ABSTRACT
A maximal surgical resection followed by radiotherapy and chemotherapy with temozolomide (TMZ) as the representative agent is the standard therapy for gliomas. However, tumor cell resistance to radiotherapy and chemotherapy leads to poor prognosis and high mortality in patients with glioma. In the present study, we demonstrated that JARID2 was downregulated and CCND1 was upregulated within glioma tissues of different grades and glioma cells. In tissue samples, JARID2 was negatively correlated with CCND1. JARID2 overexpression significantly inhibited glioma cell viability, promoted glioma cell apoptosis upon TMZ treatment, and increased p21, cleaved-PARP, and cleaved-caspase3 in TMZ-treated glioma cells. JASPAR tool predicted the possible binding sites between JARID2 and CCND1 promoter regions; through direct binding to CCND1 promoter region, JARID2 negatively regulated CCND1 expression. Under TMZ treatment, JARID2 overexpression inhibited CCND1 expression, promoted glioma cell apoptosis, and increased p21, cleaved-PARP, and cleaved-caspase3 in glioma cells treated with TMZ; meanwhile, CCND1 overexpression exerted opposite effects on glioma cells treated with TMZ and partially reversed the effects of JARID2 overexpression. In conclusion, JARID2 targets and inhibits CCND1. The JARID2/CCND1 axis modulates glioma cell growth and glioma cell sensitivity to TMZ.
Introduction
Glioma is one of the most commonly occurring primary malignant brain tumors, which begins in the glial cells and accounts for 27% of all primary brain tumors and 80% of primary malignant brain tumors.Citation1,Citation2 Among gliomas of different grades, the median survival time for high-grade gliomas was only 15–17 months, indicating a higher level of malignancy.Citation3,Citation4 The standard therapy for glioma is maximal surgical resection, followed by radiotherapy and chemotherapy with temozolomide (TMZ) as the representative agent.Citation3,Citation5,Citation6 However, malignant glioma usually spreads quickly and invades other parts of the brain, making complete surgical removal more difficult; moreover, tumor cells are prone to resistance to radiotherapy and chemotherapy, resulting in poor prognosis and high mortality in patients with glioma.Citation7,Citation8 Therefore, in-depth understanding of the molecular mechanism underlying the resistance of malignant glioma to TMZ and exploring related therapeutic targets are of great significance for improving the therapeutic effect of malignant glioma and improving the outcomes for patients.
Notably, as previously reported, isocitrate dehydrogenase (IDH)-mutated glioma patients exhibit a favorable prognosis and increased sensitivity to TMZ chemotherapy.Citation9–13 The mutant IDH1 acquires new enzymatic activity, metabolizing α-KG to produce 2-hydroxyglutarate (2-HG),Citation14 which can inhibit DNA demethylase and promote DNA and histone methylation.Citation15–17 Through, at least partially, this mechanism, IDH1 mutation and 2-HG can enhance the cytotoxicity of TMZ on glioma cells and enhance the IDH1-mutated glioma cell sensitivity to TMZ.Citation9,Citation18,Citation19 While investigating the downstream mechanism using data from Chinese Glioma Genome Atlas (CGGA), The Cancer Genome Atlas (TCGA)-Glioma (GBMLGG), and TCGA-Glioblastoma multiforme (GBM), we identified that the expression of JARID2 (Jumonji, AT Rich Interactive Domain 2), a gene closely related to histone methylation, was significantly upregulated in IDH-mutated gliomas, both low-grade glioma and glioblastoma (data not shown). Regarding the prognosis, lower JARID2 expression exhibited tight correlations with glioma patients’ poorer survival by analyzing TCGA-GBMLGG and CGGA databases (Figure S1A). More importantly, according to data from the GDSC database and GSE113510, JARID2 expression was dramatically higher within TMZ-sensitive glioma cells than that in TMZ-resistant glioma cells (Figure S1B). In myeloid neoplasms, JARID2 represses self-renewal in hematopoietic progenitor cells to exert a tumor-suppressive effect.Citation20 These previous findings strongly demonstrate that JARID2 might exert a tumor-suppressive effect on glioma, likely through enhancing glioma cell sensitivity to TMZ. No matter whether IDH is mutated in glioma, restoring JARID2 expression might enhance the toxicity of TMZ on glioma.
JARID2 is a core member of the Jumonji C (JmjC) histone demethylase family; through recruiting polycomb repressive complex 2 (PRC2) to the chromosome, JARID2 could regulate gene expression.Citation21,Citation22 As previously reported, JARID2 is involved in multiple tumors through gene expression silencing.Citation20,Citation23 Cyclin D1 (CCND1), a key regulatory factor of G1-to-S phase transition, contributes to initiating and regulating the proliferation and differentiation of tumor cells. CCND1 upregulation can decrease cancer cell sensitivity to cytotoxic agents. Within gliomas, CCND1 inhibition can inhibit the capacity of glioma cells to proliferate and to invade, and induce cell apoptosis, as well as increase the TMZ sensitivity of glioma cells.Citation24,Citation25 Interestingly, JARID2 can negatively regulate the expression of CCND1.Citation26 In leukemia cells, JARID2 exerts a negative regulatory effect on CCND1 expression by H3K27me3 binding to the CCND1 promoter and inhibits the proliferation of leukemia cells;Citation27 in mouse embryonic cardiomyocytes, JARID2 also promotes the differentiation and inhibits the proliferation of glioma cells through inhibiting CCND1 levels.Citation28 Therefore, we speculate that in glioma JARID2 may enhance glioma cell sensitivity to TMZ by inhibiting CCND1.
