ABSTRACT
As a kinase at the crossroads of numerous metabolic and cell growth signaling pathways, glycogen synthase kinase-3 beta (GSK-3β) is a highly desirable therapeutic target in cancer. Despite its involvement in pathways associated with the pathogenesis of several malignancies, no selective GSK-3β inhibitor has been approved for the treatment of cancer. The regulatory role of GSK-3β in apoptosis, cell cycle, DNA repair, tumor growth, invasion, and metastasis reflects the therapeutic relevance of this target and provides the rationale for drug combinations. Emerging data on GSK-3β as a mediator of anticancer immune response also highlight the potential clinical applications of novel selective GSK-3β inhibitors that are entering clinical studies. This manuscript reviews the preclinical and early clinical results with GSK-3β inhibitors and delineates the developmental therapeutics landscape for this potentially important target in cancer therapy.
KEYWORDS:
Introduction
Glycogen synthase kinase-3 (GSK-3), a serine/threonine protein kinase with two functionally distinct isoforms, α and β, was discovered in the context of glycogen metabolism and emerged as a ubiquitous regulator of multiple signaling pathways ().Citation1 Tyrosine 216 and serine 9 phosphorylation prime the GSK-3 beta (GSK-3β) enzyme to either activation or inhibition, of its kinase properties, respectively.Citation2 Approximately, 100 proteins have been reported as phosphorylation targets of GSK-3β.Citation3 GSK-3β can serve as either a tumor suppressor by priming oncogene products for proteasomal destruction or as a pro-oncogene, mainly through proliferative pathways such as Wnt/β-catenin.Citation4 GSK-3 has recently been linked to increased programed cell death-1 (PD-1) expression and its inhibition enhanced T-cell response.Citation5,Citation6 The majority of inhibitors developed compete with ATP for GSK-3’s ATP-binding site with low selectivity. No selective GSK-3β inhibitor has been FDA approved for treatment of cancer or other diseases as of yet. This manuscript summarizes the evidence supporting GSK‐3β as a promising cancer therapeutic target and reviews the clinical development of GSK-3β inhibitors.
Figure 1. GSK-3β-mediated signaling pathways. When the Wnt ligand is present, it inhibits GSK-3β on the target cell, which suppresses β-catenin phosphorylation and makes it stable in cytoplasm. Stable β-catenin translocates into the nucleus promoting transcription of target genes such as c-Myc and cyclin-D. GSK-3β prevents epithelial–mesenchymal transition (EMT) by inhibiting Snail, a repressor of E-cadherin gene. GSK-3β phosphorylates several upstream and downstream components of the PI3K/AKT/mTOR pathway, and AKT can phosphorylate GSK-3β and inhibit its activity.
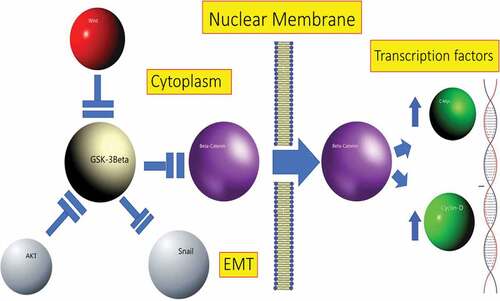
Rationale for therapeutic targeting of GSK-3β in cancer
GSK-3 has been described as a tumor suppressor in malignancies such as skin,Citation7,Citation8 breast,Citation9–Citation11 oral cavity,Citation12,Citation13 and lung cancers,Citation14,Citation15 and as a tumor promoter in other malignancies such as pancreas,Citation16–Citation19 colorectal,Citation20,Citation21 hepatocellular carcinoma,Citation22,Citation23 kidney,Citation24 leukemia,Citation25,Citation26 and glioblastoma multiforme (GBM).Citation27,Citation28
The role of GSK-3 in signaling pathways was initially described in the Wnt/β-catenin pathway. GSK-3-mediated β-catenin phosphorylation has a critical role in Wnt–β-catenin signaling. Wnt signaling inhibits GSK-3β, thus preventing β-catenin degradation and stabilizes β-catenin, which in turn increases transcriptional activity of c-Myc and cyclin-D.Citation29 GSK-3β modulates the double strand homologous repair pathway by phosphorylating FAAP2 (Fanconi Anemia-Associated Protein, 20 kDa), an important component of the Fanconi Anemia complex involved in the repair of DNA interstrand cross-links.Citation30 In GBM, GSK-3β mediates repair of DNA double strand-break through phosphorylation of 53BP1 (p53 binding protein 1).Citation31 GSK-3β can also phosphorylate several upstream and downstream components of the PI3K/AKT/mTOR pathway including AKT, RICTOR, and PTEN.Citation32 Moreover, the regulatory roles of GSK-3β in cell cycle,Citation33 apoptosis,Citation34 tumor invasion, and metastasis have been well described.Citation35,Citation36 GSK-3β also affects cellular responses to kinases.Citation37,Citation38
Regulation of GSK-3β by microRNAs (miRNAs), a class of noncoding small RNAs, has been implicated in cancer progression and treatment resistance. This interaction has been shown with multiple miRNAs in various malignancies including gastric cancer (miR-96, miR-182, and miR-183), colorectal cancer (miR-224), lung cancer (miR-26a), breast cancer (miR-1229), melanoma (miR-769), pancreatic cancer (miR-940), bladder cancer (miR-433), endometrial cancer (miR-129), ovarian cancer (let-7), and nasopharyngeal carcinoma (miR-15a).Citation8,Citation10,Citation18,Citation21,Citation39–Citation43
The cBio Cancer Genomics Portal dataset analysis reveals the landscape of GSK-3β genomic alterations among various cancer types ().Citation44,Citation45 These results highlight the rationale to target certain cancers such as myeloid, penile and colon neoplasms carrying GSK-3β gene mutation in about 15% of cases. Based on the search on Catalogue of Somatic Mutations in Cancer dataset, there are no available data on targeted drugs that have been used to treat GSK-3β mutant tumors (cancer.sanger.ac.uk).Citation46
Figure 2. Genomic alterations in GSK-3β. The landscape of GSK-3β genomic alterations among cancer types from the cBio Cancer Genomics Portal dataset.
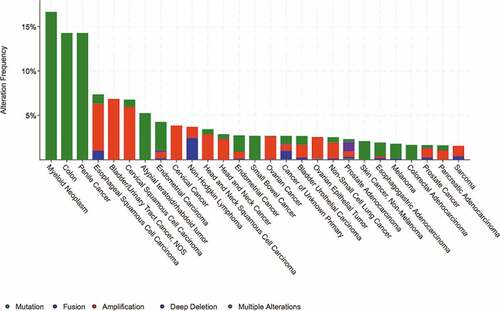
Mutations at GSK-3β phosphorylation sites prevent its ability to phosphorylate its substrates.Citation47 For instance, mutation in GSK-3β arginine 96 to alanine abrogates the ability of GSK-3 to phosphorylate pre-primed substrates.Citation48 Mutations in GSK-3 targets also limit the ability of GSK-3β to phosphorylate its substrates. Mutation of c-Myc Thr58 phosphorylation site blocks GSK-3-induced phosphorylation of c-Myc preventing its proteasomal degradation.Citation49 Similarly, genetic mutations changing the GSK-3 phosphorylation sites S33, S37, S45, or T41 present in β-catenin inhibited the GSK-3β-mediated phosphorylation of β-catenin.Citation47,Citation50 Thus, mutations at GSK-3 or its substrates can impact its kinase activity.Citation47
Cellular localization of GSK-3β also seems important to its signaling properties. Increased nuclear accumulation of GSK-3β has been demonstrated in a number of malignancies including breast, head and neck, pancreatic, renal, and bladder cancers.Citation51–Citation53 Nuclear GSK-3β accumulation is believed to enhance NF-κB binding to its target gene promoters increasing transcriptional activity of antiapoptotic molecules expression in cancer cells.Citation4,Citation54
GSK-3β role in specific cancers
Prostate cancer
GSK-3β is a positive regulator of androgen receptor (AR) transactivation and tumor growth independent of the Wnt/β-catenin pathway.Citation55 Compared to normal prostate tissue, GSK‐3β was upregulated in prostate cancer specimens, and cytoplasmic accumulation was associated with more aggressive clinicopathological features.Citation56,Citation57 TDZD-8, first non-ATP competitive inhibitor of GSK-3β, and L-803-mts, a substrate-competitive inhibitor, inhibited prostate cancer growth in preclinical models.Citation58 Recently, the kinase inhibitor 6-bromoindirubine-3′-monoxime decreased AR expression in prostate cancer cells via inhibition of both GSK-3α and GSK-3β.Citation59 The recruitment in a phase 1 study evaluating lithium, a known inhibitor of GSK-3 and its effect on localized prostate cancer, was completed with no published data as yetCitation60 (NCT02198859).
