Abstract
The Target Of Rapamycin (TOR) is an evolutionarily conserved protein kinase that forms 2 distinct protein complexes referred to as TOR complex 1 (TORC1) and 2 (TORC2). Recent extensive studies have demonstrated that TORC1 is under the control of the small GTPases Rheb and Rag that funnel multiple input signals including those derived from nutritional sources; however, information is scarce as to the regulation of TORC2. A previous study using the model system provided by the fission yeast Schizosaccharomyces pombe identified Ryh1, a Rab-family GTPase, as an activator of TORC2. Here, we show that the nucleotide-binding state of Ryh1 is regulated in response to glucose, mediating this major nutrient signal to TORC2. In glucose-rich growth media, the GTP-bound form of Ryh1 induces TORC2-dependent phosphorylation of Gad8, a downstream target of TORC2 in fission yeast. Upon glucose deprivation, Ryh1 becomes inactive, which turns off the TORC2-Gad8 pathway. During glucose starvation, however, Gad8 phosphorylation by TORC2 gradually recovers independently of Ryh1, implying an additional TORC2 activator that is regulated negatively by glucose. The paired positive and negative regulatory mechanisms may allow fine-tuning of the TORC2-Gad8 pathway, which is essential for growth under glucose-limited environment.
Keywords:
Introduction
The Target of Rapamycin (TOR) is an evolutionarily conserved serine/threonine kinase that forms 2 distinct protein complexes referred to as TOR Complex 1 (TORC1) and TOR Complex 2 (TORC2).Citation1-5 While TORC1 and TORC2 share the catalytic subunit TOR kinase, their specific regulatory subunits confer discrete physiological functions to the 2 complexes. Thus, TORC1 and TORC2 control distinct sets of downstream targets under different regulations.Citation6-13
To elucidate the function and regulation of each TOR complex, extensive studies have been conducted in a variety of model organisms. Among those is the fission yeast Schizosaccharomyces pombe, a genetically amenable eukaryote with nonessential TORC2, which eases mutational analysis of this particular TOR complex.Citation14-21 Fission yeast TORC2 is composed of the catalytic subunit Tor1 kinase and the regulatory subunits named Ste20, Sin1, Wat1 and Bit61.Citation17,19,22 The key downstream target of TORC2 in fission yeast is the AGC-family kinase Gad8, as evidenced by the observation that the gad8 deletion mutant phenocopies the TORC2-defective mutants.Citation16,19 Like Akt activation by mammalian TORC2, Gad8 is phosphorylated within its hydrophobic motif by TORC2, resulting in increased Gad8 kinase activity essential for its cellular functions.Citation16,19 On the other hand, a major upstream regulator of fission yeast TORC2 has been found as the Rab small GTPase Ryh1.Citation21 The GTP-bound, active form of Ryh1 physically interacts with TORC2 and promotes TORC2-dependent phosphorylation/activation of the Gad8 kinase.
In diverse eukaryotic species, TORC1 controls cellular anabolic and catabolic processes, such as translation and autophagy, in response to nutrients and other extracellular stimuli.Citation6,23-31 Fission yeast TORC1 is activated in the presence of nitrogen and carbon sources, and phosphorylates the major S6 kinase Psk1.Citation22,32-34 In this organism, TORC2 is also implicated in cellular nutritional responses, including the G1 arrest upon nitrogen starvation and the mitotic control in response to glucose.Citation16,19,35 Little is known, however, about the physiological stimuli that regulate fission yeast TORC2, whereas insulin and growth factors activate mammalian TORC2 (mTORC2) in a PI3K-dependent manner.Citation7,8,Citation36-38
In this report, we show that TORC2 in fission yeast is responsive to glucose and that the functional TORC2-Gad8 pathway is essential for cell proliferation in glucose-limited environment. Our mutational analysis indicated that the glucose regulation of fission yeast TORC2 is not dependent on the cAMP pathway, a signaling mechanism known to be responsive to extracellular glucose.Citation39-42 Importantly, glucose-replete conditions increase the active, GTP-bound form of Ryh1 GTPase, which induces TORC2-dependent phosphorylation of Gad8. Glucose starvation swiftly inactivates Ryh1 and suppresses the TORC2-Gad8 pathway, which is, however, gradually reactivated in a manner independent of Ryh1. Thus, the fission yeast TORC2-Gad8 pathway appears to be controlled by Ryh1-dependent and -independent mechanisms that monitor the availability of glucose, a major carbon/energy source for fission yeast.
Results
TORC2 remains active during nitrogen starvation
When starved of nitrogen, fission yeast arrests the cell cycle in G1, a prerequisite for mating and meiosis.Citation43 Nitrogen starvation inhibits the TORC1 activity, and mutational inactivation of TORC1 provokes phenotypes similar to those of nitrogen-starved cells, including G1 arrest and sexual development.Citation18,22,32,44 To examine whether the TORC2 activity is also regulated in response to nitrogen availability, exponentially growing wild-type cells in the synthetic EMM medium were shifted to the same medium lacking the nitrogen source ammonium chloride (EMM-N). As reported previously,Citation34 the TORC1-dependent phosphorylation of the S6 kinase Psk1 diminished and became undetectable within 30 min (). In contrast, the TORC2-dependent phosphorylation of Gad8-Ser546 within the hydrophobic motifCitation21 was detected along the time course of the experiment, and the phosphorylation rose to somewhat higher levels after 60 min. For up to 12 hours after shifting to the EMM-N medium, during which most of wild-type cells arrest in G1,Citation45 the hydrophobic motif of Gad8 remained phosphorylated (). The proteolytic processing of the GFP-Atg8 fusion protein, an autophagic marker, also confirmed inactivation of TORC1 during the experiment (, bottom).Citation46,47
Figure 1. Gad8 phosphorylation at the hydrophobic motif persists during nitrogen starvation. (A) A prototrophic, wild-type fission yeast strain (972) was cultured in EMM at 30°C to the early log phase and starved of nitrogen sources. Aliquots of cells were harvested at the indicated times during nitrogen starvation. The sample at 0 minute was taken immediately before nitrogen sources were depleted in the growth medium. Crude cell lysate was prepared with TCA and examined in immunoblotting with antibodies against phospho-Gad8 (P-Gad8), Gad8,Citation21 and phospho-human S6K (P-Psk1).Citation34 (B) A wild-type strain (CA101) transformed with the GFP-atg8 plasmid was cultured in EMM lacking thiamine and nitrogen-starved at 30°C. Cells were harvested at the indicated times after nitrogen depletion. Crude cell lysate was prepared with TCA and immunoblotted with antibodies against phospho-Gad8 (P-Gad8), Gad8, phospho-human S6K (P-Psk1), and GFP.Citation46
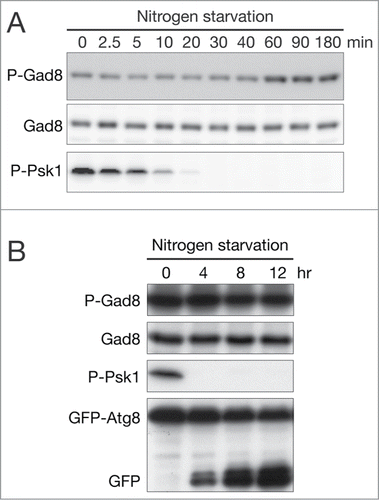
These results strongly suggest that TORC2 remains active in fission yeast cells starved of nitrogen, consistent with the observation that the activity of TORC2 and Gad8 is essential for the cell cycle arrest and sexual development after nitrogen starvation.Citation14-16,Citation19,48
The TORC2-Gad8 pathway is responsive to glucose
It has been reported that the mitotic control under low glucose conditions is altered in a strain carrying the tor1-L2045D mutation of the TORC2 catalytic subunit.Citation35 Therefore, we examined whether TORC2 activity is responsive to glucose, by shifting exponentially growing cells from the growth medium containing 3% glucose to the same medium lacking glucose. Within 5 min, Gad8 with slower electrophoretic mobility, which reflects the TORC2-dependent phosphorylation of the hydrophobic motif,Citation16,19 became undetectable (). Consistently, the in vitro kinase assayCitation19 confirmed significant reduction of the Gad8 activity after glucose starvation (). Furthermore, adding glucose back to the glucose-starved culture restored the Gad8 phosphorylation and catalytic activity (, "+G"). Immunoblotting with anti-phospho-Ser546 antibodies confirmed the glucose regulation of the Gad8 phosphorylation in the hydrophobic motif (). Indeed, phosphorylation of the hydrophobic motif is crucial to glucose-induced activation of Gad8, and alanine-substitution of Ser546 largely abrogated Gad8 activity under glucose replete conditions (Fig. S1).
