ABSTRACT
TF/FVIIa (Tissue Factor/Active Coagulation factor VII) and EGFR (Epidermal Growth Factor Receptor) signaling both promote malignant progression of colorectal cancer. However, the crosstalk of these two signaling pathways in human colorectal cancer cells remains unclear. Here we detected the changes of mRNA profile in human colorectal cancer cell SW620 exposed to FVIIa. Microarray showed that mRNA levels of EGFR ligands were significantly upregulated. Western blot analysis confirmed the upregulation of EGFR ligands and the phosphorylation of EGFR at tyrosine-845 in colorectal cancer cells exposed to FVIIa. However, knockdown of TF by RNAi could block the upregulation of EGFR ligands induced by FVIIa stimulation. On the other hand, the expression of components of TF/FVIIa signaling was significantly upregulated in LoVo cells stimulated by EGF. However, the crosstalk between the two signaling pathways could not be detected in HT-29 colon cancer cells bearing wild-type KRAS. Taken together, our study suggest that the crosstalk between TF/FVIIa and EGFR signaling pathways in colon cancer cells depends on KRAS mutation.
Introduction
Targeted therapy is one of the most effective treatments for end-stage colorectal cancer. Epidermal growth factor receptor (EGFR) has been one of the most important targets for targeted therapies, and monoclonal antibodies cetuximab and panitumumab are the most commonly used drugs for colorectal cancer.Citation1 Survival of advanced colorectal cancer patients has been significantly improved, but drug resistance restricts the efficacy of anti-EGFR therapy.Citation2,Citation3 The proto-oncogene KRAS screening was conventionally employed to determine whether the patient should receive anti-EGFR therapy.Citation4,Citation5 It was speculated that mutations of downstream signaling of KRAS, such as BRAF-V600E, PI3K-CA and AKT, might be responsible for secondary resistance.Citation6,Citation7 The dissatisfactory effect of anti-EGFR therapy due to drug resistance suggests that other important pathways beyond EGFR signaling participate in the progression of cancer.
Tissue factor (TF) is highly expressed in many malignant solid tumors.Citation8–Citation10 Our previous study showed that high expression of TF in colorectal cancer was correlated with tumor invasion and metastasis, and TF and its ligand coagulation factor VII (FVIIa) could increase the expression of matrix metalloproteinase-7 (MMP-7) via mitogen-activated protein kinase (MAPK) signaling.Citation8,Citation11 The binding of TF and FVIIa could trigger intracellular signaling to promote cancer cell proliferation, invasion and migration.Citation12–Citation14 In particular, TF/FVIIa could phosphorylate EGFR and upregulate the expression of EGFR ligands in human HaCaT cells, while EGFR inhibitor could abrogate TF/FVIIa induced upregulation of EGFR ligands, indicating the interaction between EGFR and TF/FVIIa signaling.Citation14 Therefore, in this study we aimed to investigate the crosstalk between TF/FVIIa signaling and EGFR signaling in colorectal cancer.
Materials and methods
Cell culture
All colon cancer cell lines (SW620, LoVo, SW480, HCT116 and HT29) were obtained from American Type Culture Collection (ATCC) and cultured in Dulbecco modified Eagle medium (DMEM) with high glucose (Gibco) and 10% fetal bovine serum (Gibco) in a 5% CO2 incubator at 37°C.
Microarray assay
SW620 was cultured as two groups (control and FVIIa, 3 samples for each group), 100 nM FVIIa (Novo nordist, Denmark) was added to group FVIIa and the same volume of phosphate buffer solution (PBS) was added to group control when cells reached 60% confluence. Total RNA was extracted 24 h later. RNA samples were sent to Genminix Informatics (Shanghai, China) for microarray hybridization and statistical analysis. Genes of the fold change ≥ 2.0 between the two groups were screened, and 13 malignancy-related genes were selected to verify the results of microarray.