Kidney cancer
Several lines of evidence highlight the involvement of GSK-3β in the pathogenesis of renal cell carcinoma (RCC). Increased nuclear expression of GSK-3β was described in RCC and GSK-3β inhibition decreased the expression of NF-κB target genes Bcl-2 and XIAP leading to increased apoptosis in RCC models.Citation51,Citation61 GSK-3β also phosphorylated 4EBP1 leading to activation of mTORC1 downstream signaling cascades promoting cell proliferation in RCC and the effect was reversed by GSK-3 inhibition (AR-A014418).Citation62 Inhibition of GSK-3 also potentiated antitumor activity of gemcitabine and tyrosine kinase inhibitors sorafenib and gemcitabine in preclinical models of RCC.Citation63,Citation64 These results align with significant antitumor activity of 9-ING-41, a maleimide-based small molecule-specific GSK-3β inhibitor, in two xenograft models of RCC.Citation64
Pancreas cancer
Pancreatic cancers express aberrant nuclear accumulation of GSK-3β particularly in advanced disease state.Citation52,Citation65 Nuclear accumulation of GSK-3β regulates NF-κB transcriptional activity but exact mechanism is unknown. Possible mechanisms include direct interaction with NF-κB leading to effects on DNA binding for antiapoptotic genes or through increasing accessibility of NF-κβ through chromatin structure.Citation65 Ding et al.Citation66 demonstrated that GSK‐3β promotes TGF‐α‐induced acinar to ductal metaplasia and GSK‐3β deletion reduced pancreatic intraepithelial neoplasia progression in KrasG12D transgenic mice. Another possible mechanism inducing pancreatic cancer cell proliferation is GSK-3β-dependent SP2 phosphorylation which helps NFATc2 (nuclear factor of activated T cells), oncogenic transcription factor, and protein stability in the nucleus and maintains NFATc2 activation via stabilization of NFATc2–STAT3 complexes.Citation67 In a recent study, LY2090314, a selective ATP-competitive GSK-3 inhibitor, was evaluated for possible additive effect with nab-paclitaxel, gemcitabine, oxaliplatin, and SN-38.Citation68 Inhibition of GSK-3 enhanced the effect of chemotherapy in pancreatic cancer cells via modulation of stability of oncogenic effectors, yes-associated protein, and transcriptional coactivator with PDZ-binding motif (TAZ).Citation68 A phase 1/2 study of LY2090314 in combination with chemotherapy in advanced pancreatic cancer was conducted but was terminated due to slow enrollment (NCT01632306).
GBM
Higher expression and activity of GSK-3β have been documented in GBM cell lines compared to normal brain tissue.Citation69 Tumor expression of the active form of GSK-3β (pGSK3βy216) among 57 human tumor specimens was associated with worse progression-free survival and overall survival (OS) and multivariate analysis showed that activation of GSK-3β was an independent predictor of poor outcome.Citation70 Multiple mechanisms involving GSK-3β in GBM pathophysiology have been described.Citation69,Citation71 GSK-3β inhibition can cause c-Myc-dependent glioma cell cytotoxicity.Citation72 A study by Koru et al.Citation73 suggested that GSK-3 inhibition might specifically target a subpopulation of GBM with stem cell-like characteristics. To evaluate the potential role of GSK-3β in glioblastoma stem-like cells, protein profiles from patients and normal brain samples were analyzed in another study and results suggested that GSK-3β displays distinct roles based on EGFR expression/regulation and protein phosphatase 2A (PP2A) expression.Citation74
Interestingly, GSK-3β had a tumor suppressor role in GBM cells with EGFR overexpression, while constitutively active GSK-3β in PP2A overexpression led to an oncogenic role. Several studies showed that GSK-3β inhibition attenuates the proliferation and invasion of GBM cancer cells and sensitizes them to chemotherapeutic agents and radiation.Citation69,Citation72,Citation75,Citation76 A recent study demonstrated that transcriptional targets of GSK-3β regulate GBM invasion and suggested potential therapeutic targets.Citation77 Ugolkov et al. showed that GSK-3β inhibitor 9-ING-41 in combination with lomustine, one of the standard therapies for recurrent GBM, enhanced antitumor activity in PDX models of human GBM.Citation78 The effect of 9-ING-41 was shown in staged orthotopic GBM12 and GBM6 PDX models, which had minimal response to single agent lomustine. Importantly, combination treatment resulted in histologically confirmed cures with significant increase in OS.
A phase 1/2 study evaluated a combination of drugs with GSK-3β-inhibitory activity (i.e., cimetidine, lithium, olanzapine, and valproate—CLOVA cocktail) plus temozolomide among 20 patients with recurrent GBM.Citation70 Preclinical studies had demonstrated that CLOVA cocktail inhibited GSK-3β activity in GBM cells. CLOVA cocktail in combination with temozolomide showed increased survival in recurrent GBM patients compared to the control group treated with temozolomide alone (median OS 11.2 vs. 4.3 months; p = 0.004).Citation70 While limited by the lack of specificity of multiple inhibitors and small number of patients, this phase 1/2 study supports the therapeutic potential of GSK-3β inhibitors in GBM in combination with temozolomide. In fact, preclinical studies had shown that GSK-3β inhibition increased sensitivity of temozolomide in GBM by silencing methylguanine DNA methyltransferase expression via c-Myc-mediated promoter methylation.Citation76
Acute myeloid leukemia
GSK-3β has been involved in the signaling pathways of hematologic malignancies particularly acute myeloid leukemia (AML) given its regulation of Wnt/β-catenin pathway which is associated with drug resistance in AML.Citation79 Recent data suggested that GSK-3β localization has clinical significance with worse patient survival and mediates drug resistance in both in vitro and in vivo.Citation80 GSK-3β is also upregulated in natural killer (NK) cells from AML patients compared to normal donors.Citation81 Same study showed that GSK-3β overexpression in NK cells impairs their ability to kill AML cells, while genetic or pharmacological GSK-3β inhibition enhances NK cell cytotoxic activity in human AML mouse models. Gupta et al.Citation82 showed that all-trans retinoic acid (ATRA) receptor is a target of GSK-3β and its inhibition with combination of ATRA significantly enhances ATRA-mediated AML differentiation and growth inhibition. Other studies also suggested GSK-3β as promising target in AML cells since inhibition of this kinase leads to the growth inhibition and differentiation of leukemic cells.Citation33,Citation81 These results provided the rationale for a phase 2 study evaluating the GSK-3 inhibitor LY2090314 as monotherapy among 20 patients with AML that showed an acceptable safety profile but no clinical responses.Citation83 A transient increase in the levels of β-catenin following treatment was observed in peripheral blood mononuclear cell (PBMC)s and blast cells suggesting target inhibition, but levels returned to baseline by 24 h suggesting limited exposure to the drug. Most frequent drug-related adverse events included decreased appetite, nausea, dry mouth, dyspepsia, fatigue, and prolonged QT interval (8/19 AEs were Grade 3/4). Possibly drug-related visual disturbances were reported in two patients (blurred vision, flashing lights). No further studies are being pursuing with LY2090314 in AML.
