ABSTRACT
Bladder cancer is a common and widespread cancer of the human urinary system, and its incidence is increasing. Gene therapy is a promising treatment of bladder cancer. In our study, a recombinant adeno-associated virus (rAAV9-UPII-TK-EGFP) driven by a UPII promoter was constructed. The efficacy and safety of infection of bladder cells was tested in vivo and in vitro. The ability of rAAV9-UPII-TK-EGFP to penetrate the glycosaminoglycan (GAG) layer on the surface of bladder cells and to transduce the bladder cells in vivo was very high. Additionally, we confirmed that the TK/GCV system has a powerful cytotoxic effect on bladder tumor cells in vitro and in vivo. Thus, our data indicate that rAAV9-UPII-TK-EGFP is a precise gene drug delivery system for the treatment of bladder cancer, and the TK/GCV therapeutic strategy has a powerful antitumor effect. These findings can be widely used in clinical and scientific studies.
1. Introduction
Bladder cancer is the second most common malignant tumor of the urinary system in humans worldwide.Citation1 In China, from 2007 to 2017, the incidence of bladder cancer was increased due to aging of the population. There are considerable sex-associated differences in bladder cancer mortality, and a higher incidence was reported in men.Citation2 The major forms of bladder cancer include nonmuscle invasive bladder cancer (NMIBC), muscle-invasive bladder cancer (MIBC), and metastatic disease.Citation3 At present, treatment options for bladder cancer include surgical resection, chemotherapy, and immunotherapy.Citation4-6 A significant number of patients respond to the first-line therapy; however, the majority of patients ultimately get worse. Drug toxicity and resistance limit the efficacy.Citation7
New treatment strategies are needed to improve the current treatment; gene therapy is a promising strategy.Citation8 Our previous studies confirmed that the bladder-specific human uroplakin II promoter located upstream of a suicide gene can specifically restrict the expression of therapeutic genes to bladder cells.Citation9-11 Adeno-associated virus (AAV9) is a replication-defective parvovirus with a broad range of host cells, low pathogenicity, and long-term transgene expression. The diameter of an AAV9 particle is 20–26 nm, which is only one-fifth of that of an adenovirus and one-quarter of that of a lentivirus.Citation12,Citation13
The bladder is well suited for intravesical administration because of its specific anatomy, and intravesical instillation of a viral treatment can efficiently avoid clearance by the immune system. However, the glycosaminoglycan (GAG) layer on the surface of bladder cells is an obstacle, which can considerably reduce viral transfection efficiency.Citation14 In the present study, we generated rAAV9-UPII-TK-EGFP and assessed its infection efficiency and safety in vitro and in vivo.
Infection of bladder cancer cells with a viral vector carrying a suicide gene in combination with intraperitoneal injection of GCV is a cytotoxic gene therapy strategy.Citation15 The nontoxic prodrug GCV is phosphorylated to GCV-triphosphate by prodrug-converting enzyme (TK), which subsequently interacts with cellular DNA polymerase to terminate DNA synthesis and causes the death of dividing cells. Additionally, the unique “bystander effect” can greatly improve the efficacy of suicide gene therapy.Citation15,Citation16
Hence, in our study, we demonstrated the specificity and safety of rAAV9-UPII-TK-EGFP in vitro and in vivo and used the TK/GCV gene therapy strategy to efficiently inhibit bladder tumor growth. These findings provide key evidence for clinical applications and scientific investigation of rAAV9-UPII-TK-EGFP in combination with GCV in bladder cancer.
2 Materials and methods
2.1. Cell lines and cell culture
All cell lines were purchased from Cell Bank, Chinese Academy of Sciences. Mouse bladder cancer (MB49), human bladder cancer (UMUC3), human embryonic kidney (293 T), human prostate cancer (LNCaP), human hepatoma (HUT), and human gastric cancer (SGC7901) cell lines were cultured in high-glucose DMEM (HyClone) supplemented with 10% fetal bovine serum (HyClone) and 1% penicillin/streptomycin (Invitrogen). The incubator was set to 37°C with 5% CO2.