Figure 2. Gad8 is immediately dephosphorylated upon glucose depletion in the growth medium. (A) A gad8:6HA strain (CA4770) was cultured in YES at 30°C to the early log phase and harvested at the indicated minutes after glucose depletion from the growth medium. The sample at 0 minute was taken just prior to glucose depletion. The culture was split into 2 at 20 minutes and glucose was added at the final concentration of 3% to one of the split cultures (labeled with "+G"), followed by 5 minutes incubation. Crude cell lysate was prepared with TCA and analyzed by anti-HA immunoblotting to detect slower migrating, phosphorylated Gad8 (P-Gad8).Citation16 Gad8 kinase activity was analyzed in an in vitro assay using immunopurified Gad8–6HA, bacterially produced GST-Fkh2, and [γ-32P]ATP.Citation19 Relative radioactivity in the assay was indicated as Gad8 kinase activity with the activity at 0 minute fit to 100%. A wild-type strain that expresses untagged Gad8 (CA101) was used as a negative control in the assay (the rightmost lane labeled with "N"). (B) A wild type strain (CA101) grown in YES was starved of glucose. Twenty minutes after glucose depletion, glucose was added back to an aliquot of the culture and incubated for another 10 minutes (labeled with "+G"). Crude cell lysate was prepared with TCA and Gad8 phosphorylation was analyzed as in Figure 1A. (C) A wild type strain (CA101) cultured in regular EMM containing 2% glucose was harvested before and after shifting to EMM containing the indicated concentrations of glucose. Gad8 phosphorylation was analyzed as in Figure 1A. (D) A wild type strain (972) was cultured in YES and starved of glucose for 20 minutes, followed by 5 minutes incubation in the absence ("-") or in the presence ("+G") of 0.2% glucose or in the presence of 0.2% 2-deoxyglucose ("+2DG"). Gad8 phosphorylation was analyzed as in Figure 1A. Relative levels of Gad8 phosphorylation were indicated below the "P-Gad8" panel with the level at 0 minute fit to 100%.
![Figure 2. Gad8 is immediately dephosphorylated upon glucose depletion in the growth medium. (A) A gad8:6HA strain (CA4770) was cultured in YES at 30°C to the early log phase and harvested at the indicated minutes after glucose depletion from the growth medium. The sample at 0 minute was taken just prior to glucose depletion. The culture was split into 2 at 20 minutes and glucose was added at the final concentration of 3% to one of the split cultures (labeled with "+G"), followed by 5 minutes incubation. Crude cell lysate was prepared with TCA and analyzed by anti-HA immunoblotting to detect slower migrating, phosphorylated Gad8 (P-Gad8).Citation16 Gad8 kinase activity was analyzed in an in vitro assay using immunopurified Gad8–6HA, bacterially produced GST-Fkh2, and [γ-32P]ATP.Citation19 Relative radioactivity in the assay was indicated as Gad8 kinase activity with the activity at 0 minute fit to 100%. A wild-type strain that expresses untagged Gad8 (CA101) was used as a negative control in the assay (the rightmost lane labeled with "N"). (B) A wild type strain (CA101) grown in YES was starved of glucose. Twenty minutes after glucose depletion, glucose was added back to an aliquot of the culture and incubated for another 10 minutes (labeled with "+G"). Crude cell lysate was prepared with TCA and Gad8 phosphorylation was analyzed as in Figure 1A. (C) A wild type strain (CA101) cultured in regular EMM containing 2% glucose was harvested before and after shifting to EMM containing the indicated concentrations of glucose. Gad8 phosphorylation was analyzed as in Figure 1A. (D) A wild type strain (972) was cultured in YES and starved of glucose for 20 minutes, followed by 5 minutes incubation in the absence ("-") or in the presence ("+G") of 0.2% glucose or in the presence of 0.2% 2-deoxyglucose ("+2DG"). Gad8 phosphorylation was analyzed as in Figure 1A. Relative levels of Gad8 phosphorylation were indicated below the "P-Gad8" panel with the level at 0 minute fit to 100%.](/cms/asset/4d154f00-5a75-4a4a-8de7-5fcfffe93d6c/kccy_a_1000215_f0002_oc.gif)
The standard yeast growth media contain 2∼3% glucose, but the doubling time of fission yeast is not significantly different in growth media with only 0.2% (11.1 mM) glucose; however, when the glucose concentration is reduced to 0.02%, most cells are starved and stop dividing with high viability.Citation49 Consistently, when cells grown in the presence of 3% glucose were transferred to the same medium with 0.2% glucose, the Gad8 phosphorylation was unaltered (). On the other hand, Gad8 phosphorylation disappeared within 5 min when cells were transferred to the 0.02% glucose medium. Thus, the TORC2 activity responsible for the Gad8-S546 phosphorylation appears to be responsive to glucose concentrations that affect cell physiology.