GSK-3β inhibitors
Several GSK-3β inhibitors have been developed and advanced to early stage clinical trials including multiple cancer histologies.Citation84
Tideglusib, an ATP noncompetitive GSK-3β inhibitor, originally developed to decrease tau phosphorylation in Alzheimer disease, increases proapoptotic proteins in murine models of human neuroblastoma.Citation85 Tideglusib also decreased colony formation and increased G0/G1 population in tumor cells. One study showed that tideglusib sensitizes intracranial glioblastoma xenografts to temozolomide with improvement in mouse survival.Citation86 Tideglusib also increased NK cell cytotoxic activity in human AML mouse models.Citation81 The drug was tested in phase 2 studies for Alzheimer’s disease and progressive supranuclear palsy and was found to be well tolerated.Citation87,Citation88 To our knowledge, there is no active cancer clinical trials with tideglusib.
Antiproliferative effects of LY2090314, ATP-competitive GSK-3 inhibitor, were observed in preclinical studies for melanoma and neuroblastoma.Citation89,Citation90 A phase 1 study found that LY2090314 could be dosed at a safe and tolerable level in patients with cancer.Citation91 Signal of antitumor activity was observed in combination with pemetrexed and carboplatin in patients with mesothelioma, non-small cell lung, and breast cancers. An open-label phase 2 study showed that the drug was well tolerated with minimal non-hematologic side effects (i.e., nausea and decreased appetite) found in 11 out of 20 patients.Citation83 Hematologic effects were minimal but included anemia, thrombocytopenia, and neutropenia. None of the patients in the study had complete or partial remission making LY2090314 likely a poor candidate as single agent but combination treatments might be of interest in future studies.
Solasodine, a naturally occurring aglycone of glycoalkaloid, displayed antitumor activity in different cancers by inhibiting the GSK-3β pathway and inducing apoptosis.Citation92,Citation93 AR-A014418, a highly specific GSK-3β inhibitor, enhanced the cytotoxic effects of temozolomide and gemcitabine in models of GBM and pancreatic cancer, respectively.Citation75 A preclinical study using AR-A014418 demonstrated that inhibition of GSK-3 decreased gastric cancer cell survival and proliferation through decreasing telomerase reverse transcriptase expression and telomerase activity.Citation94 Clinical studies are warranted to investigate safety and efficacy of AR-A014418.
9-ING-41 is a small ATP-competitive GSK-3 inhibitor molecule which inhibits both α and β isoforms but more selective for GSK-3β than for 320 other related kinases.Citation24 In renal cancer, GSK-3β inhibition by 9-ING-41 decreased proliferation via G0–G1 and G2-M phase arrest and induced autophagy by altering glucose metabolism.Citation24 The study also showed the antitumor activity of 9-ING-41 in two xenograft models of RCC. In addition to its antiproliferative effects, 9-ING-41 decreased tumorigenicity of cancer cells by changing their phenotype from mesenchymal state to epithelial direction via increased Ksp-cadherin level and decrease Id-1 level. Similarly, another study demonstrated that 9-ING-41 significantly enhances the cytotoxicity of gemcitabine and cabozantinib in RCC cell lines.Citation64
GSK-3β inhibition in breast cancer cells in vitro decreased cancer cell survival by suppressing the expression of antiapoptotic proteins Bcl-2 and XIAP and enhanced tumor response to irinotecan in vivo.Citation95 The study also demonstrated that 9-ING-41 provided more potent inhibition of tumor growth than other tested GSK-3 inhibitors including AR-A014418, SB-216763, and LY2090314.
Constitutively active NF-κβ has been a hallmark feature of chemotherapy-resistant glioblastoma, making GSK-3β inhibition a particularly promising target. In GBM models, single agent 9-ING-41 showed modest antitumor activity but combination with lomustine led to significant tumor regression and survival benefit.Citation78 Similar to breast cancer models, results from GBM models suggested that potential additive effect form the combination of 9-ING-41 and chemotherapy.
Antitumor effect of 9-ING-41 was also observed in animal models of neuroblastoma.Citation96 GSK-3 inhibition decreased expression of the NF-κB target XIAP leading to apoptosis. Mouse models showed that combination of 9-ING-41 with irinotecan provided a significant antitumor effect. Compared to other GSK-3 inhibitors AR-A014418 and TDZD-8, 9-ING-41 was found to be the most potent inhibitor. These results provided the support for FDA granting Rare Pediatric Disease Designation to 9-ING-41, critical to advance the clinical development in the setting of this rare and devastating malignancy.
9-ING-41 also showed antiproliferative activity in B-cell lymphoma models where it reduced cell viability of up to 70%.Citation97 GSK-3β inhibition enhanced apoptosis in aggressive B-cell lymphoma lines through downregulation of pro-survival molecules. Combinations with BCL-2 inhibitor venetoclax and a CDK-9 inhibitor (BAY-1143572) enhanced the antitumor activity of 9-ING-41 and reduced IC50 values significantly. These results provide foundational rationale to further investigate this agent in advanced non-Hodgkin’s lymphoma.
A phase I study designed to evaluate the safety and clinical efficacy of 9-ING-41 as a single agent and in combination with cytotoxic agents in patients with advanced malignancies is underway (NCT03678883).
GSK-3β regulation of immune checkpoints
Recent publications highlight the role of GSK-3β in regulating the immune response.
Gattinoni et al. described how effector T CD8+ cells are able to dedifferentiate into stem cells through GSK-3β inhibition.Citation98 GSK-3β was shown to regulate the Wnt/β-catenin pathway, which coordinates stemness through its TCF/LEF family of transcription factors by a gene expression program that suppresses differentiation in a subset of T cells. By blocking T-cell differentiation via GSK-3β inhibition, Wnt signaling was able to generate multipotent CD8+ memory stem cells with antitumor capacities. Moreover, GSK-3β inhibition enhanced the antitumoral capacity of CD8+ memory stem T cells both in vitro and in vivo via FAS ligand neutralization in a gastric cancer study.Citation99 Similarly, inhibition of GSK-3β in a model of GBM-specific CAR-T cells increased survival and memory phenotype generation with enhanced tumor-killing ability in GSK-3β-inhibited IL13CAR-T cells.Citation100,Citation101 These results suggest that GSK-3 inhibitor could enhance antitumor response of T cells and improve CAR-T-cell treatments.
PD-L1 and PD-L2 expressed by tumors bind to PD-1 in the T CD8+ cells leading to immune system evasion. Taylor et al. identified GSK-3β as a key upstream kinase regulating PD-1 expression in CD8+ T cells and its inhibition blocked PD-1 expression, resulting in increased CTL function.Citation5 In the presence of anti-PD-1, SHP-1/2 phosphorylates the CD28 YMNM motif which leads the recruitment of PI3K. PI3K phosphorylates and inhibits GSK-3α (Ser21) and GSK-3β (Ser9) by AKT. Ultimately, inhibition of GSK-3 upregulates the transcription of the transcription factor Tbx21 (Tbet) which inhibits PD-1 expression (). Thus, the study suggested the following mechanistic model: GSK-3 inhibition increases Tbx21 transcription leading to enhanced T-bet expression which represses PD-1 expression and increases T-cell killing. Moreover, it was recently discovered that the rescue of exhausted CD8+ T cells by anti-PD-1 blockade requires CD28 expressionCitation102 and inactivation of GSK-3β was shown to substitute CD28 co-stimulation in priming of cytotoxic CD8+ T cells.Citation81,Citation103 GSK-3 inhibition reversed the effects of CD28 blockade with CTLA-4-IgG in the cytotoxic response of CTLs. These results suggest a potential role of GSK-3 inhibition as a strategy to help restore exhausted CD8+ T cells.
Figure 3. Proposed model for regulation of PD-1 by GSK-3β signaling. In the presence of anti-PD-1, activation of Src homology region domain-containing phosphatase (SHP) is inhibited, thus allowing for the phosphorylation of the CD28 phosphoinositide 3-kinase (PI3K)-binding site which leads GSK-3β inhibition via AKT activation. GSK-3β induces the transcription of the transcription factor Tbx21 (Tbet) which in turn inhibits transcription and expression of PD-L1.