To address the speculation, we first examined both JARID2 and CCND1 expression within glioma tissue samples of different grades and glioma cell lines. Then, we evaluated the specific roles of JARID2 in the viability of glioma cells and cellular response to TMZ treatment. As for the molecular mechanism, the possible binding between JARID2 and CCND1 promoter region was checked using JASPAR and then by experimental analyses, and JARID2 regulation of CCND1 was investigated. Finally, the dynamic effects of JARID2 and CCND1 on glioma cell response to TMZ treatment were determined. Altogether, we attempted to demonstrate the specific effect of JARID2 on glioma cell sensitivity to TMZ and the involvement of CCND1.
Materials and methods
Clinical sampling
Eighteen glioma tissue samples (six cases of WHO grade II, III, and IV, respectively) from the tumor core region and six samples from the peritumoral brain edema (PTBE) region were obtained from patients who received treatment (surgical resection) at Xiangya Hospital, Central South University. The sampling procedure was conducted with the approval of the Ethics Committee of Xiangya Hospital, Central South University (approval no. 201803393), and signed informed consent was collected from each patient. Immediately after specimen sampling, tissues were transferred to −80°C container until subsequent experiments.
Histological examination
Tissue samples were formalin-fixed, paraffin-embedded, and cut into 5-μm sections. Then, sample slices were deparaffinized by sequential washing with xylene, 100% ethanol, 95% ethanol, 80% ethanol and phosphate buffered saline (PBS). After rehydration, some of the slides were stained with H&E and some of the sections were applied for the IHC staining. For H&E staining, sections were stained using H&E solution. For IHC staining, the sections were incubated with 0.3% H2O2 in methanol for 5 min to quench the endogenous peroxidase activity. The slides were blocked in PBS with 5% Bovine Serum Albumin (BSA) for 30 min and then incubated overnight with a 1:100 dilution of anti-JARID2 or anti-CCND1 primary antibody at 4°C. After sections were rinsed with PBS, they were incubated with poly-HRP-conjugated anti-rabbit IgG (Boster, China) for 30 min at 37°C and then stained with fresh DAB reagent (Boster, China)) for 10 min at room temperature. Finally, the section was stained with hematoxylin and observed and photographed by an optical microscope (Olympus, China).
qRT-PCR
Trizol reagent (Invitrogen, Carlsbad, CA, USA) was used to extract total RNA from target cells or tissues, and then reverse transcription was performed using the first strand cDNA synthesis kit (Promega, Madison, WI, USA). The expression levels of target factors were determined using a SYBR Green PCR Master Mix (Qiagen, Hilden, Germany) following the standard protocols. GAPDH was used as an internal reference, and the relative expression level of each target factor was calculated by Ct method. The primers are listed in .
Table 1. The primer sequence
Immunoblotting
Extract the total protein from the target cells, separate the total protein sample by SDS-PAGE, and transfer the protein sample to the polyvinylidene fluoride (PVDF) membrane. To prevent the blockage of nonspecific binding, the membrane was first incubated with 5% skim milk dissolved in diphenyltriazole buffer for 2 h at room temperature. Then, incubate the membrane with primary antibodies against JARID2 (ab192252, Abcam, Cambridge, MA, USA), CCND1 (Cat #MA5-16356; Thermo Fisher Scientific, Waltham, MA, USA), p21 (Catalog # 701151, Thermo Fisher Scientific), cleaved-PARP (Cat #44-698 G, Thermo Fisher Scientific), PARP (13371-1-AP; Proteintech, Wuhan, China), cleaved-caspase3 (ab2302, Abcam), or caspase3 (19677-1-AP, Proteintech) overnight at 4°C, followed by another incubation with the corresponding horseradish peroxidase-conjugated secondary antibody for 2 h at room temperature. Finally, perform enhanced chemiluminescence (ECL) scan for signal visualization.
Cell lineages and cell culture
The normal glial cell line HEB was obtained from Beinuo life science Company (Shanghai, China) and cultured in Dulbecco’s Modified Eagle’s Medium (Gibco, Helgerman Court, MD, USA) added with 10% FBS (Gibco). Glioma cell line SHG-44 (3131 C0001000700048) was obtained from Cell Resource Center, Shanghai Institute of Life Sciences, Chinese Academy of Sciences (Shanghai, China), and cultured in RPMI 1640 (w/o Hepes; Gibco) added with 10% FBS (Gibco). Glioma cell line LN229 (CRL-2611) was obtained from ATCC (Manassas, VA, USA) and cultured in Dulbecco’s Modified Eagle’s Medium (Gibco) added with 10% FBS (Gibco). Glioma cell line T98G (CRL-1690) was obtained from ATCC and cultured in Eagle’s Minimum Essential Medium (Gibco) added with 10% FBS (Gibco). All the cells were cultured at 37°C in 5% CO2.
Cell transfection
For JARID2 overexpression, the pCR8 JARID2 vector was obtained from addgene (addgene, USA). The empty Pcr8 vector was used as a negative control (vector). For JARID2 knockdown, small interfering RNA against JARID2 gene, as well as their nontargeting sequences were reconstructed in pLVX-shRNA2-puro (si-JARID2 compared with si-NC). For CCND1 overexpression, the cDNA of CCND1 was cloned into Pcr8 vector. Cells were seeded in a 24-well plate and the Lipofectamine 3000 reagent (Thermo Fisher Scientific, Waltham, MA, USA) was used to transfect cells with the plasmids mentioned above. Cells were collected 48 h after transfection. The primers for plasmid construction are listed in .
CCK-8 assay for cell viability
Use Cell Counting Kit-8 (SKU: CK04; Dojindo, Rockville, MD, USA) to determine cell viability. Cells (1 × 104 cells/well) were seeded in 96-well plates and incubated overnight at 37°C. After the required transfection or treatment, the cells were incubated for another 4 h at 37°C with 10 μl of CCK-8 solution and 100 μl of serum-free DMEM. Finally, use a microplate reader to measure the absorbance at 450 nm.