2.2. Production of rAAV9 vector
The human UPII promoter and the TK and EGFP genes were amplified by polymerase chain reaction (PCR); then, these exogenous gene fragments were inserted into a shuttle vector at the multiple cloning site (MCS) to create a TK- and EGFP-expressing cassette driven by the human UPII promoter. rAAV9-UPII-TK-EGFP particles were harvested 72 h after cotransfection of AAV-293 cells with the packaging plasmid (p-UPII-TK-EGFP), pAAV-helper, and pAAV-RC. The viruses were purified by two rounds of cesium chloride (CsCl) density gradient ultracentrifugation and a single ultrafiltration. Purified viruses were titrated by quantitative PCR to detect the genome copy number and diluted to create a standard curve. To confirm the sequences, all constructs were sequenced after electrophoresis by a DNA sequencing service.
2.3. Reporter gene detection in vitro
Cells were seeded in 24-well plates at a density of 1 × 105 cells/well, and 1 × 105 virion genomes (VG) of rAAV9-UPII-TK-EGFP or rAAV9-EGFP vectors was added to each well. After coculture for 24 h, EGFP expression was detected by a fluorescence microscope (BX50).
2.4. Reporter gene detection in vivo
C57BL/6 female mice (17–20 g) were purchased from the Lanzhou University Animal Center. Mice were quarantined in an SPF grade animal facility at 24°C under a 12-h light/dark cycle with food and water supplied ad libitum for a minimum of 7 days. The experimental protocols were approved by the Committee on the Ethics of Animal Experiments of the Second Hospital of Lanzhou University. Mice (n = 6) were anesthetized with 2% pentobarbital (30 mg/kg), and a 24 G catheter was introduced through the urethra into the bladder as described previously;Citation17 the animals were injected with 1 × 105 VG of rAAV9-UPII-TK-EGFP or AAV9-EGFP (diluted in 100 μl of PBS). The bladder, liver, and kidney were harvested one week after the injection and fixed in 4% paraformaldehyde overnight at 4°C. Then, the tissues were sequentially dehydrated in 10%, 20%, and 30% sucrose at 4°C. The samples were embedded in optimal cutting temperature (O.C.T.) compound and sectioned into 7-μm sections. Then, the sections were mounted, and DAPI (D9542, Sigma) was used for counterstaining. The images were acquired at 40× magnification using an Olympus fluorescence microscope (BX53).
2.5. Western blotting
Equal amounts of protein were extracted using RIPA lysis buffer (Beyotime Biotechnology, Jiangsu, China). Then, the proteins were separated by 12% sodium dodecyl sulfate polyacrylamide gel electrophoresis (SDS-PAGE) and transferred to a PVDF membrane (Amersham Life Science). The protein bands were detected using primary antibodies against TK (15691-1-AP, Proteintech) and GAPDH (ab8245, Abcam) and an HRP-conjugated secondary anti-rat antibody (ab216772, Abcam).Citation18 The bands were visualized with an enhanced chemiluminescence detection kit (Beyotime Biotechnology).
2.6. Cell survival assay
Cells were seeded in 96-well plates at a density of 1 × 104 cells per well and infected with rAAV9-UPII-TK-EGFP or AAV9-EGFP (1 × 104 VG). After coculture for 24 h, the cells were treated with or without 0.01 μg of GCV. After incubation for 24 h at 37°C, 10 μl of CCK-8 (Dojindo Laboratories, Kumamoto, Japan) was added to each well, the samples were incubated for additional 2 h, and the optical density (OD) was measured at 450 nm using a microplate reader.
2.7. Real-time cell analysis (RTCA)
An xCELLigence real-time cell analyzer system (Acea Biosciences, San Diego, CA, USA) was used to monitor the cytotoxicity/activity of the cells according to a routine protocol.Citation19 An automatic cell counter (Jimbio, Jiangsu, China) was used to count 1 × 104 cells, which were seeded in a 16-well microelectrode-coated plate. After the infection with rAAV9-UPII-TK-EGFP or rAAV9-EGFP (1 × 104 VG) for 24 h, the cells were treated with or without 0.01 μg of GCV. Impedance readings were automatically recorded and plotted as the cell index ± SD every 15 min.