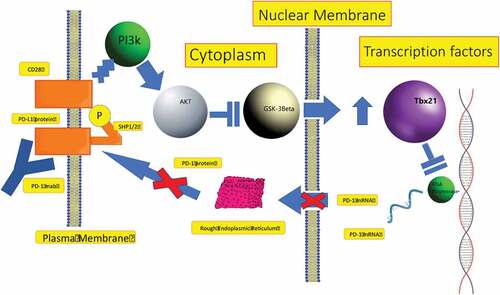
Taylor et al. investigated further how GSK-3 inhibition could downregulate PD-1 on T cells. GSK-3 inhibition decreased tumor growth and metastasis by downregulating PD-1 on CD8+ T cells in model of melanoma, while having a minimal effect on NK cells without obvious effect on CD4+ T cells.Citation6 The effect was similar to anti-PD-1 pretreatment. Importantly, GSK-3 inhibitors did not show further inhibition of tumor growth in Pdcd1−/− mice (PD-1 deficient). Anti-PD-1 treatment did not inhibit tumor growth in GSK-3α/β−/− mice supporting the hypothesis that antitumor activity from GSK-3 inhibition was mediated by downregulation of anti-PD-1. Similar results were observed in lymphoma model. Although combination therapy did not have an additional effect compared to monotherapy, this study raises an important question whether GSK inhibitors could have comparable effect to anti-PD1 inhibitors in some models or could be used in combination with other checkpoint inhibitors in the future.
In addition to its pivotal role in PD-1 pathway, the potential role GSK-3β in PD-L1 pathway was demonstrated in a breast cancer study.Citation104 The study showed that PARP inhibition increased PD-L1 expression primarily through GSK-3β inactivation. The study also demonstrated that PARP inhibition induced GSK-3β Ser9 phosphorylation which was associated with increased PD-L1 expression. Knocking out GSK-3β had similar observation. Interestingly, PARP inhibition did not increase PD-L1 expression level in GSK-3β-knockout cells which suggest that inactivation of GSK-3β is required for the PARP inhibitor-induced PD-L1 expression. More importantly, anti-PD-L1 treatment was able to sensitize PARP inhibitor-treated cancer cells to T-cell killing. Combination of PARP inhibitor with anti-PD-L1 treatment was shown to be more effective than each treatment alone suggesting the therapeutic potential of such combinations. It might be particularly relevant to tumors with mutations in DNA repair genes (i.e., breast, ovarian, and prostate cancers) known to be sensitive to PARP inhibitors but developing resistance to such treatment in relatively short period.
The role of GSK-3 in NK cells was also described. GSK-3 overexpression impaired NK cell ability to kill AML cells, while GSK-3 inhibition enhances NK cell cytotoxic activity in human AML mouse models.Citation81 In support of this data, a recent study explored inhibition of GSK-3 kinase in peripheral blood NK cells and expanded these cells ex vivo. GSK-3 inhibition caused elevated expression of several transcription factors, which are associated with NK cell maturation. Transferring conditioned NK cells to an established mouse xenograft model of ovarian cancer provided more robust and durable tumor control.Citation105
Future directions
GSK-3β is evolutionary an ancient protein that serves different roles in the cell as it is centrally located at the watershed of several signaling and metabolic pathways.
The evolution of precision medicine will move in parallel with the finding of new inhibitors. While the efficacy of new agents is proven in vivo, biomarkers from refined cancer pathways will inform the best molecular scenario in a continuous bench-to-bedside feedback loop. As an example, the absence of Myc mutations as the major player in c-Myc overexpression led scientists to focus upstream, revealing GSK-3 as a potential target in Myc overexpressing tumors.
Based on its interactions with apoptosis and chromatin remodeling process, combination strategies of GSK-3β inhibitors with Bcl-2 inhibitors, HDAC, hypomethylating agents, and PARP inhibitors have therapeutic relevance. New agents may be found while targeting bromodomains, chromodomains, Tudor, and PWWP domains, and their relationship to NF-κB (renal, pancreas) and STAT3 (pancreatic adenocarcinoma) transcription machinery may further our understanding of GSK-3 signaling.
GSK-3β inhibitors may also have an important role in regulating epithelial–mesenchymal transition and therefore metastasis control, especially in prostate and bladder cancers engendered by the Snail/E-cadherin pathway, which warrants further studies.
Finally, the understanding of GSK-3β role in regulating anticancer immune response is evolving rapidly with promising preclinical results. Particularly, dissecting the mechanisms of GSK-3β in PD-1 regulation may lead to future clinical trials with evaluation of GSK-3β inhibitors with checkpoint inhibitors.
Conflict of interest
The authors declare no potential conflicts of interest except Francis J. Giles who is a consultant and shareholder in Actuate Therapeutics Inc.
References
- Woodgett JR. Molecular cloning and expression of glycogen synthase kinase-3/factor A. EMBO J. 1990;9:2431–2438.
- Patel P, Woodgett JR. Glycogen synthase kinase 3: a kinase for all pathways? Curr Top Dev Biol. 2017;123:277–302. doi:10.1016/bs.ctdb.2016.11.011.
- Beurel E, Grieco SF, Jope RS. Glycogen synthase kinase-3 (GSK3): regulation, actions, and diseases. Pharmacol Ther. 2015;148:114–131. doi:10.1016/j.pharmthera.2014.11.016.
- Walz A, Ugolkov A, Chandra S, Kozikowski A, Carneiro BA, O’Halloran TV, Giles FJ, Billadeau DD, Mazar AP. Molecular pathways: revisiting glycogen synthase Kinase-3beta as a target for the treatment of cancer. Clin Cancer Res. 2017;23:1891–1897. doi:10.1158/1078-0432.CCR-15-2240.
- Taylor A, Harker JA, Chanthong K, Stevenson PG, Zuniga EI, Rudd CE. Glycogen synthase kinase 3 inactivation drives t-bet-mediated downregulation of co-receptor PD-1 to enhance CD8(+) cytolytic T cell responses. Immunity. 2016;44:274–286. doi:10.1016/j.immuni.2016.01.018.
- Taylor A, Rothstein D, Rudd CE. Small-molecule inhibition of PD-1 transcription is an effective alternative to antibody blockade in cancer therapy. Cancer Res. 2018;78:706–717. doi:10.1158/0008-5472.CAN-17-0491.
- Ma C, Wang J, Gao Y, Gao T-W, Chen G, Bower KA, Odetallah M, Ding M, Ke Z, Luo J. The role of glycogen synthase kinase 3beta in the transformation of epidermal cells. Cancer Res. 2007;67:7756–7764. doi:10.1158/0008-5472.CAN-06-4665.
- Qiu H-J, Lu X-H, Yang S-S, Weng C-Y, Zhang E-K, Chen F-C. MiR-769 promoted cell proliferation in human melanoma by suppressing GSK3B expression. Biomed Pharmacother. 2016;82:117–123. doi:10.1016/j.biopha.2016.04.052.
- Farago M, Dominguez I, Landesman-Bollag E, Xu X, Rosner A, Cardiff RD, Seldin DC. Kinase-inactive glycogen synthase kinase 3beta promotes Wnt signaling and mammary tumorigenesis. Cancer Res. 2005;65:5792–5801. doi:10.1158/0008-5472.CAN-05-1021.
- Tan Z, Zheng H, Liu X, Zhang W, Zhu J, Wu G, Cao L, Song J, Wu S, Song L, et al. MicroRNA-1229 overexpression promotes cell proliferation and tumorigenicity and activates Wnt/beta-catenin signaling in breast cancer. Oncotarget. 2016;7:24076–24087. doi:10.18632/oncotarget.8119.