2-deoxy-D-glucose (2-DG) is imported into the cell via glucose transporters and phosphorylated by hexokinase, without undergoing further glycolysis.Citation50 In contrast to 0.2% glucose, which induced the Gad8-S546 phosphorylation in glucose-starved cells, the same concentration of 2-DG failed to recover the Gad8 phosphorylation at all (). Thus, it appears that glucose needs to be metabolized in the cell to stimulate the TORC2 pathway.
Glucose-regulation of the Ryh1 GTPase contributes to TORC2 activation
How do glucose signals control TORC2 activity? It has been reported that, in cultured human cell lines, the integrity of mTOR complexes is severely impaired after glucose deprivation due to destabilization of the SIN1 and Rictor proteins.Citation51 In glucose-starved S. pombe cells, we found that the abundance of Sin1 and the Rictor ortholog Ste20 did not fluctuate significantly (Fig. S2). Affinity purification of TORC2 from the cell lysate before and after glucose starvation showed no striking change in the interaction between Tor1 and other TORC2 subunits (), except a modest, gradual decrease of Sin1 (Fig. S2).
Figure 3. The TORC2 integrity is unaltered on glucose depletion and Gad8 phosphorylation is unaffected by loss of the glucose-responsive cAMP-PKA pathway. (A) FLAG:tor1 (CA6530), FLAG:tor1 sin1:myc (CA7092), FLAG:tor1 ste20:myc (CA7087), and FLAG:tor1 wat1:myc (CA7213) strains cultured in YES were harvested before ("+") and 5 minutes after ("–") glucose depletion. Anti-myc immunoprecipitation was performed with non-denaturing crude cell lysate, followed by anti-FLAG and anti-myc immunoblotting to detect FLAG-Tor1 and the myc tagged TORC2 components, respectively. Gad8 phosphorylation was analyzed as in Figure 1A in crude cell lysate prepared with TCA. (B) bit61:myc (CA6859) and bit61:myc FLAG:tor1 (CA6855) strains were cultured in YES and treated for glucose starvation for 5 minutes. Anti-FLAG immunoprecipitates and input were analyzed as in Figure 3A. (C) Wild-type (CA3), Δgit3 (CA5657), Δgpa2 (CA5669), Δcyr1 (CA5647), Δpka1 (CA5603), Δcgs2 (CA5605), and Δgad8 (CA5142) strains grown in YES were examined for Gad8 phosphorylation as in Figure 1A. (D) A wild-type strain (972) was cultured in YES and starved of glucose for 20 minutes, followed by 5 minutes incubation in the presence of 0.2% glucose or 5 mM cAMP. Gad8 phosphorylation was analyzed as in Figure 1A.
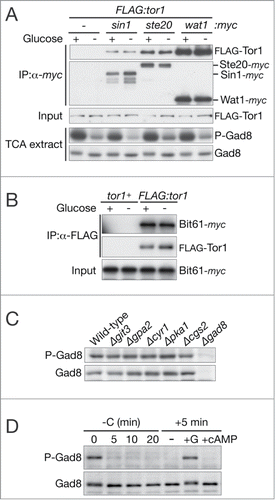
In fission yeast, extracellular glucose activates the cAMP-PKA signaling pathway, of which constituents include the glucose-sensor Git3 GPCR, the Gpa2 subunit of the heterotrimeric G protein, the adenylate cyclase Cyr1, the cAMP phosphodiesterase Cgs2 and the cAMP-dependent Pka1 kinase.Citation52 We found that the TORC2-dependent phosphorylation of Gad8-Ser546 is comparable between the wild-type and any of the strains lacking these components of the cAMP-PKA pathway (). Furthermore, as shown in , the Gad8 phosphorylation was not induced in glucose-starved cells after being treated with 5 mM cAMP, which is known to activate PKA in S. pombe.Citation40 It is therefore unlikely that the cAMP-PKA pathway mediates glucose signals to control the TORC2 activity.
The Ryh1 small GTPase is an upstream regulator of the TORC2-Gad8 pathway and its GTP-bound form is active in stimulating TORC2.Citation21 To determine whether Ryh1 is involved in glucose signaling, we developed a pull-down assay to monitor activation of Ryh1, an ortholog of human Rab6. Bicaudal D2 (BICD2) is an effector of human Rab6,Citation53 and we found that a 109-amino acid fragment of BICD2 immobilized on agarose beads can precipitate GTP-bound, active Ryh1Q70L, but not the GDP-locked Ryh1T25N mutant protein (, lanes 1 and 2). When cells expressing wild-type Ryh1 were transferred to the glucose-free medium, the active form of Ryh1 significantly decreased in 5 min, along with a drop in Gad8 phosphorylation (, lanes 3 and 4). On the other hand, in a strain expressing GTP-locked Ryh1Q70L, Gad8 remained phosphorylated even after glucose depletion, indicating that active Ryh1 is sufficient to maintain the TORC2 activity in the absence of glucose stimuli (). Together, these results suggest that the nucleotide-binding state, and therefore the activity, of the Ryh1 GTPase are responsive to extracellular glucose and that swift inactivation of Ryh1 upon glucose starvation turns off the TORC2 activity to phosphorylate Gad8.
Figure 4. Ryh1 responds to glucose and promotes Gad8 phosphorylation by TORC2. (A) Fission yeast strains expressing FLAG-Ryh1 of wild type ("WT," CA6809), the GTP-locked Q70L mutant (CA6817), or the GDP-locked T25N mutant (CA6828) were cultured in YES (Glucose "+") and subjected to glucose depletion for 5 minutes (Glucose "–"). Bacterially expressed GST-BICD2 was immobilized on glutathione (GSH) beads and used for precipitation of the active, GTP-bound Ryh1 ("active") in non-denaturing crude cell lysate. GST-BICD2 and FLAG-Ryh1 in precipitate were detected by Coomassie brilliant blue G-250 staining and by anti-FLAG immunoblotting, respectively. Gad8 phosphorylation was analyzed as in Figure 1A. (B) A wild type ("WT," CA6809) and a GTP-locked Q70L mutant (CA6817) FLAG-ryh1 strains were cultured in EMM and harvested with glucose depletion treatment. Gad8 phosphorylation was analyzed as in Figure 1A.