2.8. Animal experiment
Six-week-old C57BL/6 mice were purchased from the Lanzhou University Animal Center; half of the animals were male, and half were female. The guidelines for animals handling were followed. The mice were subcutaneously injected in the left flank with 1 × 106 MB49 cells suspended in 100 µl of PBS (pH 7.4; BioSource). The mice with implanted tumors were randomly assigned to four groups once the tumors reached a size of 100–150 mm3. Then, the viruses (1 × 107 VG/mouse) were injected into the tumors every second day for a total of three injections. Intraperitoneal injection of GCV (60 mg/kg/d) was administered every day for a week. The tumor size was measured using a caliper every day. Tumors were harvested on day 8 for additional analyses.
2.9. Histological analysis
Tumor samples were collected, fixed, and embedded in paraffin according to the standard methods. Tumor tissue sections were used for hematoxylin-eosin staining, and standard procedures were used to evaluate histological changes by three senior pathologists.
2.10. Immunofluorescence and TUNEL assays
Immunofluorescence was performed as described previously.Citation20,Citation21 The following antibodies were used for immunofluorescence: rabbit anti-Bax (Immunoway, 1:700 dilution) and rabbit anti-cleaved caspase-3 (Abcam, 1:200 dilution). The secondary antibodies were Alexa Fluor 555- and Alexa Fluor 647-conjugated (Gibco, 1:500 dilution), and the nuclei were stained with DAPI (D9542, Sigma). Images were acquired using a fluorescence system (BX50). Then, TUNEL staining was performed as described in our previous study.Citation22 TUNEL-positive cells (brown) were counted in each field, and the results are expressed as the percentage.
2.11. Statistical analysis
All data are reported as the mean ± standard deviation (SD), and GraphPad Prism 7.0 software was used to generate statistical charts. Student’s t test was used to compare the differences between groups. The significance level was defined as P< .05.
3. Results
3.1. Generation of recombinant adeno-associated virus
The human UPII promoter and the TK and EGFP genes were inserted into a shuttle plasmid to generate the rAAV9-UPII-TK-EGFP vector as described in the Materials and Methods. The rAAV9-UPII-TK-EGFP vector was isolated, concentrated, and purified by a three-step procedure (). We also constructed the rAAV9-EGFP vector as a control.
Figure 1. rAAV9-UPII-TK-EGFP construct and TK gene expression in vitro. (a) rAAV9-UPII-TK-EGFP construct map. (b) Intensity of EGFP in six cell lines transfected with rAAV9-UPII-TK-EGFP or rAAV9-EGFP. (c, d) EGFP expression in bladder cancer cell lines (UMUC3 and MB49) measured approximately 24 h after transfection with rAAV9-UPII-TK-EGFP or rAAV9-EGFP. (e) Expression of TK protein in two bladder cancer cells transfected with rAAV9-UPII-TK-EGFP or rAAV9-EGFP detected by western blot analysis. (f) Expression of TK protein in various cell lines after infection with rAAV9-UPII-TK-EGFP. Data are presented as the mean ± SD. * indicates statistical significance (*P < .05)
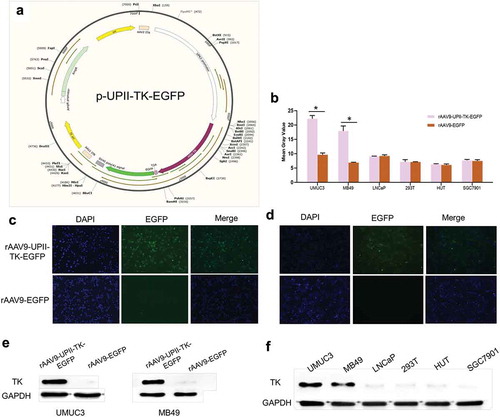
3.2.. Tropism of rAAV9-UPII-TK-EGFP vectors in vitro
To verify the specificity and safety of rAAV9-UPII-TK-EGFP, multiple cell lines were transfected, including two bladder cell lines (MB49 and UMUC3) and four additional cell lines (293 T, LNCaP, HUT, and SGC7901). Approximately 24 h after infection, the EGFP expression in the bladder cancer cells (MB49 and UMUC3) was significantly higher than that in other cells (293 T, LNCaP, HUT, and SGC7901). Infection of bladder tumor cells by rAAV9-UPII-TK-EGFP was significantly higher than that by rAAV9-EGFP (). Bladder cells (UMUC3 and MB49) were infected with two different vectors and incubated for 24 h. As anticipated, the infection by the rAAV9-UPII-TK-EGFP vector was significantly higher than that by the rAAV9-EGFP vector (). The tissue-targeting specificity of the rAAV9-UPII-TK-EGFP vector was detected at the protein level, and the results were consistent with the fluorescence expression data (). These results suggest that the UPII promoter can significantly increase the ability of rAAV9-UPII-TK-EGFP to infect bladder cancer cells.
