpha and induces G2/M arrest under hypoxia in prostate cancer cells. Prostate Cancer Prostatic Dis. 2012;15:15–27. doi:10.1038/pcan.2011.51. PMID:22083267
- Mun J, Jabbar AA, Devi NS, Liu Y, Van Meir EG, Goodman MM. Structure-activity relationship of 2,2-dimethyl-2H-chromene based arylsulfonamide analogs of 3,4-dimethoxy-N-[(2,2-dimethyl-2H-chromen-6-yl)methyl]-N-phenylbenzenesulfonamide , a novel small molecule hypoxia inducible factor-1 (HIF-1) pathway inhibitor and anti-cancer agent. Bioorg Med Chem. 2012;20:4590–7. doi:10.1016/j.bmc.2012.04.064. PMID:22682301
- Semenza GL, Jiang BH, Leung SW, Passantino R, Concordet JP, Maire P, Giallongo A. Hypoxia response elements in the aldolase A, enolase 1, and lactate dehydrogenase A gene promoters contain essential binding sites for hypoxia-inducible factor 1. J Biol Chem. 1996;271:32529–37. doi:10.1074/jbc.271.51.32529. PMID:8955077
- Liu YV, Baek JH, Zhang H, Diez R, Cole RN, Semenza GL. RACK1 competes with HSP90 for binding to HIF-1alpha and is required for O(2)-independent and HSP90 inhibitor-induced degradation of HIF-1alpha. Mol Cell. 2007;25:207–17. doi:10.1016/j.molcel.2007.01.001. PMID:17244529
- Montagner M, Enzo E, Forcato M, Zanconato F, Parenti A, Rampazzo E, Basso G, Leo G, Rosato A, Bicciato S, et al. SHARP1 suppresses breast cancer metastasis by promoting degradation of hypoxia-inducible factors. Nature. 2012;487:380–4. doi:10.1038/nature11207. PMID:22801492
- Cheng J, Kang X, Zhang S, Yeh ET. SUMO-specific protease 1 is essential for stabilization of HIF1alpha during hypoxia. Cell. 2007;131:584–95. doi:10.1016/j.cell.2007.08.045. PMID:17981124
- Hubbi ME, Hu H, Kshitiz, Ahmed I, Levchenko A, Semenza GL. Chaperone-mediated autophagy targets hypoxia-inducible factor-1alpha (HIF-1alpha) for lysosomal degradation. J Biol Chem. 2013;288:10703–14. doi:10.1074/jbc.M112.414771. PMID:23457305
- Rozpedek W, Pytel D, Mucha B, Leszczynska H, Diehl JA, Majsterek I. The Role of the PERK/eIF2alpha/ATF4/CHOP Signaling Pathway in Tumor Progression During Endoplasmic Reticulum Stress. Curr Mol Med. 2016;16:533–44. doi:10.2174/1566524016666160523143937. PMID:27211800
- Sonenberg N, Hinnebusch AG. Regulation of translation initiation in eukaryotes: mechanisms and biological targets. Cell. 2009;136:731–45. doi:10.1016/j.cell.2009.01.042. PMID:19239892
- Li J, Shi M, Cao Y, Yuan W, Pang T, Li B, Sun Z, Chen L, Zhao RC. Knockdown of hypoxia-inducible factor-1alpha in breast carcinoma MCF-7 cells results in reduced tumor growth and increased sensitivity to methotrexate. Biochem Biophys Res Commun. 2006;342:1341–51. doi:10.1016/j.bbrc.2006.02.094. PMID:16516853
- Li J, Xu Y, Long XD, Wang W, Jiao HK, Mei Z, Yin QQ, Ma LN, Zhou AW, Wang LS, et al. Cbx4 governs HIF-1alpha to potentiate angiogenesis of hepatocellular carcinoma by its SUMO E3 ligase activity. Cancer Cell. 2014;25:118–31. doi:10.1016/j.ccr.2013.12.008. PMID:24434214
- Stewart AK. Medicine. How thalidomide works against cancer. Science. 2014;343:256–7. doi:10.1126/science.1249543. PMID:24436409
- Kumar S, Meuter A, Thapa P, Langstraat C, Giri S, Chien J, Rattan R, Cliby W, Shridhar V. Metformin intake is associated with better survival in ovarian cancer: A case-control study. Cancer. 2013;119:555–62. doi:10.1002/cncr.27706. PMID:23208739
- Matsuyama M, Hayama T, Funao K, Kawahito Y, Sano H, Takemoto Y, Nakatani T, Yoshimura R. Overexpression of cysteinyl LT1 receptor in prostate cancer and CysLT1R antagonist inhibits prostate cancer cell growth through apoptosis. Oncol Rep. 2007;18:99–104. PMID:17549353
- Savari S, Liu M, Zhang Y, Sime W, Sjolander A. CysLT(1)R antagonists inhibit tumor growth in a xenograft model of colon cancer. PLoS One. 2013;8:e73466. doi:10.1371/journal.pone.0073466. PMID:24039952
- Park JY, Jung HJ, Seo I, Jha BK, Suh SI, Suh MH, Baek WK. Translational suppression of HIF-1alpha by miconazole through the mTOR signaling pathway. Cell Oncol (Dordr). 2014;37:269–79. doi:10.1007/s13402-014-0182-8. PMID:25070654
- Laughner E, Taghavi P, Chiles K, Mahon PC, Semenza GL. HER2 (neu) signaling increases the rate of hypoxia-inducible factor 1alpha (HIF-1alpha) synthesis: Novel mechanism for HIF-1-mediated vascular endothelial growth factor expression. Mol Cell Biol. 2001;21:3995–4004. doi:10.1128/MCB.21.12.3995-4004.2001. PMID:11359907
- Gingras AC, Gygi SP, Raught B, Polakiewicz RD, Abraham RT, Hoekstra MF, Aebersold R, Sonenberg N. Regulation of 4E-BP1 phosphorylation: a novel two-step mechanism. Genes Dev. 1999;13:1422–37. doi:10.1101/gad.13.11.1422. PMID:10364159
- Wek RC, Jiang HY, Anthony TG. Coping with stress: eIF2 kinases and translational control. Biochem Soc Trans. 2006;34:7–11. doi:10.1042/BST0340007. PMID:16246168
- Molle G, Dugast JY, Duclohier H, Daumas P, Heitz F, Spach G. Ionophore properties of a synthetic alpha-helical transmembrane fragment of the mitochondrial H+ ATP synthetase of Saccharomyces cerevisiae. Comparison with alamethicin. Biophys J. 1988;53:193–203.
- Marciniak SJ, Garcia-Bonilla L, Hu J, Harding HP, Ron D. Activation-dependent substrate recruitment by the eukaryotic translation initiation factor 2 kinase PERK. J Cell Biol. 2006;172:201–9. doi:10.1083/jcb.200508099. PMID:16418533