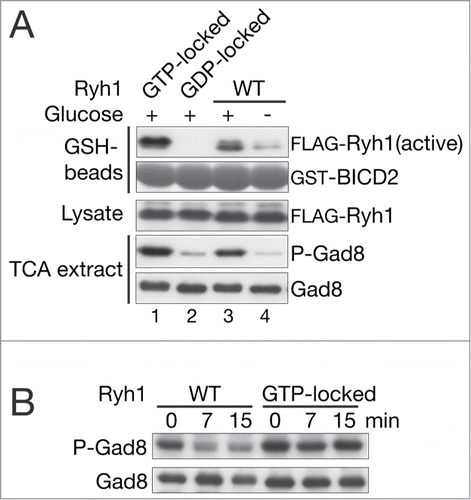
Ryh1-dependent and -independent mechanisms for TORC2 activation
In order to evaluate the contribution of the Ryh1 GTPase to the glucose regulation of TORC2, we compared the TORC2-dependent phosphorylation of Gad8 in wild-type and ryh1 null (Δryh1) mutant cells before and after glucose starvation. In the presence of glucose, Gad8 was significantly less phosphorylated in Δryh1 than in wild-type cells as reported previouslyCitation21 (, time 0), confirming the role of Ryh1 as a TORC2 activator. Within 5 min after shifted to the glucose-free medium, wild-type cells showed significant reduction in Gad8 phosphorylation, due to the inactivation of Ryh1; unexpectedly, however, the phosphorylation gradually recovered to the initial level by the 30-min time point. Also in Δryh1 cells, the Gad8 phosphorylation was induced after glucose starvation to a level comparable to that in wild-type cells grown in the presence of glucose (). Thus, the induced activation of the TORC2-Gad8 pathway after glucose starvation is likely to be independent of the Ryh1 GTPase. Importantly, adding glucose back to the starved Δryh1 cells brought down the Gad8 phosphorylation to the initial level in the presence of glucose (, "+G"), implying that the putative Ryh1-independent activator of TORC2 is negatively regulated by glucose. Similar results were obtained also with the GDP-locked ryh1T25N mutant (Fig. S3).
Figure 5. Gad8 phosphorylation is recovered after glucose depletion independently of Ryh1. (A) Wild-type (CA101) and Δryh1 (CA6217) cells cultured in EMM were starved of glucose and Gad8 phosphorylation was examined as in Figure 1A. (B) Wild type (CA101), Δtor1 (CA4593), and Δgad8 (CA5142) strains were grown at 30°C in YES. At 0 hour, each culture was split into 2 and one of them was subjected to glucose limitation in YES containing 0.1% glucose. Cell proliferation was monitored by measuring OD600 of cultures at approximately 2 hour intervals. Solid lines with filled markers represent strains grown in YES containing 3% glucose, while dotted lines with open markers in YES containing 0.1% glucose. Wild type: circles (•○); Δtor1: triangles (▴▵); Δgad8: squares (▪□). (C) Wild type (CA6809), Δgad8 (CA7366), and the GDP-locked mutant ryh1T25N ("ryh1-GDP," CA6828) strains were grown at 28°C in YES. Cultures were split into 2 at 0 hour, and one of them were subjected to glucose limitation in YES containing 0.15% glucose. Cell proliferation was monitored by measuring OD600 at approximately 3 hour intervals. Solid lines with filled markers represent strains grown in YES containing 3% glucose, while dotted lines with open markers in YES containing 0.15% glucose. Wild type: circles (•○); Δgad8: squares (▪□); ryh1T25N: diamonds (⧫⋄).
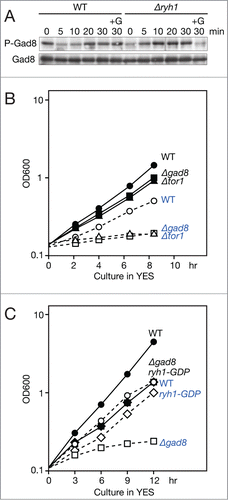
Collectively, when the growth medium is replete with glucose, the GTP-bound, active form of Ryh1 promotes TORC2-Gad8 signaling, while meager supplies of glucose inhibit Ryh1, resulting in reduced TORC2 activity. On the other hand, glucose starvation triggers a Ryh1-independent mechanism that can fully reactivate the TORC2-Gad8 pathway.
Wild-type fission yeast cells can proliferate even when the glucose concentration in the growth medium is lowered to 0.08 ∼0.2%.Citation49 We noticed, however, that the Δtor1 and Δgad8 mutant strains failed to grow under such glucose-limited conditions (), indicating that active TORC2-Gad8 signaling is essential for cellular response to glucose limitation. In contrast, cells carrying an inactive allele of ryh1 proliferated in both high and low glucose media (), consistent with the idea that the Ryh1-independent activation of the TORC2-Gad8 pathway allows cell division under glucose limitation.
Discussion
In this study, we have demonstrated that the fission yeast TORC2-Gad8 pathway is responsive to glucose and plays an essential role in cell proliferation under glucose-limited conditions. Intriguingly, the homologous mTORC2-Akt pathway in animals regulates the cellular uptake and metabolism of glucose in response to insulin;Citation54–56 it is tempting to speculate that insulin signaling in multicellular organisms may have evolved from a glucose-responsive pathway in unicellular eukaryotes. On the other hand, TORC1 in S. pombe is stimulated by nitrogen sources such as ammonium, and its mutational inactivation induces cellular responses similar to those triggered by nitrogen starvation.Citation22,32 Thus, the TOR signaling pathways in fission yeast seem to mediate major nutritional input, nitrogen and carbon/energy.
The molecular mechanism that activates mTORC2 in the insulin signaling pathway remains elusive, though involvement of lipid second messengers and ribosome has been suggested.Citation57-59 In S. pombe, a genetic screen identified the Rab-family GTPase Ryh1 and its guanine nucleotide exchange factor (GEF) as positive regulators of TORC2.Citation21 We have further extended this observation by showing in this paper that the nucleotide-binding state of Ryh1 is regulated in response to glucose. Upon glucose starvation, the GTP-bound, active form of Ryh1 significantly decreases, resulting in diminished TORC2 activity. Furthermore, the TORC2-Gad8 pathway is insensitive to glucose starvation in cells expressing the constitutively active Ryh1Q70L protein, strongly suggesting that the glucose-dependent regulation of Ryh1 controls TORC2 activity.
Comparing to other protein kinases, mTOR requires higher levels of ATP for its catalytic activity.Citation60 In cultured human cells, glucose starvation brings about a gradual decrease of cellular ATP to less than 15% of the normal level, resulting in destabilization of the essential mTORC2 subunits SIN1 and Rictor.Citation51 In contrast, we found that glucose deprivation does not significantly affect the abundance of the TORC2 subunits in fission yeast. The cellular ATP level in fission yeast goes down only to ∼80% at 30 min after a shift from 2% to 0.02% glucose,Citation49 a treatment that markedly reduces Gad8 phosphorylation by TORC2 within 5 min (). Moreover, in cells expressing the Ryh1Q70L mutant protein, TORC2 remains active even after glucose starvation. Thus, reduced cellular ATP levels do not seem to be responsible for the swift inactivation of TORC2 in fission yeast cells deprived of glucose.