Flow cytometry for cell apoptosis
Target cells were transfected and examined for cell apoptosis using a FITC-labeled Annexin V/propidium iodide (PI) Apoptosis Detection kit (Beyotime, Shanghai, China). Immediately after staining, flow cytometry was performed (Beckman, USA). Early apoptotic cells were positive for Annexin V, and late apoptotic cells were positive for both Annexin V and PI.
Flow cytometry for cell cycle
Cells after transfection were harvested and washed in PBS, then fixed in 75% cold ethanol for 1 h. After being washed with PBS twice, cells were centrifuged at 850 g, and the supernatant was removed. Treated with ribonuclease, cells were added with 50 μl of RNase to ensure that solely DNA was stained. Cells were supplemented with 400 μl of PI (50 μg/ml) and incubated at 37°C for 30 min with dark treatment in accordance with the Cell Cycle Analysis Kit (Biyuntian, Jiangsu, China). The cell rates in G0/G1, S, and G2/M phases were gained using a flow cytometer (FACS Calibur, BD Biosciences, San Jose, CA, USA).
Chromatin immunoprecipitation (ChIP)
The ChIP assay was conducted using the Pierce Agarose ChIP Kit (Pierce, Appleton, WI, USA) according to the manufacturer’s instructions. Target cells were transfected with vector/JARID2. Cells were crosslinked with 1% formaldehyde and lysed to prepare nuclei. Chromatin was sheared using micronuclease digestion. Sheared DNA was then incubated with anti-JARID2 antibody (ab192252, Abcam). Normal immunoglobulin G (IgG) was served as a negative control. DNA was purified and analyzed by qRT-PCR.
Dual-luciferase reporter assay
For JARID2 binding to CCND1 promoter, wild- and mutant-type CCND1 promoter reporter vectors (psicheck2-CCND1 and psicheck2-CCND1-mut) were constructed. 293T cells were co-transfected with psicheck2-CCND1/ psicheck2-CCND1-mut and JARID2/vector. The luciferase activity was determined using the Dual-Luciferase Reporter System (Promega).
Statistics analysis
Each experiment was repeated at least three times. Data were processed using the GraphPad software (San Diego, CA, USA) and shown as mean ± standard deviation (SD). A one-way analysis of variance (ANOVA), and then Tukey’s multiple comparison test or Student’s t-test was performed to analyze the statistical significance. A P value of < 0.05 was considered statistically significant.
Results
Expression and correlation of JARID2 and CCND1 within tissue samples and cell lines
Before investigating the specific roles of JARID2 and CCND1 in regulating glioma cell phenotypes, first, both JARID2 and CCND1 expression levels within tissue samples and cells were evaluated. Glioma tissue samples in WHO grade II, III, and IV and PTBE region samples were obtained; the histopathological features were confirmed by H&E staining (). The clinical characteristics of 18 glioma patients are listed in Table S1. In tissue samples, JARID2 and CCND1 contents in tissue samples were examined by IHC staining. shows that JARID2 content decreased, whereas CCND1 increased with the grade developing. The qRT-PCR analysis provided a further confirmation that JARID2 mRNA expression was downregulated, whereas CCND1 was upregulated in tissue samples with the grade developing (). Besides, as shown in Figure S1C, when compared to IDH-wild-type glioma patient tissues, the JARID2 expression level was observably increased in IDH-mutated glioma patient tissues. In tissue samples, JARID2 and CCND1 were negatively correlated with each other (). Similarly, JARID2 expression was negatively correlated with CCND1 expression in glioma tissues according to both TCGA-GBMLGG and CGGA databases (Figure S2). Moreover, in three glioma cell lines, SHG44, T98G, and LN229, the protein levels of JARID2 were decreased, whereas CCND1 protein levels were increased compared with those in a normal glial cell line HEB (). Considering the lower JARID2 levels and higher CCND1 levels in T98G and LN229 cells, these two cell lines were selected for further experiments.
Figure 1. Expression and correlation of JARID2 and CCND1 in tissue samples and cell lines. (a) Eighteen glioma tissue samples (six cases of WHO grade II, III, and IV, respectively) and six samples from the peritumoral brain edema (PTBE) region were obtained and examined for the histopathological features by H&E staining. (b) JARID2 and CCND1 contents in tissue samples were examined by IHC staining. (c) The mRNA expression levels of JARID2 and CCND1 contents in tissue samples were examined by qRT-PCR. (d) The correlation between JARID2 and CCND1 expression was analyzed using Pearson’s correlation analysis. (e) The protein levels of JARID2 and CCND1 were determined in a normal glial cell line HEB and three glioma cell lines, SHG44, T98G, and LN229 by immunoblotting. *P < 0.05, **P < 0.01
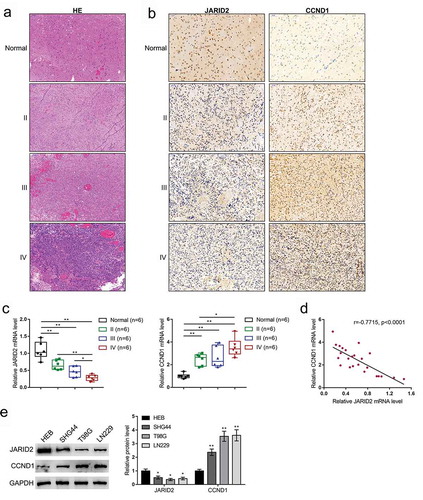
Effects of JARID2 upon the phenotypes of glioma cells
To validate the specific effects of JARID2 on glioma cell viability and response to TMZ treatment, we achieved JARID2 overexpression or knockdown in T98G and LN229 cells by transfecting JARID2 overexpression vector (JARID2) or JARID2 knockdown vector (si-JARID2) into these two cell lines. The overexpression and knockdown of JARID2 in glioma cell lines were confirmed by Immunoblotting (). In both LN229 and T98G cells, JARID2 overexpression significantly inhibited, whereas JARID2 knockdown promoted cell viability ().