3.3. Tropism of rAAV9-UPII-TK-EGFP vectors in vivo
The vectors were instilled into the bladder of C57BL/6 mice to assess the specificity and efficiency of rAAV9-UPII-TK-EGFP. Frozen sections were observed under a fluorescence microscope. The results indicated that rAAV9-UPII-TK-EGFP is strongly expressed in epithelial bladder cells, and there was no fluorescence in the kidney or liver (). The rAAV9-UPII-TK-EGFP or rAAV9-EGFP vectors were instilled into the bladder cavity, and the EGFP expression was compared in frozen bladder sections. The expression of EGFP in the rAAV9-UPII-TK-EGFP groups was significantly higher than that in the rAAV9-EGFP groups ().
Figure 2. Analysis of the in vivo targeting and efficacy of rAAV9-UPII-TK-EGFP. (a) Intravesical instillation of rAAV9-UPII-TK-EGFP can infect mouse bladder cells without infecting kidney and liver cells. (b) Variable intensity of green fluorescence is detected in frozen sections of the bladder. rAAV9-UPII-TK-EGFP can efficiently infect mouse bladder cells
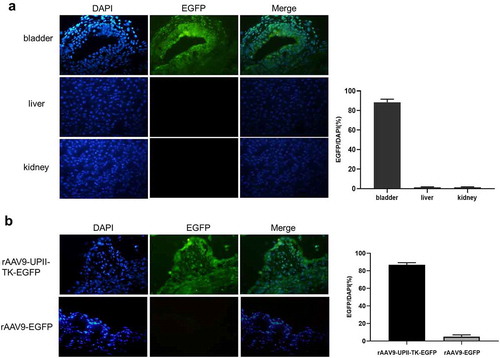
3.4. rAAV9-UPII-TK-EGFP/GCV specifically suppresses bladder cancer cell growth in vitro
To assess the growth inhibition effect of rAAV9-UPII-TK-EGFP/GCV, various types of cells were infected by rAAV9-UPII-TK-EGFP or rAAV9-EGFP for 24 h. Then, the cells were treated with or without GCV for 24 h. The CCK-8 assay was performed according to standard operating procedure. The microplate reader data showed that the survival rate of MB49 and UMUC3 cells is significantly decreased after treatment with rAAV9-UPII-TK-EGFP/GCV for 24 h. However, the proliferation and growth of other nonbladder cancer cells are not significantly affected ().
Figure 3. Efficacy and safety of the rAAV9-UPII-TK-EGFP/GCV treatment system in bladder cancer cells in vitro. (a) Cell viability was determined by the CCK-8 assay. (b) The rAAV9-UPII-TK-EGFP/GCV treatment system can efficiently suppress the growth of bladder cancer cells. The RTCA system was used for real-time monitoring of cell growth. (c) The rAAV9-UPII-TK-EGFP/GCV treatment system had no inhibitory effect on the growth of nonbladder tumor cells (LNCaP, 293 T, HUT, and SGC7901). Data are presented as the mean ± SD. * indicates statistical significance (*P < .05)
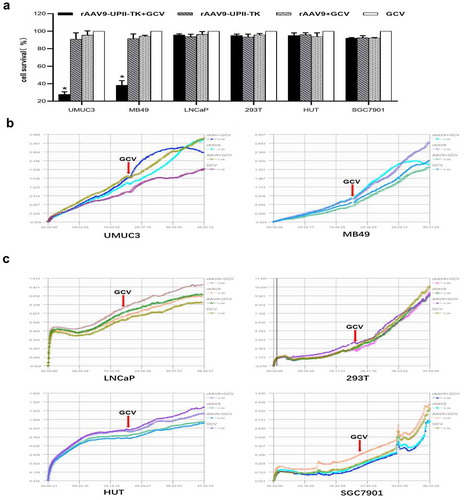
The RTCA system was used for accurate monitoring of the cytotoxicity. In the first 24 h, all cells infected with rAAV9-UPII-TK-EGFP or rAAV9-EGFP did not show changes in their growth. Thus, viral vectors are very safe for cells. Then, GCV was added for the subsequent 24 h. In bladder cancer cells, the proliferation curve in the rAAV9-UPII-TK-EGFP/GCV group indicated a decrease in proliferation; however, other control groups continued to proliferate (). No decline was observed in nonbladder cancer cells ().