Cohen et al. recently reported that glucose signals are mediated via cAMP-PKA to activate the TORC2-Gad8 pathway, because the Gad8-Ser546 phosphorylation was not detectable in the Δgit3, Δgpa2 and Δpka1 mutants even under glucose replete conditions.Citation61 In contrast, our repeated experiments detected no significant defect in the Gad8 phosphorylation among the cAMP-PKA pathway mutants tested in this study, including Δgit3, Δgpa2 and Δpka1. Because Cohen et al. prepared the cellular protein extract under non-denaturing condition without phosphatase inhibitor, it is conceivable that Gad8 was dephosphorylated by phosphatases in the crude extract. They also used strains expressing Gad8 with a C-terminal epitope tag, which could affect their experiment to monitor phosphorylation of the hydrophobic motif close to the C-terminus. In our experiments (), we examined the phosphorylation state of the endogenous Gad8 protein with no tagging. In addition, the lysate was prepared from TCA-fixed cells under denaturing condition in order to prevent protein phosphorylation/dephosphorylation after cell disruption. It should also be noted that not only cAMP but also the glucose analog 2-DG fails to activate the TORC2 pathway; therefore, glucose needs to be metabolized in the cell to stimulate TORC2, rather than being sensed by the cell-surface Git3 GPCR upstream of the cAMP-PKA pathway.
Despite the notable role of the Ryh1 GTPase in mediating glucose-induced activation of TORC2, strains without functional Ryh1 are not sensitive to low-glucose conditions. Indeed, glucose deprivation induces activation of the TORC2-Gad8 pathway in the absence of Ryh1 and therefore, the ryh1-defective cells have enough TORC2-Gad8 activity to grow with limited glucose. In wild-type cells, the TORC2 pathway is inhibited immediately after glucose starvation due to the prompt inactivation of Ryh1, but subsequently, the pathway becomes reactivated. Comparing to the Δryh1 mutant, the reactivation is slower in the wild-type strain, possibly due to the negative feedback regulation,Citation62 in which the initial high activity of Gad8 could delay the TORC2 reactivation. Currently, the mechanism for the observed Ryh1-independent activation of the TORC2-Gad8 pathway is unknown. This novel mechanism appears to be inhibited by glucose, because it is operative only after glucose starvation and, in the absence of Ryh1, added glucose immediately abrogates TORC2-Gad8 signaling induced after glucose starvation.
In summary, our study has revealed the 2 regulatory mechanisms that control the TORC2-Gad8 pathway, which is essential for cellular glucose response. One is the Ryh1 GTPase whose GTP-bound form activates the TORC2 pathway under glucose-rich conditions. The other stimulates TORC2-Gad8 signaling independently of Ryh1 and is negatively regulated by glucose. The pair of these positive and negative regulatory mechanisms for TORC2 may allow fine-tuning of the pathway to optimize the cellular growth under changing glucose conditions. The molecular nature of the glucose signals that are sensed by these mechanisms remains to be elucidated. In addition, the exact function of the TORC2-Gad8 pathway in cellular response to glucose has to be determined. Like mTORC2-Akt, the fission yeast TORC2 pathway may be involved in the uptake and/or metabolism of glucose. It has also been found that TORC2 is required to modulate the mitotic cell cycle in response to low glucose conditions.Citation35 We expect that further investigation of glucose signaling in fission yeast will contribute to the discovery of evolutionarily conserved regulation and function of TORC2 signaling.
Materials and Methods
Fission yeast strains and general techniques
Fission yeast strains used in this study are listed in . Standard growth media, basic techniques, and genetic manipulations of fission yeast have been described.Citation63,64 Crude cell lysate preparation using trichloroacetic acid (TCA) has been reported.Citation65 The Gad8 in vitro kinase assay has been described previously.Citation19 Immunoprecipitation was performed as described before.Citation21 Luminescent Image Analyzer LAS-4000 (Fujifilm) was used for signal quantification in immunoblotting. For nitrogen starvation, cells grown in liquid EMM were filter-harvested and transferred into pre-warmed fresh EMM lacking NH4Cl. For glucose depletion, cells grown in standard YES or EMM were filter-harvested and transferred to glucose free YES or EMM, in which sorbitol was added to maintain medium osmolarity after glucose depletion, except for . For glucose add-back, cells starved of glucose for 20 minutes were filter-harvested and introduced in glucose rich media, followed by 5 or 10 minutes incubation.
Table 1. Fission yeast strains used in this study
Detection of autophagy and Psk1 phosphorylation
Autophagy was monitored as described previously.Citation46 Briefly, the GFP-Atg8 fusion protein, which was expressed exogenously under the control of the thiamine-repressible nmt1 promoter of pREP41Citation66 in the absence of thiamine, was examined in immunoblotting with antibodies against GFP for its autophagy-induced cleavage. Psk1 phosphorylation levels were analyzed as described before.Citation34
Ryh1 pull-down assay
The C-terminal 706 – 814th amino acid region of human Bicaudal D2 (BICD2)Citation53 was fused with glutathione S-transferase (GST) and expressed in the E. coli BL21 strain at 16°C. The fusion protein was purified by glutathione Sepharose chromatography (GE Lifesciences) in lysis buffer (25 mM Tris-HCl [pH 7.5], 137 mM NaCl, 2.7 mM KCl, 1% triton X-100, and 1 mM PMSF). Yeast cells expressing the FLAG epitope tagged Ryh1 were cultured in YES liquid media at 30°C and harvested by filtration. Harvested cells were washed once with ice-cold water and stored at −80°C until use.
Yeast cells were lysed in ice-cold PBS containing 10 mM MgCl2, 0.5% Tween 20, 1 mM PMSF, and the protease inhibitor cocktail for use in purification of histidine-tagged proteins (Sigma #P8849). Crude cell lysate was cleared by centrifugation at 20,400 g for 15 minutes. Resulting supernatant was incubated for 45 minutes with GST-BICD2 immobilized on glutathione beads. Following the incubation, the beads were washed 3 times with PBS containing 10 mM MgCl2 and 0.5% Tween 20. Cell lysis and all the following steps were performed at 4°C.
Disclosure of Potential Conflicts of Interest
No potential conflicts of interest were disclosed.
1000215_Supplementary_Materials.zip
Download Zip (1.2 MB)Acknowledgment
We thank K. Takegawa, M. Uritani, M. Yamamoto, M. Yanagida, National BioResource Yeast Project of Japan for reagents, and T. Fukuda for helpful comments on the manuscript.
Funding
This work was supported by a National Institute of Health grant (K.S.), JSPS KAKENHI Grants 23870019, 26291024, 23770230, 25440086, 21570198, and 26440099 (K.S., H.T., S.M.), Mitsubishi Foundation, Daiichi Sankyo Foundation of Life Science, Sagawa Foundation for Promotion of Cancer Research, and Platform for Drug Discovery, Informatics, and Structural Life Science from the MEXT, Japan. T.H. was a recipient of JSPS Research Fellowship (no. 23-5163) for Young Scientists. S.M. was supported by the Nara Institute of Science and Technology Global Center of Excellence Program funded by MEXT. K.I. was a recipient of the Nihon University 2005/2006 Overseas Scholarship.