Real-time polymerase chain reaction
Primers were designed by Primer Express and their sequences were listed in . High Capacity cDNA Reverse Transcription Kit (Applied Biosystems, USA) was used for reverse transcription reaction to get cDNA from total RNA. 1.5 μL of cDNA was mixed with 7.5 μL of SYBR green master mix (Applied Biosystems, USA), 3 μL of nuclease-free water and 3 μL of primers. PCR was run on 7500 fast real-time PCR system (Applied Biosystems, USA) with 50°C for 2 min, 95°C for 10 min, 40 cycles of 95°C for 15 s, 60°C for 1 min. The relative expression levels were calculated with the Livak method (2[^-ΔΔCT]).
Table 1. Sequences of all primers used in this study.
Small interfering RNA (siRNA)
TF-siRNA and the corresponding negative control duplexes were designed and synthesized by Invitrogen. The sequences of TF-siRNA was as follows: sense strand: 5ʹ-GAACGGACUUUAGU CAGAAGGAACA-3; antisense strand: 5ʹ-UGUUCCUUCUGACUAAAGUCCG UUC-3ʹ. Sequence of the negative control was kept secret (Cat. 12935–300). siRNAs were transfected into cells according to the instruction.
Western blot analysis
Proteins were extracted from cells and subjected to Western blot analysis using routine protocols. Western Blot was employed to detect the relative expressions of Tyr845-phosphorylated EGFR. The experiment was repeated 3 times.
Statistical analysis
Data analyses were conducted by SPSS ver. 20.0 (SPSS Inc., Chicago, IL, USA). The data were presented as the mean± standard deviation (SD). Student’s t test and One-way ANOVA with Dunnet’s t test were used for pairwise comparisons and multiple comparisons. P < 0.05 was considered as statistically significant.
Results
Analysis of the changes of expression profile in colon cancer cells upon the activation of TF/FVIIa signaling
There were 49 differentially expressed genes with a test/control fold change of ≥ 2. Among them, 34 were up-regulated, including AREG, the ligand of EGFR, and 15 were downregulated). 13 cancer-related genes (up-regulated: EMP1, DUSP5, GDF15, IL-23A, SLC7A11, CCL-20 and IL-8; down-regulated: CAPN6, SMOC2, CDCA7, CA9, KIF20A and PLOD2) were selected to verify the result of microarray. Real-time PCR analysis of the up-regulated genes were consistent with those of the microarray, their relative mRNA expression levels were 0.97 ± 0.19 vs. 8.31 ± 0.21 (EMP1), 1.10 ± 0.26 vs. 12.33 ± 1.02 (DUSP5), 0.93 ± 0.21 vs. 4.15 ± 0.79 (GDF15), 1.10 ± 0.18 vs. 5.89 ± 0.50 (IL-23A), 0.93 ± 0.28 vs. 3.09 ± 0.50 (SLC7A11), 1.13 ± 0.31 vs. 5.93 ± 1.02 (CCL-20), 1.13 ± 0.28 vs. 3.48 ± 0.43 (IL-8) (all P<0.05). Among the chosen down-regulated genes, expression levels of CAPN6, SMOC2 and CDCA7 were consistent with those of microarray, their relative mRNA expression levels were 1.00 ± 0.22 vs. 0.60 ± 0.11, 1.00 ± 0.21 vs. 0.61 ± 0.13, 1.00 ± 0.08 vs. 0.65 ± 0.11, respectively (all P<0.05). Linear-regression analysis was used to further confirm the consistency of differentially expressed genes between microarray and qRT-PCR ().
Figure 1. Overview of differentially expressed genes in colon cells exposed to FVIIa. (A) Cluster dendrogram and volcano plot showed the general view of differentially expressed genes. (B) 13 malignancy-related genes were selected for qRT-PCR, and the results of 10 genes were consistent with the microarray. (C) Linear-regression analysis of the consistence of the results of microarray and qRT-PCR. *P<0.05, **P<0.01.
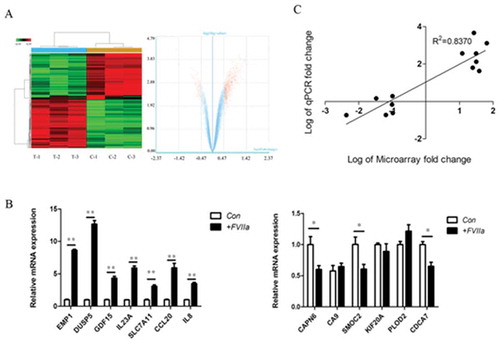
Network analysis of the results of microarray
Coexpression network analysis showed that laminin-integrin system was the most significant system correlated to TF/FVIIa signaling activation. Pathway network analysis showed that cancer related pathways were significantly changed when TF/FVIIa signaling was activated ().