Figure 2. Effects of JARID2 on glioma cell viability. (a, b) JARID2 overexpression or knockdown was achieved by transfecting JARID2 overexpression vector (JARID2) or si-JARID2 into LN229 and T98G cells. The overexpression and knockdown of JARID2 in glioma cell lines were confirmed by immunoblotting. (c, d) LN229 and T98G cells were transfected with JARID2 or si-JARID2 and examined for the cell viability by CCK-8 assay. *P < 0.05, **P < 0.01, compared to vector group. #P < 0.05, ##P < 0.01. compared to si-NC group
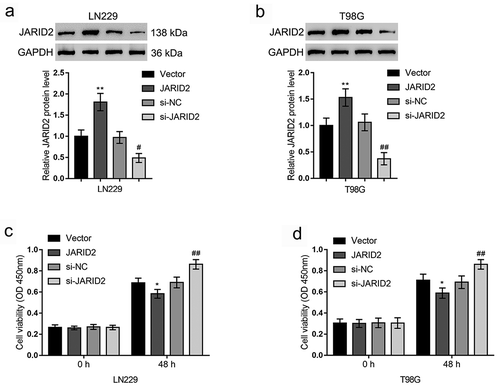
As for glioma cell response to TMZ treatment, we transfected glioma cells, exposed the cells to 100 μM TMZ, and examined cell apoptosis and protein levels of apoptosis- and cell cycle-related factors. Under TMZ treatment, JARID2 overexpression promoted, whereas JARID2 knockdown inhibited cell apoptosis in both cell lines (). Consistent with the cell apoptosis, JARID2 overexpression increased, whereas JARID2 knockdown decreased p21, cleaved-PARP, and cleaved-caspase3 protein contents (). Moreover, without TMZ treatment, the effect of JARID2 on the glioma cell cycle had been determined. Overexpression of JARID2 in both LN229 and T98G cells increased the cell proportion at G0/G1 phase and decreased cells at S and G2/M phases, while the depletion of JARID2 in both LN229 and T98G cells showed the opposite effect ( and Figure S3A).
Figure 3. Effects of JARID2 on glioma cell response to TMZ treatment. LN229 and T98G cells were transfected with JARID2 or si-JARID2, exposed to 100 μM TMZ, and examined for cell apoptosis by flow cytometry (a) and the protein levels of p21, cleaved-PARP, PARP, cleaved-caspase3, and caspase3 by immunoblotting (b). LN229 and T98G cells were only transfected with JARID2 or si-JARID2 and examined for cell cycle by flow cytometry (c). **P < 0.01, compared to vector group. ##P < 0.01, compared to si-NC group
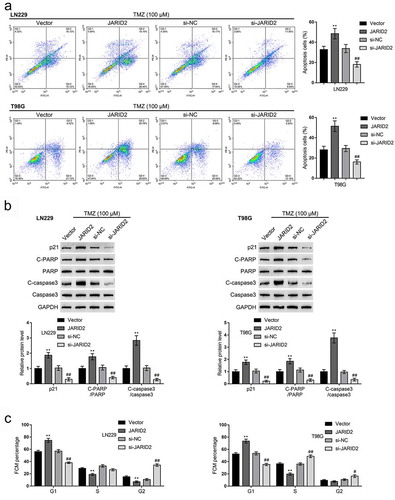
JARID2 targets the CCND1 promoter region and downregulates CCND1 expression
Since JARID2 and CCND1 are negatively correlated with each other, next, we used the online tool JASPAR to check whether there exist JARID2 binding sites in CCND1 promoter region; shows the predicted binding sites. Consistent with the negative correlation between JARID2 and CCND1, in LN229 and T98G cells, JARID2 overexpression decreased, whereas JARID2 knockdown increased the protein levels and mRNA expression levels of CCND1 (–b)). To confirm the predicted binding, we performed a ChIP assay using anti-IgG (negative control) or anti-JARID2 in LN229 cells transfected with empty vector or JARID2. As shown in , the levels of CCND1 promoter in anti-JARID2 immunoprecipitate were significantly higher in LN229 cells transfected with vector or JARID2, compared with those in anti-IgG immunoprecipitate; the levels of CCND1 promoter in anti-JARID2 immunoprecipitate were significantly higher in LN229 cells transfected with JARID2 compared with those in LN229 cells transfected with vector. Moreover, wild- and mutant-type CCND1 promoter luciferase reporter vectors (psicheck2-proCCND1 and psicheck2-proCCND1-mut) were constructed and co-transfected into 293T cells with vector or JARID2. The luciferase activity was determined. shows that when co-transfected with wild-type psicheck2-proCCND1, JARID2 overexpression significantly suppressed, whereas JARID2 inhibition enhanced the luciferase activity; when co-transfected with mutant-type psicheck2-proCCND1-mut, JARID2 caused no changes in the luciferase activity.