Supplemental Material
Supplemental data for this article can be accessed on the publisher's website.
References
- Kim DH, Sarbassov DD, Ali SM, King JE, Latek RR, Erdjument-Bromage H, Tempst P, Sabatini DM. mTOR interacts with raptor to form a nutrient-sensitive complex that signals to the cell growth machinery. Cell 2002; 110: 163-75; PMID:12150925
- Loewith R, Jacinto E, Wullschleger S, Lorberg A, Crespo JL, Bonenfant D, Oppliger W, Jenoe P, Hall MN. Two TOR complexes, only one of which is rapamycin sensitive, have distinct roles in cell growth control. Mol Cell 2002; 10:457-68; PMID:12408816
- Hara K, Maruki Y, Long X, Yoshino K, Oshiro N, Hidayat S, Tokunaga C, Avruch J, Yonezawa K. Raptor, a binding partner of target of rapamycin (TOR), mediates TOR action. Cell 2002; 110:177-89; PMID:12150926
- Sarbassov DD, Ali SM, Kim DH, Guertin DA, Latek RR, Erdjument-Bromage H, Tempst P, Sabatini DM. Rictor, a novel binding partner of mTOR, defines a rapamycin-insensitive and raptor-independent pathway that regulates the cytoskeleton. Curr Biol 2004; 14:1296-302; PMID:15268862; http://dx.doi.org/10.1016/j.cub.2004.06.054
- Wullschleger S, Loewith R, Hall MN. TOR signaling in growth and metabolism. Cell 2006; 124:471-84; PMID:16469695; http://dx.doi.org/10.1016/j.cell.2006.01.016
- Gingras A-C, Raught B, Sonenberg N. Regulation of translation initiation by FRAP/mTOR. Genes Dev 2001; 15:807-26; PMID:11297505; http://dx.doi.org/10.1101/gad.887201
- Sarbassov DD, Guertin DA, Ali SM, Sabatini DM. Phosphorylation and regulation of Akt/PKB by the rictor-mTOR complex. Science 2005; 307:1098-101; PMID:15718470; http://dx.doi.org/10.1126/science.1106148
- Guertin DA, Stevens DM, Thoreen CC, Burds AA, Kalaany NY, Moffat J, Brown M, Fitzgerald KJ, Sabatini DM. Ablation in mice of the mTORC components raptor, rictor, or mLST8 reveals that mTORC2 is required for signaling to Akt-FOXO and PKCalpha, but not S6K1. Dev Cell 2006; 11:859-71; PMID:17141160; http://dx.doi.org/10.1016/j.devcel.2006.10.007
- García-Martínez JM, Alessi DR. mTOR complex 2 (mTORC2) controls hydrophobic motif phosphorylation and activation of serum- and glucocorticoid-induced protein kinase 1 (SGK1). Biochem J 2008; 416:375-85; PMID:18925875; http://dx.doi.org/10.1042/BJ20081668
- Ikenoue T, Inoki K, Yang Q, Zhou X, Guan KL. Essential function of TORC2 in PKC and Akt turn motif phosphorylation, maturation and signalling. EMBO J 2008; 27:1919-31; PMID:18566587; http://dx.doi.org/10.1038/emboj.2008.119
- Jacinto E, Lorberg A. TOR regulation of AGC kinases in yeast and mammals. Biochem J 2008; 410:19-37; PMID:18215152; http://dx.doi.org/10.1042/BJ20071518
- Durán RV, Hall MN. Regulation of TOR by small GTPases. EMBO Rep 2012; 13:121-8; PMID:22240970; http://dx.doi.org/10.1038/embor.2011.257
- Laplante M, Sabatini DM. mTOR signaling in growth control and disease. Cell 2012; 149:274-93; PMID:22500797; http://dx.doi.org/10.1016/j.cell.2012.03.017
- Kawai M, Nakashima A, Ueno M, Ushimaru T, Aiba K, Doi H, Uritani M. Fission yeast tor1 functions in response to various stresses including nitrogen starvation, high osmolarity, and high temperature. Curr Genet 2001; 39:166-74; PMID:11409178
- Weisman R, Choder M. The fission yeast TOR homolog, tor1+, is required for the response to starvation and other stresses via a conserved serine. J Biol Chem 2001; 276:7027-32; PMID:11096119; http://dx.doi.org/10.1074/jbc.M010446200
- Matsuo T, Kubo Y, Watanabe Y, Yamamoto M. Schizosaccharomyces pombe AGC family kinase Gad8p forms a conserved signaling module with TOR and PDK1-like kinases. EMBO J 2003; 22:3073-83; PMID:12805221; http://dx.doi.org/10.1093/emboj/cdg298
- Hayashi T, Hatanaka M, Nagao K, Nakaseko Y, Kanoh J, Kokubu A, Ebe M, Yanagida M. Rapamycin sensitivity of the Schizosaccharomyces pombe tor2 mutant and organization of two highly phosphorylated TOR complexes by specific and common subunits. Genes Cells 2007; 12:1357-70; PMID:18076573; http://dx.doi.org/10.1111/j.1365-2443.2007.01141.x
- Weisman R, Roitburg I, Schonbrun M, Harari R, Kupiec M. Opposite effects of tor1 and tor2 on nitrogen starvation responses in fission yeast. Genetics 2007; 175:1153-62; PMID:17179073; http://dx.doi.org/10.1534/genetics.106.064170
- Ikeda K, Morigasaki S, Tatebe H, Tamanoi F, Shiozaki K. Fission yeast TOR complex 2 activates the AGC-family Gad8 kinase essential for stress resistance and cell cycle control. Cell Cycle 2008; 7:358-64; PMID:18235227; http://dx.doi.org/10.4161/cc.7.3.5245
- Otsubo Y, Yamamato M. TOR signaling in fission yeast. Crit Rev Biochem Mol Biol 2008; 43:277-83; PMID:18756382; http://dx.doi.org/10.1080/10409230802254911
- Tatebe H, Morigasaki S, Murayama S, Zeng CT, Shiozaki K. Rab-family GTPase regulates TOR complex 2 signaling in fission yeast. Curr Biol 2010; 20:1975-82; PMID:21035342; http://dx.doi.org/10.1016/j.cub.2010.10.026
- Matsuo T, Otsubo Y, Urano J, Tamanoi F, Yamamoto M. Loss of the TOR kinase Tor2 mimics nitrogen starvation and activates the sexual development pathway in fission yeast. Mol Cell Biol 2007; 27:3154-64; PMID:17261596; http://dx.doi.org/10.1128/MCB.01039-06