3.5. Antitumor activity of rAAV9-UPII-TK-EGFP/GCV in vivo
The antitumor effect of rAAV9-UPII-TK-EGFP/GCV was investigated in vivo. A mouse tumor model was generated by endermic injection of MB49 cells; then, intratumor injection of rAAV9-UPII-TK-EGFP or rAAV9-EGFP was performed every second day, and intraperitoneal injection of GCV was performed every day for a week. The results suggested that the tumors treated for one week with rAAV9-UPII-TK-EGFP/GCV were considerably smaller than those in the three control groups ().
Figure 4. Efficacy of the rAAV9-UPII-TK-EGFP/GCV treatment system in bladder cancer cells in vivo. (a) Comparison of tumor size in each group. (b) The tumor weight in each group was measured, and the data were compared. (c) The expression of the apoptotic factors Bax (red) and cleaved caspase-3 (green) in tumor tissues was determined using immunofluorescence. Bar, 50 μm. (d, e) Apoptotic factors were significantly increased in the rAAV9-UPII-HSV-TK-EGFP/GCV treatment group. (f) TUNEL staining indicated the presence of apoptotic cells (red arrow points) and nonapoptotic cells (green arrow points) in the tumor tissue sections. Bar, 50 μm. (g) Statistical evaluation of TUNEL-positive cells in the field of view. (h) H&E staining of paraffin sections of tumors of various groups (red arrows indicate apoptotic cells). Bar, 50 μm. Data are presented as the mean ± SD. * indicates statistical significance (*P < .05)
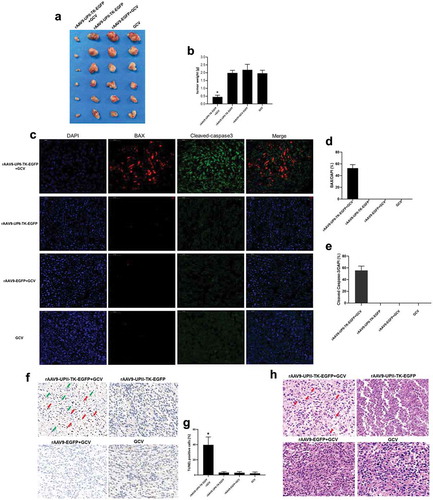
Apoptotic changes in the tumor were detected by immunofluorescence assays of Bax (red) and cleaved caspase-3 (green). The data indicated the presence of apoptosis in the rAAV9-UPII-TK-EGFP/GCV group, and apoptosis was not detected in the rAAV9-UPII-TK-EGFP, rAAV9-EGFP/GCV, and GCV groups (). Apoptosis was also detected by the TUNEL assay, and the results were consistent with the immunofluorescence data (). The results of H&E staining showed that various pathological changes are present in the experimental and control groups, and apoptosis and necrosis can be observed in the rAAV9-UPII-TK-EGFP/GCV group (). Thus, apoptosis plays an important role in the mechanism of bladder cancer cell death.
4. Discussion
The progress in the recent development of novel antitumor strategies was reviewed. Gene therapy is a new biological drug and an important and promising area of cancer therapy. Detailed studies demonstrated that an increasing number of suicide genes, ribozymes, and noncoding RNAs have great potential in cancer treatment.Citation18,Citation23-25 The number of viral vectors used for gene transfer has been increasing in recent years, including lentiviruses, herpes simplex viruses, adenoviruses, poxvirus, and adeno-associated virus.Citation26,Citation27 The AAV vector is one of the most promising gene delivery vectors in cancer gene therapy due to its low immunogenicity, wide tropisms, and long-term transgenic expression in vivo.Citation28
Viral vectors targeting of bladder cells are one of the most significant obstacles. In this study, we investigated the efficacy and safety of rAAV9-UPII-TK-EGFP transfected into bladder cells in vitro and in vivo under the control of the bladder-specific UPII promoter. Our results indicate that under uniform conditions, the infection of bladder cancer cells (MB49 and UMUC3) by the rAAV9-UPII-TK-EGFP vector was significantly higher than that of nonbladder cancer cells (293 T, LNCaP, HUT, and SGC7901) (). High expression of the “suicide gene” in bladder cancer cells was also detected at the protein level ().