Figure 2. Network analysis of differentially expressed genes. (A) Network of differentially expressed genes. Red spot indicated upregulated gene, blue spot indicated downregulated gene, the line between the spots indicated their correlation. (B) Network of differentially expressed genes related pathways. Red spot indicated upregulated pathway, blue spot indicated downregulated pathway, yellow spot indicated both upregulated and downregulated pathway, the line between the spots indicated their correlation.
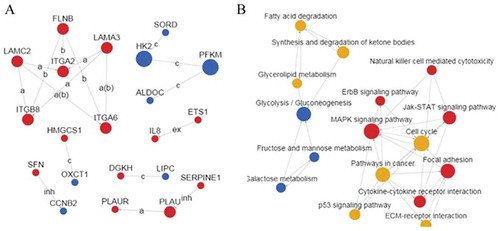
Upregulation of the expression of EGFR ligands in colon cancer cells
Microarray showed that AREG, EREG, HB-EGF and TGF-α were all upregulated following the activation of TF/FVIIa signaling. We then detected the ligands of EGFR in LoVo, SW620, SW480 and HCT116 colon cancer cell lines, including AREG, EREG, HB-EGF, TGF-α, BTC, EGF and EPGN. EGFR ligands were highly expressed in each of the cell lines. Among all seven ligands, AREG was the only one that was up-regulated in all four cell lines, indicating that AREG might play a principal role in promoting colorectal cancer cell malignant progression following FVIIa stimulation.
Knockdown of TF inhibited the upregulation of AREG expression in colon cancer cells stimulated by FVIIa
Next we used TF siRNA to knockdown TF in LoVo cells () and found that TF knockdown had no significant effect on endogenous AREG expression at mRNA and protein levels, but abolished FVIIa-induced upregulation of AREG expression at mRNA and protein levels ().
Figure 3. TF knockdown by siRNA blocked FVIIa induced AREG upregulation in LoVo cells. (A) TF expression was significantly downregulated in group TFi at mRNA and protein levels. (B) AREG expression was significantly upregulated in group FVIIa but downregulated in group TFi+ FVIIa. *P<0.05, **P<0.01.
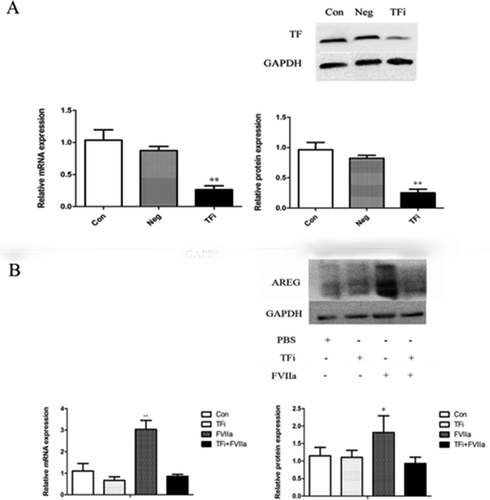
FVIIa stimulated the phosphorylation of EGFR in colon cancer cells
We detect the level of phosphorylation of EGFR on Tyr845 site, one of the most important phosphorylation sites for triggering EGFR pathway. We found significantly higher levels of Tyr845 phosphorylation at 3 and 10 min after FVIIa stimulation ().
EGF activated TF/FVIIa pathway in colon cancer cells harboring mutant-KRAS
To find whether EGF activated TF/FVIIa pathway in colon cells, we treated LoVo cells with EGF and found that the expression of downstream genes of TF/FVIIa pathway such as MMP7, ATG5 and PAR2 were significantly upregulated (). In addition, EGF induced the upregulation of FVII mRNA levels in a dose- and time- dependent manner ().
Figure 5. EGF activated TF/FVIIa pathway in LoVo cells. (A) Expression of downstream genes of TF/FVIIa pathway MMP7, ATG5 and PAR2 was significantly upregulated. (B) FVIIa expression was significantly increased by EGF in a dose and time dependent manner. **P<0.01.