- Brunn GJ, Hudson CC, Sekulić A, Williams JM, Hosoi H, Houghton PJ, Lawrence JC Jr, Abraham RT. Phosphorylation of the Translational Repressor PHAS-I by the Mammalian Target of Rapamycin. Science 1997; 277:99-101; PMID:9204908; http://dx.doi.org/10.1126/science.277.5322.99
- Noda T, Ohsumi Y. Tor, a Phosphatidylinositol Kinase Homologue, Controls Autophagy in Yeast. J Biol Chem 1998; 273:3963-6; PMID:9461583; http://dx.doi.org/10.1074/jbc.273.7.3963
- Powers T, Walter P. Regulation of ribosome biogenesis by the rapamycin-sensitive TOR-signaling pathway in Saccharomyces cerevisiae. Mol Biol Cell 1999; 10:987-1000; PMID:10198052
- Ma XM, Blenis J. Molecular mechanisms of mTOR-mediated translational control. Nat Rev Mol Cell Biol 2009; 10:307-18; PMID:19339977; http://dx.doi.org/10.1038/nrm2672
- Hosokawa N, Hara T, Kaizuka T, Kishi C, Takamura A, Miura Y, Iemura S, Natsume T, Takehana K, Yamada N. Nutrient-dependent mTORC1 association with the ULK1-Atg13-FIP200 complex required for autophagy. Mol Biol Cell 2009; 20:1981-91; PMID:19211835; http://dx.doi.org/10.1091/mbc.E08-12-1248
- Jung CH, Jun CB, Ro SH, Kim YM, Otto NM, Cao J, Kundu M, Kim DH. ULK-Atg13-FIP200 complexes mediate mTOR signaling to the autophagy machinery. Mol Biol Cell 2009; 20:1992-2003; PMID:19225151; http://dx.doi.org/10.1091/mbc.E08-12-1249
- Ganley IG, Lam du H, Wang J, Ding X, Chen S, Jiang X. ULK1.ATG13.FIP200 complex mediates mTOR signaling and is essential for autophagy. J Biol Chem 2009; 284:12297-305; PMID:19258318; http://dx.doi.org/10.1074/jbc.M900573200
- Dibble CC, Manning BD. Signal integration by mTORC1 coordinates nutrient input with biosynthetic output. Nat Cell Biol 2013; 15:555-64; PMID:23728461; http://dx.doi.org/10.1038/ncb2763
- Takahara T, Maeda T. Evolutionarily conserved regulation of TOR signalling. J Biochem 2013; 154:1-10; PMID:23698095; http://dx.doi.org/10.1093/jb/mvt047
- Uritani M, Hidaka H, Hotta Y, Ueno M, Ushimaru T, Toda T. Fission yeast Tor2 links nitrogen signals to cell proliferation and acts downstream of the Rheb GTPase. Genes Cells 2006; 11:1367-79; PMID:17121544; http://dx.doi.org/10.1111/j.1365-2443.2006.01025.x
- Nakashima A, Sato T, Tamanoi F. Fission yeast TORC1 regulates phosphorylation of ribosomal S6 proteins in response to nutrients and its activity is inhibited by rapamycin. J Cell Sci 2010; 123:777-86; PMID:20144990; http://dx.doi.org/10.1242/jcs.060319
- Nakashima A, Otsubo Y, Yamashita A, Sato T, Yamamoto M, Tamanoi F. Psk1, an AGC kinase family member in fission yeast, is directly phosphorylated and controlled by TORC1 and functions as S6 kinase. J Cell Sci 2012; 125:5840-9; PMID:22976295; http://dx.doi.org/10.1242/jcs.111146
- Ikai N, Nakazawa N, Hayashi T, Yanagida M. The reverse, but coordinated, roles of Tor2 (TORC1) and Tor1 (TORC2) kinases for growth, cell cycle and separase-mediated mitosis in Schizosaccharomyces pombe. Open Biol 2011; 1:110007; PMID:22645648; http://dx.doi.org/10.1098/rsob.110007
- Shiota C, Woo JT, Lindner J, Shelton KD, Magnuson MA. Multiallelic disruption of the rictor gene in mice reveals that mTOR complex 2 is essential for fetal growth and viability. Dev Cell 2006; 11:583-9; PMID:16962829; http://dx.doi.org/10.1016/j.devcel.2006.08.013
- Jacinto E, Facchinetti V, Liu D, Soto N, Wei S, Jung SY, Huang Q, Qin J, Su B. SIN1/MIP1 maintains rictor-mTOR complex integrity and regulates Akt phosphorylation and substrate specificity. Cell 2006; 127:125-37; PMID:16962653; http://dx.doi.org/10.1016/j.cell.2006.08.033
- Oh WJ, Jacinto E. mTOR complex 2 signaling and functions. Cell Cycle 2011; 10:2305-16; http://dx.doi.org/10.4161/cc.10.14.16586
- Hoffman CS, Winston F. Isolation and characterization of mutants constitutive for expression of the fbp1 gene of Schizosaccharomyces pombe. Genetics 1990; 124:807-16; PMID:2157626
- Hoffman CS, Winston F. Glucose repression of transcription of the Schizosaccharomyces pombe fbp1 gene occurs by a cAMP signaling pathway. Genes Dev 1991; 5:561-71; PMID:1849107
- Byrne SM, Hoffman CS. Six git genes encode a glucose-induced adenylate cyclase activation pathway in the fission yeast Schizosaccharomyces pombe. J Cell Sci 1993; 105 ( Pt 4):1095-100; PMID:8227198
- Nocero M, Isshiki T, Yamamoto M, Hoffman CS. Glucose repression of fbp1 transcription of Schizosaccharomyces pombe is partially regulated by adenylate cyclase activation by a G protein α subunit encoded by gpa2 (git8). Genetics 1994; 138:39-45; PMID:8001792
- Egel R. in Molecular biology of the fission yeast 31-73 (Academic Press, San Diego, 1989)
- Álvarez B, Moreno S. Fission yeast Tor2 promotes cell growth and represses cell differentiation. J Cell Sci 2006; 119:4475-85; PMID:17046992; http://dx.doi.org/10.1242/jcs.03241
- Shiozaki K, Russell P. Conjugation, meiosis, and the osmotic stress response are regulated by Spc1 kinase through Atf1 transcription factor in fission yeast. Genes Dev 1996; 10:2276-88; PMID:8824587
- Mukaiyama H, Kajiwara S, Hosomi A, Giga-Hama Y, Tanaka N, Nakamura T, Takegawa K. Autophagy-deficient Schizosaccharomyces pombe mutants undergo partial sporulation during nitrogen starvation. Microbiology 2009; 155:3816-26; PMID:19778961; http://dx.doi.org/10.1099/mic.0.034389-0
- Takahara T, Maeda T. TORC1 of fission yeast is rapamycin-sensitive. Genes Cells 2012; 17:698-708; PMID:22762302; http://dx.doi.org/10.1111/j.1365-2443.2012.01618.x