Table 2. JARID2 binding sites to the upstream promoter region of the CCND1 transcription start point
Figure 4. JARID2 targets CCND1 promoter region and downregulates CCND1 expression. LN229 and T98G cells were transfected with JARID2 or si-JARID2 and examined for the protein levels of CCND1 by immunoblotting (a) and the mRNA levels of CCND1 by qRT-PCR (b). (c) ChIP assay was performed using anti-IgG (negative control) or anti-JARID2 in LN229 cells transfected with vector or JARID2. The levels of CCND1 promoter in immunoprecipitate of anti-IgG and anti-JARID2 were determined using qRT-PCR. (d) Wild- and mutant-type CCND1 promoter luciferase reporter vectors were constructed and co-transfected into 293T cells with empty vector or JARID2. The luciferase activity was determined. *P < 0.05, **P < 0.01, #P < 0.05, ##P < 0.01
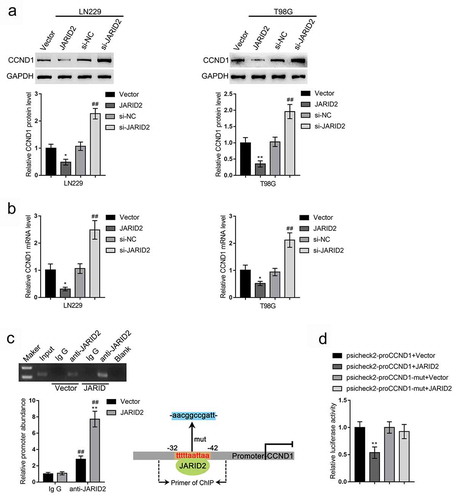
Dynamic effects of the JARID2/CCND1 axis on glioma cell response to TMZ treatment
After confirming the binding between JARID2 and CCND1, finally, we investigated the dynamic effects of the JARID2/CCND1 axis on the response of glioma cells to TMZ treatment. We co-transfected LN229 and T98G cell lines with JARID2 and CCND1-overexpressing vector, exposed to 100 μM TMZ, and determined JARID2 and CCND1 protein contents. shows that, under TMZ treatment, JARID2 overexpression increased JARID2 but decreased CCND1 protein levels, whereas CCND1 overexpression increased CCND1 protein levels but caused no changes in JARID2; CCND1 overexpression partially reversed the effects of JARID2 overexpression on CCND1 protein but caused no changes in JARID2 overexpression effects on JARID2 protein. These data indicate that CCND1 is downstream of JARID2 and could reverse the effects of JARID2 on CCND1.
Figure 5. Dynamic effects of the JARID2/CCND1 axis on glioma cell response to TMZ treatment. LN229 and T98G cells were co-transfected with JARID2 and CCND1-overexpressing vector, exposed to 100 μM TMZ, and examined for the protein levels of JARID2 and CCND1 by immunoblotting (a); cell apoptosis by flow cytometry (b); the protein levels of p21, cleaved-PARP, PARP, cleaved-caspase3, and caspase3 by immunoblotting (c). LN229 and T98G cells were only transfected with JARID2 or/and CCND1-overexpressing vector and examined for cell cycle by flow cytometry (d). **P < 0.01, ##P < 0.01
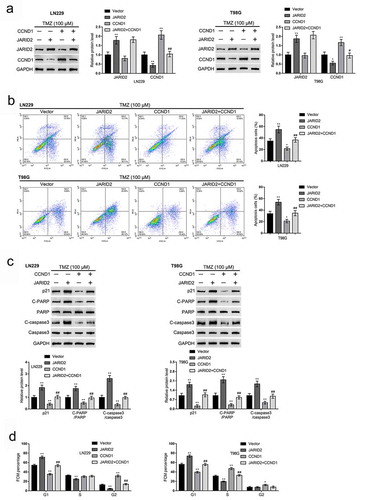
Regarding the cellular functions, under TMZ treatment, JARID2 overexpression promoted cell apoptosis () and upregulated p21, cleaved-PARP/PARP, and cleaved-caspase3/caspase3 protein contents (). On the contrary, under TMZ treatment, CCND1 overexpression inhibited cell apoptosis () and decreased p21, cleaved-PARP/PARP, and cleaved-caspase3/caspase3 protein contents (). Without TMZ treatment, overexpression of JARID2 increased the cell proportion at G0/G1 phase and decreased cell proportion at S and G2/M phases, and CCND1 overexpression inhibited the cell proportion at G0/G1 phase and increased cell proportion at S and G2/M phases ( and Figure S3B). The effects of JARID2 overexpression were partially reversed by CCND1 overexpression.
Discussion
Herein, the study demonstrated that JARID2 was downregulated and CCND1 was upregulated within glioma tissues of different grades and glioma cells. In tissue samples, JARID2 was negatively correlated with CCND1. JARID2 overexpression significantly inhibited glioma cell viability, promoted glioma cell apoptosis upon TMZ treatment, and increased p21, cleaved-PARP, and cleaved-caspase3 in TMZ-treated glioma cells. JASPAR tool predicted the possible binding sites between JARID2 and CCND1 promoter region; through direct binding to CCND1 promoter region, JARID2 negatively regulated CCND1 expression. Under TMZ treatment, JARID2 overexpression inhibited CCND1 expression, promoted glioma cell apoptosis, and increased p21, cleaved-PARP, and cleaved-caspase3 in glioma cells treated with TMZ; meanwhile, CCND1 overexpression exerted opposite effects on glioma cells treated with TMZ and partially reversed the effects of JARID2 overexpression.
JARID2 plays a multifaceted role in different cancers. The tumor-suppressive role of JARID2 has been reported in myeloid neoplasms,Citation20 leukemia,Citation27,Citation29 and B-cell lymphoma.Citation30 As for the expression levels, we observed frequently deleted JARID2 within the process of chronic myeloid malignant tumors transforming to leukemia.Citation31 Herein, both online data and experimental analyses on glioma tissue samples and cell lines indicated the aberrant downregulation of JARID2, particularly in high-grade glioma tissues. Currently, clinical first-line treatment of glioma is surgery combined with anti-neoplastic treatment including TMZ and radiotherapy,Citation32 while the chemoresistance of TMZ is a major issue in the management of patients with glioma.Citation33 Various studies had found that multiple genes could regulate TMZ sensitivity and thus play an important role in the progression of glioma. Wen et al. reported that loss of ATG4C inhibits gliomas progression and promotes TMZ chemosensitivity.Citation34 Bao et al. proposed that knockdown of SLC34A2 elevates chemosensitivity in glioma.Citation35 In the current study, it was the first time to observe the upregulation of JARID2 in TMZ-sensitive glioma cells, hinting JARID2 may exert a crucial role in the TMZ sensitivity of glioma. In the meantime, we also observed the abnormal upregulation of CCND1, a key regulator of cell cycle G1/S phase transformation and an identified oncogenic factor in gliomaCitation24 in glioma tissue samples and cell lines. Considering JARID2 upregulation in TMZ-sensitive glioma cells and that higher JARID2 associates with better survival in patients with glioma, JARID2 might serve as a tumor suppressor in glioma by affecting glioma sensitivity to TMZ, possibly in a CCND1-related way.