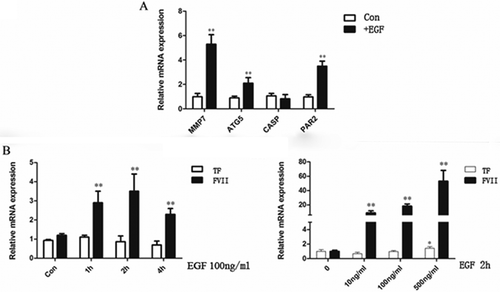
In contrast to LoVo cells, in colon cancer cell line HT-29 which bears wild-KRAS, FVIIa stimulation led to the downregulation of AREG and EREG expression (, ). In addition, EGF had no significant effect on the expression of TF and FVII ().
Figure 6. Comparison of the interaction of TF/FVIIa and EGFR pathways between LoVo (mutant KRAS) and HT-29 (wild-type KRAS) cells. (A) mRNA expression of EGFR ligands. (B) Protein expression of EGFR ligands. (C) EGF significantly increased mRNA expression of FVII in LoVo cells, but FVII mRNA was not detected in both control and EGF stimulated HT-29 cells. *P<0.05.
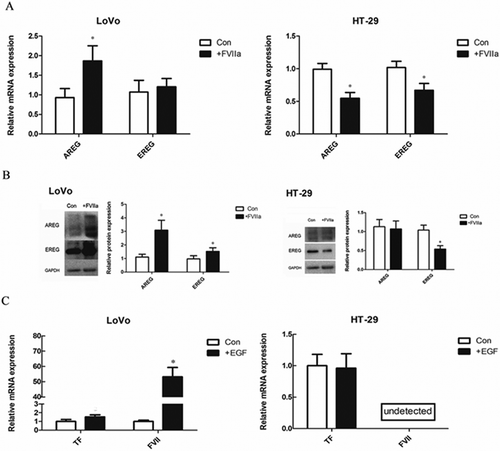
Discussion
In this study we showed that tumor-related genes were differentially expressed in colon cancer cells when TF/FVIIa pathway was activated. In particular, the expression of different ligands of EGFR was upregulated respectively in different colon cancer cells SW620, LoVo, SW480 and HCT116. Among them, AREG was upregulated in all four colon cancer cell lines, which indicated that AREG is commonly engaged in the regulation of EGFR pathway by TF/FVIIa signaling. FVII can be only expressed in liver cell under physical condition, however, it is ectopically highly expressed in pathologic conditions such as cancer.Citation15 For example, EGFRvIII, an aberrantly activated variant of EGFR, could promote the expression of FVII in human glioma cells.Citation16
We have previously reported that FVII was highly expressed in colorectal tumor tissues, and a high dose of FVII surrounding tumor cells might result in a tendency to migrate.Citation17 In this study, we found that the activation of EGFR pathway could upregulate TF expression in colon cancer cells. Therefore, understanding the crosstalk between EGFR pathway and TF/FVIIa pathway will help design better cancer therapy strategies targeting TF.Citation18–Citation22 In addition, KRAS mutation results in constitutive active Ras independent of the activation of EGFR, causing the failure of anti-EGFR therapy.Citation23,Citation24 In this study we found that the activation of EGFR pathway in LoVo cells with mutant KRAS could activate TF/FVIIa signaling, on the one hand, the activation of TF/FVIIa signaling could upregulate the expression of EGFR ligands to activate EGFR pathway, thus forming a positive feedback loop to promote malignant phenotypes of cancer cells.
Interestingly, we observed no crosstalk between TF/FVIIa and EGFR signaling pathways in colon cancer cells such as HT-29 cells harboring wide-type KRAS. We speculate that single targeted therapy is effective to kill these cancer cells. However, in colon cancer cells with mutant KRAS such as LoVo cells, the synergistic effect between EGFR and TF/FVIIa signaling may play a more important role than single EGFR pathway, thus therapeutic effects could be significantly improved using dual-targeting treatment aimed at both EGFR and TF in patients with mutant KRAS. Taken together, our study suggest that the crosstalk between TF/FVIIa and EGFR signaling pathways in colon cancer cells depends on KRAS mutation and present a novel strategy for the treatment of colon cancer with KRAS mutation.