The bladder is an easily accessible organ; however, the GAG layer on the surface of bladder cells acts as a nonspecific barrier that hinders viral infection of bladder cells.Citation14 Our previous studies aimed to increase the infection of bladder cells by adenovirus.Citation9-11 Unfortunately, our results are consistent with previous studies that indicated that less than 10% of bladder cells are transfected in vivo.Citation29 The role of the GAG layer on the bladder surface as a barrier against urine and pathogens has been acknowledged.Citation30 We were reluctant to improve the infection efficiency by destroying the GAG layer; hence, we generated a novel bladder cell-specific rAAV9-UPII-TK-EGFP vector to deliver therapeutic genes. As expected, the ability of rAAV9-UPII-TK-EGFP to penetrate the GAG layer and transduce bladder cells in vivo was robust (). We did not detect EGFP expression in the kidney or liver, indicating that the rAAV9-UPII-TK-EGFP vector may have high specificity for bladder cells ().
In our previous studies, the TK/GCV system had a potent antitumor effect in vitro and in vivo.Citation11 In addition to traditional methods, RTCA was used to monitor the therapeutic efficacy in real time (). Our results confirmed the inhibitory effect of the rAAV9-UPII-TK-EGFP/GCV system on bladder cancer cells. Notably, the rAAV9-UPII-TK-EGFP/GCV treatment strategy directly killed bladder cancer cells and considerably improved the killing effect via the “bystander effect”. Additional experiments demonstrated that tumors treated with rAAV9-UPII-TK-EGFP/GCV were significantly smaller than those in the control groups (). Immunofluorescence, TUNEL assay, and H&E staining were used to demonstrated that apoptosis was present in the treatment group (). Thus, we demonstrated the efficacy and safety of the rAAV9-UPII-TK-EGFP vector in vivo and in vitro and confirmed that the rAAV9-UPII-TK-EGFP/GCV gene therapy strategy is effective in bladder cancer.
Overall, rAAV9-UPII-TK-EGFP is an ideal vector that can be used to accurately deliver bladder-targeted drugs, improve drug efficacy, and reduce side effects. Our data suggest that the rAAV9-UPII-TK-EGFP/GCV system is a powerful gene therapy strategy that can efficiently inhibit tumor growth. The combination of a precise drug delivery system with therapeutic drugs is expected to be developed into a new adjunctive therapy.
Disclosure of potential conflicts of interest
No potential conflicts of interest were disclosed.
Authors’ contributions
Zhiping Wang and Degui Wang conceived and designed the study. Foyan Lian and Qiang Ye developed the protocol and performed the data analysis. Foyan Lian, Bing Feng, Hui Cheng, Shaomin Niu, and Ning Fan wrote the manuscript. All authors discussed and commented on the study. All authors read and approved the final manuscript.
References
- Torre LA, Bray F, Siegel RL, Ferlay J, Lortet-Tieulent J, Jemal A. Global cancer statistics, 2012. CA Cancer J Clin. 2015;65:87–108. doi:10.3322/caac.21262. PMID:25651787.
- Liu X, Jiang J, Yu C, Wang Y, Sun Y, Tang J, Chen T, Bi Y, Liu Y, Zhang ZJ. Secular trends in incidence and mortality of bladder cancer in China, 1990–2017: A joinpoint and age-period-cohort analysis. Cancer Epidemiol. 2019;61:95–103. doi:10.1016/j.canep.2019.05.011. PMID:31176961.