Indeed, when JARID2 was overexpressed in glioma cells, cell viability was inhibited; moreover, under TMZ treatment, JARID2 overexpression significantly promoted glioma cell apoptosis, confirming that JARID2 suppresses glioma cell growth and enhances TMZ cytotoxicity on glioma cells. Although the apoptosis signaling contributes to maintaining the balance between cell survival and death, the dysfunction of pro-apoptotic and anti-apoptotic factors promotes the resistance to chemotherapy via inhibiting cell apoptosis. Further evidence suggests that agents inducing pro-apoptotic factors to promote apoptosis, such as p21, caspase3, and PARP, could play a role in sensitizing glioma cells with resistance to TMZ treatment.Citation36–42 Thus, we examined the function of JARID2 overexpression upon the above factors and confirmed that JARID2 overexpression promoted p21, cleaved-caspase3, and cleaved-PARP protein contents. Conversely, JARID2 knockdown reduced p21, cleaved-caspase3, and cleaved-PARP. Therefore, we considered that JARID2 overexpression elevated glioma cell sensitivity to TMZ through regulating apoptosis.
Notably, in addition to JARID2, the deregulation of CCND1 was also observed in glioma tissues and cells. Similar to our observation, Ma et al. reported that CCND1 affected glioma cell proliferation and cell cycle G1/S transition and regulated by miR-519d-3p.Citation43 Considering that JARID2 exerts its biological roles in cancers through regulating gene silencing,Citation20,Citation23 and JARID2 could regulate CCND1 expression in multiple diseases,Citation27,Citation28 we checked whether JARID2 could bind to the CCND1 promoter region using JASPAR and found possible binding sites. Further experimental analyses also indicated that through direct binding, JARID2 negatively regulated CCND1 expression, whereas CCND1 overexpression failed to alter the effects of JARID2 overexpression on JARID2 protein levels, confirming that CCND1 was the downstream target of JARID2. In TMZ-treated glioma cells, CCND1 overexpression inhibited cell apoptosis and decreased the levels of p21, cleaved-caspase3, and cleaved-PARP1. As a downstream factor, CCND1 overexpression partially reversed the effects of JARID2 overexpression on TMZ-treated glioma cells.
In conclusion, JARID2 targets and inhibits CCND1. The JARID2/CCND1 axis modulates glioma cell growth and glioma cell sensitivity to TMZ.
Ethical approval and consent to participate
All procedures performed in studies involving human participants were in accordance with the ethical standards of Xiangya Hospital (approval no. 201803393) and with the 1964 Helsinki Declaration. Informed consent to participate in the study has been obtained from participants.
Consent for publication
Consent for publication was obtained from the participants.
Availability of data and materials
The datasets used and analyzed during the current study are available from the corresponding author on reasonable request.
Supplemental Material
Download Zip (2 MB)Disclosure statement
No potential conflict of interest was reported by the author(s).
Supplementary material
Supplemental data for this article can be accessed on the publisher’s website.
Additional information
Funding
References
- Ostrom QT, Gittleman H, Fulop J, Liu M, Blanda R, Kromer C, Wolinsky Y, Kruchko C, Barnholtz-Sloan JS. 2015. CBTRUS statistical report: primary brain and central nervous system tumors diagnosed in the United States in 2008–2012. Neuro Oncol. 17(Suppl 4):iv1–iv62. doi:10.1093/neuonc/nov189.
- Ostrom QT, Gittleman H, De Blank PM, Finlay JL, Gurney JG, McKean-Cowdin R, Stearns DS, Wolff JE, Liu M, Wolinsky Y, et al. 2016. American brain tumor association adolescent and young adult primary brain and central nervous system tumors diagnosed in the United States in 2008-2012. Neuro Oncol. 18(Suppl 1):i1–i50. doi:10.1093/neuonc/nov297.
- Zanders ED, Svensson F, Bailey DS. 2019. Therapy for glioblastoma: is it working? Drug Discov Today. 24(5):1193–1201. doi:10.1016/j.drudis.2019.03.008.
- Gilbert MR, Dignam JJ, Armstrong TS, Wefel JS, Blumenthal DT, Vogelbaum MA, Colman H, Chakravarti A, Pugh S, Won M, et al. 2014. A randomized trial of bevacizumab for newly diagnosed glioblastoma. N Engl J Med. 370(8):699–708. doi:10.1056/NEJMoa1308573.
- Weller M, van den Bent M, Hopkins K, Tonn JC, Stupp R, Falini A, Cohen-Jonathan-Moyal E, Frappaz D, Henriksson R, Balana C, et al. 2014. EANO guideline for the diagnosis and treatment of anaplastic gliomas and glioblastoma. Lancet Oncol. 15(9):e395–403. doi:10.1016/S1470-2045(14)70011-7.
- Friedman HS, Kerby T, Calvert H. 2000. Temozolomide and treatment of malignant glioma. Clin Cancer Res. 6(7):2585–2597.
- Karachi A, Dastmalchi F, Mitchell DA, Rahman M. 2018. Temozolomide for immunomodulation in the treatment of glioblastoma. Neuro Oncol. 20(12):1566–1572. doi:10.1093/neuonc/noy072.
- Hombach-Klonisch S, Mehrpour M, Shojaei S, Harlos C, Pitz M, Hamai A, Siemianowicz K, Likus W, Wiechec E, Toyota BD, et al. 2018. Glioblastoma and chemoresistance to alkylating agents: involvement of apoptosis, autophagy, and unfolded protein response. Pharmacol Ther. 184:13–41. doi:10.1061/j.pharmthera.2017.10.017.