- Shen H, Li L, Yang S, Wang D, Zhong S, Zhao J, Tang J. MicroRNA-29a contributes to drug-resistance of breast cancer cells to adriamycin through PTEN/AKT/GSK3beta signaling pathway. Gene. 2016;593:84–90. doi:10.1016/j.gene.2016.08.016.
- Mishra R, Nagini S, Rana A. Expression and inactivation of glycogen synthase kinase 3 alpha/beta and their association with the expression of cyclin D1 and p53 in oral squamous cell carcinoma progression. Mol Cancer. 2015;14:20. doi:10.1186/s12943-014-0278-9.
- He K, Tong D, Zhang S, Cai D, Wang L, Yang Y, Gao L, Chang S, Guo B, Song T, et al. miRNA-99b-3p functions as a potential tumor suppressor by targeting glycogen synthase kinase-3beta in oral squamous cell carcinoma Tca-8113 cells. Int J Oncol. 2015;47:1528–1536. doi:10.3892/ijo.2015.3135.
- Zheng H, Saito H, Masuda S, Yang X, Takano Y. Phosphorylated GSK3beta-ser9 and EGFR are good prognostic factors for lung carcinomas. Anticancer Res. 2007;27:3561–3569.
- Lin G, Liu B, Meng Z, Liu Y, Li X, Wu X, Zhou Q, Xu K. MiR-26a enhances invasive capacity by suppressing GSK3beta in human lung cancer cells. Exp Cell Res. 2017;352:364–374. doi:10.1016/j.yexcr.2017.02.033.
- Garcea G, Manson MM, Neal CP, Pattenden CJ, Sutton CD, Dennison AR, Berry DP. Glycogen synthase kinase-3 beta; a new target in pancreatic cancer? Curr Cancer Drug Targets. 2007;7:209–215.
- Marchand B, Arsenault D, Raymond-Fleury A, Boisvert F-M, Boucher M-J. Glycogen synthase kinase-3 (GSK3) inhibition induces prosurvival autophagic signals in human pancreatic cancer cells. J Biol Chem. 2015;290:5592–5605. doi:10.1074/jbc.M114.616714.
- Yang H-W, Liu G-H, Liu Y-Q, Zhao H-C, Yang Z, Zhao C-L, Zhang X-F, Ye H. Over-expression of microRNA-940 promotes cell proliferation by targeting GSK3beta and sFRP1 in human pancreatic carcinoma. Biomed Pharmacother. 2016;83:593–601. doi:10.1016/j.biopha.2016.06.057.
- Zhou W, Li Y, Gou S, Xiong J, Wu H, Wang C, Yan H, Liu T. MiR-744 increases tumorigenicity of pancreatic cancer by activating Wnt/beta-catenin pathway. Oncotarget. 2015;6:37557–37569. doi:10.18632/oncotarget.5317.
- Shakoori A, Mai W, Miyashita K, Yasumoto K, Takahashi Y, Ooi A, Kawakami K, Minamoto T. Inhibition of GSK-3 beta activity attenuates proliferation of human colon cancer cells in rodents. Cancer Sci. 2007;98:1388–1393. doi:10.1111/j.1349-7006.2007.00545.x.
- Li T, Lai Q, Wang S, Cai J, Xiao Z, Deng D, He L, Jiao H, Ye Y, Liang L, Ding, Y. MicroRNA-224 sustains Wnt/beta-catenin signaling and promotes aggressive phenotype of colorectal cancer. J Exp Clin Cancer Res. 2016;35:21. doi:10.1186/s13046-016-0444-6.
- Park NR, Cha JH, Jang JW, Bae SH, Jang B, Kim J-H, Hur W, Choi JY, Yoon SK. Synergistic effects of CD44 and TGF-beta1 through AKT/GSK-3beta/beta-catenin signaling during epithelial-mesenchymal transition in liver cancer cells. Biochem Biophys Res Commun. 2016;477:568–574. doi:10.1016/j.bbrc.2016.06.077.
- Zhuang L, Wang X, Wang Z, Ma X, Han B, Zou H, Wu Z, Dong S, Qu Z, Zang Y, Wu L. MicroRNA-23b functions as an oncogene and activates AKT/GSK3beta/beta-catenin signaling by targeting ST7L in hepatocellular carcinoma. Cell Death Dis. 2017;8:e2804. doi:10.1038/cddis.2017.518.
- Pal K, Cao Y, Gaisina IN, Bhattacharya S, Dutta SK, Wang E, Gunosewoyo H, Kozikowski AP, Billadeau DD, Mukhopadhyay D. Inhibition of GSK-3 induces differentiation and impaired glucose metabolism in renal cancer. Mol Cancer Ther. 2014;13:285–296. doi:10.1158/1535-7163.MCT-13-0681.
- Abrahamsson AE, Geron I, Gotlib J, Dao K-HT, Barroga CF, Newton IG, Giles FJ, Durocher J, Creusot RS, Karimi M, et al. Glycogen synthase kinase 3beta missplicing contributes to leukemia stem cell generation. Proc Natl Acad Sci USA. 2009;106:3925–3929. doi:10.1073/pnas.0900189106.
- Zhou F, Zhang L, van Laar T, van Dam H, Ten Dijke P. GSK3beta inactivation induces apoptosis of leukemia cells by repressing the function of c-Myb. Mol Biol Cell. 2011;22:3533–3540. doi:10.1091/mbc.E11-06-0483.
- Nagini S, Sophia J, Mishra R. Glycogen synthase kinases: moonlighting proteins with theranostic potential in cancer. Semin Cancer Biol. 2018. doi:10.1016/j.semcancer.2017.12.010.
- Xiao S, Yang Z, Lv R, Zhao J, Wu M, Liao Y, Liu Q, Alonso MM. miR-135b contributes to the radioresistance by targeting GSK3beta in human glioblastoma multiforme cells. PLoS One. 2014;9:e108810. doi:10.1371/journal.pone.0108810.
- Yost C, Torres M, Miller JR, Huang E, Kimelman D, Moon RT. The axis-inducing activity, stability, and subcellular distribution of beta-catenin is regulated in Xenopus embryos by glycogen synthase kinase 3. Genes Dev. 1996;10:1443–1454.
- Wang J, Jo U, Joo SY, Kim H. FBW7 regulates DNA interstrand cross-link repair by modulating FAAP20 degradation. Oncotarget. 2016;7:35724–35740. doi:10.18632/oncotarget.9595.
- Yang Y, Lei T, Du S, Tong R, Wang H, Yang J, Huang J, Sun M, Wang Y, Dong Z. Nuclear GSK3beta induces DNA double-strand break repair by phosphorylating 53BP1 in glioblastoma. Int J Oncol. 2018;52:709–720. doi:10.3892/ijo.2018.4237.
- Hermida MA, Dinesh Kumar J, Leslie NR. GSK3 and its interactions with the PI3K/AKT/mTOR signalling network. Adv Biol Regul. 2017;65:5–15. doi:10.1016/j.jbior.2017.06.003.
- Wang Z, Smith KS, Murphy M, Piloto O, Somervaille TCP, Cleary ML. Glycogen synthase kinase 3 in MLL leukaemia maintenance and targeted therapy. Nature. 2008;455:1205–1209. doi:10.1038/nature07284.
- Beurel E, Jope RS. The paradoxical pro- and anti-apoptotic actions of GSK3 in the intrinsic and extrinsic apoptosis signaling pathways. Prog Neurobiol. 2006;79:173–189. doi:10.1016/j.pneurobio.2006.07.006.
- Kroon J, In ‘T Veld LS, Buijs JT, Cheung H, van der Horst G, van der Pluijm G. Glycogen synthase kinase-3beta inhibition depletes the population of prostate cancer stem/progenitor-like cells and attenuates metastatic growth. Oncotarget. 2014;5:8986–8994. doi:10.18632/oncotarget.1510.
- Matsuo FS, Andrade MF, Loyola AM, Da Silva SJ, Silva MJB, Cardoso SV, de Faria PR. Pathologic significance of AKT, mTOR, and GSK3beta proteins in oral squamous cell carcinoma-affected patients. Virchows Arch. 2018;472:983–997. doi:10.1007/s00428-018-2318-0.