- Robertson AG, Kim J, Al-Ahmadie H, Bellmunt J, Guo G, Cherniack AD, Hinoue T, Laird PW, Hoadley KA, Akbani R, et al. Comprehensive molecular characterization of muscle-invasive bladder cancer. Cell. 2017;171:540–56.e25. doi:10.1016/j.cell.2017.09.007. PMID:28988769.
- Babjuk M, Böhle A, Burger M, Capoun O, Cohen D, Compérat EM, Hernández V, Kaasinen E, Palou J, Rouprêt M, et al. EAU guidelines on non-muscle-invasive urothelial carcinoma of the bladder: update 2016. Eur Urol. 2017;71:447–461. doi:10.1016/j.eururo.2016.05.041. PMID:27324428.
- Chang SS, Bochner BH, Chou R, Dreicer R, Kamat AM, Lerner SP, Lotan Y, Meeks JJ, Michalski JM, Morgan TM, et al. Treatment of non-metastatic muscle-invasive bladder cancer: AUA/ASCO/ASTRO/SUO guideline. J Urol. 2017;198:552–559. doi:10.1016/j.juro.2017.04.086. PMID:28456635.
- Witjes JA, Compérat E, Cowan NC, De Santis M, Gakis G, Lebret T, Ribal MJ, Van der Heijden AG, Sherif A. European Association of Urology. EAU guidelines on muscle-invasive and metastatic bladder cancer: summary of the 2013 guidelines. Eur Urol. 2014;65:778–792. doi:10.1016/j.eururo.2013.11.046. PMID:24373477.
- Luqmani YA. Mechanisms of drug resistance in cancer chemotherapy. Med Princ Pract. 2005;14(Suppl 1):35–48. doi:10.1159/000086183. PMID:16103712.
- Cross D, Burmester JK. Gene therapy for cancer treatment: past, present and future. Clin Med Res. 2006;4:218–227. doi:10.3121/cmr.4.3.218. PMID:16988102.
- He XD, Wang ZP, Wei HY, Zhou Q, Wang DG, Tian JQ, Fu SJ, Rodriguez R. Construction of urothelium-specific recombinant adenovirus and its inhibition in bladder cancer cell. Urol Int. 2009;82:209–213. doi:10.1159/000200802. PMID:19322012.
- Wang D, Wang Z, Tian J, He X, Chowdhury WH, Zhang X, Li S, Rodriguez R. Prostate stem cell antigen enhancer and uroplakin II promoter based bladder cancer targeted tissue-specific vector. Urol Oncol. 2010;28:164–169. doi:10.1016/j.urolonc.2008.02.002. PMID:18440837.
- Wang DG, Zhao MJ, Liu YQ, Liu XW, Niu HT, Song YF, Tian YX. Fiber-modified adenovirus-mediated suicide gene therapy can efficiently eliminate bladder cancer cells in vitro and in vivo. Oncotarget. 2016;7:71710–71717. doi:10.18632/oncotarget.12324. PMID:27687590.
- Ai J, Wang D, Wei Q, Li H, Gao G. Adeno-associated virus serotype vectors efficiently transduce normal prostate tissue and prostate cancer cells. Eur Urol. 2016;69:179–181. doi:10.1016/j.eururo.2015.10.019. PMID:26526960.
- Cataldi MP, McCarty DM. Differential effects of DNA double-strand break repair pathways on single-strand and self-complementary adeno-associated virus vector genomes. J Virol. 2010;84:8673–8682. doi:10.1128/JVI.00641-10. PMID:20538857.
- Wyndaele J, Riedl C, Taneja R, Lovász S, Ueda T, Cervigni M. GAG replenishment therapy for bladder pain syndrome/interstitial cystitis. Neurourol Urodyn. 2019;38:535–544. doi:10.1002/nau.23900. PMID:30592544.
- Pan JG, Zhou X, Luo R, Han RF. The adeno-associated virus-mediated HSV-TK/GCV suicide system: a potential strategy for the treatment of bladder carcinoma. Med Oncol. 2012;29:1938–1947. doi:10.1007/s12032-011-0091-x. PMID:22011935.
- Leten C, Trekker J, Struys T, Dresselaers T, Gijsbers R, Vande Velde G, Lambrichts I, Van Der Linden A, Verfaillie CM, Himmelreich U. Assessment of bystander killing-mediated therapy of malignant brain tumors using a multimodal imaging approach. Stem Cell Res Ther. 2015;6:163. doi:10.1186/s13287-015-0157-3. PMID:26345383.