- Lu Y, Kwintkiewicz J, Liu Y, Tech K, Frady LN, Su Y-T, Bautista W, Moon SI, MacDonald J, Ewend MG, et al. 2017. Chemosensitivity of IDH1-Mutated Gliomas due to an impairment in PARP1-Mediated DNA repair. Cancer Res. 77(7):1709–1718. doi:10.1158/0008-5472.CAN-16-2773.
- SongTao Q, Lei Y, Si G, YanQing D, HuiXia H, XueLin Z, LanXiao W, Fei Y. 2012. IDH mutations predict longer survival and response to temozolomide in secondary glioblastoma. Cancer Sci. 103(2):269–273. doi:10.1111/j.1349-7006.2011.02134.x.
- Houillier C, Wang X, Kaloshi G, Mokhtari K, Guillevin R, Laffaire J, Paris S, Boisselier B, Idbaih A, Laigle-Donadey F, et al. 2010. IDH1 or IDH2 mutations predict longer survival and response to temozolomide in low-grade gliomas. Neurology. 75(17):1560–1566. doi:10.1212/WNL.0b013e3181f96282.
- Lin L, Cai J, Tan Z, Meng X, Li R, Li Y, Jiang C. 2021. Mutant IDH1 Enhances Temozolomide Sensitivity via Regulation of the ATM/CHK2 Pathway in Glioma. Cancer Res Treat. 53(2):367–377. doi:10.4143/crt.2020.506
- Tran AN, Lai A, Li S, Pope WB, Teixeira S, Harris RJ, Woodworth DC, Nghiemphu PL, Cloughesy TF, Ellingson BM. 2014. Increased sensitivity to radiochemotherapy in IDH1 mutant glioblastoma as demonstrated by serial quantitative MR volumetry. Neuro Oncol. 16(3):414–420. doi:10.1093/neuonc/not198
- Dang L, White DW, Gross S, Bennett BD, Bittinger MA, Driggers EM, Fantin VR, Jang HG, Jin S, Keenan MC, et al. 2010. Cancer-associated IDH1 mutations produce 2-hydroxyglutarate. Nature. 465(7300):966. doi:10.1038/nature09132.
- Chowdhury R, Yeoh KK, Tian Y-M, Hillringhaus L, Bagg EA, Rose NR, Leung IKH, Li XS, Woon ECY, Yang M, et al. 2011. The oncometabolite 2-hydroxyglutarate inhibits histone lysine demethylases. EMBO Rep. 12(5):463–469. doi:10.1038/embor.2011.43.
- Lu C, Thompson CB. 2012. Metabolic regulation of epigenetics. Cell Metab. 16(1):9–17. doi:10.1016/j.cmet.2012.06.001.
- Xu W, Yang H, Liu Y, Yang Y, Wang P, Kim S-H, Ito S, Yang C, Wang P, Xiao M-T, et al. 2011. Oncometabolite 2-hydroxyglutarate is a competitive inhibitor of alpha-ketoglutarate-dependent dioxygenases. Cancer Cell. 19(1):17–30. doi:10.1016/j.ccr.2010.12.014.
- Wang P, Wu J, Ma S, Zhang L, Yao J, Hoadley K, Wilkerson M, Perou C, Guan K-L, Ye D, et al. 2015. Oncometabolite D-2-Hydroxyglutarate inhibits ALKBH DNA repair enzymes and sensitizes IDH mutant cells to alkylating agents. Cell Rep. 13(11):2353–2361. doi:10.1016/j.celrep.2015.11.029.
- Yamashita AS, Da Costa Rosa M, Borodovsky A, Festuccia WT, Chan T, Riggins GJ. 2019. Demethylation and epigenetic modification with 5-azacytidine reduces IDH1 mutant glioma growth in combination with temozolomide. Neuro Oncol. 21(2):189–200. doi:10.1093/neuonc/noy146.
- Celik H, Koh WK, Kramer AC, Ostrander EL, Mallaney C, Fisher DAC, Xiang J, Wilson WC, Martens A, Kothari A, et al. 2018. JARID2 functions as a tumor suppressor in myeloid neoplasms by repressing self-renewal in hematopoietic progenitor cells. Cancer Cell. 34(5):741–756 e8. doi:10.1016/j.ccell.2018.10.008.
- Li G, Margueron R, Ku M, Chambon P, Bernstein BE, Reinberg D. 2010. Jarid2 and PRC2, partners in regulating gene expression. Genes Dev. 24(4):368–380. doi:10.1101/gad.1886410.
- Herz HM, Shilatifard A. 2010. The JARID2-PRC2 duality. Genes Dev. 24(9):857–861. doi:10.1101/gad.1921610.
- Cifuentes-Rojas C, Hernandez A, Sarma K, Lee J. 2014. Regulatory interactions between RNA and polycomb repressive complex 2. Mol Cell. 55(2):171–185. doi:10.1016/j.molcel.2014.05.009.
- Wang J, Wang Q, Cui Y, Liu ZY, Zhao W, Wang CL, Dong Y, Hou L, Hu G, Luo C, et al. 2012. Knockdown of cyclin D1 inhibits proliferation, induces apoptosis, and attenuates the invasive capacity of human glioblastoma cells. J Neurooncol. 106(3):473–484. doi:10.1007/s11060-011-0692-4.
- Arcella A, Oliva MA, Staffieri S, Aalberti S, Grillea G, Madonna M, Bartolo M, Pavone L, Giangaspero F, Cantore G, et al. 2015. In vitro and in vivo effect of human lactoferrin on glioblastoma growth. J Neurosurg. 123(4):1026–1035. doi:10.3171/2014.12.JNS14512.
- Shirato H, Ogawa S, Nakajima K, Inagawa M, Kojima M, Tachibana M, Shinkai Y, Takeuchi T. 2009. A jumonji (Jarid2) protein complex represses cyclin D1 expression by methylation of histone H3-K9. J Biol Chem. 284(2):733–739. doi:10.1074/jbc.M804994200.