Disclosure of Potential Conflicts of Interest
No potential conflicts of interest were disclosed.
Acknowledgments
This study was supported by the grants from the National Natural Science Foundation of China (No. 81272710) and National Natural Science Youth Foundation of China (No. 30801092).
Additional information
Funding
References
- Bertotti A, Papp E, Jones S, Adleff V, Anagnostou V, Lupo B, Sausen M, Phallen J, Hruban CA, Tokheim C, et al. The genomic landscape of response to EGFR blockade in colorectal cancer. Nature. 2015;526(7572):263–267. doi:10.1038/nature14969.
- Van Emburgh BO, Sartore-Bianchi A, Di Nicolantonio F, Siena S, Bardelli A. Acquired resistance to EGFR-targeted therapies in colorectal cancer. Mol Oncol. 2014;8(6):1084–1094. doi:10.1016/j.molonc.2014.05.003.
- Hynes NE, Lane HA. ERBB receptors and cancer: the complexity of targeted inhibitors. Nat Rev Cancer. 2005;5(5):341–354. doi:10.1038/nrc1609.
- Van Cutsem E, Kohne CH, Hitre E, Zaluski J, Chang Chien C-R, Makhson A, D’Haens G, Pintér T, Lim R, Bodoky G, et al. Cetuximab and chemotherapy as initial treatment for metastatic colorectal cancer. N Engl J Med. 2009;360(14):1408–1417. doi:10.1056/NEJMoa0805019.
- Sartore-Bianchi A, Di Nicolantonio F, Nichelatti M, Molinari F, De Dosso S, Saletti P, Martini M, Cipani T, Marrapese G, Mazzucchelli L, et al. Multi-determinants analysis of molecular alterations for predicting clinical benefit to EGFR-targeted monoclonal antibodies in colorectal cancer. PLoS One. 2009;4(10):e7287. doi:10.1371/journal.pone.0007287.
- Perkins G, Pilati C, Blons H, Laurent-Puig P. Beyond KRAS status and response to anti-EGFR therapy in metastatic colorectal cancer. Pharmacogenomics. 2014;15(7):1043–1052. doi:10.2217/pgs.14.66.
- Evaluation of Genomic Applications in Practice and Prevention(EGAPP) Working Group.. Recommendations from the EGAPP working group: can testing of tumor tissue for mutations in EGFR pathway downstream effector genes in patients with metastatic colorectal cancer improve health outcomes by guiding decisions regarding anti-EGFR therapy?. Genet Med. 2013;15(7):517–527. doi:10.1038/gim.2012.184.
- Jia ZC, Wan YL, Tang JQ, Dai Y, Liu Y-C, Wang X, Zhu J. Tissue factor/activated factor VIIa induces matrix metalloproteinase-7 expression through activation of c-Fos via ERK1/2 and p38 MAPK signaling pathways in human colon cancer cell. Int J Colorectal Dis. 2012;27(4):437–445. doi:10.1007/s00384-011-1351-0.
- Zhou H, Shi W, Zhou B, Guo D, Wang T. Tissue factor-factor VIIa regulates interleukin-8, tissue factor and caspase-7 expression in SW620 cells through protease-activated receptor-2 activation. Mol Med Rep. 2010;3(2):269–274. doi:10.3892/mmr_00000250.
- Carneiro-Lobo TC, Konig S, Machado DE, Nasciutti LE, Forni MF, Francischetti IMB, Sogayar MC, Monteiro RQ. Ixolaris, a tissue factor inhibitor, blocks primary tumor growth and angiogenesis in a glioblastoma model. J Thromb Haemost. 2009;7(11):1855–1864. doi:10.1111/j.1538-7836.2009.03553.x.
- Tian M, Wan Y, Tang J, Li H, Yu G, Zhu J, Ji S, Guo H, Zhang N, Li W, et al. Depletion of tissue factor suppresses hepatic metastasis and tumor growth in colorectal cancer via the downregulation of MMPs and the induction of autophagy and apoptosis. Cancer Biol Ther. 2011;12(10):896–907. doi:10.4161/cbt.12.10.17679.