- Zychlinsky Scharff A, Albert ML, Ingersoll MA. Urinary tract infection in a small animal model: transurethral catheterization of male and female mice. J Vis Exp. 2017. doi:10.3791/54432. PMID:29286380.
- Shen Y, Li Y, Ma X, Wan Q, Jiang Z, Liu Y, Zhang D, Liu X, Wu W. Connexin 43 SUMOylation improves gap junction functions between liver cancer stem cells and enhances their sensitivity to HSVtk/GCV. Int J Oncol. 2018;52:872–880. doi:10.3892/ijo.2018.4263. PMID:29393359.
- Jiang H, Deng R, Yang X, Shang J, Lu S, Zhao Y, Song K, Liu X, Zhang Q, Chen Y, et al. Peptidomimetic inhibitors of APC-Asef interaction block colorectal cancer migration. Nat Chem Biol. 2017;13:994–1001. doi:10.1038/nchembio.2442. PMID:28759015.
- Tang M, Luo Z, Wu Y, Zhuang J, Li K, Hu D, Rong H, Xian B, Ge J. BAM15 attenuates transportation-induced apoptosis in iPS-differentiated retinal tissue. Stem Cell Res Ther. 2019;10:64. doi:10.1186/s13287-019-1151-y. PMID:30795805.
- Zhao D, Zhang M, Yuan H, Meng C, Zhang B, Wu H. Ginsenoside Rb1 protects against spinal cord ischemia-reperfusion injury in rats by downregulating the Bax/Bcl-2 ratio and caspase-3 and p-Ask-1 levels. Exp Mol Pathol. 2018;105:229–235. doi:10.1016/j.yexmp.2018.09.001. PMID:30184471.
- Zhao MJ, Song YF, Niu HT, Tian YX, Yang XG, Xie K, Jing YH, Wang DG. Adenovirus-mediated downregulation of the ubiquitin ligase RNF8 sensitizes bladder cancer to radiotherapy. Oncotarget. 2016;7:8956–8967. doi:10.18632/oncotarget.6909. PMID:26788910.
- Chen Y, Huang H, Yao C, Su F, Guan W, Yan S, Ni Z. Antitumor activity of combined endostatin and thymidine kinase gene therapy in C6 glioma models. Cancer Med. 2016;5:2477–2486. doi:10.1002/cam4.798. PMID:27366865.
- Choi SS, Chi BH, Chang IH, Kim KD, Lee SR, Kim SU, Lee HJ. Human neural stem cells overexpressing a carboxylesterase inhibit bladder tumor growth. Mol Cancer Ther. 2016;15:1201–1207. doi:10.1158/1535-7163.MCT-15-0636. PMID:27009215.
- Zhang Y, Wang J, Cheng H, Sun Y, Liu M, Wu Z, Pei R. Conditional control of suicide gene expression in tumor cells with theophylline-responsive ribozyme. Gene Ther. 2017;24:84–91. doi:10.1038/gt.2016.78. PMID:27874855.
- Appaiahgari MB, Vrati S. Adenoviruses as gene/vaccine delivery vectors: promises and pitfalls. Expert Opin Biol Ther. 2015;15:337–351. doi:10.1517/14712598.2015.993374. PMID:25529044.
- Irie A. Advances in gene therapy for bladder cancer. Curr Gene Ther. 2003;3:1–11. PMID:12553531.
- Baruteau J, Waddington SN, Alexander IE, Gissen P. Gene therapy for monogenic liver diseases: clinical successes, current challenges and future prospects. J Inherit Metab Dis. 2017;40:497–517. doi:10.1007/s10545-017-0053-3. PMID:28567541.
- Lin LF, Zhu G, Yoo JJ, Soker S, Sukhatme VP, Atala A. A system for the enhancement of adenovirus mediated gene transfer to uro-epithelium. J Urol. 2002;168:813–818. doi:10.1016/S0022-5347(05)64749-0. PMID:12131373.
- Janssen D. ls clinical practice aligned with the latest scientific evidence on GAG therapy. Urologia. 2017;84:16–20. doi:10.5301/uj.5000259. PMID:28862729.