- Thorne CA, Wichaidit C, Coster AD, Posner BA, Wu LF, Altschuler SJ. GSK-3 modulates cellular responses to a broad spectrum of kinase inhibitors. Nat Chem Biol. 2015;11:58–63. doi:10.1038/nchembio.1690.
- Momcilovic M, Bailey ST, Lee JT, Fishbein MC, Braas D, Go J, Graeber TG, Parlati F, Demo S, Li R, et al. The GSK3 signaling axis regulates adaptive glutamine metabolism in lung squamous cell carcinoma. Cancer Cell. 2018;33:905–921.e5. doi:10.1016/j.ccell.2018.04.002.
- Tang X, Zheng D, Hu P, Zeng Z, Li M, Tucker L, Monahan R, Resnick MB, Liu M, Ramratnam B. Glycogen synthase kinase 3 beta inhibits microRNA-183-96-182 cluster via the beta-Catenin/TCF/LEF-1 pathway in gastric cancer cells. Nucleic Acids Res. 2014;42:2988–2998. doi:10.1093/nar/gkt1275.
- Xu X, Zhu Y, Liang Z, Li S, Xu X, Wang X, Wu J, Hu Z, Meng S, Liu B, et al. c-Met and CREB1 are involved in miR-433-mediated inhibition of the epithelial-mesenchymal transition in bladder cancer by regulating Akt/GSK-3beta/Snail signaling. Cell Death Dis. 2016;7:e2088. doi:10.1038/cddis.2015.274.
- Chen S, Sun K-X, Liu B-L, Zong Z-H, Zhao Y. The role of glycogen synthase kinase-3beta (GSK-3beta) in endometrial carcinoma: A carcinogenesis, progression, prognosis, and target therapy marker. Oncotarget. 2016;7:27538–27551. doi:10.18632/oncotarget.8485.
- Guo R, Abdelmohsen K, Morin PJ, Gorospe M, Zhang L. Novel MicroRNA reporter uncovers repression of let-7 by GSK-3beta. PLoS One. 2013;8:e66330. doi:10.1371/journal.pone.0066330.
- Zhu K, He Y, Xia C, Yan J, Hou J, Kong D, Yang Y, Zheng G. MicroRNA-15a inhibits proliferation and induces apoptosis in CNE1 nasopharyngeal carcinoma cells. Oncol Res. 2016;24:145–151. doi:10.3727/096504016X14611963142290.
- Cerami E, Gao J, Dogrusoz U, Gross BE, Sumer SO, Aksoy BA, Jacobsen A, Byrne CJ, Heuer ML, Larsson E, et al. The cBio cancer genomics portal: an open platform for exploring multidimensional cancer genomics data. Cancer Discov. 2012;2:401–404. doi:10.1158/2159-8290.CD-12-0095.
- Gao J, Aksoy BA, Dogrusoz U, Dresdner G, Gross B, Sumer SO, Sun Y, Jacobsen A, Sinha R, Larsson E, et al. Integrative analysis of complex cancer genomics and clinical profiles using the cBioPortal. Sci Signal. 2013;6:pl1–pl1. doi:10.1126/scisignal.2004088.
- Forbes SA, Beare D, Bindal N, Bamford S, Ward S, Cole CG, Ward S, Dawson E, Ponting L, Stefancsik R. COSMIC: high-resolution cancer genetics using the catalogue of somatic mutations in cancer. Curr Protoc Hum Genet. 2016;91:10 1 1–1 37.
- McCubrey JA, Steelman LS, Bertrand FE, Davis NM, Sokolosky M, Abrams SL, Montalto G, D’Assoro AB, Libra M, Nicoletti F, et al. GSK-3 as potential target for therapeutic intervention in cancer. Oncotarget. 2014;5:2881–2911. doi:10.18632/oncotarget.2037.
- Frame S, Cohen P, Biondi RM. A common phosphate binding site explains the unique substrate specificity of GSK3 and its inactivation by phosphorylation. Mol Cell. 2001;7:1321–1327.
- Bahram F, von der Lehr N, Cetinkaya C, Larsson LG. c-Myc hot spot mutations in lymphomas result in inefficient ubiquitination and decreased proteasome-mediated turnover. Blood. 2000;95:2104–2110.
- Yeh KT, Chang JG, Lin TH, Wang YF, Chang JY, Shih MC, Lin CC. Correlation between protein expression and epigenetic and mutation changes of Wnt pathway-related genes in oral cancer. Int J Oncol. 2003;23:1001–1007.
- Bilim V, Ougolkov A, Yuuki K, Naito S, Kawazoe H, Muto A, Oya M, Billadeau D, Motoyama T, Tomita Y. Glycogen synthase kinase-3: a new therapeutic target in renal cell carcinoma. Br J Cancer. 2009;101:2005–2014. doi:10.1038/sj.bjc.6605437.
- Ougolkov AV, Fernandez-Zapico ME, Bilim VN, Smyrk TC, Chari ST, Billadeau DD. Aberrant nuclear accumulation of glycogen synthase kinase-3beta in human pancreatic cancer: association with kinase activity and tumor dedifferentiation. Clin Cancer Res. 2006;12:5074–5081. doi:10.1158/1078-0432.CCR-06-0196.
- Naito S, Bilim V, Yuuki K, Ugolkov A, Motoyama T, Nagaoka A, Kato T, Tomita Y. Glycogen synthase kinase-3beta: a prognostic marker and a potential therapeutic target in human bladder cancer. Clin Cancer Res. 2010;16:5124–5132. doi:10.1158/1078-0432.CCR-10-0275.
- Ougolkov AV, Bone ND, Fernandez-Zapico ME, Kay NE, Billadeau DD. Inhibition of glycogen synthase kinase-3 activity leads to epigenetic silencing of nuclear factor kappaB target genes and induction of apoptosis in chronic lymphocytic leukemia B cells. Blood. 2007;110:735–742. doi:10.1182/blood-2006-12-060947.
- Li B, Thrasher JB, Terranova P. Glycogen synthase kinase-3: a potential preventive target for prostate cancer management. Urol Oncol. 2015;33:456–463. doi:10.1016/j.urolonc.2015.05.006.
- Li R, Erdamar S, Dai H, Sayeeduddin M, Frolov A, Wheeler TM, Ayala GE. Cytoplasmic accumulation of glycogen synthase kinase-3beta is associated with aggressive clinicopathological features in human prostate cancer. Anticancer Res. 2009;29:2077–2081.
- Darrington RS, Campa VM, Walker MM, Bengoa-Vergniory N, Gorrono-Etxebarria I, Uysal-Onganer P, Kawano Y, Waxman J, Kypta RM. Distinct expression and activity of GSK-3alpha and GSK-3beta in prostate cancer. Int J Cancer. 2012;131:E872–83. doi:10.1002/ijc.v131.6.
- Zhu Q, Yang J, Han S, Liu J, Holzbeierlein J, Thrasher JB, Li B. Suppression of glycogen synthase kinase 3 activity reduces tumor growth of prostate cancer in vivo. Prostate. 2011;71:835–845. doi:10.1002/pros.21300.
- Zhang X, Castanotto D, Nam S, Horne D, Stein C. 6BIO enhances oligonucleotide activity in cells: a potential combinatorial anti-androgen receptor therapy in prostate cancer cells. Mol Ther. 2017;25:79–91. doi:10.1016/j.ymthe.2016.10.017.
- Zhang F, Phiel CJ, Spece L, Gurvich N, Klein PS. Inhibitory phosphorylation of glycogen synthase kinase-3 (GSK-3) in response to lithium. Evidence for autoregulation of GSK-3. J Biol Chem. 2003;278:33067–33077. doi:10.1074/jbc.M212635200.