- Hilti N, Baumann D, Schweingruber AM, Bigler P, Schweingruber ME. Gene ste20 controls amiloride sensitivity and fertility in Schizosaccharomyces pombe. Curr Genet 1999; 35:585-92; PMID:10467002
- Pluskal T, Hayashi T, Saitoh S, Fujisawa A, Yanagida M. Specific biomarkers for stochastic division patterns and starvation-induced quiescence under limited glucose levels in fission yeast. FEBS J 2011; 278:1299-315; PMID:21306563; http://dx.doi.org/10.1111/j.1742-4658.2011.08050.x
- Weindruch R, Keenan KP, Carney JM, Fernandes G, Feuers RJ, Floyd RA, Halter JB, Ramsey JJ, Richardson A, Roth GS. Caloric restriction mimetics: metabolic interventions. J Gerontol A Biol Sci Med Sci 2001; 56 Spec No 1:20-33; PMID:12088209
- Chen CH, Kiyan V, Zhylkibayev AA, Kazyken D, Bulgakova O, Page KE, et al. Autoregulation of the mechanistic target of rapamycin (mTOR) complex 2 integrity is controlled by an ATP-dependent mechanism. J Biol Chem 2013; 288:27019-30; PMID:23928304; http://dx.doi.org/10.1074/jbc.M113.498055
- Hoffman CS. Glucose sensing via the protein kinase A pathway in Schizosaccharomyces pombe. Biochem Soc Trans 2005; 33:257-60; PMID:15667320; http://dx.doi.org/10.1042/BST0330257
- Bergbrede T, Chuky N, Schoebel S, Blankenfeldt W, Geyer M, Fuchs E, Goody RS, Barr F, Alexandrov K. Biophysical analysis of the interaction of Rab6a GTPase with its effector domains. J Biol Chem 2009; 284:2628-35; PMID:19019823; http://dx.doi.org/10.1074/jbc.M806003200
- Whiteman EL, Cho H, Birnbaum MJ. Role of Akt/protein kinase B in metabolism. Trends Endocrinol Metab 2002; 13:444-51; PMID:12431841
- Manning BD, Cantley LC. AKT/PKB signaling: navigating downstream. Cell 2007; 129:1261-74; PMID:17604717; http://dx.doi.org/10.1016/j.cell.2007.06.009
- Sarbassov DD, Ali SM, Sabatini DM. Growing roles for the mTOR pathway. Curr Opin Cell Biol 2005; 17:596-603; PMID:16226444; http://dx.doi.org/10.1016/j.ceb.2005.09.009
- Toschi A, Lee E, Xu L, Garcia A, Gadir N, Foster DA. Regulation of mTORC1 and mTORC2 complex assembly by phosphatidic acid: competition with rapamycin. Mol Cell Biol 2009; 29:1411-20; PMID:19114562; http://dx.doi.org/10.1128/MCB.00782-08
- Gan X, Wang J, Su B, Wu D. Evidence for direct activation of mTORC2 kinase activity by phosphatidylinositol 3,4,5-trisphosphate. J Biol Chem 2011; 286:10998-1002; PMID:21310961; http://dx.doi.org/10.1074/jbc.M110.195016
- Zinzalla V, Stracka D, Oppliger W, Hall MN. Activation of mTORC2 by association with the ribosome. Cell 2011; 144:757-68; PMID:21376236; http://dx.doi.org/10.1016/j.cell.2011.02.014
- Dennis PB, Jaeschke A, Saitoh M, Fowler B, Kozma SC, Thomas G. Mammalian TOR:a homeostatic ATP sensor. Science 2001; 294:1102-5; PMID:11691993; http://dx.doi.org/10.1126/science.1063518
- Cohen A, Kupiec M, Weisman R. Glucose activates TORC2-Gad8 via positive regulation of the cAMP/PKA pathway and negative regulation of the Pmk1-MAPK pathway. J Biol Chem 2014; PMID:24928510; http://dx.doi.org/10.1074/jbc.M114.573824
- Hálová L, Du W, Kirkham S, Smith DL, Petersen J. Phosphorylation of the TOR ATP binding domain by AGC kinase constitutes a novel mode of TOR inhibition. J Cell Biol 2013; 203:595-604; PMID:24247430; http://dx.doi.org/10.1083/jcb.201305103
- Bähler J, Wu JQ, Longtine MS, Shah NG, McKenzie A, Steever AB, Wach A, Philippsen P, Pringle JR. Heterologous modules for efficient and versatile PCR-based gene targeting in Schizosaccharomyces pombe. Yeast 1998; 14:943-51; PMID:9717240; http://dx.doi.org/10.1002/(SICI)1097-0061(199807)14: 10<943::AID-YEA292>3.0.CO;2-Y
- Alfa C, Fantes P, Hyams J, McLeod M Warbrick E Experiments with fission yeast : a laboratory course manual (Cold Spring Harbor Laboratory Press, Plainview, NY, 1993)
- Tatebe H, Shiozaki K. Identification of Cdc37 as a novel regulator of the stress-responsive mitogen-activated protein kinase. Mol Cell Biol 2003; 23:5132-42; PMID:12861001
- Basi G, Schmid E, Maundrell K. TATA box mutations in the Schizosaccharomyces pombe nmt1 promoter affect transcription efficiency but not the transcription start point or thiamine repressibility. Gene 1993; 123:131-6; PMID:8422997
- Hatanaka M, Shimoda C. The cyclic AMP/PKA signal pathway is required for initiation of spore germination in Schizosaccharomyces pombe. Yeast 2001; 18:207-17; PMID:11180454; http://dx.doi.org/10.1002/1097-0061(200102)18: 3<207::AID-YEA661>3.0.CO;2-I
- Maeda T, Mochizuki N, Yamamoto M. Adenylyl cyclase is dispensable for vegetative cell growth in the fission yeast Schizosaccharomyces pombe. Proc Natl Acad Sci U S A 1990; 87:7814-8; PMID:2172964
- Tatebe H, Shiozaki K. Rab small GTPase emerges as a regulator of TOR complex 2. Small GTPases 2010; 1:180-2; PMID:21625337; http://dx.doi.org/10.4161/sgtp.1.3.14936
- Maeda T, Watanabe Y, Kunitomo H, Yamamoto M. Cloning of the pka1 gene encoding the catalytic subunit of the cAMP-dependent protein kinase in Schizosaccharomyces pombe. J Biol Chem 1994; 269:9632-7; PMID:8144551
- Isshiki T, Mochizuki N, Maeda T, Yamamoto M. Characterization of a fission yeast gene, gpa2, that encodes a G α subunit involved in the monitoring of nutrition. Genes Dev 1992; 6:2455-62; PMID:1340462