- Versteeg HH. Tissue factor: old and new links with cancer biology. Semin Thromb Hemost. 2015;41(7):747–755. doi:10.1055/s-0035-1556048.
- Dorfleutner A, Hintermann E, Tarui T, Takada Y, Ruf W. Cross-talk of integrin alpha3beta1 and tissue factor in cell migration. Mol Biol Cell. 2004;15(10):4416–4425. doi:10.1091/mbc.e03-09-0640.
- Wiiger MT, Prydz H. The epidermal growth factor receptor (EGFR) and proline rich tyrosine kinase 2 (PYK2) are involved in tissue factor dependent factor VIIa signalling in HaCaT cells. Thromb Haemost. 2004;92(1):13–22. doi:10.1160/TH03-08-0549.
- Magnus N, Garnier D, Rak J. Oncogenic epidermal growth factor receptor up-regulates multiple elements of the tissue factor signaling pathway in human glioma cells. Blood. 2010;116(5):815–818. doi:10.1182/blood-2009-10-250639.
- Rong Y, Belozerov VE, Tucker-Burden C, Chen G, Durden DL, Olson JJ, Van Meir EG, Mackman N, Brat DJ. Epidermal growth factor receptor and PTEN modulate tissue factor expression in glioblastoma through JunD/activator protein-1 transcriptional activity. Cancer Res. 2009;69(6):2540–2549. doi:10.1158/0008-5472.CAN-08-1547.
- Tang JQ, Fan Q, Wan YL, Liu YC, Wang X, Wu T, Pan YS, Wu WH, Zhu J. Ectopic expression and clinical significance of tissue factor/coagulation factor VII complex in colorectal cancer. Beijing Da Xue Xue Bao Yi Xue Ban. 2009;41(5):531–536.
- Luo H, England CG, Shi S, Graves SA, Hernandez R, Liu B, Theuer CP, Wong HC, Nickles RJ, Cai W. Dual targeting of tissue factor and CD105 for preclinical PET imaging of pancreatic cancer. Clin Cancer Res. 2016;22(15):3821–3830. doi:10.1158/1078-0432.CCR-15-2054.
- Nielsen CH, Jeppesen TE, Kristensen LK, Jensen MM, El Ali HH, Madsen J, Wiinberg B, Petersen LC, Kjaer A. PET imaging of tissue factor in pancreatic cancer using 64Cu-labeled active site-inhibited factor VII. J Nucl Med. 2016;57(7):1112–1119. doi:10.2967/jnumed.115.170266.
- Shi S, Hong H, Orbay H, Graves SA, Yang Y, Ohman JD, Liu B, Nickles RJ, Wong HC, Cai W. ImmunoPET of tissue factor expression in triple-negative breast cancer with a radiolabeled antibody Fab fragment. Eur J Nucl Med Mol Imaging. 2015;42(8):1295–1303. doi:10.1007/s00259-015-3038-1.
- Zhu S, Kisiel W, Lu YJ, Petersen LC, Ndungu JM, Moore TW, Parker ET, Sun A, Sarkaria JN, Snyder JP, et al. Visualizing cancer and response to therapy in vivo using Cy5.5-labeled factor VIIa and anti-tissue factor antibody. J Drug Target. 2015;23(3):257–265. doi:10.3109/1061186X.2014.988217.
- Sato R, Obonai T, Tsumura R, Tsumoto K, Koga Y, Yasunaga M, Matsumura Y. Preparation and characterization of anti-tissue factor single-chain variable fragment antibody for cancer diagnosis. Cancer Sci. 2014;105(12):1631–1637. doi:10.1111/cas.12557.
- Hu J, Muller KA, Furnari FB, Cavenee WK, VandenBerg SR, Gonias SL. Neutralizing the EGF receptor in glioblastoma cells stimulates cell migration by activating uPAR-initiated cell signaling. Oncogene. 2015;34(31):4078–4088. doi:10.1038/onc.2014.336.
- Cheng L, Ren W, Xie L, Li M, Liu J, Hu J, Liu B-R, Qian X-P. Anti-EGFR MoAb treatment in colorectal cancer: limitations, controversies, and contradictories. Cancer Chemother Pharmacol. 2014;74(1):1–13. doi:10.1007/s00280-014-2489-6.