- Tsukigi M, Bilim V, Yuuki K, Ugolkov A, Naito S, Nagaoka A, Kato T, Motoyama T, Tomita Y. Re-expression of miR-199a suppresses renal cancer cell proliferation and survival by targeting GSK-3beta. Cancer Lett. 2012;315:189–197. doi:10.1016/j.canlet.2011.10.008.
- Ito H, Ichiyanagi O, Naito S, Bilim VN, Tomita Y, Kato T, Nagaoka A, Tsuchiya N. GSK-3 directly regulates phospho-4EBP1 in renal cell carcinoma cell-line: an intrinsic subcellular mechanism for resistance to mTORC1 inhibition. BMC Cancer. 2016;16:393. doi:10.1186/s12885-016-2418-7.
- Kawazoe H, Bilim VN, Ugolkov AV, Yuuki K, Naito S, Nagaoka A, Kato T, Tomita Y. GSK-3 inhibition in vitro and in vivo enhances antitumor effect of sorafenib in renal cell carcinoma (RCC). Biochem Biophys Res Commun. 2012;423:490–495. doi:10.1016/j.bbrc.2012.05.147.
- Kuroki H, Anraku T, Bilim V, Tasaki M, Schmitt D, Mazar A, Ugolkov A, Tomita Y 9-ING-41, a clinically relevant inhibitor of Glycogen Synthase Kinase-3 (GSK-3), is active pre-clinically in human bladder and renal cell cancers. ESMO: Munich (Germany); 2018
- Ougolkov AV, Fernandez-Zapico ME, Savoy DN, Urrutia RA, Billadeau DD. Glycogen synthase kinase-3beta participates in nuclear factor kappaB-mediated gene transcription and cell survival in pancreatic cancer cells. Cancer Res. 2005;65:2076–2081. doi:10.1158/0008-5472.CAN-04-3642.
- Ding L, Liou G-Y, Schmitt DM, Storz P, Zhang J-S, Billadeau DD. Glycogen synthase kinase-3beta ablation limits pancreatitis-induced acinar-to-ductal metaplasia. J Pathol. 2017;243:65–77. doi:10.1002/path.2017.243.issue-1.
- Baumgart S, Chen N-M, Zhang J-S, Billadeau DD, Gaisina IN, Kozikowski AP, Singh SK, Fink D, Ströbel P, Klindt C, et al. GSK-3β governs inflammation-induced NFATc2 signaling hubs to promote pancreatic cancer progression. Mol Cancer Ther. 2016;15:491–502. doi:10.1158/1535-7163.MCT-15-0309.
- Santoro R, Zanotto M, Piro G, Carbone C, Tortora G. Modulating TAK1 expression through the inhibition of GSK3 impairs YAP/TAZ oncogenic functions in pancreatic cancer. ASCO: Chicago (IL), June 1-5; 2018
- Miyashita K, Kawakami K, Nakada M, Mai W, Shakoori A, Fujisawa H, Hayashi Y, Hamada J-I, Minamoto T. Potential therapeutic effect of glycogen synthase kinase 3beta inhibition against human glioblastoma. Clin Cancer Res. 2009;15:887–897. doi:10.1158/1078-0432.CCR-08-0760.
- Furuta T, Sabit H, Dong Y, Miyashita K, Kinoshita M, Uchiyama N, Hayashi Y, Hayashi Y, Minamoto T, Nakada M. Biological basis and clinical study of glycogen synthase kinase- 3beta-targeted therapy by drug repositioning for glioblastoma. Oncotarget. 2017;8:22811–22824. doi:10.18632/oncotarget.15206.
- Atkins RJ, Stylli SS, Luwor RB, Kaye AH, Hovens CM. Glycogen synthase kinase-3beta (GSK-3beta) and its dysregulation in glioblastoma multiforme. J Clin Neurosci. 2013;20:1185–1192. doi:10.1016/j.jocn.2013.02.003.
- Kotliarova S, Pastorino S, Kovell LC, Kotliarov Y, Song H, Zhang W, Bailey R, Maric D, Zenklusen JC, Lee J, et al. Glycogen synthase kinase-3 inhibition induces glioma cell death through c-MYC, nuclear factor-kappa, and glucose regulation. Cancer Res. 2008;68:6643–6651. doi:10.1158/0008-5472.CAN-08-0850.
- Korur S, Huber RM, Sivasankaran B, Petrich M, Morin P Jr., Hemmings BA, Merlo A, Lino MM, Cordes N. Regulates differentiation and growth arrest in glioblastoma. PLoS One. 2009;4:e7443. doi:10.1371/journal.pone.0007443.
- Gürsel DB, Banu MA, Berry N, Marongiu R, Burkhardt J-K, Kobylarz K, Kaplitt MG, Rafii S, Boockvar JA. Tight regulation between cell survival and programmed cell death in GBM stem-like cells by EGFR/GSK3b/PP2A signaling. J Neurooncol. 2015;121:19–29. doi:10.1007/s11060-014-1602-3.
- Domoto T, Pyko IV, Furuta T, Miyashita K, Uehara M, Shimasaki T, Nakada M, Minamoto T. Glycogen synthase kinase-3beta is a pivotal mediator of cancer invasion and resistance to therapy. Cancer Sci. 2016;107:1363–1372. doi:10.1111/cas.13028.
- Pyko IV, Nakada M, Sabit H, Teng L, Furuyama N, Hayashi Y, Kawakami K, Minamoto T, Fedulau AS, Hamada J-I. Glycogen synthase kinase 3beta inhibition sensitizes human glioblastoma cells to temozolomide by affecting O6-methylguanine DNA methyltransferase promoter methylation via c-Myc signaling. Carcinogenesis. 2013;34:2206–2217. doi:10.1093/carcin/bgt182.
- Bruning-Richardson A, Droop A, Tams D, Boissinot M, Hayes J, Cheng V, Cockle J, Ismail A, Morton R, Esteves F, et al. Identification of transcriptional targets of GSK3 involved in glioblastoma invasion. Neuro-Oncology. 2018;20:i26. doi:10.1093/neuonc/nox238.117.
- Ugolkov A, Qiang W, Bondarenko G, Procissi D, Gaisina I, James CD, Chandler J, Kozikowski A, Gunosewoyo H, O’Halloran T, et al. Combination treatment with the GSK-3 inhibitor 9-ING-41 and CCNU cures orthotopic chemoresistant glioblastoma in patient-derived xenograft models. Transl Oncol. 2017;10:669–678. doi:10.1016/j.tranon.2017.06.003.
- McCubrey JA, Steelman LS, Bertrand FE, Davis NM, Abrams SL, Montalto G, D’Assoro AB, Libra M, Nicoletti F, Maestro R, et al. Multifaceted roles of GSK-3 and Wnt/beta-catenin in hematopoiesis and leukemogenesis: opportunities for therapeutic intervention. Leukemia. 2014;28:15–33. doi:10.1038/leu.2013.184.
- Ignatz-Hoover JJ, Wang V, Mackowski NM, Roe AJ, Ghansah IK, Ueda M, Lazarus HM, de Lima M, Paietta E, Fernandez H, et al. Aberrant GSK3beta nuclear localization promotes AML growth and drug resistance. Blood Adv. 2018;2:2890–2903. doi:10.1182/bloodadvances.2018016006.
- Parameswaran R, Ramakrishnan P, Moreton SA, Xia Z, Hou Y, Lee DA, Gupta K, deLima M, Beck RC, Wald DN. Repression of GSK3 restores NK cell cytotoxicity in AML patients. Nat Commun. 2016;7:11154. doi:10.1038/ncomms11154.
- Gupta K, Gulen F, Sun L, Aguilera R, Chakrabarti A, Kiselar J, Agarwal MK, Wald DN. GSK3 is a regulator of RAR-mediated differentiation. Leukemia. 2012;26:1277–1285. doi:10.1038/leu.2012.2.
- Rizzieri DA, Cooley S, Odenike O, Moonan L, Chow KH, Jackson K, Wang X, Brail L, Borthakur G. An open-label phase 2 study of glycogen synthase kinase-3 inhibitor LY2090314 in patients with acute leukemia. Leuk Lymphoma. 2016;57:1800–1806. doi:10.3109/10428194.2015.1122781.