- Su C-L, Deng T-R, Shang Z, Xiao Y. 2015. JARID2 inhibits leukemia cell proliferation by regulating CCND1 expression. Int J Hematol. 102(1):76–85. doi:10.1007/s12185-015-1797-x.
- Nakajima K, Inagawa M, Uchida C, Okada K, Tane S, Kojima M, Kubota M, Noda M, Ogawa S, Shirato H, et al. 2011. Coordinated regulation of differentiation and proliferation of embryonic cardiomyocytes by a jumonji (Jarid2)-cyclin D1 pathway. Development. 138(9):1771–1782. doi:10.1242/dev.059295.
- Mayer RL, Schwarzmeier JD, Gerner MC, Bileck A, Mader JC, Meier-Menches SM, Gerner SM, Schmetterer KG, Pukrop T, Reichle A, et al. 2018. Proteomics and metabolomics identify molecular mechanisms of aging potentially predisposing for chronic lymphocytic leukemia. Mol Cell Proteomics. 17(2):290–303. doi:10.1074/mcp.RA117.000425.
- Slezak-Prochazka I, Kluiver J, de Jong D, Smigielska-Czepiel K, Kortman G, Winkle M, Rutgers B, Koerts J, Visser L, Diepstra A, et al. 2016. Inhibition of the miR-155 target NIAM phenocopies the growth promoting effect of miR-155 in B-cell lymphoma. Oncotarget. 7(3):2391–2400. doi:10.18632/oncotarget.6165.
- Puda A, Milosevic JD, Berg T, Klampfl T, Harutyunyan AS, Gisslinger B, Rumi E, Pietra D, Malcovati L, Elena C, et al. 2012. Frequent deletions of JARID2 in leukemic transformation of chronic myeloid malignancies. Am J Hematol. 87(3):245–250. doi:10.1002/ajh.22257.
- Jiang T, Nam D-H, Ram Z, Poon W-S, Wang J, Boldbaatar D, Mao Y, Ma W, Mao Q, You Y, et al. 2021. Clinical practice guidelines for the management of adult diffuse gliomas. Cancer Lett. 499:60–72. doi:10.1016/j.canlet.2020.10.050.
- Han B, Meng X, Wu P, Li Z, Li S, Zhang Y, Zha C, Ye Q, Jiang C, Cai J, et al. 2020. ATRX/EZH2 complex epigenetically regulates FADD/PARP1 axis, contributing to TMZ resistance in glioma. Theranostics. 10(7):3351–3365. doi:10.7150/thno.41219.
- Wen Z-P, Zeng W-J, Chen Y-H, Li H, Wang J-Y, Cheng Q, Yu J, Zhou -H-H, Liu -Z-Z, Xiao J, et al. 2019. Knockdown ATG4C inhibits gliomas progression and promotes temozolomide chemosensitivity by suppressing autophagic flux. J Exp Clin Cancer Res. 38(1):298. doi:10.1186/s13046-019-1287-8.
- Bao Z, Chen L, Guo S. 2019. Knockdown of SLC34A2 inhibits cell proliferation, metastasis, and elevates chemosensitivity in glioma. J Cell Biochem. 120(6):10205–10214. doi:10.1002/jcb.28305.
- Li M, Liang RF, Wang X, Mao Q, Liu YH. 2017. BKM120 sensitizes C6 glioma cells to temozolomide via suppression of the PI3K/Akt/NF-kappaB/MGMT signaling pathway. Oncol Lett. 14(6):6597–6603. doi:10.3892/ol.2017.7034.
- Cao Y, Li X, Kong S, Shang S, Qi Y. 2020. CDK4/6 inhibition suppresses tumour growth and enhances the effect of temozolomide in glioma cells. J Cell Mol Med. 24(9):5135–5145. doi:10.1111/jcmm.15156.
- Sumorek-Wiadro J, Zając A, Bądziul D, Langner E, Skalicka-Woźniak K, Maciejczyk A, Wertel I, Rzeski W, Jakubowicz-Gil J. 2020. Coumarins modulate the anti-glioma properties of temozolomide. Eur J Pharmacol. 881:173207. doi:10.1016/j.ejphar.2020.173207.
- Wu Y, Dong L, Bao S, Wang M, Yun Y, Zhu R. 2016. FK228 augmented temozolomide sensitivity in human glioma cells by blocking PI3K/AKT/mTOR signal pathways. Biomed Pharmacother. 84:462–469. doi:10.1016/j.biopha.2016.09.051.
- Shao N, Mao J, Xue L, Wang R, Zhi F, Lan Q. 2019. Carnosic acid potentiates the anticancer effect of temozolomide by inducing apoptosis and autophagy in glioma. J Neurooncol. 141(2):277–288. doi:10.1007/s11060-018-03043-5.
- Liu X, Kang J, Liu F, Wen S, Zeng X, Liu K, Luo Y, Ji X, Zhao S. 2016. Overexpression of iASPP-SV in glioma is associated with poor prognosis by promoting cell viability and antagonizing apoptosis. Tumour Biol. 37(5):6323–6330. doi:10.1007/s13277-015-4503-y.
- Chen R, Liu H, Cheng Q, Jiang B, Peng R, Zou Q, Yang W, Yang X, Wu X, Chen Z, et al. 2016. MicroRNA-93 promotes the malignant phenotypes of human glioma cells and induces their chemoresistance to temozolomide. Biol Open. 5(6):669–677. doi:10.1242/bio.015552.
- Ma L, Li J. 2020. MicroRNA-519d-3p inhibits cell proliferation and cell cycle G1/S transition in glioma by targeting CCND1. Biosci Biotechnol Biochem. 84(2):297–304. doi:10.1080/09168451.2019.1682510.