- Palomo V, Martinez A. Glycogen synthase kinase 3 (GSK-3) inhibitors: a patent update (2014-2015). Expert Opin Ther Pat. 2017;27:657–666. doi:10.1080/13543776.2017.1259412.
- Mathuram TL, Ravikumar V, Reece LM, Karthik S, Sasikumar CS, Cherian KM. Tideglusib induces apoptosis in human neuroblastoma IMR32 cells, provoking sub-G0/G1 accumulation and ROS generation. Environ Toxicol Pharmacol. 2016;46:194–205. doi:10.1016/j.etap.2016.07.013.
- Zhou A, Lin K, Zhang S, Chen Y, Zhang N, Xue J, Wang Z, Aldape KD, Xie K, Woodgett JR, et al. Nuclear GSK3beta promotes tumorigenesis by phosphorylating KDM1A and inducing its deubiquitylation by USP22. Nat Cell Biol. 2016;18:954–966. doi:10.1038/ncb3396.
- Lovestone S, Boada M, Dubois B, Hüll M, Rinne JO, Huppertz H-J, Calero M, Andrés MV, Gómez-Carrillo B, León T, et al. A phase II trial of tideglusib in Alzheimer’s disease. J Alzheimers Dis. 2015;45:75–88. doi:10.3233/JAD-141959.
- Tolosa E, Litvan I, Höglinger GU, Burn D, Lees A, Andrés MV, Gómez-Carrillo B, León T, Del Ser T. A phase 2 trial of the GSK-3 inhibitor tideglusib in progressive supranuclear palsy. Mov Disord. 2014;29:470–478. doi:10.1002/mds.25824.
- Atkinson JM, Rank KB, Zeng Y, Capen A, Yadav V, Manro JR, Engler TA, Chedid M, Liu C. Activating the Wnt/beta-Catenin pathway for the treatment of melanoma–Application of LY2090314, a novel selective inhibitor of glycogen synthase kinase-3. PLoS One. 2015;10:e0125028. doi:10.1371/journal.pone.0125028.
- Le Page C, Rahimi VK, Köbel M, Tonin PN, Meunier L, Portelance L, Bernard M, Nelson BH, Bernardini MQ, Bartlett JMS, et al. Characteristics and outcome of the COEUR Canadian validation cohort for ovarian cancer biomarkers. BMC Cancer. 2018;18:560. doi:10.1186/s12885-018-4242-8.
- Gray JE, Infante JR, Brail LH, Simon GR, Cooksey JF, Jones SF, Farrington DL, Yeo A, Jackson KA, Chow KH, et al. A first-in-human phase I dose-escalation, pharmacokinetic, and pharmacodynamic evaluation of intravenous LY2090314, a glycogen synthase kinase 3 inhibitor, administered in combination with pemetrexed and carboplatin. Invest New Drugs. 2015;33:1187–1196. doi:10.1007/s10637-015-0278-7.
- Zhuang Y-W, Wu C-E, Zhou J-Y, Chen X, Wu J, Jiang S, Peng H-Y, Zou X, Liu J-Y, Wu D-P, et al. Solasodine inhibits human colorectal cancer cells through suppression of the AKT/glycogen synthase kinase-3beta/beta-catenin pathway. Cancer Sci. 2017;108:2248–2264. doi:10.1111/cas.13354.
- Hameed A, Ijaz S, Mohammad IS, Muhammad KS, Akhtar N, Khan HMS. Aglycone solanidine and solasodine derivatives: A natural approach towards cancer. Biomed Pharmacother. 2017;94:446–457. doi:10.1016/j.biopha.2017.07.147.
- Mai W, Kawakami K, Shakoori A, Kyo S, Miyashita K, Yokoi K, Jin M, Shimasaki T, Motoo Y, Minamoto T. Deregulated GSK3{beta} sustains gastrointestinal cancer cells survival by modulating human telomerase reverse transcriptase and telomerase. Clin Cancer Res. 2009;15:6810–6819. doi:10.1158/1078-0432.CCR-09-0973.
- Ugolkov A, Gaisina I, Zhang J-S, Billadeau DD, White K, Kozikowski A, Jain S, Cristofanilli M, Giles F, O’Halloran T, et al. GSK-3 inhibition overcomes chemoresistance in human breast cancer. Cancer Lett. 2016;380:384–392. doi:10.1016/j.canlet.2016.07.006.
- Ugolkov AV, Bondarenko GI, Dubrovskyi O, Berbegall AP, Navarro S, Noguera R, O’Halloran TV, Hendrix MJ, Giles FJ, Mazar AP. 9-ING-41, a small-molecule glycogen synthase kinase-3 inhibitor, is active in neuroblastoma. Anticancer Drugs. 2018:1. doi:10.1097/CAD.0000000000000652
- Karmali R, Chukkapalli V, Gordon LI, Borgia JA, Ugolkov A, Mazar AP, Giles FJ. GSK-3beta inhibitor, 9-ING-41, reduces cell viability and halts proliferation of B-cell lymphoma cell lines as a single agent and in combination with novel agents. Oncotarget. 2017;8:114924–114934. doi:10.18632/oncotarget.22414.
- Gattinoni L, Zhong X-S, Palmer DC, Ji Y, Hinrichs CS, Yu Z, Wrzesinski C, Boni A, Cassard L, Garvin LM, et al. Wnt signaling arrests effector T cell differentiation and generates CD8+ memory stem cells. Nat Med. 2009;15:808–813. doi:10.1038/nm.1982.
- Thomas A, Routh ED, Pullikuth A, Jin G, Su J, Chou JW, Hoadley KA, Print C, Knowlton N, Black MA, et al. Modulation of CD8(+) memory stem T cell activity and glycogen synthase kinase 3beta inhibition enhances anti-tumoral immunity in gastric cancer. Oncoimmunology. 2018;7:e1412900. doi:10.1080/2162402X.2018.1490854.
- Sengupta S, Guha P, Katz S, Sampath P. Inhibition of GSK3beta leads to increased survival, proliferation and memory phenotype generation of GBM-specific CAR T cells. J Immunol. 2015; 194(1 Supplement): 73.10. doi:10.4049/jimmunol.1402705
- Sengupta S, Katz SC, Sengupta S, Sampath P. Glycogen synthase kinase 3 inhibition lowers PD-1 expression, promotes long-term survival and memory generation in antigen-specific CAR-T cells. Cancer Lett. 2018;433:131–139. doi:10.1016/j.canlet.2018.06.035.
- Kamphorst AO, Wieland A, Nasti T, Yang S, Zhang R, Barber DL, Konieczny BT, Daugherty CZ, Koenig L, Yu K, et al. Rescue of exhausted CD8 T cells by PD-1-targeted therapies is CD28-dependent. Science. 2017;355:1423–1427. doi:10.1126/science.aaf0683.
- Taylor A, Rudd CE. Glycogen synthase kinase 3 inactivation compensates for the lack of CD28 in the priming of CD8(+) cytotoxic T-cells: implications for anti-PD-1 Immunotherapy. Front Immunol. 2017;8:1653. doi:10.3389/fimmu.2017.01653.
- Jiao S, Xia W, Yamaguchi H, Wei Y, Chen M-K, Hsu J-M, Hsu JL, Yu W-H, Du Y, Lee -H-H, et al. PARP inhibitor upregulates PD-L1 expression and enhances cancer-associated immunosuppression. Clin Cancer Res. 2017;23:3711–3720. doi:10.1158/1078-0432.CCR-16-3215.
- Cichocki F, Valamehr B, Bjordahl R, Zhang B, Rezner B, Rogers P, Gaidarova S, Moreno S, Tuininga K, Dougherty P, et al. GSK3 inhibition drives maturation of NK cells and enhances their antitumor activity. Cancer Res. 2017;77:5664–5675. doi:10.1158/0008-5472.CAN-17-0799.